The peripheral T-cell lymphoma: can we pivot from the chemotherapy-predicated paradigm?
Abstract
Peripheral T-cell lymphomas (PTCL) are uncommon and aggressive diseases that are difficult to study. Combination chemotherapy such as cyclophosphamide, doxorubicin, vincristine, and prednisone has been the mainstay of treatment for almost 30 years, but outcomes remain poor. The development of new targeted therapies is changing the landscape of how we treat patients with these difficult diseases. For instance, the addition of brentuximab vedotin to combination chemotherapy enhanced the outcomes in patients with CD30-positive anaplastic large cell lymphomas, but there is still a need for better therapies in the other numerous subtypes. Here we discuss the data for the existing treatment paradigm of PTCL as well as the merits of shifting toward a chemotherapy-free approach.
Keywords
INTRODUCTION
Peripheral T-cell lymphomas (PTCL) are a group of diseases that are both very heterogeneous and rare, making it a significant challenge to advance care for patients affected by these diseases. The most recent World Health Organization (WHO) classification recognizes 29 distinct types, with the cell-of-origin of these non-Hodgkin lymphomas (NHL) arising from post-thymic T- and natural killer (NK) cells[1]. Due to such a wide range in subtypes, clinical manifestations, and response to therapies, clinical trials are often limited by small numbers and lack of representation for the whole spectrum of disease. Adding to the complexity, prevalence also varies geographically, suggesting both genetic and environmental factors. In North America, the most frequent subtypes are PTCL not otherwise specified (NOS), angioimmunoblastic T-cell lymphoma (AITL), and ALK-positive anaplastic large cell lymphoma (ALCL), at 34%, 16%, and 16%, respectively[2]. In Europe, the most diagnosed subtypes are PTCL NOS, AITL, and ALK-negative ALCL, making up 34%, 29%, and 9% of the cases, respectively[3]. In Asia, however, the most common subtypes are adult T-cell leukemia/lymphoma (ATLL), PTCL NOS, and NK/T-cell lymphoma (NKTCL) at 25%, 22%, and 22% of PTCL cases, respectively[3]. With the exception of ALK-positive ALCL, the PTCL carry a poor prognosis with the 5-year overall survival (OS) ranging from 14% in ATLL, 20% for enteropathy-type, 32% for AITL and PTCL NOS, to 59% in ALK-negative ALCL and 70% in ALK-positive ALCL[3].
The poor outcomes are broadly related to intrinsic chemo-resistance, with at least 15%-20% of cases that are primary refractory to frontline treatment and short progression-free survival (PFS) in patients who do initially respond. Based on the very disappointing results of standard chemotherapy in the majority of PTCL subtypes, excluding ALK-positive ALCL, it should not come as a surprise that one additional novel agent combined with the chemotherapy backbone has often yielded disappointing results[4-6]. In addition, retrospective data have demonstrated that patients who received novel drugs had improved outcomes when compared to those who were never treated with targeted agents[7,8], further suggesting that combinations of novel agents could yield the future treatment for patients with PTCL. In this review, based on the intrinsic characteristics of these diseases, we present the merits of shifting to a chemotherapy-free paradigm.
THE MOLECULAR DRIVERS OF PTCL
In T-cell lymphomagenesis, the molecular pathways are poorly understood given the wide array of clinical and biologic presentations associated with so many subtypes, but despite these challenges, it has been possible to identify some recurring themes. We describe below the more prominent genetic and epigenetic aberrations described in PTCL NOS and AITL.
Mutation in epigenetic factors
Epigenetics refer to clonal changes in the pattern of gene expression without alterations in the primary genetic sequence. Over the last 10 years, it has become evident that PTCL are characterized by gross epigenetic dysregulation. The first suggestion that epigenetic pathways were relevant in the pathogenesis of PTCL comes from the clinic, where PTCL exhibit a consistent sensitivity to the histone deacetylase inhibitors (HDAC). PTCL are in fact the only malignant disease for which we have 4 HDAC inhibitors approved as monotherapy around the world. Only about thirty percent of patients can expect a response to HDAC inhibitors, but the duration of response that patients may experience is indeed remarkable. For example, cutaneous T-cell lymphomas (CTCL), for which HDAC inhibitors were first approved, are diseases currently being redefined and reclassified based on their genetic, epigenetic, and transcriptional changes landscape[9,10].
The epigenetic machinery is responsible for maintaining the adaptative transcriptional memory of normal T-cells, and this improves the efficiency of T-helper subsets to transcribe inducible genes when needed[11,12]. Dysregulation of epigenetic modulation likely contributes to the progression of normal T-cells in the development of PTCL, especially with recurrent reports of mutations in genes involved in methylation, acetylation, and other epigenetic functions[13-16]. These mutations may lead to hypomethylation across the genome to silence tumor suppressor genes, especially in specific subtypes including AITL and PTCL with
Mutations in tet methylcytosine dioxygenase 2 (TET2), which encodes a methylcytosine dioxygenase that is important in normal cell function, are commonly reported in myeloid diseases but are also seen in lymphoid malignancies: 2% of B-cell lymphomas and almost 12% of PTCL including 33% of AITL[17]. TET2 mutations with and without DNA methyltransferase 3 alpha (DNMT3A) mutations can be found in non-tumor DNA in some PTCL patients, signifying that clonal hematopoiesis may contribute to pathogenesis[18-20]. TET2 mutations are common and may occur in almost half of AITL and over one-third of PTCL-NOS cases[21]. A second study showed three-quarters of patients with AITL have mutations in TET2, with two-thirds of these cases with more than one mutation in the gene[22]. Preclinical studies have demonstrated a causal relationship between mutations in TET2 and the development of both lymphoid and myeloid cancers[17]. Murine models with mutated TET2 demonstrated TFH cell proliferation and progression to PTCL with TFH phenotype after a prolonged latency, possibly due to dysregulation of both cytokine expression and T-cell differentiation[23,24]. Mutations in TET2 have been found in patients without malignancies, related to an entity named clonal hematopoiesis of indeterminate potential (CHIP), which highlights that aberrancies in TET2 are not enough for transformation but can predispose the cells to transform into diverse malignancies upon acquisition of secondary genetic hits.
DNA methyltransferase 3 alpha (DNMT3A) encodes a DNA methyltransferase and is found to be mutated in 10%-40% of AITL cases[22]. Alterations in DNMT3A are thought to be the initial event in malignant transformation and often occur with mutations in TET2[22,25]. Murine models demonstrate loss-of-function mutations in both TET2 and DNMT3A lead to lymphomagenesis[26].
Finally, isocitrate dehydrogenase-2 (IDH2) encodes a mitochondrial enzyme that is involved in
Mutations in RhoA
RHOA is part of the Rho family of GTPases and is important in signaling pathways, such as cellular responses and cell motility[30-32]. The role of RHOA in tumorigenesis is controversial, but cell-type-specific functions of RHOA have recently been better defined by gene targeting techniques. Previously, there was little understanding of RHOA in cancer pathogenesis until RHOA mutations were repeatedly reported in AITL and PTCL NOS TFH subtypes[14,33,34]. The RHOA mutations are unique to these subtypes of PTCL, where glycine at the 17th position is replaced by valine (G17V). RHOA G17V did not bind GTP like its normal counterpart, but rather, it inhibited the GTP binding to wild-type RHOA. The exact biochemical role of RHOA in T-cell signaling, however, is far from resolved. As described above, TET2 is the most frequently mutated gene in AITL, and these tumors are commonly found to occur with RHOA G17V[14,33]. Multiple transgenic murine models with RHOA G17V have demonstrated increased TFH proliferation as well as an overly reactive response to T-cell receptor (TCR) stimuli[35,36]. Coupling RHOA G17V expression with TET2 mutations resulted in the development of PTCL with TFH phenotype, i.e., AITL[36,37]. Adding evidence to the clonal hematopoiesis story, mice with defects in both TET2 and RHOA developed myeloid tumors before seven months but tended to produce T-cell lymphomas after this time[35]. One possibility, suggested by the experimental evidence described above, is that loss-of-function mutation in epigenetic factors, e.g., TET2, is an early event in the process of transformation that requires a second and final hit in RHOA G17V to induce cell differentiation toward TFH and the development of AITL.
VAV1 encodes a hematopoietic-specific Rho family-specific guanine exchange factor and is found to be mutated in up to 10% of AITL and PTCL-NOS patients[38]. These mutations generally include recurrent frame deletion that is generated by alternative splicing and multiple VAV1 gene fusions. Mutations in RHOA and VAV1 are mutually exclusive, suggesting that they could be affecting the same genetic pathway[39].
Mutations in T-cell receptor pathway genes
Defects in TCR signaling can result in aberrant activation, differentiation, and proliferation of T-cells. AITL or PTCL-NOS with a TFH phenotype specimens have recurrent mutations in T-cell receptor signaling including phospho-lipase C gamma 1 (PLCG1), CD28, phosphoinositide-3-kinase (PI3K) regulatory and catalytic subunits as well as other genes[40]. Activating TCR alterations seemed to be adequate to drive malignant transformation since these cases rarely had more than one TCR gene mutation. TCR dysregulation was also associated with activation of NF-κB, leading to cellular proliferation. CD28 mutations were reported in both AITL and PTCL NOS subtypes and was found to bind its ligand more tightly than wild-type CD28, which presumably is responsible for its activating ability[41]. Interestingly, CD28-mutated AITL patients have worse survival compared to wild-type cases[41]. The most common mutation in PLCG1 p.Ser345Phe interferes with the enzymatic catalytic domain to increase NF-κB/NFAT activity and, interestingly, is also found in CTCL, suggesting a shared mechanism of disease[42].
Other insights into the genetic drivers of PTCL NOS
Consistent with the heterogeneous presentations of the PTCL, there is a variety of mutations reported to describe the diversity. In addition to the molecular drivers of disease described above, chromosomal translocations may offer insight into oncogenic pathways and potential therapeutic targets. The translocation t(5;9)(q33;q22) between interleukin-2-inducible T-cell kinase (ITK) and spleen tyrosine kinase (SYK) has been reported in 17% of PTCL NOS and 18% of PTCL NOS TFH patients[43,44]. By immunohistochemistry, the majority (94%) of PTCL samples overexpress SYK[45]. A mechanistic murine model demonstrated that overexpression of an ITK-SYK fusion gene is sufficient to activate TCR signaling and drive the production of T-cell lymphomas[44,46]. Other novel kinase fusion genes have been described and warrant further investigation[38]. Recent analysis of a large PTCL NOS cohort has identified additional altered genes, such as YTHDF2 and PD1, and even more interestingly, a novel subtype characterized by TP53 and/or CDKN2A mutations and deletions has been described and associated, not surprisingly, with a worse prognosis[47].
In summary, the implementation of high-throughput genomic techniques in the field of PTCL has brought significant improvement in our understanding of the molecular complexity and genetic drivers of the disease. The identification of recurrently deregulated pathways has paved the way to the development of more rationally planned drug development and to the design of early phase clinical trials aiming to target these pathways that will hopefully yield better clinical outcomes for patients with PTCL.
STANDARDS OF CARE
Cyclophosphamide, doxorubicin, vincristine, and prednisone
Combination chemotherapy with cyclophosphamide, doxorubicin, vincristine, and prednisone (CHOP) is currently considered the standard of care based on a large, randomized phase 3 study led by Fisher et al. that explored the value of different intensified chemotherapy regimens compared to CHOP in patients with intermediate- and high-grade NHL that included both B-cell and T-cell lymphomas[48]. End points of the study were overall response rate (ORR), time to treatment failure (TTF), disease-free survival (DFS), overall survival (OS), and incidence of severe or life-threatening toxicity. This landmark trial showed no difference in ORR, PFS, and DFS, but patients treated with CHOP developed fewer adverse events. At the time of the study, there was no standardized differentiation between aggressive B-cell and T-cell lymphomas, and CHOP chemotherapy has become the default standard of therapy for both subtypes for the past 30 years. PTCL patients treated with doxorubicin-based chemotherapy have a 3-year PFS of 32% and overall survival (OS) of 55%[49]. Since then, there has been better characterization of lymphomas, e.g., diffuse large B-cell lymphoma (DLBCL) and addition of anti-CD20 monoclonal antibody rituximab with improvement in survival, but much less progress has been made for the T-cell lymphomas compared to the B-cell counterpart.
Cyclophosphamide, doxorubicin, vincristine, etoposide, and prednisone
Adding more chemotherapy to the CHOP backbone has been studied as a means to improve outcomes. In a secondary analysis of the German High-Grade NHL Study Group trials, the addition of etoposide to standard CHOP chemotherapy has been shown to improve the 3-year event-free survival (EFS) to 75% from 51% with CHOP alone (P = 0.003) in patients younger than 60 with normal lactate dehydrogenase though there was not a significant difference in OS[50]. This finding is mostly because of treatment effect on patients with ALK-positive ALCL, where treatment with Cyclophosphamide, doxorubicin, vincristine, etoposide, and prednisone (CHOEP) was associated with a 3-year EFS of 91% compared to 57% when treated with CHOP (P = 0.012); the effect was no longer significant in other subtypes of PTCL[50]. An additional retrospective study of Danish and Swedish patients with ALK-positive ALCL treated with CHOEP was associated with better PFS (hazard ratio [HR] 0.48, P = 0.034) and OS (HR 0.34, P = 0.008) compared to treatment with CHOP[51]. In a meta-analysis performed in Asian patients, however, the addition of etoposide did not improve response rates when compared to CHOP[52].
Infusional etoposide, doxorubicin, and vincristine with cyclophosphamide and prednisone
Given evidence of multidrug resistance by expression in relapsed and refractory lymphoma, prolonged exposure to doxorubicin and vincristine over a 4-day infusion may overcome resistance mechanism compared to the 1-day infusion used in CHOP[53]. In the phase II study of dose-adjusted Infusional etoposide, doxorubicin, and vincristine with cyclophosphamide and prednisone (EPOCH), 41 previously untreated patients with PTCL (excluding ATLL) were treated, leading to an ORR of 78% (with 61% achieving a complete response [CR]). The most common grade 3 or higher adverse events included neutropenia (75%), anemia (41%), and thrombocytopenia (22%). After a median follow-up of 2 years, the median PFS and OS were not reached. The 2-year PFS and OS were 53% and 73%, respectively. The decision to choose CHOP, CHOEP, or EPOCH is usually dependent on the provider and/or institution.
Brentuximab vedotin plus CHP
Adding brentuximab vedotin (an antibody drug conjugate linking a monoclonal antibody against cluster of differentiation 30 (CD30) and cytotoxic agent, monomethyl auristatin E [MMAE], Bv) to cyclophosphamide, doxorubicin, and prednisone (Bv-CHP) compared to CHOP in the phase 3 frontline ECHELON-2 trial met its primary endpoint and was the first regimen to get FDA approval in frontline CD30-positive (defined as 10% of more expression by immunohistochemistry) PTCL[6]. The median progression-free survival (PFS) was 48.2 months and 20.8 months after a median follow-up of 36.2 months in the Bv-CHP and CHOP arms, respectively. The median OS was not reached for either group, but the hazard ratio was 0.66 (95%CI: 0.46-0.95), P = 0.0244. The ORR and CR rate for the Bv-CHOP and CHOP arms were 83% and 68% vs. 72% and 56%, respectively. Both groups had similar rates of adverse events (AE), grade 3 or higher AE, and serious AE. This is now the standard of care in patients with CD30-positive PTCL, though the trial was enriched for ALCL and not sufficiently powered for other subtypes. The 5-year update demonstrated that after a median follow-up of 47.6 months, the median PFS was 62.3 months vs. 22.8 months and median OS was not reached vs. 54 months in the Bv-CHP and CHOP arms, respectively[54]. A multicenter study is now recruiting to study Bv-CHP in patients with untreated PTCL with less than 10% CD30 expression (NCT04569032).
Does adding a novel agent to CHOP-like regimens improve outcomes?
Save the addition of brentuximab vedotin in CD30-positive PTCL, the treatment for PTCL has not shared the same advancement of B-cell lymphomas with the addition of the CD20 monoclonal antibody rituximab despite many recent attempts to add additional targeted agents FDA-approved in the relapsed or refractory (R/R) setting to the CHOP-backbone in the past 5 years[55].
Pralatrexate plus CHOP-like Combinations
Pralatrexate was the first drug approved for patients with relapsed or refractory PTCL. It is a folate analog that binds the reduced folate carrier (RFC), leading to the inhibition of dihydrofolate reductase (DHFR) intracellularly[56]. PROPEL was an international single-arm Phase 2 study conducted in patients with R/R PTCL that led to the US FDA approval in 2009[57]. Following cyclophosphamide, etoposide, vincristine, and prednisone (COEP) on day 1 of each cycle, pralatrexate was added on days 15, 22, and 29 in a phase 2 study to treat 33 patients with untreated PTCL[58]. The ORR was 70% (CR 50%). After a median follow-up of 21.5 months, the estimated 2-year PFS and OS were 64% and 80%, respectively. Common grade 3 or 4 AE included anemia (27%), febrile neutropenia (18%), and mucositis (18%). This combination did not demonstrate improvement in outcomes seen with CHOP and was not deemed a regimen that warranted additional exploration by the authors.
The sequential addition of pralatrexate followed by COEP did not improve outcomes compared to CHOP, so the folate analog metabolic inhibitor was added to CHOP (Fol-CHOP) in a phase I study (NCT02594267)[5]. The study was completed in October 2020, but the results are not readily available at the time of writing this manuscript.
Romidepsin plus CHOP (Ro-CHOP)
It has been a decade since romidepsin, a histone deacetylase (HDAC) inhibitor, was approved in some countries based on the phase II study in patients with R/R PTCL by Coiffier et al.[59-61]. The phase III Ro-CHOP study (NCT01796002) comparing CHOP with CHOP plus romidepsin in patients with untreated PTCL, excluding ALK-positive ALCL, was a highly anticipated trial. Unfortunately, the clinical trial revealed that the addition of romidepsin did not improve ORR, PFS, or OS compared to CHOP alone[4]. After a median follow up of almost 28 months, the study failed to meet its primary endpoint with a median PFS of 12.0 months compared to 10.2 months in the Ro-CHOP and CHOP groups, respectively. The hazard ratio of 0.81 was not significant (P = 0.096)[4]. The secondary endpoints of overall response rate (ORR) and OS were also not statistically different, but the addition of HDAC inhibitor, however, was associated with more treatment-emergent adverse events related to cytopenias and gastrointestinal side effects. This led to the withdrawal of romidepsin’s PTCL indication in August 2021, though appeals are pending.
Romidepsin was also added to CHOEP but the trial was stopped because there was no improvement in the primary endpoint of PFS[62].
Azacytidine and CHOP
Given the enrichment of mutations related to the epigenetic machinery in AITL and PTCL NOS TFH, an oral hypomethylating agent (HMA), azacytidine was added to the CHOP backbone in a phase II study that prioritized PTCL TFH subtypes (NCT03542266). At a median follow up of 21 months, the CR was 75% overall (n = 20) and 88% in patients with TFH subtypes (n = 17)[63]. The 2-year OS was 68% for the entire cohort and 76% for TFH subtypes, with an estimated median PFS of 36 months[64]. In addition, the TET2 mutations were associated with CR (P = 0.007), favorable PFS (P = 0.004), and OS (P = 0.015) in contrast to DNMT3A mutations, which were associated with a worse PFS (P = 0.016)[63]. The biomarker approach may be a promising way to decide which patient gets the right treatment. Longer follow-up is needed to determine the impact on survival.
A phase II cooperative group study is testing the addition of duvelisib or azacytidine to CHOP with or without etoposide in patients with CD30-negative PTCL (NCT04803201).
Alemtuzumab plus CHOP
The phase 3 Act-2 trial added alemtuzumab (a monoclonal antibody targeting CD52) to CHOP in older patients with untreated PTCL and also failed to demonstrate improvement compared to CHOP alone[64]. There was no statistically significant benefit in ORR, EFS, PFS, or OS, but there were higher rates of toxicity, including grade 3 or higher infections (40% vs. 21%)[64]. The planned sample sizes for elderly and younger cohorts were not reached due to poor accrual.
Lenalidomide plus CHOP
Lenalidomide, an oral immune modulator that acts by inhibiting cereblon, was added to 8 cycles of CHOP chemotherapy as frontline therapy to treat older patients with AITL in a phase 2 trial[65]. Patients also received 4 injections of intrathecal methotrexate during the study. The ORR was 56% (CR 41%), which was not better than CHOP alone in historic controls (e.g., ECHELON-2 study)[6,65]. After a median follow-up of 45 months, the 2-year PFS and OS were 42% and 59%, respectively. The most common AE was neutropenia, with 71% of patients developing grade 4 neutropenia. In addition, 23% developed grade 3 or 4 infections and 5% succumbed to grade 5 sepsis. The authors did not believe the results warranted the addition of lenalidomide to CHOP in this subset of patients. Lenalidomide is being studied with CHOP-like therapies in different populations, both with CHOP (NCT04922567) and CHOEP in the frontline setting (NCT02561273) with results pending.
Nivolumab and EPOCH
A multicenter pilot study investigating nivolumab with EPOCH showed that 78% of 18 patients enrolled developed immune related adverse events (irAEs), and 44% required discontinuation of nivolumab due to irAEs[66]. The trial protocol permitted patients to receive one cycle of chemotherapy prior to enrollment, and interestingly, patients who received one cycle of EPOCH prior to the addition of nivolumab to subsequent cycles did not experience dose-limiting irAEs. These data suggest pre-treatment with chemotherapy may result in less toxicity. Of note, the ORR was 83% at the end of induction and 2 patients proceeded with a consolidative autologous stem cell transplant[66].
Ongoing “CHOP-like plus” Trials
There are still many studies looking to add targeted agents to combination chemotherapy and results are pending. In China, a phase 3 study comparing decitabine plus CHOP vs. CHOP is ongoing (NCT03553537). The study began in 2015, was completed in 2020, and is pending analysis and publication of the results. Two phase II frontline studies investigate the safety and efficacy of adding chidamide, an HDAC inhibitor, or apatinib, a multitargeted kinase inhibitor, to CHOP in China (NCT03268889 and NCT03631862, respectively). Table 1 summarizes completed and ongoing trials studying CHOP-like regimens with and without targeted therapies.
CHOP plus trials in Untreated PTCL
Phase | Population | Study drugs | Primary endpoint | Result | Reference |
3 | Untreated CD30-positive PTCL | Bv-CHP vs. CHOP (ECHELON-2) | PFS, met | Median PFS Bv-CHP 48.2 months vs. CHOP 20.8 months, HR 0.71 P = 0.0110 | [6] |
2 | Untreated PTCL with | Bv-CHP | ORR | Recruiting | NCT04569032 |
2 | Untreated patients with PTCL-NOS, AITL, ALCL | Sequential COEP followed by pralatrexate | CR, met | CR 51% (goal CR > 40%), but 2-year PFS and OS are not better than historic controls | [58] |
1 | Untreated PTCL | Pralatrexate + CHOP | MTD | Study completed 8 Oct 2020, data unavailable | [5] |
3 | Untreated PTCL, excluding ALK-positive ALCL | Ro-CHOP vs. CHOP | PFS, not met | Median PFS Ro-CHOP 12.0 months vs. CHOP 10.2 months, HR 0.81 P = 0.0962 | [4] |
2 | Untreated PTCL, excluding ALK-positive ALCL | Romidepsin plus CHOEP | 18-month PFS, not met | 18-month PFS 48% (goal 70%) | [62] |
2 | Untreated PTCL | Chidamide + CHOP vs. CHOP | CR | Results pending | NCT03268889 |
2 | Untreated PTCL | Chidamide + CHOEP and ASCT | 2-year PFS | Results pending | NCT02987244 |
2 | Untreated PTCL | Chidamide + CHOPE vs. CHOPE | CR | Results pending | NCT03617432 |
2 | Untreated PTCL, TFH prioritized | Azacytidine and CHOP | CR | CR overall 75%, PTCL-TFH 88% (goal N/A) | [63] |
2 | Untreated PTCL with | Azacytidine or Duvelisib plus CHO(E)P | CR | Recruiting | NCT04803201 |
3 | Untreated PTCL | Decitabine + CHOP vs. CHOP | 3-year PFS | Results pending | NCT03553537 |
1/2 | Untreated PTCL | Nivolumab + EPOCH | Safety, tolerability, ORR | Grade 3/4 IRAE 39%, ORR 83% | [66] |
3 | Untreated PTCL | Alemtuzumab + CHOP vs. CHOP | 3-year EFS, not met | 3-year EFS A-CHOP 32% vs. CHOP 25%, P = 0.159 | [64] |
2 | Untreated older AITL patients | Lenalidomide and CHOP (REVAIL) | CMR, not met | CMR 41% (goal 55%) | [65] |
1/2 | Untreated PTCL | Lenalidomide and CHOP | MTD, safety, ORR | Recruiting | NCT04423926 |
2 | Untreated PTCL | Lenalidomide + CHOP vs. CHOP | ORR | Recruiting | NCT04922567 |
2 | Untreated PTCL | Biomarker targeted treatments plus CHOP vs. CHOP | CR | Recruiting | NCT04480099 |
3 | Untreated PTCL | Apatinib + CHOP vs. CHOP | 2-year PFS and ORR | Results pending | NCT03631862 |
2 | Untreated PTCL | Tenalisib + CHOP | CR | Not yet recruiting | NCT05239910 |
THE MANY NEW DRUGS COMING ALONG IN PTCL
Approved agents
About 70% of patients with PTCL treated with chemotherapy eventually will relapse or have refractory disease, and these patients have particularly poor outcomes. The British Columbia Cancer Agency Lymphoid Cancer found that patients with PTCL who relapse or are refractory to first-line therapy had a short median PFS of 3.1 months and median OS of 5.5 months[67]. Over the past decade, the FDA approved pralatrexate in the United States in 2009, romidepsin for PTCL in the United States in 2011, mogamulizumab for ATLL in Japan in 2012, belinostat in the United States in 2014, brentuximab vedotin in CD30-positive ALCL and mycosis fungoides in the United States in 2014, chidamide in China in 2014 based on registration trials[57,59,68-71]. Treatment with novel agents in the relapsed and refractory setting is associated with improved survival compared to additional, less efficacious chemotherapy[7,8]. In August 2021, romidepsin was withdrawn from the indication, despite the efficacy of novel combinations. Over the past 5 years, pralatrexate has been approved in Japan and China, romidepsin, forodesine, and mogamulizumab were approved for the treatment of R/R PTCL in Japan, and numerous other targeted agents have been studied worldwide.
Pralatrexate
As previously mentioned, pralatrexate was the first novel agent approved in the treatment of R/R PTCL in 2009 based on the results from the phase 2 registration PROPEL study[57]. The ORR in these patients was 29%, and treatment was associated with a median duration of response (DOR) of 12.4 months[57]. In the past 5 years, pralatrexate has been studied around the world and has obtained additional FDA approvals in Asia. In two phase II registration studies in Japan and China, pralatrexate was studied in R/R PTCL, resulting in ORRs of 45%-52% (CR 9%-10%) in patients from both cohorts[72,73]. The most common AEs included mucositis (68%-88%), thrombocytopenia (37%-68%), and liver function test abnormalities (41%-64%). Almost half of the patients (48%-49%) developed serious AEs, including febrile neutropenia and pneumonia. A study in Taiwan using pralatrexate in R/R PTCL is also ongoing (NCT03150602). Pralatrexate remains an important option in the treatment of these patients with R/R PTCL.
Romidepsin
Romidepsin was studied in patients with R/R T-cell lymphomas with ORR ranging from 25% to 34%, leading to its approval in R/R CTCL in 2009 and R/R PTCL in 2011[59,74,75]. More recently, the HDAC inhibitor has been studied in 40 Japanese patients with R/R PTCL, leading to FDA approval in Japan[76]. There were no unexpected AEs, and the most common grade 3 or higher AEs included lymphopenia (74%), neutropenia (54%), leukopenia (46%), and thrombocytopenia (38%). The ORR was 43% with 23% of patients achieving a CR, which is better than previous cohorts. In patients with the rarer subtype, extranodal
Belinostat
Belinostat is a hydroxamic acid-derived pan-HDAC inhibitor that was approved in 2014 for the treatment of R/R PTCL because of the phase 2 registration BELIEF trial[69]. Patients enrolled in the study tolerated treatment with the most common grade 3 or 4 adverse events consisting of anemia (11%), thrombocytopenia (7%), neutropenia (6%), and dyspnea (6%)[69]. Similar to other HDAC inhibitors, the ORR was 26% with a median DOR of 13.6 months. In a subset analysis, it was notable that in patients with AITL, the ORR was 46%, suggesting a higher sensitivity to this class of drug[79].
Forodesine
Purine nucleoside phosphorylase (PNP) seems important in T-cell biology, and forodesine is a novel oral PNP inhibitor that has been approved in Japan for the treatment of R/R PTCL[80]. Of 41 evaluable patients, the ORR was 25% (CR 10%). Given the mechanism of action, the AEs were expected with grade 3/4 lymphopenia (96%), leukopenia (42%), neutropenia (35%), and thrombocytopenia (25%). Five patients (3 with AITL, 2 with PTCL NOS) developed secondary B-cell lymphomas. The drug has not been approved elsewhere.
Mogamulizumab
Mogamulizumab is an antibody that targets C-C chemokine receptor 4 (CCR4) and was approved in Japan for R/R PTCL with CCR4 expression (mostly ATLL) almost a decade ago. In order to study other ATLL patient populations, a phase II international study was conducted in 22 centers to compare mogamulizumab and investigator’s choice (IC): pralatrexate, gemcitabine plus oxaliplatin (GemOx), or dexamethasone, cisplatin, and cytarabine (DHAP)[81]. The ORR was 11% (CR 2%) in the mogamulizumab arm compared to 0% in the IC arm. Though treatment was well tolerated, mogamulizumab did not improve outcomes for this difficult-to-treat group of patients. In addition, caution must be taken if mogamulizumab is given to patients prior to allogeneic stem cell transplant because there is an increased risk of graft-versus-host-disease (GVHD)-related mortality, which is normally tempered by CCR4-expressing Tregs that would be inhibited by the drug[82].
Chidamide
In China, chidamide, another HDAC inhibitor, was approved for the treatment of R/R PTCL patients in 2014 based on the trial that produced an ORR of 29% with a median DOR of 10 months[71]. There are many clinical trials studying chidamide-based combinations in the treatment of Chinese patients with PTCL.
Investigational novel agents
Given the need for more efficacious treatments in this difficult group of diseases, there are many new targeted agents being studied as single agents. Below we discuss data from investigational novel agents as single agents and summarize ongoing studies in Table 2.
Novel Therapies in Ongoing Clinical Trials
Phase | Patient population | Drug | Mechanism of action | NCT number |
2 | Untreated PTCL, ineligible for chemotherapy | Brentuximab vedotin, intravenous | Antibody-drug conjugate targeting CD30 | NCT01716806 |
2b | R/R PTCL | HBI-8000, oral | HDAC inhibitor | NCT02953652 |
2 | R/R PTCL | Valemetostat tosylate (DS-3201b), oral | Enhancer of zeste homoglog 1/2 dual inhibitor | NCT04703192 |
2 | R/R PTCL | TQ-B3525, oral | PI3K α/δ inhibitor | NCT04615468 |
2 | R/R PTCL | YY-20394, oral | PI3K δ inhibitor | NCT04705090 |
2 | R/R PTCL | AZD4205, oral | Janus kinase inhibitor | NCT04105010 |
2 | R/R PTCL | AZD4573, intravenous | CDK9 inhibitor | NCT05140382 |
2 | R/R PTCL | Tipifarnib, oral | Farnesyl protein transferase inhibitor | NCT02464228 |
2 | R/R PTCL | F520, intravenous | Anti-PDL1 antibody | NCT04457830 |
2 | R/R malignancies | STI-3031 | Anti-PDL1 antibody | NCT03999658 |
1/2 | R/R PTCL | AK104, intravenous | PD1/CTLA4 bispecific antibody | NCT04444141 |
1/2 | R/R PTCL | Tolinapant (ASTX660), oral | Dual antagonist of cellular and X-linked inhibitors of apoptosis proteins | NCT04362007 |
1/2 | R/R solid tumors or lymphomas with WT TP53 | ALRN-6924 | Dual MDMX and MDM2 inhibitor | NCT02264613 |
2 | R/R PTCL | Fenretinide, intravenous emulsion | Synthetic retinoid derivative | NCT02495415 |
1 | R/R PTCL | nanoFenretinide | Synthetic retinoid derivative | NCT04234048 |
1 | R/R PTCL | CPI-818, oral | Interleukin-2-Inducible T-Cell Kinase Inhibitor | NCT03952078 |
1 | R/R lymphoid malignancies | BP1002 | L-Bcl2 antisense oligonucleotide | NCT04072458 |
1 | R/R solid tumors and lymphomas | SEA-TGT | Inhibitory immune receptor | NCT04254107 |
1 | R/R PTCL | Carfilzomib | Proteosome inhibitor | NCT01336920 |
1 | R/R PTCL TFH | MEDI-570 | Anti-ICOS monoclonal antibody | NCT02520791 |
Azacytidine
The serendipitous discovery of the effectiveness of azacytidine in the treatment of AITL began with the treatment of 2 patients with both AITL and chronic myelomonocytic leukemia who achieved CR[83,84]. Due to recurrent mutations in the epigenetic machinery in AITL and PTCL TFH, azacytidine is a logical choice of therapy and is associated with an ORR of 9/12 (75%) with 6/12 achieving CR[85]. All patients had a TET2 mutation, and some had additional mutations in DNMT3A, IDH2, and RHOA. The median PFS and OS were 15 months and 21 months, respectively. Since then, there have been many clinical studies testing azacytidine in combinations to treat patients with untreated and R/R PTCL.
Phosphatidylinositol 3-kinase (PI3K) inhibitors
Duvelisib, a dual PI3K-δ,γ inhibitor, has been studied in patients with R/R PTCL (NCT03372057) with treatment limited by low CD4 counts[86]. Various doses were studied, but the patients enrolled in the expansion phase received oral duvelisib 75 mg twice daily for 2 cycles, followed by 25 mg twice daily until disease progression or toxicity[87]. The ORR in 78 evaluable patients in the expansion phase was 50%
Immune checkpoint inhibitors
Pembrolizumab is an anti-Programmed cell death protein 1 (PD1) monoclonal antibody and was studied in a phase II trial that resulted in an ORR of 33% in patients with R/R PTCL (n = 15)[89]. Nivolumab, another drug in the same class, was studied in a phase II trial and resulted in an ORR of 33% in patients with R/R PTCL[90]. There were reports of hyperprogression during treatment in 10 out of the 12 patients. Both trials were terminated early due to futility and the latter also cited safety concerns. Nivolumab was also associated with hyperprogression in patients with ATLL, likely due to effects on tumor-infiltrating Tregs after immune checkpoint inhibition[91,92]. Due to concern for hyperprogression in ATLL, a phase 2 multicenter study in R/R PTCL, excluding ATLL, was performed and showed that anti-PD1 antibody geptanolimab was safe without report of hyperprogression[93]. In this study by Shi et al., patients with PDL1 expression of 50% or greater seemed to predict response to treatment with ORR of 53% compared PDL1 expression of < 50% with ORR of 25%[93].
In R/R ENKTCL where there is high Programmed death ligand 1 (PDL1) expression (54% of cases), however, pembrolizumab is associated with an ORR of 6/14 (43%) to 7/7 (100%) with 2/7 (29%) to 5/7 (71%) achieving a CR[94-96]. Given the expression of PDL1 in ENKTCL, avelumab, an anti-PDL1 monoclonal antibody, has been used in a phase 2 trial with R/R ENKTCL[97]. Out of 21 patients, the ORR was 38% (CR 24%) with a median PFS of 2.7 months after a median follow-up of 15.7 months. In this patient population, the trial was terminated because of lower response rate than expected. Avelumab is also being studied alone in a phase 2 trial in R/R PTCL (NCT03046953) as well as in a phase 1 trial in combination with interleukin-15 (NCT03905135).
Immunotherapy agents have been studied in combination to treat PTCL without cases of hyperprogression, warranting further study as later discussed below.
Cerdulatinib
Spleen tyrosine kinase (SYK) and Janus kinase (JAK) are enzymes important in cellular processes and have been found to be important in T-cell lymphomagenesis[98]. Cerdulatinib is a SYK, JAK1, and JAK3 inhibitor that is being studied in R/R lymphomas, including PTCL (NCT01994382). 65 patients with R/R PTCL were treated with cerdulatinib in a phase 2 study, resulting in an ORR of 36.2% in 58 evaluable patients[98]. Of note, the AITL/TFH subtype had a higher ORR of 51.9% (CR 37%) with a median DOR of 12.9 months after a median follow-up of 16.4 months. The most common treatment-emergent grade 3 or higher adverse events were elevation in amylase (23%), anemia (20%), elevation in lipase (18.5%) as well as neutropenia (12%) and sepsis (9.2%)[58]. As we understand disease biology, we can better tailor treatments to optimize efficacy while limiting side effects.
Alisertib
Alisertib is an aurora kinase inhibitor that was randomized against investigator’s choice of pralatrexate, romidepsin, and gemcitabine in the phase III LUMIERE trial in patients with R/R PTCL[99]. Surprisingly, the ORR and CR rates achieved with alisertib compared to comparators were 33% and 18% vs. 45% and 27%, respectively. The median PFS and OS did not differ significantly between the two groups.
Valemetostat
Given known epigenetic dysfunction found in PTCL, valemetostat, a selective inhibitor of enhancer of zeste homolog 1 and 2 (EZH1/EZH2), has been studied in R/R PTCL patients[100,101]. EZH1 and EZH2 catalyze methylation of histone 3 to downregulate gene expression, and dysregulation of the delicate balance of tumor suppression and cell processes may lead to lymphomagenesis[101]. Valemetostat is given orally once a day until disease progression or toxicity, leading to an ORR of 48%[101]. Longer follow-up is needed for these ongoing studies.
NOVEL DRUG COMBINATIONS: COULD THEY FORM THE BASIS OF NEW TREATMENT PLATFORMS?
The track record of adding targeted therapies to CHOP-based chemotherapy has so far mostly been disappointing, and the field is looking to study novel combinations in the treatment of both untreated and R/R PTCL. We discuss the combinations where data are available and list other ongoing trials with novel combination in Table 3.
Novel combinations in ongoing trials
Phase | Patient population | Drugs | Mechanisms of action | NCT number |
2b | R/R PTCL | Azacytidine and romidepsin vs. investigator’s choice | HMA and HDAC inhibitor vs. approved agents | NCT04747236 |
2 | R/R PTCL | Sintilimab and chidamide | PD1 antibody and HDAC inhibitor | NCT04512534 |
2 | R/R PTCL | Sintilimab, chidamide and azacytidine | PD1 antibody, HDAC inhibitor, HMA | NCT04052659 |
1 | R/R NHL | Carfilzomib and belinostat | Proteosome inhibitor and HDAC inhibitor | NCT02142530 |
2 | R/R PTCL | Nivolumab and cabrializumab | PD1 antibody and anti-CSF-1R antibody | NCT03927105 |
2 | R/R PTCL | Ixazomib and romidepsin | Proteosome inhibitor and HDAC inhibitor | NCT03547700 |
1/2 | R/R PTCL | Romidepsin and carfilzomib | HDAC inhibitor and proteosome inhibitor | NCT03141203 |
2 | R/R PTCL | Brentuximab vedotin and pembrolizumab | Antibody-drug conjugate targeting CD30 and PD1 inhibitor | NCT04795869 |
2 | R/R PTCL | Chidamide and lenalidomide | HDAC inhibitor and immune modulator | NCT04329130 |
2 | Untreated PTCL | Azacytidine and chidamide | HMA and HDAC inhibitor | NCT04480125 |
2 | Untreated PTCL | Romidepsin and lenalidomide | HDAC inhibitor and immune modulator | NCT02232516 |
1 | R/R PTCL | Bortezomib and azacytidine | Proteosome inhibitor and HMA | NCT01129180 |
1/2 | R/R PTCL | Pembrolizumab and pralatrexate | PD1 inhibitor and antifolate | NCT03598998 |
1/2 | R/R PTCL | Durvalumab and lenalidomide | PDL1 inhibitor and immune modulator | NCT03011814 |
2 | R/R PTCL | Venetoclax and romidepsin | BCL2 inhibitor and HDAC inhibitor | NCT03534180 |
1/2 | R/R PTCL | Pembrolizumab and romidepsin | PD1 inhibitor and HDAC inhibitor | NCT03278782 |
1/2 | R/R PTCL | Pralatrexate and romidepsin | Antifolate and HDAC inhibitor | NCT01947140 |
1 | R/R PTCL | Brentuximab vedotin and lenalidomide | Antibody-drug conjugate targeting CD30 and immune modulator | NCT03302728 |
1 | R/R PTCL | Romidepsin, azacytidine, and lenalidomide | HDAC inhibitor, HMA, immune modulator | NCT04447027 |
2 | R/R EBV-positive lymphomas | Nanatinostat and valganciclovir | HDAC inhibitor and antiviral | NCT05011058 |
1 | R/R CD30-positive lymphomas | AFM13-NK and AFM13 | Modified immune cells and bispecific antiCD30 and CD16A antibody | NCT04074746 |
1 | R/R PTCL | Ruxolitinib and duvelisib | JAK inhibitor and PI3K inhibitor | NCT05010005 |
Azacytidine and Romidepsin
Preclinical data by Marchi et al. show that the combination of HDAC inhibitors and HMAs are synergistic in modulating gene expression leading to the rationale development of this regimen in patients with PTCL[102,103]. Twenty-three patients (10 untreated, 13 R/R) were evaluable in the phase 2 trial with oral azacytidine and romidepsin[103]. Though all subtypes were enrolled, patients with AITL or PTCL TFH
Pralatrexate and Romidepsin
In a murine model of T-cell lymphoma, the administration of pralatrexate in conjunction with romidepsin demonstrated synergy in treatment efficacy than either agent alone[104]. To further explore the safety and efficacy of this combination, patients with R/R lymphomas enrolled in a phase 1 study of pralatrexate and romidepsin[105]. The most common grade 3 and 4 AEs included anemia (29%), thrombocytopenia (28%), febrile neutropenia (14%), and oral mucositis (14%). The ORR for PTCL compared to non-PTCL were 71% (CR 40%) vs. 33% (CR 0%), respectively. Enrollment of patients with R/R PTCL in the phase 2 trial is ongoing (NCT01947140).
Tenalisib and Romidepsin
Tenalisib, a selective PI3K and SIK3 inhibitor, was studied in combination with romidepsin in 33 patients with R/R T-cell lymphomas[106]. In the phase 1/2 trial, there were no dose-limiting toxicities, but the most frequent grade 3 or 4 treatment-emergent adverse events included thrombocytopenia (21%), ALT elevation (18%), and neutropenia (15%). The ORR was 63% in 27 evaluable patients (CR 26%), and 3 out of 6 PTCL patients who achieved a CR were bridged to transplant[106].
Immune Checkpoint Inhibitors Combined with Epigenetic Modifiers
To improve on the results of the doublets: romidepsin plus azacytidine and romidepsin plus pralatrexate, two innovative trials are combining immune checkpoint inhibitors with epigenetic modifiers[107]. In the phase 1 EMBOLDEN trial, pembrolizumab, an anti-PD1 monoclonal antibody, is combined with decitabine and/or pralatrexate and has resulted in an ORR of 2/13 (CR 1/13). Durvalumab in different combinations with pralatrexate, azacytidine, and romidepsin in the phase I/IIa DURABILITY trial has promising results with an ORR of 3/5 (CR 3/5). These trials are ongoing and actively enrolling patients (NCT03240211, NCT03161223).
CHIMERIC ANTIGEN RECEPTOR (CAR) T CELL THERAPY
CAR T cell therapy has been approved for B-cell malignancies and is currently in development for T-cell malignancies. Due to the genetic engineering of T-cells to target neoplastic T-cells, several considerations have arisen, such as T-cell aplasia, fratricide, and the contamination of modified T-cells with neoplastic T-cells that can lead to resistance[108]. Targets have included CD4, CD5, CD7, CD30, and TCR beta chain-1 (TRBC1) have progressed to clinical trials as summarized in Table 4. Results in patients are limited. LB1901 is a CAR T cell therapy targeting CD4, for example, has resulted in low CD4 T-cell counts, leading to a clinical hold[109]. A phase I trial using autologous CD5 CAR T-cells to bridge 9 patients with R/R T-cell malignancies to allogeneic transplant resulted in ORR of 44% without T-cell aplasia[110]. CD30-targeted CAR T cell therapies have been studied in ALCL where of 2 patients treated, 1 had a CR and 1 died of progression[111]. This is a promising modality for treating patients with R/R disease.
CAR T cell therapies in ongoing trials
Phase | Patient population | CAR T | Target antigen | Costimulatory domain | NCT number |
1 | R/R PTCL or CTCL with CD4 expression | LB1901 (Legend Biotech, on clinical hold) | CD4 | 4-1BB | NCT04712864 |
1 | R/R CD5 positive lymphomas | CT125A (Huazhong) | CD5 | Unknown | NCT04767308 |
1/2 | R/R CD5 positive PTCL | MT-101 (Myeloid) | CD5 | Unknown | NCT05138458 |
1 | R/R T-cell leukemia or lymphoma with CD7 expression | CD7.CAR/CD28zeta (Baylor) | CD7 | CD28 | NCT03690011 |
1 | R/R CD7 positive hematologic malignancies | PG-CART-07-001 (PersonGen) | CD7 | Unknown | NCT04480788 |
1 | R/R T-cell hematologic malignancies with CD7 expression | SHYSXY-202105-CD7-CART (Shanghai) | CD7 | Unknown | NCT05290155 |
1/2 | R/R CD7 positive leukemia and lymphoma | anti-CD7 CAR-pNK cells (PersonGen) | CD7 | Unknown | NCT02742727 |
1 | R.R CD30 positive lymphomas | Fully human anti-CD30 CAR (National Cancer Institute) | CD30 | CD28[113] | NCT03049449 |
1/2 | R/R CD30 positive lymphomas | CD30.CAR T cells (Baylor) | CD30 | CD28[114] | NCT02690545, NCT02917083 |
2 | R/R CD30 positive lymphomas | ATLCAR.CD30 T cells (North Carolina) | CD30 | CD28 | NCT04083495 |
1 | R/R PTCL with CD30 expression | CD30.CAR-T (Tessa) | CD30 | Unknown | NCT04526834 |
1 | R/R CD30 positive lymphocyte malignancies | WHUH-CART-CD30-01 (Wuhan) | CD30 | Unknown | NCT04008394 |
1 | R/R CD30 positive | ARM002-CN-2021-01 (Zhejiang) | CD30 | Unknown | NCT05208853 |
1 | CD70 positive malignant hematologic diseases | CD70 CAR T-cells (Zhejiang) | CD70 | Unknown | NCT04662294 |
1/2 | R/R PTCL with TRBC1 expression | AUTO4, RQR8/aTRBC1 (Autolus) | TRBC1 | N/A | NCT03590574 |
1 | R/R TRBC1 positive T-cell hematologic malignancies | SHYSXY-202101-CART (Shanghai) | TRBC1 | N/A | NCT04828174 |
CONCLUSION
The future of PTCL care could look like a precision oncology-based approach where the biology of the disease is targeted for optimal efficacy and limited side effects. Combination chemotherapy has been the default standard of care, but the minority of patients are cured and there are lasting side effects that may impact quality of life. Rather than categorizing patients with PTCL, the future may focus on subtypes to make more appropriate application of clinical trials rather than extrapolating to all patients, even when rarer subtypes are not represented. Patients with ATLL have a range of outcomes based on the subtype of disease, but more treatments need to be developed for the aggressive acute and lymphomatous types. HDAC inhibitors are approved for R/R PTCL but can be associated with hyperprogression in ATLL, highlighting that caution is needed when generalizing clinical trials. Historically, patients with ALCL are associated with better outcomes and CD30-targeted therapy have improved outcome, but more options are needed in those who relapse. Given the enrichment of epigenetic dysregulation as pathogenesis, AITL and TFH are associated with better outcomes when treated with hypomethylating agents and HDAC inhibitors, and there are ongoing trials around the world that are building on the success of these doublets. Patients with ENKTCL have aggressive disease that have found success with immunotherapy in the R/R setting, which can be a different story for patients with nodal PTCL. Nivolumab may be associated with hyperprogression as a single agent, and yet, it has also been found to be efficacious when combined with other drugs, both chemotherapy and targeted agents. CAR T cell therapy offers another modality of treating patients with R/R disease but is still early in development.
The PTCL are rare and heterogeneous, making it difficult to study but many advances, such as technology, diagnostics, and treatments, have been made since 1993, when CHOP was determined to be the standard. Technology permits interconnectedness in a global scientific community where communication across the world can be instant and international, multicenter clinical trials are more feasible than ever before. In addition, the diagnose of lymphomas has become more sophisticated. The Non-Hodgkin’s Lymphoma Pathologic Classification Project from 1982 was used to classify patients with low, intermediate, and high-grade lymphomas[112]. In the trial by Fisher and colleagues, patients with aggressive lymphomas were included and there was no differentiation between B-cell and T-cell[48]. In this trial, subtypes from this working classification, subtypes encompassed both intermediate- and high-grade groups: follicular lymphoma that was predominantly large cell, diffuse small-cleaved cell, diffuse mixed small and large cell, diffuse large cell, immunoblastic, and small noncleaved[89,112]. If these lymphomas were diagnosed today, the revised 2016 WHO classification could categorize these intermediate and aggressive lymphomas in 50 different ways[1]. Finally, the development of novel agents has transformed how to diagnosis and treat lymphomas. In the past, there were only combinations of chemotherapy, and maybe the specific subtype would not change how patients could be treated. Now there are novel agents that specifically target disease pathways and specifying the subtype of lymphoma may change management.
International, multicenter trials are easier to coordinate than ever before, and global consortiums are important in systematically designing trials that answer important questions in the treatment of patients with PTCL. Given the difficulty of enrolling patients in clinical trials, which is also coupled with the fact that at any given moment, there are numerous competing trials, collaborations to enroll patients on trials specific for their disease subtype should be prioritized. Drug development for orphan diseases has drawbacks and challenges as often the number of patients limits impact, but FDA pathways to prioritize will continue to help support these efforts.
DECLARATIONS
AcknowledgmentsThe authors would like to thank the patients and their families. Enrica Marchi would like to thank the U.S. Food and Drug Administration for their research support through the Orphan Products Development (OPD) grant #FD-R-006814-01.
Authors’ contributionsMade substantial contributions to conception and design of the study and performed data analysis and interpretation: Ma H, Marchi E
Availability of data and materialsNot applicable.
Financial support and sponsorshipThe U.S. Food and Drug Administration for their research support through the Orphan Products Development (OPD) grant #FD-R-006814-01.
Conflicts of interestHelen Ma declared no conflicts of interest. Enrica Marchi reports research support from Merck, Celgene, and Astex and scientific advisory roles with Myeloid Therapeutic and Kyowa Kirin.
Ethical approval and consent to participateNot applicable.
Consent for publicationNot applicable.
Copyright© The Author(s) 2022.
REFERENCES
1. Swerdlow SH, Campo E, Pileri SA, et al. The 2016 revision of the World Health Organization classification of lymphoid neoplasms. Blood 2016;127:2375-90.
2. Vose J, Armitage J, Weisenburger D. International peripheral T-cell and natural killer/T-cell lymphoma study: pathology findings and clinical outcomes. J Clin Oncol 2008;26:4124-30.
3. Czuczman MS, Vose JM, Witzig TE, et al. The differential effect of lenalidomide monotherapy in patients with relapsed or refractory transformed non-Hodgkin lymphoma of distinct histological origin. Br J Haematol 2011;154:477-81.
4. Bachy E, Camus V, Thieblemont C, et al. Romidepsin plus CHOP versus CHOP in patients with previously untreated peripheral T-Cell lymphoma: results of the Ro-CHOP phase III study (conducted by LYSA). J Clin Oncol 2022;40:242-51.
5. Shustov AR, Oki Y, Barta SK, et al. CHOP in combination with pralatrexate, a novel folate analogue metabolic inhibitor in patients with previously untreated peripheral t-cell lymphoma (PTCL): interim results of the phase 1 trial. Blood 2016;128:5355-5355.
6. Horwitz S, O’connor OA, Pro B, et al. Brentuximab vedotin with chemotherapy for CD30-positive peripheral T-cell lymphoma (ECHELON-2): a global, double-blind, randomised, phase 3 trial. Lancet 2019;393:229-40.
7. Ma H, Cheng B, Falchi L, et al. Survival benefit in patients with peripheral T-cell lymphomas after treatments with novel therapies and clinical trials. Hematol Oncol 2020;38:51-8.
8. Stuver RN, Khan N, Schwartz M, et al. Single agents vs combination chemotherapy in relapsed and refractory peripheral T-cell lymphoma: Results from the comprehensive oncology measures for peripheral T-cell lymphoma treatment (COMPLETE) registry. Am J Hematol 2019;94:641-9.
9. Choi J, Goh G, Walradt T, et al. Genomic landscape of cutaneous T cell lymphoma. Nat Genet 2015;47:1011-9.
10. van Doorn R, van Kester MS, Dijkman R, et al. Oncogenomic analysis of mycosis fungoides reveals major differences with Sezary syndrome. Blood 2009;113:127-36.
11. Dunn J, McCuaig R, Tu WJ, Hardy K, Rao S. Multi-layered epigenetic mechanisms contribute to transcriptional memory in T lymphocytes. BMC Immunol 2015;16:27.
12. Antignano F, Zaph C. Regulation of CD4 T-cell differentiation and inflammation by repressive histone methylation. Immunol Cell Biol 2015;93:245-52.
13. Iqbal J, Wright G, Wang C, et al. Gene expression signatures delineate biological and prognostic subgroups in peripheral T-cell lymphoma. Blood 2014;123:2915-23.
14. Palomero T, Couronné L, Khiabanian H, et al. Recurrent mutations in epigenetic regulators, RHOA and FYN kinase in peripheral T cell lymphomas. Nat Genet 2014;46:166-70.
15. Ji MM, Huang YH, Huang JY, et al. Histone modifier gene mutations in peripheral T-cell lymphoma not otherwise specified. Haematologica 2018;103:679-87.
16. Sandell RF, Boddicker RL, Feldman AL. Genetic landscape and classification of peripheral T Cell lymphomas. Curr Oncol Rep 2017;19:28.
17. Quivoron C, Couronné L, Della Valle V, et al. TET2 inactivation results in pleiotropic hematopoietic abnormalities in mouse and is a recurrent event during human lymphomagenesis. Cancer Cell 2011;20:25-38.
18. Genovese G, Kähler AK, Handsaker RE, et al. Clonal hematopoiesis and blood-cancer risk inferred from blood DNA sequence. N Engl J Med 2014;371:2477-87.
19. Bowman RL, Levine RL. TET2 in normal and malignant hematopoiesis. Cold Spring Harb Perspect Med 2017;7:a026518.
20. Couronné L, Bastard C, Bernard OA. TET2 and DNMT3A mutations in human T-cell lymphoma. N Engl J Med 2012;366:95-6.
21. Lemonnier F, Couronné L, Parrens M, et al. Recurrent TET2 mutations in peripheral T-cell lymphomas correlate with TFH-like features and adverse clinical parameters. Blood 2012;120:1466-9.
22. Odejide O, Weigert O, Lane AA, et al. A targeted mutational landscape of angioimmunoblastic T-cell lymphoma. Blood 2014;123:1293-6.
23. Muto T, Sashida G, Hasegawa N, et al. Myelodysplastic syndrome with extramedullary erythroid hyperplasia induced by loss of Tet2 in mice. Leuk Lymphoma 2015;56:520-3.
24. Ichiyama K, Chen T, Wang X, et al. The methylcytosine dioxygenase Tet2 promotes DNA demethylation and activation of cytokine gene expression in T cells. Immunity 2015;42:613-26.
25. Cortés JR, Palomero T. Biology and molecular pathogenesis of mature T-Cell lymphomas. Cold Spring Harb Perspect Med 2021;11:a035402.
26. Scourzic L, Couronné L, Pedersen MT, et al. DNMT3A(R882H) mutant and Tet2 inactivation cooperate in the deregulation of DNA methylation control to induce lymphoid malignancies in mice. Leukemia 2016;30:1388-98.
27. Cairns RA, Iqbal J, Lemonnier F, et al. IDH2 mutations are frequent in angioimmunoblastic T-cell lymphoma. Blood 2012;119:1901-3.
28. Ward PS, Patel J, Wise DR, et al. The common feature of leukemia-associated IDH1 and IDH2 mutations is a neomorphic enzyme activity converting alpha-ketoglutarate to 2-hydroxyglutarate. Cancer Cell 2010;17:225-34.
29. Wang C, McKeithan TW, Gong Q, et al. IDH2R172 mutations define a unique subgroup of patients with angioimmunoblastic T-cell lymphoma. Blood 2015;126:1741-52.
30. Ridley AJ. RhoA, RhoB and RhoC have different roles in cancer cell migration. J Microsc 2013;251:242-9.
31. Jaffe AB, Aspenström P, Hall A. Human CNK1 acts as a scaffold protein, linking Rho and Ras signal transduction pathways. Mol Cell Biol 2004;24:1736-46.
32. Morin P, Flors C, Olson MF. Constitutively active RhoA inhibits proliferation by retarding G(1) to S phase cell cycle progression and impairing cytokinesis. Eur J Cell Biol 2009;88:495-507.
33. Sakata-Yanagimoto M, Enami T, Yoshida K, et al. Somatic RHOA mutation in angioimmunoblastic T cell lymphoma. Nat Genet 2014;46:171-5.
34. Yoo HY, Sung MK, Lee SH, et al. A recurrent inactivating mutation in RHOA GTPase in angioimmunoblastic T cell lymphoma. Nat Genet 2014;46:371-5.
35. Ng SY, Brown L, Stevenson K, et al. RhoA G17V is sufficient to induce autoimmunity and promotes T-cell lymphomagenesis in mice. Blood 2018;132:935-47.
36. Cortes JR, Ambesi-Impiombato A, Couronné L, et al. RHOA G17V induces T follicular helper cell specification and promotes lymphomagenesis. Cancer Cell 2018;33:259-273.e7.
37. Zang S, Li J, Yang H, et al. Mutations in 5-methylcytosine oxidase TET2 and RhoA cooperatively disrupt T cell homeostasis. J Clin Invest 2017;127:2998-3012.
38. Boddicker RL, Razidlo GL, Dasari S, et al. Integrated mate-pair and RNA sequencing identifies novel, targetable gene fusions in peripheral T-cell lymphoma. Blood 2016;128:1234-45.
39. Fujisawa M, Sakata-Yanagimoto M, Nishizawa S, et al. Activation of RHOA-VAV1 signaling in angioimmunoblastic T-cell lymphoma. Leukemia 2018;32:694-702.
40. Vallois D, Dobay MP, Morin RD, et al. Activating mutations in genes related to TCR signaling in angioimmunoblastic and other follicular helper T-cell-derived lymphomas. Blood 2016;128:1490-502.
41. Rohr J, Guo S, Huo J, et al. Recurrent activating mutations of CD28 in peripheral T-cell lymphomas. Leukemia 2016;30:1062-70.
42. Manso R, Rodríguez-Pinilla SM, González-Rincón J, et al. Recurrent presence of the PLCG1 S345F mutation in nodal peripheral T-cell lymphomas. Haematologica 2015;100:e25-7.
43. Streubel B, Vinatzer U, Willheim M, Raderer M, Chott A. Novel t(5;9)(q33;q22) fuses ITK to SYK in unspecified peripheral T-cell lymphoma. Leukemia 2006;20:313-8.
44. Huang Y, Moreau A, Dupuis J, et al. Peripheral T-cell lymphomas with a follicular growth pattern are derived from follicular helper T cells (TFH) and may show overlapping features with angioimmunoblastic T-cell lymphomas. Am J Surg Pathol 2009;33:682-90.
45. Feldman AL, Sun DX, Law ME, et al. Overexpression of syk tyrosine kinase in peripheral T-cell lymphomas. Leukemia 2008;22:1139-43.
46. Pechloff K, Holch J, Ferch U, et al. The fusion kinase ITK-SYK mimics a T cell receptor signal and drives oncogenesis in conditional mouse models of peripheral T cell lymphoma. J Exp Med 2010;207:1031-44.
47. Watatani Y, Sato Y, Miyoshi H, et al. Molecular heterogeneity in peripheral T-cell lymphoma, not otherwise specified revealed by comprehensive genetic profiling. Leukemia 2019;33:2867-83.
48. Fisher RI, Gaynor ER, Dahlberg S, et al. Comparison of a standard regimen (CHOP) with three intensive chemotherapy regimens for advanced non-hodgkin’s lymphoma. N Engl J Med 1993;328:1002-6.
49. Abramson JS, Feldman T, Kroll-Desrosiers AR, et al. Peripheral T-cell lymphomas in a large US multicenter cohort: prognostication in the modern era including impact of frontline therapy. Ann Oncol 2014;25:2211-7.
50. Schmitz N, Trümper L, Ziepert M, et al. Treatment and prognosis of mature T-cell and NK-cell lymphoma: an analysis of patients with T-cell lymphoma treated in studies of the German high-grade non-hodgkin lymphoma study group. Blood 2010;116:3418-25.
51. Cederleuf H, Bjerregård Pedersen M, Jerkeman M, Relander T, d’Amore F, Ellin F. The addition of etoposide to CHOP is associated with improved outcome in ALK+ adult anaplastic large cell lymphoma: A Nordic Lymphoma Group study. Br J Haematol 2017;178:739-46.
52. Deng S, Lin S, Shen J, Zeng Y. Comparison of CHOP vs CHOPE for treatment of peripheral T-cell lymphoma: a meta-analysis. Onco Targets Ther 2019;12:2335-42.
53. Lai GM, Chen YN, Mickley LA, Fojo AT, Bates SE. P-glycoprotein expression and schedule dependence of adriamycin cytotoxicity in human colon carcinoma cell lines. Int J Cancer 1991;49:696-703.
54. Horwitz S, O’Connor OA, Pro B, et al. The ECHELON-2 Trial: 5-year results of a randomized, phase III study of brentuximab vedotin with chemotherapy for CD30-positive peripheral T-cell lymphoma. Ann Oncol 2022;33:288-98.
55. Coiffier B, Lepage E, Briere J, et al. CHOP chemotherapy plus rituximab compared with CHOP alone in elderly patients with diffuse large-B-cell lymphoma. N Engl J Med 2002;346:235-42.
56. Wang ES, O’Connor O, She Y, Zelenetz AD, Sirotnak FM, Moore MA. Activity of a novel anti-folate (PDX, 10-propargyl 10-deazaaminopterin) against human lymphoma is superior to methotrexate and correlates with tumor RFC-1 gene expression. Leuk Lymphoma 2003;44:1027-35.
57. O’Connor OA, Pro B, Pinter-Brown L, et al. Pralatrexate in patients with relapsed or refractory peripheral T-cell lymphoma: results from the pivotal PROPEL study. J Clin Oncol 2011;29:1182-9.
58. Advani RH, Ansell SM, Lechowicz MJ, et al. A phase II study of cyclophosphamide, etoposide, vincristine and prednisone (CEOP) Alternating with Pralatrexate (P) as front line therapy for patients with peripheral T-cell lymphoma (PTCL): final results from the T- cell consortium trial. Br J Haematol 2016;172:535-44.
59. Coiffier B, Pro B, Prince HM, et al. Results from a pivotal, open-label, phase II study of romidepsin in relapsed or refractory peripheral T-cell lymphoma after prior systemic therapy. J Clin Oncol 2012;30:631-6.
60. Foss F, Horwitz S, Pro B, et al. Romidepsin for the treatment of relapsed/refractory peripheral T cell lymphoma: prolonged stable disease provides clinical benefits for patients in the pivotal trial. J Hematol Oncol 2016;9:22.
61. Foss F, Horwitz S, Pro B, et al. Erratum to: Romidepsin for the treatment of relapsed/refractory peripheral T cell lymphoma: prolonged stable disease provides clinical benefits for patients in the pivotal trial. J Hematol Oncol 2017;10:154.
62. Chiappella A, Carniti C, Re A, et al. Adding romidepsin to CHOEP in first line treatment of peripheral T-Cell lymphomas does not improve the response rate: final analysis of phase II PTCL13 study. Blood 2021;138:134-134.
63. Ruan J, Moskowitz AJ, Mehta-Shah N, et al. High Rates of remission with the initial treatment of oral azacitidine plus CHOP for peripheral T-Cell lymphoma (PTCL): clinical outcomes and biomarker analysis of a multi-center phase II study. Blood 2021;138:138.
64. Wulf GG, Altmann B, Ziepert M, et al. Alemtuzumab plus CHOP versus CHOP in elderly patients with peripheral T-cell lymphoma: the DSHNHL2006-1B/ACT-2 trial. Leukemia 2021;35:143-55.
65. Lemonnier F, Safar V, Beldi-Ferchiou A, et al. Integrative analysis of a phase 2 trial combining lenalidomide with CHOP in angioimmunoblastic T-cell lymphoma. Blood Adv 2021;5:539-48.
66. Haverkos B, Zain J, Kamdar M, et al. A pilot study using nivolumab in combination with standard of care chemotherapy in newly diagnosed peripheral T-Cell lymphomas. Blood 2021;138:2444.
67. Mak V, Hamm J, Chhanabhai M, et al. Survival of patients with peripheral T-cell lymphoma after first relapse or progression: spectrum of disease and rare long-term survivors. J Clin Oncol 2013;31:1970-6.
68. Ogura M, Ishida T, Hatake K, et al. Multicenter phase II study of mogamulizumab (KW-0761), a defucosylated anti-cc chemokine receptor 4 antibody, in patients with relapsed peripheral T-cell lymphoma and cutaneous T-cell lymphoma. J Clin Oncol 2014;32:1157-63.
69. O’Connor OA, Horwitz S, Masszi T, et al. Belinostat in patients with relapsed or refractory peripheral T-Cell lymphoma: results of the pivotal phase II BELIEF (CLN-19) study. J Clin Oncol 2015;33:2492-9.
70. Horwitz SM, Advani RH, Bartlett NL, et al. Objective responses in relapsed T-cell lymphomas with single-agent brentuximab vedotin. Blood 2014;123:3095-100.
71. Shi Y, Dong M, Hong X, et al. Results from a multicenter, open-label, pivotal phase II study of chidamide in relapsed or refractory peripheral T-cell lymphoma. Ann Oncol 2015;26:1766-71.
72. Maruyama D, Nagai H, Maeda Y, et al. Phase I/II study of pralatrexate in Japanese patients with relapsed or refractory peripheral T-cell lymphoma. Cancer Sci 2017;108:2061-8.
73. Hong X, Song Y, Huang H, et al. Pralatrexate in chinese patients with relapsed or refractory peripheral T-Cell lymphoma: a single-arm, multicenter study. Target Oncol 2019;14:149-58.
74. Piekarz RL, Frye R, Turner M, et al. Phase II multi-institutional trial of the histone deacetylase inhibitor romidepsin as monotherapy for patients with cutaneous T-cell lymphoma. J Clin Oncol 2009;27:5410-7.
75. Whittaker SJ, Demierre MF, Kim EJ, et al. Final results from a multicenter, international, pivotal study of romidepsin in refractory cutaneous T-cell lymphoma. J Clin Oncol 2010;28:4485-91.
76. Maruyama D, Tobinai K, Ogura M, et al. Romidepsin in Japanese patients with relapsed or refractory peripheral T-cell lymphoma: a phase I/II and pharmacokinetics study. Int J Hematol 2017;106:655-65.
77. Kim SJ, Kim JH, Ki CS, Ko YH, Kim JS, Kim WS. Epstein-Barr virus reactivation in extranodal natural killer/T-cell lymphoma patients: a previously unrecognized serious adverse event in a pilot study with romidepsin. Ann Oncol 2016;27:508-13.
78. Kim JH, Kim WS, Park C. Sildenafil prevents HDACi-induced Epstein-Barr virus reactivation through the PKG pathway in NK/T cell lymphoma; potential implications for HDACi-mediated fatal complications. Antiviral Res 2021;189:105063.
79. Sawas A, Ma H, Shustov A, et al. Characterizing the belinostat response in patients with relapsed or refractory angioimmunoblastic T-cell lymphoma. Leuk Lymphoma 2020;61:2003-7.
80. Maruyama D, Tsukasaki K, Uchida T, et al. Multicenter phase 1/2 study of forodesine in patients with relapsed peripheral T cell lymphoma. Ann Hematol 2019;98:131-42.
81. Phillips AA, Fields PA, Hermine O, et al. Mogamulizumab versus investigator’s choice of chemotherapy regimen in relapsed/refractory adult T-cell leukemia/lymphoma. Haematologica 2019;104:993-1003.
82. Fuji S, Inoue Y, Utsunomiya A, et al. Pretransplantation anti-CCR4 antibody mogamulizumab against adult T-Cell leukemia/lymphoma is associated with significantly increased risks of severe and corticosteroid-refractory graft-versus-host disease, nonrelapse mortality, and overall mortality. J Clin Oncol 2016;34:3426-33.
83. Saillard C, Guermouche H, Derrieux C, et al. Response to 5-azacytidine in a patient with TET2-mutated angioimmunoblastic T-cell lymphoma and chronic myelomonocytic leukaemia preceded by an EBV-positive large B-cell lymphoma. Hematol Oncol 2017;35:864-8.
84. Cheminant M, Bruneau J, Kosmider O, et al. Efficacy of 5-azacytidine in a TET2 mutated angioimmunoblastic T cell lymphoma. Br J Haematol 2015;168:913-6.
85. Lemonnier F, Dupuis J, Sujobert P, et al. Treatment with 5-azacytidine induces a sustained response in patients with angioimmunoblastic T-cell lymphoma. Blood 2018;132:2305-9.
86. Horwitz SM, Mehta-shah N, Pro B, et al. Dose optimization of duvelisib in patients with relapsed or refractory peripheral T-Cell lymphoma from the phase 2 primo trial: selection of regimen for the dose-expansion phase. Blood 2019;134:1567.
87. Brammer JE, Zinzani PL, Zain J, et al. Duvelisib in patients with relapsed/refractory peripheral T-Cell lymphoma from the phase 2 Primo trial: results of an interim analysis. Blood 2021;138:2456-2456.
88. Huen A, Haverkos BM, Zain J, et al. Phase I/Ib study of Tenalisib (RP6530), a Dual PI3K δ/γ inhibitor in patients with relapsed/refractory T-Cell lymphoma. Cancers (Basel) 2020;12:2293.
89. Barta SK, Zain J, MacFarlane AW, et al. Phase II study of the PD-1 Inhibitor pembrolizumab for the treatment of relapsed or refractory mature T-cell lymphoma. Clin Lymphoma Myeloma Leuk 2019;19:356-364.e3.
90. Bennani NN, Pederson LD, Atherton P, et al. A phase II study of nivolumab in patients with relapsed or refractory peripheral T-Cell lymphoma. Blood 2019;134:467-467.
91. Ratner L, Waldmann TA, Janakiram M, Brammer JE. Rapid progression of adult T-Cell leukemia-lymphoma after PD-1 inhibitor therapy. N Engl J Med 2018;378:1947-8.
92. Rauch DA, Conlon KC, Janakiram M, et al. Rapid progression of adult T-cell leukemia/lymphoma as tumor-infiltrating Tregs after PD-1 blockade. Blood 2019;134:1406-14.
93. Shi Y, Wu J, Wang Z, et al. Efficacy and safety of geptanolimab (GB226) for relapsed or refractory peripheral T cell lymphoma: an open-label phase 2 study (Gxplore-002). J Hematol Oncol 2021;14:12.
94. Li X, Cheng Y, Zhang M, et al. Activity of pembrolizumab in relapsed/refractory NK/T-cell lymphoma. J Hematol Oncol 2018;11:15.
95. Kwong YL, Chan TSY, Tan D, et al. PD1 blockade with pembrolizumab is highly effective in relapsed or refractory NK/T-cell lymphoma failing l-asparaginase. Blood 2017;129:2437-42.
96. Kim SJ, Hyeon J, Cho I, Ko YH, Kim WS. Comparison of efficacy of pembrolizumab between epstein-barr virus-positive and -negative relapsed or refractory non-hodgkin lymphomas. Cancer Res Treat 2019;51:611-22.
97. Kim SJ, Lim JQ, Laurensia Y, et al. Avelumab for the treatment of relapsed or refractory extranodal NK/T-cell lymphoma: an open-label phase 2 study. Blood 2020;136:2754-63.
98. Horwitz SM, Feldman TA, Ye JC, et al. Phase 2a study of the dual SYK/JAK inhibitor cerdulatinib (ALXN2075) as monotherapy in patients with relapsed/refractory peripheral T-Cell lymphoma. Blood 2021;138:622
99. O’Connor OA, Özcan M, Jacobsen ED, et al. Randomized phase III study of alisertib or investigator’s choice (selected single agent) in patients with relapsed or refractory peripheral T-Cell lymphoma. J Clin Oncol 2019;37:613-23.
100. Foss FM, Porcu P, Horwitz SM, et al. A global phase 2 study of valemetostat tosylate (valemetostat) in patients with relapsed or refractory (R/R) peripheral T-Cell lymphoma (PTCL), including R/R adult T-Cell leukemia/lymphoma (ATL) - valentine-PTCL01. Blood 2021;138:2533
101. Yoshimitsu M, Izutsu K, Makita S, et al. Pivotal phase 2 study of the EZH1 and EZH2 inhibitor valemetostat tosylate (DS-3201b) in patients with relapsed or refractory adult T-Cell leukemia/lymphoma. Blood 2021;138:303-303.
102. Marchi E, Zullo KM, Amengual JE, et al. The combination of hypomethylating agents and histone deacetylase inhibitors produce marked synergy in preclinical models of T-cell lymphoma. Br J Haematol 2015;171:215-26.
103. Falchi L, Ma H, Klein S, et al. Combined oral 5-azacytidine and romidepsin are highly effective in patients with PTCL: a multicenter phase 2 study. Blood 2021;137:2161-70.
104. Jain S, Jirau-Serrano X, Zullo KM, et al. Preclinical pharmacologic evaluation of pralatrexate and romidepsin confirms potent synergy of the combination in a murine model of human T-cell lymphoma. Clin Cancer Res 2015;21:2096-106.
105. Amengual JE, Lichtenstein R, Lue J, et al. A phase 1 study of romidepsin and pralatrexate reveals marked activity in relapsed and refractory T-cell lymphoma. Blood 2018;131:397-407.
106. Iyer SP, Huen A, Ai WZ, et al. Safety and efficacy of tenalisib given in combination with romidepsin in patients with relapsed/refractory T-Cell lymphoma: final results from a phase I/II open label multi-center study. Blood 2021;138:1365
107. Marchi E, Ma H, Montanari F, et al. The integration of PD1 blockade with epigenetic therapy is highly active and safe in heavily treated patients with T-cell lymphoma (PTCL) and cutaneous T-cell lymphoma (CTCL). JCO 2020;38:8049-8049.
108. Scarfò I, Frigault MJ, Maus MV. CAR-based approaches to cutaneous T-Cell lymphoma. Front Oncol 2019;9:259.
109. Biotech L. Legend biotech announces FDA clinical hold of its phase 1 clinical trial for LB1901. Available from: https://www.businesswire.com/news/home/20220215005555/en/Legend-Biotech-Announces-FDA-Clinical-Hold-of-its-Phase-1-Clinical-Trial-for-LB1901 [Last accessed on 28 June 2022].
110. Alcantara M, Tesio M, June CH, Houot R. CAR T-cells for T-cell malignancies: challenges in distinguishing between therapeutic, normal, and neoplastic T-cells. Leukemia 2018;32:2307-15.
111. Hucks GE, Savoldo B, Dotti G, et al. CD30-directed chimeric antigen receptor (CAR)-T cells for treatment of hodgkin lymphoma and non-hodgkin lymphoma in pediatric patients. Blood 2021;138:2829.
112. Non-Hodgkin’s Lymphoma Pathologic Classification Project. National cancer institute sponsored study of classifications of non-hodgkin’s lymphomas. Summary and description of a working formulation for clinical usage. Cancer 1982;49:2112-35.
113. Choi S, Pegues MA, Lam N, Geldres C, Vanasse D, Kochenderfer JN. Design and assessment of novel anti-cd30 chimeric antigen receptors with human antigen-recognition domains. Hum Gene Ther 2021;32:730-43.
Cite This Article
How to Cite
Download Citation
Export Citation File:
Type of Import
Tips on Downloading Citation
Citation Manager File Format
Type of Import
Direct Import: When the Direct Import option is selected (the default state), a dialogue box will give you the option to Save or Open the downloaded citation data. Choosing Open will either launch your citation manager or give you a choice of applications with which to use the metadata. The Save option saves the file locally for later use.
Indirect Import: When the Indirect Import option is selected, the metadata is displayed and may be copied and pasted as needed.
About This Article
Special Issue
Copyright
Data & Comments
Data
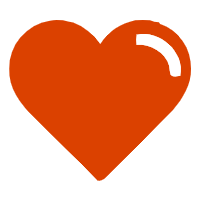
Comments
Comments must be written in English. Spam, offensive content, impersonation, and private information will not be permitted. If any comment is reported and identified as inappropriate content by OAE staff, the comment will be removed without notice. If you have any queries or need any help, please contact us at [email protected].