Novel players in the development of chemoresistance in ovarian cancer: ovarian cancer stem cells, non-coding RNA and nuclear receptors
Abstract
Ovarian cancer (OC) ranks as the fifth leading factor for female mortality globally, with a substantial burden of new cases and mortality recorded annually. Survival rates vary significantly based on the stage of diagnosis, with advanced stages posing significant challenges to treatment. OC is primarily categorized as epithelial, constituting approximately 90% of cases, and correct staging is essential for tailored treatment. The debulking followed by chemotherapy is the prevailing treatment, involving platinum-based drugs in combination with taxanes. However, the efficacy of chemotherapy is hindered by the development of chemoresistance, both acquired during treatment (acquired chemoresistance) and intrinsic to the patient (intrinsic chemoresistance). The emergence of chemoresistance leads to increased mortality rates, with many advanced patients experiencing disease relapse shortly after initial treatment. This review delves into the multifactorial nature of chemoresistance in OC, addressing mechanisms involving transport systems, apoptosis, DNA repair, and ovarian cancer stem cells (OCSCs). While previous research has identified genes associated with these mechanisms, the regulatory roles of non-coding RNA (ncRNA) and nuclear receptors in modulating gene expression to confer chemoresistance have remained poorly understood and underexplored. This comprehensive review aims to shed light on the genes linked to different chemoresistance mechanisms in OC and their intricate regulation by ncRNA and nuclear receptors. Specifically, we examine how these molecular players influence the chemoresistance mechanism. By exploring the interplay between these factors and gene expression regulation, this review seeks to provide a comprehensive mechanism driving chemoresistance in OC.
Keywords
INTRODUCTION
Ovarian cancer (OC), ranking fifth in global women’s mortality, recorded 313,959 incidences and 207,252 deaths[1]. Survival rates at 5 years for stages I-IV are 92.4%, 72.9%, 72.9%, and 31.5%, respectively[2]. Early-stage diagnosis is challenging, with only 20% identified at stage I, while 70% are discovered at higher stages. The International Federation of Gynaecology and Obstetrics (FIGO) classifies OC based on spreading, with 5-year survival rates of 93%, 75%, and 31% for localized, regional, and distant cases, respectively[3]. Epithelial OC (EOC), causing 90% of cases, is a major OC death contributor. The correct staging of OC determines the specific treatment because it provides information about how many cancerous cells are present in body and their location, and cytoreductive surgery followed by chemotherapy is employed in most cases as a treatment strategy. Chemotherapy includes platinum-based drugs such as cisplatin or carboplatin in combination with taxane, generally paclitaxel, and they often develop resistance during chemotherapy or after a few months of the last chemotherapy. The cisplatin reacts N7 of deoxy-guanosine and deoxy-adenine (with low affinity) to form intrastrand, and interstrand crosslink leading to DNA replication blocks, and transcription to induce cell death, while paclitaxel induces cell death via preventing tubulin depolymerization through microtubules stabilization by interacting with β-subunit of tubulin leading to cell cycle arrest during anaphase which required separation of sister chromatids[4,5]. Each drug treatment course is termed a cycle, and six cycles of chemotherapy are provided, with each cycle being 3 weeks in length. The neoadjuvant chemotherapy, in which chemotherapy is given both before and after surgery, provides better responses and high 5-year relative survival rates[6]. The primary challenge in OC is early-stage detection, hindered by the absence of biomarkers and the asymptomatic nature in the initial stages. Another critical issue is chemotherapy-related mortality, wherein patients develop acquired chemoresistance or exhibit intrinsic chemoresistance. Initially, 70% respond to platinum and taxane-based therapies, but resistance develops via several mechanisms such as alteration of drug efflux/influx, increased antioxidant to neutralize reactive oxygen species (ROS) generated due to platinum-based drugs, decreased apoptosis, and hyperactive DNA repair contributing to increased mortality and relapse within 2 years for many patients[7-9]. Understanding chemoresistance involves complex mechanisms with poorly understood regulation of gene expression involved in chemoresistance mechanisms. Factors like ncRNA and nuclear receptors influencing chemoresistance lack comprehensive exploration, making them vital areas for further study. This review delves into chemoresistance related to transport systems, apoptosis, DNA repair, and OCSCs. It scrutinizes the modulation of key cellular processes that foster chemoresistance, encompassing efficient DNA repair, efflux transporter upregulation, OCSCs proliferation, apoptosis inhibition, and influx transporter downregulation. The discussion extends to the role of ncRNA and nuclear receptors for regulating genes associated with various chemoresistance mechanisms in OC.
THE ROLE OF APOPTOSIS IN CHEMORESISTANCE IN OC
This segment of the review explores the intricate interconnection between drug resistance and apoptosis within the context of OC. In our thorough examination, we have delved into the pivotal modulators affected under both sensitive and resistant conditions. Under sensitive conditions, our review highlights key modulators, such as apoptotic protein mechanisms, that play essential roles in maintaining cellular homeostasis and preventing tumorigenesis. Conversely, in resistance conditions, these modulators undergo alterations, compromising their responsiveness to therapeutic interventions. Our comprehensive review has methodically summarized the modifications in these key modulators, elucidating their significance in mediating drug resistance in OC.
For convenience and improved understanding, we have compiled an extensive table [Table 1] detailing the observed alterations in key modulators under both sensitive and resistant conditions. Additionally, we have created a comprehensive figure [Figure 1A] that visually represents the intricate pathways associated with drug resistance in OC. This schematic diagram is designed to enhance your comprehension of the intricate interplay between apoptosis and drug resistance mechanisms.
Figure 1. (A) Apoptosis modulation to enhance chemoresistance in OC. The increased expression of Bcl-2, MCL-1, Bim, Bcl-XL, IAPs (Survivin, XIAP, &cIAP2), Bax phosphorylation and apoptosis regulation by ubiquitination, galectin, glycosylation, and epigenetic regulation inhibit apoptosis to confer chemoresistance in OC; (B) Increased DNA repair activity to enhance chemoresistance in OC. The aberrant expression of DNA repair genes, i.e., BRCA1/2, RAD51, and its paralogs RAD5IC and RAD5ID, RAD52, MRE11, RIFI, hMLH1, hMSIH2, ERCC1, XPF, RPA1, APE1, and XRCC1, promotes drug resistance in OC. Created with BioRender.com. OC: Ovarian cancer; Bcl-2: B cell lymphoma gene 2; MCL-1: myeloid cell leukemia sequence 1; IAPs: inhibitors of apoptosis proteins; X-linked inhibitor of apoptosis protein; BRCA 1/2: breast cancer gene 1/2; MRE11: meiotic recombination 11; hMLH1: human MutL homolog 1; hMSH2: human MutS homolog 2; ERCC1: excision repair cross-complementation group 1; XPF: xeroderma pigmentosum complementation group F; XRCC1: X-ray repair cross-complementing 1.
The role of apoptosis in chemoresistance in OC
Effector molecule | Differential expression profile | Model system | Chemotherapy agents | Associated-mechanism | Ref. | ||
Intrinsic pathway apoptosis | Anti-apoptotic | Bcl-2 | Up | SKOV3 spheroid | Cisplatin | - | [10] |
SKOV3 | Paclitaxel | PKR-Bcl2 | [11] | ||||
Bcl-XL | Up | SKOV3 | Cisplatin | - | [12] | ||
MCL-1 | Up | OVCAR3, A2780 | Carboplatin | MGMT-DUB3-MCL1 | [13] | ||
Pro-apoptotic | Bax | - | MDA‐MB‐468 | ABT‐737 | Akt-Bax | [14] | |
Bim | Down | ES2, TOV21G, SKOV3, OVTOKO | ABT-263 | ZEB1-BIM | [15] | ||
Extrinsic pathway apoptosis | Membrane TRAIL-R2 | Down | OAW42, SKOV3, A2780 | TRAIL | CHKA-TRAIL-R2 | [16] | |
FLIP | Up | OV2008, C13 | Cisplatin | - | [17] | ||
IAPs mediated apoptosis | XIAP | Up | SKOV3 | Docetaxel | - | [18] | |
A2780, BALB/c nude mice | Cisplatin | - | [19] | ||||
Survivin | Up | IGROV-1, OAW42 | Taxol | - | [20] | ||
cIAP2 | Up | SKOV3, OVCAR3 | Cisplatin | Il-6-cIAP2 | [21] | ||
Ubiquitination mediated apoptosis | EDD/UBR5 | Up | A2780ip2, OVCAR5, ES-2 | Cisplatin | EDD-Mcl-1 | [22] | |
A2780 and Tyknu | Cisplatin | EDD/Dyrk2-MOAP-1 | [23] | ||||
ITCH | - | OV2008, A2780s | Cisplatin | FLIP-p53-Itch | [24] | ||
HOIP | Up | A2780 | Cisplatin | JNK pathway | [25] | ||
CRL4 | Up | A2780 | Cisplatin | CRL4-STAT3-BIRC3 | [26] | ||
Glycosylation mediated apoptosis | ST6Gal1 | Up | A2780 | Cisplatin | - | [27] | |
HSPG(Syndecan-2) | Up | SKOV-3, OVCAR-3 | Cisplatin | DcR3-HSPG | [28] | ||
N-Glycosylation | - | OVCAR-3 | Tunicamycin | ER-stress | [29] | ||
Galectin mediated apoptosis | Galectin-1 | Up | A2780/CP | Cisplatin | H-Ras/Raf-1/ERK pathway, p21, Bcl-2 | [30] | |
Galectin-3 | Up | OVCAR-3 | Cisplatin | Mitochondrial dysfunction | [31] | ||
Epigenetic mediated apoptosis | hMOF | Up | OVCAR3/DDP | Cisplatin | hMOF-MDM2 | [32] | |
DNA methyltransferase inhibitors decitabine | - | platinum-resistant OC patients | Carboplatin | - | [33] | ||
HDAC inhibitors entinostat, avelumab | - | Recurrent OC patient | Platinum | - | Clinical trail (NCT02915523) |
THE ROLE OF DNA REPAIR IN CHEMORESISTANCE IN OC
The role of DNA repair mechanisms in drug resistance within OC is a critical aspect of understanding the challenges and complexities associated with treatment. DNA repair processes have a significant role in the response of cancerous cells to various therapeutic agents[34]. Understanding the intricate relationship between DNA repair mechanisms and drug resistance in OC is crucial for formulating targeted therapies that can overcome or exploit these mechanisms, ultimately improving treatment outcomes for patients with this challenging disease. To address this complexity, we have chosen a strategic approach to streamline and simplify the information for our audience. We condensed the details concerning the role of drug resistance on DNA repair pathways into an extensive table [Table 2]. This tabular format serves as a comprehensive reference, presenting the main insights and discoveries in an organized manner. It offers a concise yet informative overview of the subtle interactions within the DNA repair pathways influenced by drug resistance in OC. Additionally, alongside the table, we have created an illustrative figure [Figure 1B], that visually illustrates the impact of drug resistance on DNA repair pathways in OC. This visual representation aims to enhance comprehension of the intricate relationships between drug resistance mechanisms and the complex processes of DNA repair.
Overview of the DNA repair mechanisms contributing to chemoresistance in OC
DNA repair pathway | Gene | Differential expression profile | Model system | Chemotherapy agent | Associated-mechanism | Ref. |
HR | BRCA1 | - | Recurrent OC | Platinum | Reversion mutation, increased loss of methylation | [35] |
BRCA1/2 | Recurrent OC | Platinum, PARPi | Somatic mutation | [36] | ||
RAD51 | Up | CP70 and SKOV3 | PARPi | LCK-RAD51/BRCA1/2 | [37] | |
RAD51C, RAD51D | - | OC patient | PARPi | Secondary mutation | [38] | |
RAD52 | Up | A2780 cisR | Cisplatin | PAF1/PD2-RAD52 | [39] | |
MRE11 | - | COV362 | PARPi | DYNLL1-MRE11 | [40] | |
NHEJ | RIF1 | Up | EOC patients | Cisplatin | - | [41] |
NER | ERCC1-XPF | Up | A2780 | Cisplatin | - | [42] |
ERCC1 | Up | OC patient | Platinum | - | [43] | |
RPA1 | OVCAR8 | Camptothecin | DOCK7-RPA1 | [44] | ||
BER | XRCC1 | Up | OVCAR-3, OVCAR-4 | Cisplatin | - | [45] |
- | SKOV3/DDP | Cisplatin | HSP90-XRCC1 | [46] | ||
- | OC patient | Cisplatin | XRCC1 194 Trp/Trp, XRCC1 399Arg/Arg polymorphism | [47] | ||
APE1 | Up | OC patient | Platinum | - | [48] | |
MMR | hMLH1 | - | A2780/cp70 | Cisplatin | Hypermethylation | [49] |
hMSH2 | - | A2780 | Cisplatin | Hypermethylation | [50] |
THE ROLE OF TRANSPORT SYSTEM IN CHEMORESISTANCE IN OC
The optimal effectiveness of a drug within cells depends on its optimal cytoplasmic concentration. Membrane transporters, particularly ABC transporter, P-type ATPase transporter, and solute carrier (SLC) transporter, are key factors influencing the bioavailability of drugs in ovarian cancer
Figure 2. (A) Schematic diagram of drug transport in chemoresistance in OC. ABC transporters such as ABCB1, ABCC1, ABCC2, ABCC4, ABCC10, and ABCG2, P-type ATPase ATP7A, and SLC transporter CTR2 responsible for drug efflux are upregulated, while SLC transporter CTR1 that functions for drug influx is downregulated, and P-type ATPase transporter ATP7B does not directly facilitate drug efflux, but its genetic polymorphism can influence drug efflux; (B) OCSCs confer chemoresistance in OC. OSCs are transformed into OCSCs due to genetic alteration, which then proliferate into progenitor cells and subsequently differentiate into cells that contribute to the relapse of ovarian tumors after some time instead of undergoing cell death upon chemotherapy. Created with BioRender.com. OC: Ovarian cancer; ABCB1: ATP-binding cassette subfamily B member 1; ABCC: ATP-binding cassette subfamily C; ABCG2: ATP-binding cassette subfamily G member 2; SLC: solute carrier; CTR1/2: copper transporter 1/2; OCSCs: ovarian cancer stem cells.
The role of transport system in chemoresistance in OC
Differential expression profile | Model system | Chemotherapy agent | Associated-mechanism | Ref. | ||
ABC superfamily transporters | ABCB1 | Up | A2780, SKOV3 | Cisplatin | SORL1-EEA1-ABCB1 | [51] |
Gli2-ABCB1 | [52] | |||||
ABCC1 | Up | OC patient | Cisplatin, paclitaxel | - | [53] | |
ABCC2 | Up | A2780, SKOV3 | Cisplatin | HnRNPA2B1-ABCC2 | [54] | |
ABCC4 | Up | 27/87 | Topotecan | MYCN-ABCC4 | [55] | |
ABCC10 | Up | SKOV3 | Paclitaxel, docetaxel, vincristine, vinorelbine | - | [56] | |
ABCG2 | Up | OVCAR-3 S, CAOV-3 S | Adriamycin | HIF-2α-ABCG2 | [57] | |
P-type ATPase superfamily transporter | ATP7A | Up | A2780-CP20 | Cisplatin | - | [58] |
OC patient | Cisplatin | Polymorphism | [59] | |||
ATP7B | Up | A2780-CP20 | Cisplatin | - | [60] | |
IGROV-CP20 | Cisplatin | TFEB-ATP7B | [61] | |||
SLC superfamily transporters | CTR1 | Down | A2780-CP | Cisplatin | - | [62] |
A2780 | Cisplatin | Core fucosylation | [63] | |||
CTR2 | Up | OV-2008 | Cisplatin | - | [64] |
ABC superfamily transporters
ABC transporters, with seven families, use ATP to facilitate substrate efflux/influx. Comprising 49 members and 21 pseudo-members, they mainly efflux substrates. Structurally, ABC transporters have single polypeptides with two nucleotide binding domains (NBDs) and two transmembrane domains (TMDs)[65]. The SNAIL, ZEBs, and SLUG promote MDR, while VDR, ER, PXR, KLF, Gli, and Sp are also known to modulate ABC transporters[66]. The altered ABCB1 structure or drug binding, inhibition of its expression or knockout of the ABCB1 gene are the most potential strategies to overcome ABCB1-mediated drug resistance[67]. Similarly, Basic helix-loop-helix family member e40 (BHLHE40) is inversely related to ABCB1, suggesting that the upstream target of ABCB1 can be used to overcome ABCB1-mediated chemoresistance[68]. Recent research has explored the broad substrate specifity, and conversion of efflux to influx pump via engineering of ABC transporter, and the importance of membrane transporters is also highlighted in the development of precision medicine[69,70].
ABCB1 elevation resists cisplatin/paclitaxel and knockdown restore sensitivity[71-74]. Paclitaxel, a direct substrate of ABCB1, regains sensitivity upon ABCB1 mutation, while 14 residues replacement in helices 6 and 12 reverses ABCB1’s efflux to influx of taxol derivative Flutax-1[75,76]. Sortilin-related receptor 1 (SORL1) silencing inhibits the endosomal antigen 1 pathway, delaying ABCB1 stabilization, sensitizing cis-diamminedichloroplatinum(II) (CDDP)-resistant ovarian cells[77-79].
ABCB1 is regulated by the Hedgehog pathway, with Gli2 directly targeting and positively modulating its expression[80]. ABCB1 Single Nucleotide Polymorphism (SNP), 3435C>T, enhances docetaxel efflux in OC[81]. ABCC1, ABCC2, and ABCC4 are associated with chemoresistance. ABCC1 has significantly elevated levels in primary drug (cisplatin and paclitaxel) resistant EOC tissues[82,83]. Heterogeneous nuclear ribonucleoprotein A2/B1 (HnRNPA2B1) affects ABCC2 translation, blocked by interferon-stimulated gene 15 (ISG15), downregulated in cisplatin-resistant ovarian cancer[84]. ABCC4 confers resistance to topotecan and irinotecan in high myc expression OC[85].
ABCC10 overexpressed in established SKOV3 cell line resistance to paclitaxel, docetaxel, vincristine, and vinorelbine enhanced epithelial to mesenchymal transition (EMT) in OC, restored by cepharanthine, ABCC10 inhibitor[86]. ABCG2, elevated in topotecan-resistant A2780 cells, regains sensitivity with ABCG2 antagonists. Hypoxia inducible factor 2 alpha (HIF-2α) upregulation promotes OCSC stemness and ABCG2-mediated Adriamycin resistance[87].
P-type ATPase superfamily transporter
P-type ATPase superfamily transporter, with five subfamilies (P1-P5), transports ions across membranes using ATP hydrolysis energy. P-type ATPases have cytosolic domains (A, P, N) and transmembrane domains (M1-M6, with P1 having M7-M10)[88]. ATP7B knockdown enhances cisplatin sensitivity. ATP7A silencing lacks impact on resistance, but ATP7A polymorphism is linked to cisplatin resistance in ovarian cancer[89]. The transcription factor EB (TREB) binds the promoter's first intron region at coordinated lysosomal expression and regulation (CLEAR) sites of ATP7B, modulating its expression when exposed to platinum drugs in ovarian cancer[90].
SLC superfamily transporters
SLC transporters, classified into 65 families based on sequence similarity, generally uptake substrates but can be bidirectional or efflux. They exist as homodimers or heterodimers[91]. Cisplatin-sensitive A2780 shows higher copper transporter 1 (CTR1), while cisplatin-resistant has reduced uptake due to CTR1 downregulation upon exposure[92,93]. The CTR1 core fucosylation is higher in A2780 resistant, suppressing CTR1-CDDP interaction and affecting cisplatin uptake[94]. Copper transporter 2 (CTR2) downregulation increases cisplatin sensitivity, linked to platinum efflux[95].
THE ROLE OF OCSC IN CHEMORESISTANCE IN OC
New research indicates that contrary to previous beliefs about a constant follicle in the ovary at birth, there are ovarian stem cells (OSCs) present. These include a dormant group of very small embryonic-like stem cells (VSELs) and a larger subset of dividing OSCs. OSCs are undifferentiated cells inherently capable of self-renewal, proliferation, multipotency, and differentiation. VSELs, which express embryonic markers such as octamer-binding transcription factor 4 (OCT-4), are located in ovary surface epithelium, and can divide asymmetrically to self-renew to form OSC[96]. OSCs are maintained by a niche microenvironment composed of ECM, immune cells, stromal cells, mesenchymal cells, and vascular network[97]. A recent study identified the tubal-peritoneal junction & hilum region as stem cell niche within the ovary[98]. Ovarian tumors exhibit heterogeneity with distinct cell types expressing stem cell markers cluster of differentiation 133 (CD133), leucine-rich repeat-containing G-protein coupled receptor 5 (LGR5), aldehyde dehydrogenase 1 (ALDH1), and cytokeratin 6B (CK6B). These cells, known as OCSCs, arise from genetic instability in OSCs, contributing to chemoresistance, cancer initiation, and treatment failure[98]. OCSC, characterized by various markers, may display diverse phenotypes, offering selective advantages[99]. OCSCs inherently resist chemotherapy. Targeting OCSC and pathways offers strategies against OC chemoresistance[99] [Figure 2B and Table 4].
The role of OCSC in chemoresistance in OC
OCSC marker | Differential expression profile | Chemotherapy agent | Associated-mechanism | Ref. |
ALDHA1A1 | Up | Carboplatin | RAD6-H2B-SOX2 | [100] |
SOX2 | Up | Carboplatin | RAD6-H2B-SOX2 | [100] |
Cisplatin, paclitaxel | - | [101] | ||
- | Hypoxia-notch-SOX2 | [102] | ||
- | SOX2- to β-catenin | [103] | ||
CD133 | Up | - | NF-κB, MAPK pathway | [104] |
NANOG | Up | Cisplatin, paclitaxel | - | [105] |
OCT4 | Up | Cisplatin, paclitaxel | - | [105] |
- | AKT pathway | [106] | ||
CD44 | Up | Cisplatin, paclitaxel | AKT pathway | [106] |
EpCAM | Up | Doxorubicin | - | [107] |
ALDH1 | Up | Platinum | - | [108] |
CD24 | Up | Cisplatin | EMT | [109] |
CD133 | Up | Cisplatin, paclitaxel | - | [110] |
CD177 | Up | Cisplatin, paclitaxel | CD177-ABCG2 | [111] |
HLF | Up | Carboplatin | HLF-YAP1 | [112] |
OCSC markers
SRY-box transcription factor 2 (SOX2) overexpression induces cisplatin, carboplatin, and paclitaxel resistance in OC, and higher Nanog Homeobox (NANOG) and OCT-4 also found to confer cisplatin and paclitaxel resistance in OCSCs[113]. Overexpression of c-kit, NANOG, ABCG2, ABCG5, and MDR1 is implicated in OCSC maintenance[114]. CD133 promotes OCSC stemness, increasing cisplatin and paclitaxel resistance in OC, and Epithelial cell adhesion molecule (EpCAM) overexpression is stimulated with doxorubicin promoting stemness in SKOV3 and OVCAR5[115,116]. The enrichment of OCSC markers ALDH1 is found in OC patients after platinum-based chemotherapy treatment[117]. RAD6 upregulation in chemoresistant OC mediates histone 2B ubiquitination, regulating stem cell genes (ALDH1A1 and SOX2). Silencing RAD6 reduces DNA repair signaling and OCSC markers, sensitizing OC to carboplatin[117]. CD117 expression correlates with chemotherapy response, with CD44+CD117+ cells exhibiting cisplatin and paclitaxel resistance compared to CD44-CD117- cells. CD177+ cells overexpress ABCG2, conferring cisplatin and paclitaxel resistance, while CD24 expression induces EMT and cisplatin resistance in OC OC[117,118]. CD44 and ALDH1A1+ OCSCs confer platinum and taxane resistance, with ALDH1 silencing sensitizing cells to chemotherapy[119-122]. The platinum treatment induces OCSC proliferation, blocked by a combination of platinum and oxidative phosphorylation (OXPHOS) inhibitors[123].
OCSCs signaling pathways
The activated Wnt/β-catenin pathway activation promotes the OCSC and STAT3 also promotes the stemness through the Wnt/β-catenin pathway[124]. The SOX2 elevated in OCSC binds to β-catenin to sustain stem cell-like features of OCSCs[124]. Calcitriol decreases OCSCs through downregulation of Wnt/β-catenin[124]. NF-κB and MAPK signaling pathways promote CD133+ OCSCs[124]. DAPT inhibits Notch signaling, reducing OCSC self-renewal and Notch3 silencing enhances platinum sensitivity[124]. Galectin-3 activates Notch1, maintaining OCSCs[124]. The H/ACA box 72 (SNORA72) promotes OCSC activation via Notch1-Cellular-Myc (c-Myc) axes[125]. Notch3 upregulation in recurrent tumors suggests a role in tumor relapse[126]. The PI3K/AKT pathway enhances OCSC markers, conferring cisplatin and paclitaxel resistance in OC[127]. Akt inhibition (NV-128) induces apoptosis in CD44+/Myeloid Differentiation Primary Response 88 (MyD88)+ OCSCs via Reactive ROS-dependent ERK activation. 2-(4-morpholinyl)-8-phenyl-chromone (LY294002) suppresses Oct 4, ABCG2, and P-gp in SKOV3 OCSCs, potentially reducing chemoresistance[127]. Hepatic leukemia factor (HLF) upregulation in OCSCs maintains OCSCs and confers carboplatin resistance. Mechanistically, HLF activates YAP1 expression, modulating the Hippo signaling pathway. Silencing HLF or using the YAP1 inhibitor verteporfin attenuates carboplatin resistance in OC[128].
The interplay between non-coding RNA and OCSCs
The non-coding RNAs are RNA molecules that are transcribed but not translated, and categorized based on length and shape, such as circular RNA (circRNA) with a circular structure, long non-coding RNA (lncRNA) exceeding 200 nucleotides, and microRNA (miRNA) with an average length of 22 nucleotides. The lncRNA, miRNA, and circRNA are found to regulate the OCSCs, leading to tumor relapse and chemotherapy resistance[129]. The lncRNA SNORD89 is highly upregulated in OCSCs and promotes its stemness by upregulating the Notch1-c-Myc pathway[130]. Similarly, the silencing of another lncRNA MALAT1 decreases the OC cell stemness and increases the cisplatin sensitivity through interaction with yes-associated protein (YAP), blocking its movement from the nucleus to the cytoplasm and bolstering the stability of the YAP[131]. lncRNA HOTAIR increased in OCSCs to confer cisplatin resistance and its depletion resulted in reduced resistance to cisplatin in OCSCs. Mechanistic study shows HOTAIR promotes the T-box transcription factor 3 (TBX3), and maintains stemness of OC expression by sponging miR-206[131]. Using PNA3 to target HOTAIR, thus interrupting its binding with EZH2, along with a DNA methyltransferase (DNMT) inhibitor, leads to a decrease in ALDH+ (OCSCs)[132]. The downregulation of lncRNA TUG1 and overexpressed miR-186-5p suppressed OCSCs. A mechanistic study shows that TUG1 sponges miR-186-5p to release ZEB1 to promote the stemness of OC cells. The downregulation of miR-429 and miR-591 targets ZEB1 to confer cisplatin and paclitaxel resistance, respectively, in OC[132,133]. lncRNA LINC01234 adsorb miRNA-27b-5p to promote the silent information regulator 5 (SIRT5) expression to induce OCSCs progression[134]. The lncRNA XIST is found to be downregulated in OC to confer paclitaxel resistance, and mechanistic study shows that it increases Lysine N-methyltransferase 2C (KMT2C) via targeting miR-93-5p to regulate CD44+/CD24- OSCs[135]. lncRNA-H19 sponges miR-29b-3p to promote STAT3 to promote carboplatin-resistant EOC[136]. Several miRNAs are also known to regulate the OCSCs. The downregulation of miR-200c promotes Gab2-enhanced expansion of ALDH+(stem cell maker) cells[137]. The overexpression of Yin Yang 1 (YY1) promotes the OCSCs via recruiting HDAC5 to the miR-99a, and enhancing the miR-99a deacetylation and decreased miR-99a[138]. The miR-26b is downregulated in CD117+CD44+ OCSCs to promote its stemness and mechanistic study shows its functional target is PTEN[139]. miR-181a is found to promote stemness and cisplatin resistance in HGSOC via the Wnt/β-catenin pathway[140]. miR-600 binds to the 3’-untranslated region of Krueppel-like factor 9 (KLF9) to supress its expression to promotes OCSCs[140].Similarly, miR-181a-2-3p suppresses the stemness of CD44-positive OCSCs through its interaction with EGR1[141]. Downregulating miR-21 significantly decreased CD133+ population and cancer stem/progenitor cells (CSPC) sphere formation, while miR-21 overexpression increased CD133+ cells and CSPC spheres[142]. circRNA, like circ_0000745, modulated by insulin-like growth factor 2 mRNA-binding protein 2 (IGF2BP2) boosts SKOV3 stemness by sponging miR-3187-3p to enhance ERBB4, thus phosphorylating the PI3K/AKT signalling[143]. The circRNA microarray analysis revealed 159 upregulated and 55 downregulated circRNAs in OCSCs, suggesting circular RNA is a crucial player in driving OCSC stemness[144].
THE ROLE OF NUCLEAR RECEPTORS IN CHEMORESISTANCE IN OC
Nuclear receptors, activated by lipid-soluble signals like steroid hormones, regulate gene expression via hormone response elements (HRE), impacting proliferation, apoptosis, and metabolism[145]. There are 48 nuclear receptors in humans, and when their normal functioning is disrupted, it is frequently associated with various diseases. Nuclear receptors are classified into seven families, i.e., NR0-6, based on sequence homology[146]. Nuclear receptors are structured into four segments: the unstructured N-terminal domain (NTD) housing activation function 1 (AF-1), DNA binding domain (DBD), Hinge region, and ligand binding domain (LBD). The LBD binds to ligands and engages with co-regulator proteins via activation function 2 (AF-2) [Figure 3A][146]. The nuclear receptor co-regulators are divided into two categories, i.e., coactivators and corepressors, which directly interact with AF-1 and AF-2 regions of nuclear receptors. Coactivators bind via LXXLL motifs, and corepressors via CoRNR box motifs[146]. Nuclear receptors are also classified into classes I, II, III, and IV based on ligand binding and DNA binding [Figure 3B]. Class I nuclear receptors are sequestered in cytoplasm with chaperon proteins, but upon ligand (cholesterol-derived steroidal hormones) activation, they enter inside the nucleus to bind DNA response elements (RE) composed of two inverted repeats as homodimers[146]. Class II nuclear receptors are present in nucleus with corepressor, but upon ligand activation, corepressor is swapped with coactivators and binds to DNA RE consisting of direct repeat sequence as heterodimers[146]. Class III nuclear receptors are similar in working mechanism to class II, Table 5 except they bind to DNA RE comprising direct repeat sequence as homodimers[146]. Class IV are also similar to the working mechanism of class II, except they bind to extended half-sites within DNA RE as monomers[146]. Chemotherapy is among the top three commonly used treatments for OC. Unfortunately, its effectiveness is restricted by OC cells that have become resistant to the drugs[147]. The importance of various nuclear receptor families in controlling drug metabolism and distribution is gaining recognition, and therapies aimed at these receptors offer new possibilities to mitigate or potentially prevent drug resistance[148]. In this context, we will explore the latest findings concerning the roles and control of different nuclear receptors in the emergence of drug resistance in OC. We will also shed light on how nuclear receptors are linked to drug resistance during chemotherapy and nuclear receptors associated with chemoresistance in OC [Table 5 and Figure 3C].
Figure 3. (A) Nuclear receptors domain architecture; (B) Diagram illustrating the categorization of nuclear receptors associated with chemoresistance in OC, with a focus on their interaction with ligands; (C) Demonstrating various mechanisms associated with the involvement of nuclear receptors in OC drug# resistance, primarily encompassing the inhibition of apoptosis, drug efflux, and the presence of OCSCs. Created with BioRender.com. OC: Ovarian cancer; OCSCs: ovarian cancer stem cells.
Overview of different nuclear receptors implicated in drug resistance in OC, covering specific drug, differential expression patterns, and their associated mechanisms
Family of NRs | Class of NRs | Gene name | Common name | Differential expression profile | Chemotherapy agent | Target-gene | Associated-mechanism | Ref. |
1C | II | PPARG | PPAR γ | Down | Cisplatin; paclitaxel | - | Apoptosis inhibition | [149] |
1H | II | NR1H3/NR1H2 | LXR α/LXR β | Up | Cisplatin; paclitaxel | MDR1 | Drug efflux | [150] |
1I | II | NR1I2 | PXR | Up | Cisplatin; paclitaxel | MDR1 | Drug efflux | [151] |
1I | II | NR1I3 | CAR | Up | Cisplatin; paclitaxel | MDR1 | Drug efflux | [152] |
2F | III | NR2F6 | COUP-TF γ | Up | Cisplatin | Notch3 | OCSCs | [153] |
3A | I | ESR1 | ER α | Up | Cisplatin | Bcl-2 | Apoptosis inhibition | [154] |
3A | I | ESR2 | ER β | Up | - | SOX2, OCT4 | OCSCs | [155] |
3B | IV | ESRRA | ERR α | Up | - | - | OCSCs | [156] |
3C | I | AR | AR | Up | Paclitaxel | ABCB1, ABCB6, ABCG2; AR/Ahr-ABCG2; TLR/IL-6/IRF1; NANOG | Drug efflux; OCSCs | [157-163] |
4A | IV | NR4A1 | NGF1-B | Down | Cisplatin | Bcl-2 | Apoptosis inhibition | [164] |
4A | IV | NR4A3 | NOR1 | Up | Cisplatin | - | - | [165] |
PPARγ inhibits apoptosis, while LXRα/β upregulates cholesterol and MDR1 for drug efflux to confer cisplatin and paclitaxel resistance in OC[166,167]. PXR and CAR activation boosts cisplatin and paclitaxel resistance and downregulation suppress MDR1/ABCB1, enhancing apoptosis[168-170]. ERα and androgen receptor (AR) from family 3 contribute to chemoresistance, with ERα inducing cisplatin resistance in OC[171]. Tamoxifen resistance is associated with IL-6, impacting ERα, ERβ, and steroid receptor coactivator-1 (SRC-1) expression and inhibition of estrogen-induced ER nuclear translocation[172,173]. FK506 binding protein 5 (FKBP5) silencing sensitizes OC to taxol, forming a complex with AR and modulating taxol resistance genes ABCB1, ABCB6, and ABCG2[174]. Taxol activates the Akt pathway, triggering p300-mediated increases in AR expression and chromatin remodeling of the ABCB1 gene. This involves AR/H3K9ac and AR/H3K14ac interactions, followed by AR and p300 binding at the androgen-response elements (ARE4) of ABCB1, leading to heightened expression and chemoresistance in OC[175]. Upregulated AR promotes paclitaxel resistance by complexing with aryl hydrocarbon receptor (Ahr), binding to an alternative ARE on ABCG2 promoter, increasing expression in taxol-resistant serous EOC[176]. Histone H1-0 knockdown decreases AR, sensitizing OC. PI3K/Akt overexpression elevates H1.0, which activates the GCN5, a histone acetyltransferase that acetylates the H3 histone, leading to AR-mediated ABCB1, and ABCG2 expression, ultimately conferring paclitaxel resistance[177]. Toll-like receptor 4 (TLR4) overexpression in taxol resistance OC activates AR, forming a TLR4/AR axis linked to taxol resistance[178]. Further study shows that AR is regulated by the TLR4/IL-6/interferon regulatory factor 1 (IRF1) signaling axis in OC[179]. NGF1-B and NOR1 from family 4, with class IV features, are associated with cisplatin chemoresistance. NR4A1 downregulation is observed in cisplatin-resistant OC[180]. Cisplatin-induced cytoplasmic translocation of NR4A1 is lower in resistant cell lines, where it regulates apoptosis through un N-terminal kinase (JNK) activation, Akt inhibition, and interaction with Bcl-2[181]. NR4A2 has also been reported to confer 5-fluorouracil and oxaliplatin resistance in gastric and colorectal cancer[182,183]. The NR4A3 has high expression in cisplatin resistance cell lines SKOV3 and A2780[184].
The interplay between nuclear receptors and OCSCs
The interaction between nuclear receptors and OCSCs promotes OC relapse and numerous research studies have uncovered their correlation. PPARγ, family 1, and class II member, linked with OCSCs, drives M2 polarization of Raw264.7 cells, inhibiting NF-κB, and GW9662, a PPARγ antagonist, counters these effects on macrophages[185]. COUP-TFγ from family 2, with class I features, is linked to cisplatin resistance by promoting EOC stem cells and sustaining Notch3 signaling by enrichment of p300 and increasing p300 acetylated histone acetylation H3(H9, K27) at Notch3 promoter[186]. ERβ, especially isoform 1, from family 3, exhibiting class I features, shows increased presence in OCSCs, while LY500307, an ERβ agonist, reduces OCSC viability and downregulates SOX2, OCT4, and NANOG[187]. The ERRα from family 3, with class IV features, is linked to OCSCs. The miR-200 family regulates Snail through ERRα, and reducing miR-200a/b expression reverses EMT and stem cell characteristics in OC[188]. AR from family 3 with class I features facilitates growth in CSPC-rich OVTC PA1 cells, governing the self-renewal of stem cells. AR is more abundant in CD133+ cells, and its enrichment downregulates p53 and p16[189]. AR is upregulated in OCSC and androgen 5α-dihydrotestosterone (DHT) promotes OC stemness by enhancing NANOG expression[190].
THE ROLE OF NCRNAS IN CHEMORESISTANCE IN OC
ncRNAs serve as gene expression regulators across multiple biological processes, such as cell division, programmed cell death, cellular transport, EMT, OCSCs, and DNA mending[191]. Recent studies highlight ncRNAs as crucial regulators of chemoresistance in ovarian, breast, and lung cancers[192-194]. Understanding the identification and mechanisms of ncRNAs in gene expression regulation can aid in biomarker development for early detection. Additionally, targeting ncRNAs in chemotherapy can enhance cell death, ultimately leading to a higher 5-year survival rate. This review summarizes the roles of ncRNAs, particularly lncRNA, circRNA, and miRNA, in chemoresistance in OC [Table 6 and Figure 4].
Figure 4. The well-defined mechanisms associated with various ncRNAs in OC drug# resistance primarily involve autophagy, drug efflux, inhibiting cell apoptosis, DNA repair, EMT, and OCSCs. Created with BioRender.com and CorelDRAW. ncRNAs: Non-coding RNAs; OC: ovarian cancer; EMT: epithelial to mesenchymal transition; OCSCs: ovarian cancer stem cells.
Summary of various non-coding RNAs (lnc-RNA, circular RNA and miRNA) involved in OC drug resistance, encompassing the details about specific drugs and their associated mechanism
Name of non-coding RNAs | Differential expression patterns | Model system | Chemotherapy agent | Mode of mechanism | Target/ pathway axis | Ref. |
Long non-coding RNA | ||||||
MALAT1 | Up | A2780, OVCAR3, COC1 | Cisplatin | Drug efflux | Notch1-ABCC1 | [195] |
SKOV3 | Cisplatin | OCSCs | YAP | [196] | ||
GAS5 | Down | HEY, A2780, HO8910, HO8910PM SKOV3, IOSE | Cisplatin | DNA repair; apoptosis inhibition | E2F4-PARP1-MAPK | [197] |
ZFAS1 | Up | SKOV3, Caov3, OVCAR3, A2780, COV644 | Cisplatin | Apoptosis inhibition | miR-548e-CXCR4- let-7a/BCL-XL/S | [198] |
CCAT1 | Up | A2780, SKOV3 | Cisplatin | Apoptosis inhibition | miR-454-survivin | [199] |
LINC00161 | Up | SKOV3 | Cisplatin | Apoptosis inhibition | miR-128-MAPK1 | [200] |
LINC01118 | Up | SKOV3, A2780, COC1 | Paclitaxel | Drug efflux | miR-134-ABCC1 | [201] |
CHRF | Up | ES2 | Cisplatin | EMT | miR-10b-STAT3 | [202] |
PANDAR | Up | SKOV3, HO-8910, HO8910PM, A2780 | Cisplatin | Apoptosis inhibition | SFRS2-P53/ P53-Ser15 | [203] |
PRLB | Up | CAOV3, SKOV3 | Paclitaxel | Apoptosis inhibition | RSF1-NF-κB | [204] |
SNHG5 | Down | HeyA8, SKOV3 | Paclitaxel | Apoptosis inhibition | miR-23a | [205] |
SNHG22 | Up | Hey, OAW28, COV362, OVCAR3, CAOV3, SKOV3, A2780 | Cisplatin | Apoptosis inhibition | miR-2467-Gal-1 | [206] |
LINC01125 | Down | SKOV3, A2780 | Cisplatin | Apoptosis inhibition | miR-1972 | [207] |
NEAT1 | Up | SKOV3, A2780 | Cisplatin | DNA repair | miR-770- 5p-PARP1 | [208] |
SKOV3, HeyA8 | Paclitaxel | EMT | miR-194-ZEB1 | [209] | ||
HOTAIR | Up | A2780, SKOV3, HEYC2, OV90, IOSE, IGROV, OVMUNA, OV90 | Cisplatin | DNA repair | NF-κB | [210] |
SKOV3, A2780 | Cisplatin | Autophagy | ATG7 | [211] | ||
OVCAR3, CAOV3, OVCAR5, COV362, Kuramochi, HOSEs | Cisplatin | OCSCs | EZH2 | [212] | ||
SKOV3, ES2, OVCAR3 | miR-206-TBX3 | [213] | ||||
UCA1 | Up | SKOV3, HeyA8 | Paclitaxel | Drug efflux | miR-129-ABCB1 | [214] |
OAW42, OVCAR3 | Cisplatin | Apoptosis inhibition | miR-27a- 5p-UBE2N | [215] | ||
A2780, SKOV3, IOSE80 | Cisplatin | Apoptosis inhibition | miR-143-FOSL2 | [216] | ||
A2780, OAW42, OVCAR4, SKOV3, HeyA8, IOSE-386 | Paclitaxel | Apoptosis inhibition | miR-654-5p-SIK2 | [217] | ||
ANRIL | Up | HOSEPiCs, SKOV3 | Cisplatin | Apoptosis inhibition | let-7a-HMGA2 | [218] |
TUG1 | Up | IOSE80, IOSE386 SKOV3, A2780 | Paclitaxel | Autophagy | miR-29b-3p | [219] |
IOSE80, A2780, SKOV3, HO8910 | - | OCSCs | 186-5p-ZEB1 | [220] | ||
FER1L4 | Down | IOSE80, HOSEpiC, OVCAR3, Caov3, SKOV3 | Paclitaxel | Apoptosis inhibition | MAPK | [221] |
H19 | Up | A2780 | Cisplatin | Apoptosis inhibition | EZH2-p21/PTEN | [222] |
SKOV3 | Carboplatin | OCSCs | miR-29b- 3p-STAT3 | [223] | ||
SNORD89 | Up | HOSEpiC, OVCAR3, CAOV3 | - | OCSCs | Notch1-c-Myc | [224] |
LINC01234 | Up | SKOV3, CAOV3, HO8910, A2780, IOSE80 | - | OCSCs | miRNA-27b- 5p-SIRT5 | [225] |
XIST | Down | SKOV3, ES2, TOV21G, RMG1 | Paclitaxel | OCSCs | miR-93- 5p-KMT2C | [226] |
Circular RNA | ||||||
circTNPO3 | Up | SKOV3, HeyA8, IOSE80 | Paclitaxel | Apoptosis inhibition | miR-1299-NEK2 | [227] |
circNRIP1 | Up | HOEC, A2780, SKOV3 | Paclitaxel | EMT | miR-211-5p-HOXC8 | [228] |
circ0000714 | Up | SKOV3, A2780 | Paclitaxel | Apoptosis inhibition | miR-370-3p-RAB17 | [229] |
circdr1as | Down | IOSE80, A2780, SKOV3 | Cisplatin | Apoptosis inhibition | miR-1270-SCAI | [230] |
circCELSR1 | Up | IOSE80, SKOV3, HeyA8 | Paclitaxel | Apoptosis inhibition | miR-1252-FOXR2 | [231] |
circFOXP1 | Up | COC1, OVCAR3, SKOV3, IOSE80 | Cisplatin | Apoptosis inhibition | miR-22-CEBPG, circFOXP1-miR -150-3p-FMNL3 | [232] |
circEXOC6B | Down | IOSE80, A2780, SKOV3 | Paclitaxel | Apoptosis inhibition | miR-376c- 3p-FOXO3 | [233] |
circ0000231 | Up | SKOV3 | Paclitaxel | EMT | miR-140-RAP1B | [234] |
circATL2 | Up | IOSE80, HEYA8, SKOV3 Cells | Paclitaxel | Apoptosis inhibition | miR-506-3p-NFIB | [235] |
circ0000745 | Up | IOSE80, CoC1, ES2, SW626, SKOV3 | - | OCSCs | miRNA-3187-3p-ERBB4/PI3K/AKT | [236] |
miRNA | ||||||
miR-9 | Down | CaOV3, SKOV3, OV2008, A2780 | Cisplatin; AG014699 | DNA repair | BRAC1 | [237] |
miR-21 | Up | SKOV3, A2780 | Cisplatin | Apoptosis inhibition | PDCD4-cIAP2 | [238] |
PA1 | - | OCSCs | - | [239] | ||
miR-26b | Down | SKOV3 | - | OCSCs | PTEN | [240] |
miR-27a | Down | A2780 | Paclitaxel | Drug efflux | HIPK2/MDR1/P-gp | [241] |
miR-29b | Down | ES2, AMOC2 | Paclitaxel | Apoptosis inhibition | BAG3/miR-29b/ MCL-1 | [242] |
miR-30a-5p | Down | Cisplatin resistance OC cell | Cisplatin | DNA repair | RIF1 | [243] |
miR-34c-5p | Down | OVS1, SKOV-I6 | Carboplatin | Apoptosis inhibition | AREG-EGFR-ERK | [244] |
miR-99a | Down | IOSE80, HO8910, SKOV3 | - | OCSCs | YY1 | [245] |
miR-106a | Up | SKOV3 | Paclitaxel | Apoptosis inhibition | caspase-7, BCL10 | [246] |
miR-591 | Down | SKOV3 | Paclitaxel | OCSCs | ZEB1 | [246] |
miR-125b | Up | OV2008 | Cisplatin | Apoptosis inhibition | BAK1 | [247] |
miR-130a | Down | A2780 | Cisplatin | Apoptosis inhibition | XIAP | [248] |
miR-137 | Down | SKOV3, A2780 | Cisplatin | Apoptosis inhibition | XIAP | [249] |
miR-139 | Down | CAOV3, SNU119 | Cisplatin | Drug efflux | ATP7A/B | [250] |
miR-142-5p | Down | OVCAR3, SKOV3 | Cisplatin | Apoptosis inhibition | XIAP, BIRC3, BCL2, BCL2L2, MCL1 | [251] |
miR-181a | Up | OV81.2-CP10, OV236, OCI-P5X, HEYA8 | Cisplatin | OCSCs | SFRP4 | [252] |
miR-181a | Down | OV90, SKOV3 | Cisplatin; carboplatin | OCSC | CD24-miR- 181a-MET | [253] |
miR-181a-2-3p | Down | OC tumor clinical tissues | - | OCSCs | EGR1 | [254] |
miR-182 | Up | T29, T80, HEY, OVCAR3, SKOV3, OV2008, 3AO, A2780, HO8910 | Cisplatin; taxol | Apoptosis inhibition | PDCD4 | [255] |
miR-200c | Down | Caov3, OVCAR5, OVCAR8 | - | OCSCs | Gab2 | [256] |
miR-205-5p | Up | OV2008 | Cisplatin | Apoptosis inhibition | PTEN/AKT | [257] |
miR-215 | Down | OVCAR3, CAOV3, SKOV3, HEY | Paclitaxel | Apoptosis inhibition | XIAP | [258] |
miR-216a | Up | SKOV3, OVCA433 | Cisplatin | Apoptosis inhibition | PTEN | [259] |
miR-216b | Down | SKOV3 | Cisplatin | DNA Repair | PARP1 | [260] |
miR-335-5p | Down | A2780 | Cisplatin | Apoptosis inhibition | BCL2L2 | [261] |
miR-363 | Down | OV2008, A2780s | Cisplatin | EMT | Snai1 | [262] |
miR-411 | Down | SKOV3, OVCAR3 | Cisplatin | Drug efflux | ABCG2 | [263] |
miR-429 | Down | SKOV3 | Cisplatin | OCSCs | ZEB1 | [264] |
miR-483-3p | Up | IGROV1 | Cisplatin | Apoptosis inhibition | PKC-alpha | [265] |
miR-490-3p | Down | SKOV3, OVCAR3 | Cisplatin | Drug efflux | ABCC2 | [266] |
miR-493-5p | Up | VU423, OVSAHO, kuramochi | Cisplatin | DNA repair | BRAC2 | [267] |
miR-503 | Down | SKOV3 | Cisplatin | Apoptosis inhibition | PI3K p85 | [268] |
miR-506 | Down | HeyA8, OVCA433, SKOV3 | Cisplatin; olaparib | DNA repair | RAD51 | [269] |
miR-506-3p | Down | SKOV3, CAOV3, OAW42, OV90 | Cisplatin | DNA repair | RAD17 | [270] |
miR-514 | Down | SKOV3, OVCA433 | Cisplatin | Drug efflux | ABCA1, ABCA10, ABCF2 | [271] |
miR-600 | Up | HO8910, A2780 | - | OCSCs | KLF9 | [272] |
miR-622 | Up | UWB1.289 | Cisplatin; olaparib | DNA repair | Ku complex | [273] |
miR-770-5p | Down | A2780S, OV2008 | Cisplatin | DNA repair | ERCC1 | [274] |
miR-873 | Down | OVCAR3, A2780 | Cisplatin | Drug efflux | ABCB1 | [275] |
miR-1301 | Down | SKOV3 | Cisplatin | Autophagy | E-cadherin, N-cadherin, ATG5, beclin1 | [276] |
Let-7d-3p | Up | SKOV3 | Carboplatin | Drug efflux | ABC transporters, HIF-1, RAS, ErbB | [277] |
Let-7e | Down | A2780, HO8910, ES2, CAOV3, SKOV3, OV2008 | Cisplatin | DNA repair | BRAC1, Rad51 | [278] |
A2780, SKOV3, Caov3 | PARP1 | [279] |
CONCLUSION
Chemoresistance poses a significant challenge in cancer treatment, contributing to elevated mortality rates among OC patients. Despite limited understanding, this review delineates four pivotal factors, namely transport systems, DNA repair, apoptosis, and OCSCs, along with the regulatory roles of ncRNA and nuclear receptors in conferring chemoresistance in OC. The involvement of specific transporters, including ABCB1, ABCC1, ABCC4, ABCC10, ABCG2, P-type ATPases ATP7A and ATP7B, and SLC transporters CTR1 and CTR2, are found in the development of chemoresistance. Additionally, proteins such as Bcl-2, Bcl-XL, MCL-1, Bax, Bim, XIAP, survivin, and cIAP2, along with ubiquitination proteins (HOIP, ITCH. CRL4 and UBR5), glycosylation, galectins (galectin-1, and -3), and epigenetic regulation (DNA methyltransferase, HDAC, and hMOF) contribute to chemoresistance in OC. Disruption of extrinsic pathways through TRAILR2 and FLIP further enhances chemoresistance. DNA repair mechanisms play a crucial role, with components like HR (BRCA1/2, RAD51, and its paralogs RAD51C, and RAD51D, RAD52, MRE11), NHEJ (RIF1), NER (ERCC1, XPF, RPA1), MMR (hMLH1, hMSH2), and BER (APE1, and XRCC1) identified as factors responsible for chemoresistance development. OCSCs are recognized as key contributors to tumor relapse, identified through various markers like CD133, CD24, CD44, CD117, ALDH1, SOX2, OCT4, NANOG, and EpCAM. ncRNA, as revealed in recent studies, exerts roles in tumor relapse by influencing drug efflux, apoptosis inhibition, DNA repair, EMT, autophagy, and OCSCs. Moreover, nuclear receptors (PPARγ, LXRα/β, PXR, CAR, COUP-TFγ, ERα, ERβ, ERRα AR, NGF1-B, NOR1) have emerged as significant contributors to chemoresistance in OC, modulating apoptosis, drug efflux, and OCSCs. The current treatment approach for the heterogeneous nature of OC lacks a multifactorial perspective. ncRNA and nuclear receptors, given their regulatory influence on multiple gene expressions, hold promise for targeted therapies. Exploring OCSCs further and understanding their role in promoting tumor relapse can guide effective interventions. Ongoing research utilizing advanced technology is expected to uncover additional resistance mechanisms, paving the way for tailored or combination therapies that enhance the survival of OC patients.
DECLARATIONS
Acknowledgment
The authors thank colleagues and researchers for their insightful discussion and constructive feedback. We extend our apologies to fellow researchers whose research could not be referenced due to limitations in available space.
Authors’ contributions
Conceptualized and constructed the outline of the review: Giri PK
Writing of the manuscript: Giri PK, Alam S
Availability of data and materials
The corresponding author may be contacted for any data inquiries.
Financial support and sponsorship
We gratefully acknowledge the support provided by the Ramalingaswami Re-entry Fellowship, DBT-INDIA (Grant No. BT/RLF/Re-entry/35/2018), and SRG-SERB-DST, India (Grant No. SRG/2019/002115). Additionally, Alam S is acknowledged for receiving support from the SAU-PhD scholarship.
Conflicts of interest
All authors declared that there are no conflicts of interest.
Ethical approval and consent to participate
Not applicable.
Consent for publication
Not applicable.
Copyright
© The Author(s) 2024.
REFERENCES
1. WHO. Global Cancer Observatory. Available from: https://gco.iarc.fr/. [Last accessed on 23 Feb 2024].
2. City of Hope. Ovarian cancer stages. Available from: https://www.cancercenter.com/cancer-types/ovarian-cancer/stages. [Last accessed on 23 Feb 2024].
3. American Cancer Society. Survival rates for ovarian cancer. Available from: https://www.cancer.org/cancer/types/ova rian-cancer/detection-diagnosis-staging/survival-rates.html. [Last accessed on 26 Feb 2024].
4. Wang Z, Zhu G. DNA Damage Repair Pathways and Repair of Cisplatin-Induced DNA Damage. Available from: https://www.semanticscholar.org/paper/DNA-Damage-Repair-Pathways-and-Repair-of-DNA-Damage-Wang-Zhu/de35aacc1d2ece7f2a067b86a9367176402e40c0. [Last accessed on 26 Feb 2024].
5. Tossetta G. Metformin improves ovarian cancer sensitivity to paclitaxel and platinum-based drugs: a review of in vitro findings. Int J Mol Sci 2022;23:12893.
6. Patel A, Iyer P, Matsuzaki S, Matsuo K, Sood AK, Fleming ND. Emerging trends in neoadjuvant chemotherapy for ovarian cancer. Cancers 2021;13:626.
7. Marchetti C, De Felice F, Romito A, et al. Chemotherapy resistance in epithelial ovarian cancer: mechanisms and emerging treatments. Semin Cancer Biol 2021;77:144-66.
8. Tossetta G, Marzioni D. Natural and synthetic compounds in ovarian cancer: a focus on NRF2/KEAP1 pathway. Pharmacol Res 2022;183:106365.
9. Tossetta G, Fantone S, Goteri G, Giannubilo SR, Ciavattini A, Marzioni D. The role of NQO1 in ovarian cancer. Int J Mol Sci 2023;24:7839.
10. Yang Y, Li S, Sun Y, Zhang D, Zhao Z, Liu L. Reversing platinum resistance in ovarian cancer multicellular spheroids by targeting Bcl-2. Onco Targets Ther 2019;12:897-906.
11. Yin L, Zeng Y, Zeng R, et al. Protein kinase RNA-activated controls mitotic progression and determines paclitaxel chemosensitivity through B-cell lymphoma 2 in ovarian cancer. Oncogene 2021;40:6772-85.
12. Villedieu M, Louis MH, Dutoit S, et al. Absence of Bcl-xL down-regulation in response to cisplatin is associated with chemoresistance in ovarian carcinoma cells. Gynecol Oncol 2007;105:31-44.
13. Wu X, Luo Q, Zhao P, et al. MGMT-activated DUB3 stabilizes MCL1 and drives chemoresistance in ovarian cancer. Proc Natl Acad Sci U S A 2019;116:2961-6.
14. Kale J, Kutuk O, Brito GC, et al. Phosphorylation switches Bax from promoting to inhibiting apoptosis thereby increasing drug resistance. EMBO Rep 2018;19:e45235.
15. Inoue-Yamauchi A, Oda H. EMT-inducing transcription factor ZEB1-associated resistance to the BCL-2/BCL-XL inhibitor is overcome by BIM upregulation in ovarian clear cell carcinoma cells. Biochem Biophys Res Commun 2020;526:612-7.
16. Rizzo A, Satta A, Garrone G, et al. Choline kinase alpha impairment overcomes TRAIL resistance in ovarian cancer cells. J Exp Clin Cancer Res 2021;40:5.
17. Abedini MR, Qiu Q, Yan X, Tsang BK. Possible role of FLICE-like inhibitory protein (FLIP) in chemoresistant ovarian cancer cells in vitro. Oncogene 2004;23:6997-7004.
18. Sapi E, Alvero AB, Chen W, et al. Resistance of ovarian carcinoma cells to docetaxel is XIAP dependent and reversible by phenoxodiol. Oncol Res 2004;14:567-78.
19. Ma JJ, Chen BL, Xin XY. XIAP gene downregulation by small interfering RNA inhibits proliferation, induces apoptosis, and reverses the cisplatin resistance of ovarian carcinoma. Eur J Obstet Gynecol Reprod Biol 2009;146:222-6.
20. Zaffaroni N, Pennati M, Colella G, et al. Expression of the anti-apoptotic gene survivin correlates with taxol resistance in human ovarian cancer. Cell Mol Life Sci 2002;59:1406-12.
21. Cohen S, Bruchim I, Graiver D, et al. Platinum-resistance in ovarian cancer cells is mediated by IL-6 secretion via the increased expression of its target cIAP-2. J Mol Med 2013;91:357-68.
22. Bradley A, Zheng H, Ziebarth A, et al. EDD enhances cell survival and cisplatin resistance and is a therapeutic target for epithelial ovarian cancer. Carcinogenesis 2014;35:1100-9.
23. Matsuura K, Huang NJ, Cocce K, Zhang L, Kornbluth S. Downregulation of the proapoptotic protein MOAP-1 by the UBR5 ubiquitin ligase and its role in ovarian cancer resistance to cisplatin. Oncogene 2017;36:1698-706.
24. Abedini MR, Muller EJ, Brun J, Bergeron R, Gray DA, Tsang BK. Cisplatin induces p53-dependent FLICE-like inhibitory protein ubiquitination in ovarian cancer cells. Cancer Res 2008;68:4511-7.
25. MacKay C, Carroll E, Ibrahim AFM, et al. E3 ubiquitin ligase HOIP attenuates apoptotic cell death induced by cisplatin. Cancer Res 2014;74:2246-57.
26. Hu X, Meng Y, Xu L, et al. Cul4 E3 ubiquitin ligase regulates ovarian cancer drug resistance by targeting the antiapoptotic protein BIRC3. Cell Death Dis 2019;10:104.
27. Schultz MJ, Swindall AF, Wright JW, Sztul ES, Landen CN, Bellis SL. ST6Gal-I sialyltransferase confers cisplatin resistance in ovarian tumor cells. J Ovarian Res 2013;6:25.
28. Connor JP, Felder M, Kapur A, Onujiogu N. DcR3 binds to ovarian cancer via heparan sulfate proteoglycans and modulates tumor cells response to platinum with corresponding alteration in the expression of BRCA1. BMC Cancer 2012;12:176.
29. Shu J, Dang L, Zhang D, et al. Dynamic analysis of proteomic alterations in response to N-linked glycosylation inhibition in a drug-resistant ovarian carcinoma cell line. FEBS J 2019;286:1594-605.
30. Zhang P, Zhang P, Shi B, et al. Galectin-1 overexpression promotes progression and chemoresistance to cisplatin in epithelial ovarian cancer. Cell Death Dis 2014;5:e991.
31. Wang D, You D, Li L. Galectin-3 regulates chemotherapy sensitivity in epithelial ovarian carcinoma via regulating mitochondrial function. J Toxicol Sci 2019;44:47-56.
32. Cai M, Xu S, Jin Y, et al. hMOF induces cisplatin resistance of ovarian cancer by regulating the stability and expression of MDM2. Cell Death Discov 2023;9:179.
33. Matei D, Fang F, Shen C, et al. Epigenetic resensitization to platinum in ovarian cancer. Cancer Res 2012;72:2197-205.
34. Jurkovicova D, Neophytou CM, Gašparović AČ, Gonçalves AC. DNA damage response in cancer therapy and resistance: challenges and opportunities. Int J Mol Sci 2022;23:14672.
35. Patch AM, Christie EL, Etemadmoghadam D, et al; Australian Ovarian Cancer Study Group. Whole-genome characterization of chemoresistant ovarian cancer. Nature 2015;521:489-94.
36. Norquist B, Wurz KA, Pennil CC, et al. Secondary somatic mutations restoring BRCA1/2 predict chemotherapy resistance in hereditary ovarian carcinomas. J Clin Oncol 2011;29:3008-15.
37. Dey G, Bharti R, Braley C, et al. LCK facilitates DNA damage repair by stabilizing RAD51 and BRCA1 in the nucleus of chemoresistant ovarian cancer. J Ovarian Res 2023;16:122.
38. Kondrashova O, Nguyen M, Shield-Artin K, et al; AOCS Study Group. Secondary somatic mutations restoring RAD51C and RAD51D associated with acquired resistance to the PARP inhibitor rucaparib in high-grade ovarian carcinoma. Cancer Discov 2017;7:984-98.
39. Rauth S, Ganguly K, Atri P, et al. Elevated PAF1-RAD52 axis confers chemoresistance to human cancers. Cell Rep 2023;42:112043.
40. Berkel C, Cacan E. Involvement of ATMIN-DYNLL1-MRN axis in the progression and aggressiveness of serous ovarian cancer. Biochem Biophys Res Commun 2021;570:74-81.
41. Sad LMAE, Mohamed DA, Elanwar NM, Elkady A. CXCR4 and RIF1 overexpression induces resistance of epithelial ovarian cancer to cisplatin-based chemotherapy. J Cancer Res Ther 2021;17:1454-61.
42. Mesquita KA, Alabdullah M, Griffin M, et al. ERCC1-XPF deficiency is a predictor of olaparib induced synthetic lethality and platinum sensitivity in epithelial ovarian cancers. Gynecol Oncol 2019;153:416-24.
43. Du P, Wang Y, Chen L, Gan Y, Wu Q. High ERCC1 expression is associated with platinum-resistance, but not survival in patients with epithelial ovarian cancer. Oncol Lett 2016;12:857-62.
44. Gao M, Guo G, Huang J, et al. DOCK7 protects against replication stress by promoting RPA stability on chromatin. Nucleic Acids Res 2021;49:3322-37.
45. Abdel-Fatah T, Sultana R, Abbotts R, et al. Clinicopathological and functional significance of XRCC1 expression in ovarian cancer. Int J Cancer 2013;132:2778-86.
46. Zhang Z, Xie Z, Sun G, et al. Reversing drug resistance of cisplatin by hsp90 inhibitors in human ovarian cancer cells. Int J Clin Exp Med 2015;8:6687-701.
47. Miao J, Zhang X, Tang QL, Wang XY, Kai L. Prediction value of XRCC 1 gene polymorphism on the survival of ovarian cancer treated by adjuvant chemotherapy. Asian Pac J Cancer Prev 2012;13:5007-10.
48. Al-Attar A, Gossage L, Fareed KR, et al. Human apurinic/apyrimidinic endonuclease (APE1) is a prognostic factor in ovarian, gastro-oesophageal and pancreatico-biliary cancers. Br J Cancer 2010;102:704-9.
49. Plumb JA, Strathdee G, Sludden J, Kaye SB, Brown R. Reversal of drug resistance in human tumor xenografts by 2’-deoxy-5-azacytidine-induced demethylation of the hMLH1 gene promoter. Cancer Res 2000;60:6039-44.
50. Tian H, Yan L, Xiao-Fei L, Hai-Yan S, Juan C, Shan K. Hypermethylation of mismatch repair gene hMSH2 associates with platinum-resistant disease in epithelial ovarian cancer. Clin Epigenetics 2019;11:153.
51. Zheng Z, Li X, Yang B, et al. SORL1 stabilizes ABCB1 to promote cisplatin resistance in ovarian cancer. Funct Integr Genomics 2023;23:147.
52. Wang Q, Wei X, Hu L, Zhuang L, Zhang H, Chen Q. Hedgehog-Gli2 signaling promotes chemoresistance in ovarian cancer cells by regulating MDR1. Front Oncol 2021;11:794959.
53. Tong X, Zhao J, Zhang Y, Mu P, Wang X. Expression levels of MRP1, GST-π, and GSK3β in ovarian cancer and the relationship with drug resistance and prognosis of patients. Oncol Lett 2019;18:22-8.
54. Wang JM, Liu BQ, Zhang Q, et al. ISG15 suppresses translation of ABCC2 via ISGylation of hnRNPA2B1 and enhances drug sensitivity in cisplatin resistant ovarian cancer cells. Biochim Biophys Acta Mol Cell Res 2020;1867:118647.
55. Jung M, Gao J, Cheung L, et al; Australian Ovarian Cancer Study. ABCC4/MRP4 contributes to the aggressiveness of Myc-associated epithelial ovarian cancer. Int J Cancer 2020;147:2225-38.
56. Wang JQ, Wu ZX, Yang Y, et al. Establishment and characterization of a novel multidrug resistant human ovarian cancer cell line with heterogenous MRP7 overexpression. Front Oncol 2021;11:731260.
57. He M, Wu H, Jiang Q, et al. Hypoxia-inducible factor-2α directly promotes BCRP expression and mediates the resistance of ovarian cancer stem cells to adriamycin. Mol Oncol 2019;13:403-21.
58. Lukanović D, Herzog M, Kobal B, Černe K. The contribution of copper efflux transporters ATP7A and ATP7B to chemoresistance and personalized medicine in ovarian cancer. Biomed Pharmacother 2020;129:110401.
59. Li T, Peng J, Zeng F, et al. Association between polymorphisms in CTR1, CTR2, ATP7A, and ATP7B and platinum resistance in epithelial ovarian cancer. Int J Clin Pharmacol Ther 2017;55:774-80.
60. Mangala LS, Zuzel V, Schmandt R, et al. Therapeutic targeting of ATP7B in ovarian carcinoma. Clin Cancer Res 2009;15:3770-80.
61. Petruzzelli R, Mariniello M, De Cegli R, et al. TFEB regulates ATP7B expression to promote platinum chemoresistance in human ovarian cancer cells. Cells 2022;11:219.
62. Kalayda GV, Wagner CH, Jaehde U. Relevance of copper transporter 1 for cisplatin resistance in human ovarian carcinoma cells. J Inorg Biochem 2012;116:1-10.
63. Lv X, Song J, Xue K, et al. Core fucosylation of copper transporter 1 plays a crucial role in cisplatin-resistance of epithelial ovarian cancer by regulating drug uptake. Mol Carcinog 2019;58:794-807.
64. Blair BG, Larson CA, Adams PL, Abada PB, Safaei R, Howell SB. Regulation of copper transporter 2 expression by copper and cisplatin in human ovarian carcinoma cells. Mol Pharmacol 2010;77:912-21.
66. Barzegar S, Pirouzpanah S. Zinc finger proteins and ATP-binding cassette transporter-dependent multidrug resistance. Eur J Clin Invest 2024;54:e14120.
67. Tian Y, Lei Y, Wang Y, Lai J, Wang J, Xia F. Mechanism of multidrug resistance to chemotherapy mediated by P‑glycoprotein (Review). Int J Oncol 2023;63:119.
68. Yin Y, Xin Y, Zhang F, et al. Overcoming ABCB1-mediated multidrug resistance by transcription factor BHLHE40. Neoplasia 2023;39:100891.
69. Sajid A, Rahman H, Ambudkar SV. Advances in the structure, mechanism and targeting of chemoresistance-linked ABC transporters. Nat Rev Cancer 2023;23:762-79.
70. Galetin A, Brouwer KLR, Tweedie D, et al. Membrane transporters in drug development and as determinants of precision medicine. Nat Rev Drug Discov 2024.
71. Hille S, Rein DT, Riffelmann M, et al. Anticancer drugs induce mdr1 gene expression in recurrent ovarian cancer. Anticancer Drugs 2006;17:1041-4.
72. Masanek U, Stammler G, Volm M. Messenger RNA expression of resistance proteins and related factors in human ovarian carcinoma cell lines resistant to doxorubicin, taxol and cisplatin. Anticancer Drugs 1997;8:189-98.
73. Pan J, Mendes LP, Yao M, et al. Polyamidoamine dendrimers-based nanomedicine for combination therapy with siRNA and chemotherapeutics to overcome multidrug resistance. Eur J Pharm Biopharm 2019;136:18-28.
74. Yang X, Iyer AK, Singh A, et al. MDR1 siRNA loaded hyaluronic acid-based CD44 targeted nanoparticle systems circumvent paclitaxel resistance in ovarian cancer. Sci Rep 2015;5:8509.
75. Sajid A, Lusvarghi S, Murakami M, et al. Reversing the direction of drug transport mediated by the human multidrug transporter P-glycoprotein. Proc Natl Acad Sci U S A 2020;117:29609-17.
76. Vahedi S, Chufan EE, Ambudkar SV. Global alteration of the drug-binding pocket of human P-glycoprotein (ABCB1) by substitution of fifteen conserved residues reveals a negative correlation between substrate size and transport efficiency. Biochem Pharmacol 2017;143:53-64.
77. Pietilä M, Sahgal P, Peuhu E, et al. SORLA regulates endosomal trafficking and oncogenic fitness of HER2. Nat Commun 2019;10:2340.
78. Kim JY, Bahar E, Lee JY, et al. ARL6IP5 reduces cisplatin-resistance by suppressing DNA repair and promoting apoptosis pathways in ovarian carcinoma. Cell Death Dis 2022;13:239.
79. Christie EL, Bowtell DDL. Acquired chemotherapy resistance in ovarian cancer. Ann Oncol 2017;28:viii13-5.
80. Miller JS, Bennett NE, Rhoades JA. Targeting hedgehog-driven mechanisms of drug-resistant cancers. Front Mol Biosci 2023;10:1286090.
81. Yin B, Lu P, Liang J, et al. The ABCB1 3435C > T polymorphism influences docetaxel transportation in ovarian cancer. J Int Med Res 2019;47:5256-69.
82. Ohishi Y, Oda Y, Uchiumi T, et al. ATP-binding cassette superfamily transporter gene expression in human primary ovarian carcinoma. Clin Cancer Res 2002;8:3767-75.
83. Ehrlichova M, Mohelnikova-Duchonova B, Hrdy J, et al. The association of taxane resistance genes with the clinical course of ovarian carcinoma. Genomics 2013;102:96-101.
84. Wang Y, Huang Z, Li B, Liu L, Huang C. The emerging roles and therapeutic implications of epigenetic modifications in ovarian cancer. Front Endocrinol 2022;13:863541.
85. Varier L, Sundaram SM, Gamit N, Warrier S. An overview of ovarian cancer: the role of cancer stem cells in chemoresistance and a precision medicine approach targeting the wnt pathway with the antagonist sFRP4. Cancers 2023;15:1275.
86. Chen DQ, Xie Y, Cao LQ, et al. The role of ABCC10/MRP7 in anti-cancer drug resistance and beyond. Drug Resist Updat 2024;73:101062.
87. Terraneo N, Jacob F, Dubrovska A, Grünberg J. Novel therapeutic strategies for ovarian cancer stem cells. Front Oncol 2020;10:319.
88. Dyla M, Kjærgaard M, Poulsen H, Nissen P. Structure and mechanism of P-type ATPase ion pumps. Annu Rev Biochem 2020;89:583-603.
89. Ortiz M, Wabel E, Mitchell K, Horibata S. Mechanisms of chemotherapy resistance in ovarian cancer. Cancer Drug Resist 2022;5:304-16.
90. Pan Z, Zhang H, Dokudovskaya S. The role of mTORC1 pathway and autophagy in resistance to platinum-based chemotherapeutics. Int J Mol Sci 2023;24:10651.
91. Bai X, Moraes TF, Reithmeier RAF. Structural biology of solute carrier (SLC) membrane transport proteins. Mol Membr Biol 2017;34:1-32.
92. Holzer AK, Katano K, Klomp LW, Howell SB. Cisplatin rapidly down-regulates its own influx transporter hCTR1 in cultured human ovarian carcinoma cells. Clin Cancer Res 2004;10:6744-9.
93. Holzer AK, Samimi G, Katano K, et al. The copper influx transporter human copper transport protein 1 regulates the uptake of cisplatin in human ovarian carcinoma cells. Mol Pharmacol 2004;66:817-23.
94. Song M, Cui M, Liu K. Therapeutic strategies to overcome cisplatin resistance in ovarian cancer. Eur J Med Chem 2022;232:114205.
95. Yoshida H, Teramae M, Yamauchi M, et al. Association of copper transporter expression with platinum resistance in epithelial ovarian cancer. Anticancer Res 2013;33:1409-14.
96. Bhartiya D, Patel H, Sharma D. Heterogeneity of stem cells in the ovary. In: Birbrair A, editor. Stem cells heterogeneity in different organs. Cham: Springer International Publishing; 2019. pp. 213-23.
97. Nowicki A, Kulus M, Wieczorkiewicz M, et al. Ovarian cancer and cancer stem cells - cellular and molecular characteristics, signaling pathways, and usefulness as a diagnostic tool in medicine and oncology. Cancers 2021;13:4178.
98. Testa U, Petrucci E, Pasquini L, Castelli G, Pelosi E. Ovarian cancers: genetic abnormalities, tumor heterogeneity and progression, clonal evolution and cancer stem cells. Medicines 2018;5:16.
99. Ahmed N, Kadife E, Raza A, Short M, Jubinsky PT, Kannourakis G. Ovarian cancer, cancer stem cells and current treatment strategies: a potential role of magmas in the current treatment methods. Cells 2020;9:719.
100. Somasagara RR, Spencer SM, Tripathi K, et al. RAD6 promotes DNA repair and stem cell signaling in ovarian cancer and is a promising therapeutic target to prevent and treat acquired chemoresistance. Oncogene 2017;36:6680-90.
101. Bareiss PM, Paczulla A, Wang H, et al. SOX2 expression associates with stem cell state in human ovarian carcinoma. Cancer Res 2013;73:5544-55.
102. Seo EJ, Kim DK, Jang IH, et al. Hypoxia-NOTCH1-SOX2 signaling is important for maintaining cancer stem cells in ovarian cancer. Oncotarget 2016;7:55624-38.
103. Wen Y, Hou Y, Huang Z, Cai J, Wang Z. SOX2 is required to maintain cancer stem cells in ovarian cancer. Cancer Sci 2017;108:719-31.
104. Xiang T, Long H, He L, et al. Interleukin-17 produced by tumor microenvironment promotes self-renewal of CD133+ cancer stem-like cells in ovarian cancer. Oncogene 2015;34:165-76.
105. Zhang S, Balch C, Chan MW, et al. Identification and characterization of ovarian cancer-initiating cells from primary human tumors. Cancer Res 2008;68:4311-20.
106. Alvero AB, Montagna MK, Holmberg JC, Craveiro V, Brown D, Mor G. Targeting the mitochondria activates two independent cell death pathways in ovarian cancer stem cells. Mol Cancer Ther 2011;10:1385-93.
107. Nunes T, Hamdan D, Leboeuf C, et al. Targeting cancer stem cells to overcome chemoresistance. Int J Mol Sci 2018;19:4036.
108. Ayub TH, Keyver-Paik MD, Debald M, et al. Accumulation of ALDH1-positive cells after neoadjuvant chemotherapy predicts treatment resistance and prognosticates poor outcome in ovarian cancer. Oncotarget 2015;6:16437-48.
109. Nakamura K, Terai Y, Tanabe A, et al. CD24 expression is a marker for predicting clinical outcome and regulates the epithelial-mesenchymal transition in ovarian cancer via both the Akt and ERK pathways. Oncol Rep 2017;37:3189-200.
110. Liu CL, Chen YJ, Fan MH, Liao YJ, Mao TL. Characteristics of CD133-sustained chemoresistant cancer stem-like cells in human ovarian carcinoma. Int J Mol Sci 2020;21:6467.
111. Motohara T, Katabuchi H. Ovarian cancer stemness: biological and clinical implications for metastasis and chemotherapy resistance. Cancers 2019;11:907.
112. Han T, Chen T, Chen L, et al. HLF promotes ovarian cancer progression and chemoresistance via regulating Hippo signaling pathway. Cell Death Dis 2023;14:606.
113. Alatise KL, Gardner S, Alexander-Bryant A. Mechanisms of drug resistance in ovarian cancer and associated gene targets. Cancers 2022;14:6246.
114. McAuliffe SM, Morgan SL, Wyant GA, et al. Targeting notch, a key pathway for ovarian cancer stem cells, sensitizes tumors to platinum therapy. Proc Natl Acad Sci U S A 2012;109:E2939-48.
115. Wang Z, Li R, Yang G, Wang Y. Cancer stem cell biomarkers and related signalling pathways. J Drug Target 2024;32:33-44.
116. Meirelles K, Benedict LA, Dombkowski D, et al. Human ovarian cancer stem/progenitor cells are stimulated by doxorubicin but inhibited by Mullerian inhibiting substance. Proc Natl Acad Sci U S A 2012;109:2358-63.
117. Królewska-Daszczyńska P, Wendlocha D, Smycz-Kubańska M, Stępień S, Mielczarek-Palacz A. Cancer stem cells markers in ovarian cancer: clinical and therapeutic significance (Review). Oncol Lett 2022;24:465.
118. Chau WK, Ip CK, Mak AS, Lai HC, Wong AS. c-Kit mediates chemoresistance and tumor-initiating capacity of ovarian cancer cells through activation of Wnt/β-catenin-ATP-binding cassette G2 signaling. Oncogene 2013;32:2767-81.
119. Januchowski R, Wojtowicz K, Sterzyſska K, et al. Inhibition of ALDH1A1 activity decreases expression of drug transporters and reduces chemotherapy resistance in ovarian cancer cell lines. Int J Biochem Cell Biol 2016;78:248-59.
120. Landen CN Jr, Goodman B, Katre AA, et al. Targeting aldehyde dehydrogenase cancer stem cells in ovarian cancer. Mol Cancer Ther 2010;9:3186-99.
121. Alvero AB, Chen R, Fu HH, et al. Molecular phenotyping of human ovarian cancer stem cells unravels the mechanisms for repair and chemoresistance. Cell Cycle 2009;8:158-66.
122. Shi MF, Jiao J, Lu WG, et al. Identification of cancer stem cell-like cells from human epithelial ovarian carcinoma cell line. Cell Mol Life Sci 2010;67:3915-25.
123. Sriramkumar S, Sood R, Huntington TD, et al. Platinum-induced mitochondrial OXPHOS contributes to cancer stem cell enrichment in ovarian cancer. J Transl Med 2022;20:246.
124. Ma H, Tian T, Cui Z. Targeting ovarian cancer stem cells: a new way out. Stem Cell Res Ther 2023;14:28.
125. Zhang L, Ma R, Gao M, et al. SNORA72 activates the Notch1/c-Myc pathway to promote stemness transformation of ovarian cancer cells. Front Cell Dev Biol 2020;8:583087.
126. Park JT, Chen X, Tropè CG, Davidson B, Shih IeM, Wang TL. Notch3 overexpression is related to the recurrence of ovarian cancer and confers resistance to carboplatin. Am J Pathol 2010;177:1087-94.
127. Pieterse Z, Amaya-Padilla MA, Singomat T, et al. Ovarian cancer stem cells and their role in drug resistance. Int J Biochem Cell Biol 2019;106:117-26.
128. Yang Y, Liu L, Tian Y, et al. Autophagy-driven regulation of cisplatin response in human cancers: exploring molecular and cell death dynamics. Cancer Lett 2024:216659.
129. Chen M, Lei N, Tian W, Li Y, Chang L. Recent advances of non-coding RNAs in ovarian cancer prognosis and therapeutics. Ther Adv Med Oncol 2022;14:17588359221118010.
130. Zhang X, Wang C, Xia S, et al. The emerging role of snoRNAs in human disease. Genes Dis 2023;10:2064-81.
131. Naz F, Tariq I, Ali S, Somaida A, Preis E, Bakowsky U. The role of long non-coding RNAs (lncRNAs) in female oriented cancers. Cancers 2021;13:6102.
132. Xu L, Zhang J, Sun J, et al. Epigenetic regulation of cancer stem cells: shedding light on the refractory/relapsed cancers. Biochem Pharmacol 2022;202:115110.
133. Si W, Shen J, Zheng H, Fan W. The role and mechanisms of action of microRNAs in cancer drug resistance. Clin Epigenetics 2019;11:25.
134. Kong M, Yu X, Zheng Q, Zhang S, Guo W. Oncogenic roles of LINC01234 in various forms of human cancer. Biomed Pharmacother 2022;154:113570.
135. Jafari RM, Tahan A, Askari MA, Roshandel H, Gharizadeh SMA, Farzaneh M. The role of LncRNA XIST in gynecologic cancers. CCTR 2023;19:172-6.
136. Qiu J, Sun Y, Ni H, Li L, Xi Q, Jiang H. Research progress of long non-coding RNA in ovarian cancer: a narrative review. Biotarget 2023;6:1.
137. Mirahmadi Y, Nabavi R, Taheri F, et al. MicroRNAs as biomarkers for early diagnosis, prognosis, and therapeutic targeting of ovarian cancer. J Oncol 2021;2021:3408937.
138. Hosea R, Hillary S, Wu S, Kasim V. Targeting transcription factor YY1 for cancer treatment: current strategies and future directions. Cancers 2023;15:3506.
139. Yang W, Kim D, Kim DK, Choi KU, Suh DS, Kim JH. Therapeutic strategies for targeting ovarian cancer stem cells. Int J Mol Sci 2021;22:5059.
140. Ismail A, Abulsoud AI, Fathi D, et al. The role of miRNAs in ovarian cancer pathogenesis and therapeutic resistance - a focus on signaling pathways interplay. Pathol Res Pract 2022;240:154222.
141. Zhao Y, Wei D, Zhang Y, Ji J. Panoramic view of microRNAs in regulating cancer stem cells. Essays Biochem 2022;66:345-58.
142. Singh A, Singh AK, Giri R, et al. The role of microRNA-21 in the onset and progression of cancer. Future Med Chem 2021;13:1885-906.
143. Najafi S. The emerging roles and potential applications of circular RNAs in ovarian cancer: a comprehensive review. J Cancer Res Clin Oncol 2023;149:2211-34.
144. Sohn EJ. Differentially expression and function of circular RNAs in ovarian cancer stem cells. J Ovarian Res 2022;15:97.
145. Sever R, Glass CK. Signaling by nuclear receptors. Cold Spring Harb Perspect Biol 2013;5:a016709.
146. Weikum ER, Liu X, Ortlund EA. The nuclear receptor superfamily: a structural perspective. Protein Sci 2018;27:1876-92.
147. Norouzi-Barough L, Sarookhani MR, Sharifi M, Moghbelinejad S, Jangjoo S, Salehi R. Molecular mechanisms of drug resistance in ovarian cancer. J Cell Physiol 2018;233:4546-62.
148. Zhao L, Zhou S, Gustafsson JÅ. Nuclear receptors: recent drug discovery for cancer therapies. Endocr Rev 2019;40:1207-49.
149. Bräutigam K, Biernath-Wüpping J, Bauerschlag DO, et al. Combined treatment with TRAIL and PPARγ ligands overcomes chemoresistance of ovarian cancer cell lines. J Cancer Res Clin Oncol 2011;137:875-86.
150. Kim S, Lee M, Dhanasekaran DN, Song YS. Activation of LXRα/β by cholesterol in malignant ascites promotes chemoresistance in ovarian cancer. BMC Cancer 2018;18:1232.
151. Masuyama H, Nakamura K, Nobumoto E, Hiramatsu Y. Inhibition of pregnane X receptor pathway contributes to the cell growth inhibition and apoptosis of anticancer agents in ovarian cancer cells. Int J Oncol 2016;49:1211-20.
152. Wang Y, Masuyama H, Nobumoto E, Zhang G, Hiramatsu Y. The inhibition of constitutive androstane receptor-mediated pathway enhances the effects of anticancer agents in ovarian cancer cells. Biochem Pharmacol 2014;90:356-66.
153. Li H, Zhang W, Niu C, et al. Nuclear orphan receptor NR2F6 confers cisplatin resistance in epithelial ovarian cancer cells by activating the Notch3 signaling pathway. Int J Cancer 2019;145:1921-34.
154. Matsumura S, Ohta T, Yamanouchi K, et al. Activation of estrogen receptor α by estradiol and cisplatin induces platinum-resistance in ovarian cancer cells. Cancer Biol Ther 2017;18:730-9.
155. He Y, Alejo S, Venkata PP, et al. Therapeutic targeting of ovarian cancer stem cells using estrogen receptor beta agonist. Int J Mol Sci 2022;23:7159.
156. Lam SS, Mak AS, Yam JW, Cheung AN, Ngan HY, Wong AS. Targeting estrogen-related receptor alpha inhibits epithelial-to-mesenchymal transition and stem cell properties of ovarian cancer cells. Mol Ther 2014;22:743-51.
157. Sun NK, Huang SL, Chang PY, Lu HP, Chao CC. Transcriptomic profiling of taxol-resistant ovarian cancer cells identifies FKBP5 and the androgen receptor as critical markers of chemotherapeutic response. Oncotarget 2014;5:11939-56.
158. Sun NK, Huang SL, Lu HP, Chang TC, Chao CC. Integrative transcriptomics-based identification of cryptic drivers of taxol-resistance genes in ovarian carcinoma cells: analysis of the androgen receptor. Oncotarget 2015;6:27065-82.
159. Chung WM, Ho YP, Chang WC, et al. Increase paclitaxel sensitivity to better suppress serous epithelial ovarian cancer via ablating androgen receptor/Aryl hydrocarbon receptor-ABCG2 axis. Cancers 2019;11:463.
160. Kohli A, Huang SL, Chang TC, Chao CC, Sun NK. H1.0 induces paclitaxel-resistance genes expression in ovarian cancer cells by recruiting GCN5 and androgen receptor. Cancer Sci 2022;113:2616-26.
161. Huang SL, Chang TC, Chao CCK, Sun NK. Role of the TLR4-androgen receptor axis and genistein in taxol-resistant ovarian cancer cells. Biochem Pharmacol 2020;177:113965.
162. Huang SL, Chang TC, Chao CCK, Sun NK. TLR4/IL-6/IRF1 signaling regulates androgen receptor expression: a potential therapeutic target to overcome taxol resistance in ovarian cancer. Biochem Pharmacol 2021;186:114456.
163. Ling K, Jiang L, Liang S, et al. Nanog interaction with the androgen receptor signaling axis induce ovarian cancer stem cell regulation: studies based on the CRISPR/Cas9 system. J Ovarian Res 2018;11:36.
164. Wilson AJ, Liu AY, Roland J, et al. TR3 modulates platinum resistance in ovarian cancer. Cancer Res 2013;73:4758-69.
165. Zhang K, Wang W, Chen L, et al. Cross-validation of genes potentially associated with neoadjuvant chemotherapy and platinum-based chemoresistance in epithelial ovarian carcinoma. Oncol Rep 2020;44:909-26.
166. Wang Y, Lei F, Lin Y, Han Y, Yang L, Tan H. Peroxisome proliferator-activated receptors as therapeutic target for cancer. J Cell Mol Med 2023;28:e17931.
167. Han N, Yuan M, Yan L, Tang H. Emerging insights into liver X receptor α in the tumorigenesis and therapeutics of human cancers. Biomolecules 2023;13:1184.
168. Gupta D, Venkatesh M, Wang H, et al. Expanding the roles for pregnane X receptor in cancer: proliferation and drug resistance in ovarian cancer. Clin Cancer Res 2008;14:5332-40.
169. Chai SC, Cherian MT, Wang YM, Chen T. Small-molecule modulators of PXR and CAR. Biochim Biophys Acta 2016;1859:1141-54.
170. Niu X, Wu T, Li G, Gu X, Tian Y, Cui H. Insights into the critical role of the PXR in preventing carcinogenesis and chemotherapeutic drug resistance. Int J Biol Sci 2022;18:742-59.
171. Alexandrova E, Pecoraro G, Sellitto A, et al. An overview of candidate therapeutic target genes in ovarian cancer. Cancers 2020;12:1470.
172. Wang Y, Qu Y, Zhang XL, et al. Autocrine production of interleukin-6 confers ovarian cancer cells resistance to tamoxifen via ER isoforms and SRC-1. Mol Cell Endocrinol 2014;382:791-803.
173. Wang Y, Guo XQ, Niu XL, Wu J, Zhu YQ, Mao LQ. [Relationship of IL-6 and IL-8 secretion in epithelial ovarian cancer cell lines with their sensitivity to tamoxifen as well as MAPK, Akt and estrogen receptor phosphorylation]. Xi Bao Yu Fen Zi Mian Yi Xue Za Zhi 2010;26:21-4. (in Chinese).
174. Mizushima T, Miyamoto H. The role of androgen receptor signaling in ovarian cancer. Cells 2019;8:176.
175. Sun NK, Kohli A, Huang SL, Chang TC, Chao CCK. Androgen receptor transcriptional activity and chromatin modifications on the ABCB1/MDR gene are critical for taxol resistance in ovarian cancer cells. J Cell Physiol 2019;234:8760-75.
176. Chung WM, Chen L, Chang WC, Su SY, Hung YC, Ma WL. Androgen/androgen receptor signaling in ovarian cancer: molecular regulation and therapeutic potentials. Int J Mol Sci 2021;22:7748.
177. Zou J. Site-specific delivery of cisplatin and paclitaxel mediated by liposomes: a promising approach in cancer chemotherapy. Environ Res 2023;238:117111.
178. Li H, Liu Y, Wang Y, Zhao X, Qi X. Hormone therapy for ovarian cancer: emphasis on mechanisms and applications (Review). Oncol Rep 2021;46:223.
179. Wu S, Yu K, Lian Z, Deng S. Molecular regulation of androgen receptors in major female reproductive system cancers. Int J Mol Sci 2022;23:7556.
180. Zheng H, Zhang M, Ma S, et al. Identification of the key genes associated with chemotherapy sensitivity in ovarian cancer patients. Cancer Med 2020;9:5200-9.
181. Zamarin D. Novel therapeutics: response and resistance in ovarian cancer. Int J Gynecol Cancer 2019;29:s16-21.
182. Han Y, Cai H, Ma L, et al. Nuclear orphan receptor NR4A2 confers chemoresistance and predicts unfavorable prognosis of colorectal carcinoma patients who received postoperative chemotherapy. Eur J Cancer 2013;49:3420-30.
183. Han Y, Cai H, Ma L, et al. Expression of orphan nuclear receptor NR4A2 in gastric cancer cells confers chemoresistance and predicts an unfavorable postoperative survival of gastric cancer patients with chemotherapy. Cancer 2013;119:3436-45.
184. Liu J, Jiao X, Gao Q. Neoadjuvant chemotherapy-related platinum resistance in ovarian cancer. Drug Discov Today 2020;25:1232-8.
185. Deng X, Zhang P, Liang T, Deng S, Chen X, Zhu L. Ovarian cancer stem cells induce the M2 polarization of macrophages through the PPARγ and NF-κB pathways. Int J Mol Med 2015;36:449-54.
186. Kumar V, Vashishta M, Kong L, et al. The role of notch, hedgehog, and wnt signaling pathways in the resistance of tumors to anticancer therapies. Front Cell Dev Biol 2021;9:650772.
187. Zhang M, Xu H, Zhang Y, et al. Research progress of estrogen receptor in ovarian cancer. Clin Exp Obstet Gynecol 2023;50:199.
188. Suster N, Virant-Klun I. Presence and role of stem cells in ovarian cancer. World J Stem Cells 2019;11:383-97.
189. Chung WM, Chang WC, Chen L, et al. Ligand-independent androgen receptors promote ovarian teratocarcinoma cell growth by stimulating self-renewal of cancer stem/progenitor cells. Stem Cell Res 2014;13:24-35.
190. Vasefifar P, Motafakkerazad R, Maleki LA, et al. Nanog, as a key cancer stem cell marker in tumor progression. Gene 2022;827:146448.
192. Le P, Romano G, Nana-Sinkam P, Acunzo M. Non-coding RNAs in cancer diagnosis and therapy: focus on lung cancer. Cancers 2021;13:1372.
193. Tian JH, Liu SH, Yu CY, Wu LG, Wang LB. The role of non-coding RNAs in breast cancer drug resistance. Front Oncol 2021;11:702082.
194. Lan H, Yuan J, Zeng D, et al. The emerging role of non-coding RNAs in drug resistance of ovarian cancer. Front Genet 2021;12:693259.
195. Bai L, Wang A, Zhang Y, Xu X, Zhang X. Knockdown of MALAT1 enhances chemosensitivity of ovarian cancer cells to cisplatin through inhibiting the Notch1 signaling pathway. Exp Cell Res 2018;366:161-71.
196. Wu X, Wang Y, Zhong W, Cheng H, Tian Z. The long non-coding RNA MALAT1 enhances ovarian cancer cell stemness by inhibiting YAP translocation from nucleus to cytoplasm. Med Sci Monit 2020;26:e922012.
197. Long X, Song K, Hu H, et al. Long non-coding RNA GAS5 inhibits DDP-resistance and tumor progression of epithelial ovarian cancer via GAS5-E2F4-PARP1-MAPK axis. J Exp Clin Cancer Res 2019;38:345.
198. Zhang J, Quan LN, Meng Q, et al. miR-548e sponged by ZFAS1 regulates metastasis and cisplatin resistance of OC by targeting CXCR4 and let-7a/BCL-XL/S signaling axis. Mol Ther Nucleic Acids 2020;20:621-38.
199. Wang DY, Li N, Cui YL. Long non-coding RNA CCAT1 sponges miR-454 to promote chemoresistance of ovarian cancer cells to cisplatin by regulation of surviving. Cancer Res Treat 2020;52:798-814.
200. Xu M, Zhou K, Wu Y, Wang L, Lu S. Linc00161 regulated the drug resistance of ovarian cancer by sponging microRNA-128 and modulating MAPK1. Mol Carcinog 2019;58:577-87.
201. Shi C, Wang M. LINC01118 modulates paclitaxel resistance of epithelial ovarian cancer by regulating miR-134/ABCC1. Med Sci Monit 2018;24:8831-9.
202. Tan WX, Sun G, Shangguan MY, et al. Novel role of lncRNA CHRF in cisplatin resistance of ovarian cancer is mediated by miR-10b induced EMT and STAT3 signaling. Sci Rep 2020;10:14768.
203. Wang H, Fang L, Jiang J, et al. The cisplatin-induced lncRNA PANDAR dictates the chemoresistance of ovarian cancer via regulating SFRS2-mediated p53 phosphorylation. Cell Death Dis 2018;9:1103.
204. Zhao Y, Hong L. lncRNA-PRLB confers paclitaxel resistance of ovarian cancer cells by regulating RSF1/NF-κB signaling pathway. Cancer Biother Radiopharm 2021;36:202-10.
205. Lin H, Shen L, Lin Q, et al. SNHG5 enhances paclitaxel sensitivity of ovarian cancer cells through sponging miR-23a. Biomed Pharmacother 2020;123:109711.
206. Zhang PF, Wu J, Luo JH, et al. SNHG22 overexpression indicates poor prognosis and induces chemotherapy resistance via the miR-2467/Gal-1 signaling pathway in epithelial ovarian carcinoma. Aging 2019;11:8204-16.
207. Guo J, Pan H. Long noncoding RNA LINC01125 enhances cisplatin sensitivity of ovarian cancer via miR-1972. Med Sci Monit 2019;25:9844-54.
208. Zhu M, Yang L, Wang X. NEAT1 knockdown suppresses the cisplatin resistance in ovarian cancer by regulating miR-770-5p/PARP1 axis. Cancer Manag Res 2020;12:7277-89.
209. An J, Lv W, Zhang Y. LncRNA NEAT1 contributes to paclitaxel resistance of ovarian cancer cells by regulating ZEB1 expression via miR-194. Onco Targets Ther 2017;10:5377-90.
210. Özeş AR, Miller DF, Özeş ON, et al. NF-κB-HOTAIR axis links DNA damage response, chemoresistance and cellular senescence in ovarian cancer. Oncogene 2016;35:5350-61.
211. Yu Y, Zhang X, Tian H, Zhang Z, Tian Y. Knockdown of long non-coding RNA HOTAIR increases cisplatin sensitivity in ovarian cancer by inhibiting cisplatin-induced autophagy. J BUON 2018;23:1396-401.
212. Wang W, Fang F, Ozes A, Nephew KP. Targeting ovarian cancer stem cells by dual inhibition of HOTAIR and DNA methylation. Mol Cancer Ther 2021;20:1092-101.
213. Zhang Y, Guo J, Cai E, et al. HOTAIR maintains the stemness of ovarian cancer stem cells via the miR-206/TBX3 axis. Exp Cell Res 2020;395:112218.
214. Wang J, Ye C, Liu J, Hu Y. UCA1 confers paclitaxel resistance to ovarian cancer through miR-129/ABCB1 axis. Biochem Biophys Res Commun 2018;501:1034-40.
215. Wambecke A, Ahmad M, Morice PM, et al. The lncRNA 'UCA1' modulates the response to chemotherapy of ovarian cancer through direct binding to miR-27a-5p and control of UBE2N levels. Mol Oncol 2021;15:3659-78.
216. Li Z, Niu H, Qin Q, et al. lncRNA UCA1 mediates resistance to cisplatin by regulating the miR-143/FOSL2-signaling pathway in ovarian cancer. Mol Ther Nucleic Acids 2019;17:92-101.
217. Li ZY, Wang XL, Dang Y, et al. Long non-coding RNA UCA1 promotes the progression of paclitaxel resistance in ovarian cancer by regulating the miR-654-5p/SIK2 axis. Eur Rev Med Pharmacol Sci 2020;24:591-603.
218. Miao JT, Gao JH, Chen YQ, Chen H, Meng HY, Lou G. LncRNA ANRIL affects the sensitivity of ovarian cancer to cisplatin via regulation of let-7a/HMGA2 axis. Biosci Rep 2019;39:BSR20182101.
219. Gu L, Li Q, Liu H, Lu X, Zhu M. Long noncoding RNA TUG1 promotes autophagy-associated paclitaxel resistance by sponging miR-29b-3p in ovarian cancer cells. Onco Targets Ther 2020;13:2007-19.
220. Zhan FL, Chen CF, Yao MZ. LncRNA TUG1 facilitates proliferation, invasion and stemness of ovarian cancer cell via miR-186-5p/ZEB1 axis. Cell Biochem Funct 2020;38:1069-78.
221. Liu S, Zou B, Tian T, et al. Overexpression of the lncRNA FER1L4 inhibits paclitaxel tolerance of ovarian cancer cells via the regulation of the MAPK signaling pathway. J Cell Biochem 2019;120:7581-9.
222. Sajadpoor Z, Amini-Farsani Z, Teimori H, et al. Valproic acid promotes apoptosis and cisplatin sensitivity through downregulation of H19 noncoding RNA in ovarian A2780 cells. Appl Biochem Biotechnol 2018;185:1132-44.
223. Tian X, Zuo X, Hou M, Li C, Teng Y. LncRNA-H19 regulates chemoresistance to carboplatin in epithelial ovarian cancer through microRNA-29b-3p and STAT3. J Cancer 2021;12:5712-22.
224. Zhu W, Niu J, He M, et al. SNORD89 promotes stemness phenotype of ovarian cancer cells by regulating Notch1-c-Myc pathway. J Transl Med 2019;17:259.
225. Ke F, Ren C, Zhai Z, et al. LINC01234 regulates microRNA-27b-5p to induce the migration, invasion and self-renewal of ovarian cancer stem cells through targeting SIRT5. Cell Cycle 2022;21:1020-33.
226. Huang R, Zhu L, Zhang Y. XIST lost induces ovarian cancer stem cells to acquire taxol resistance via a KMT2C-dependent way. Cancer Cell Int 2020;20:436.
227. Xia B, Zhao Z, Wu Y, Wang Y, Zhao Y, Wang J. Circular RNA circTNPO3 regulates paclitaxel resistance of ovarian cancer cells by miR-1299/NEK2 signaling pathway. Mol Ther Nucleic Acids 2020;21:780-91.
228. Li M, Cai J, Han X, Ren Y. Downregulation of circNRIP1 suppresses the paclitaxel resistance of ovarian cancer via regulating the miR-211-5p/HOXC8 axis. Cancer Manag Res 2020;12:9159-71.
229. Guo M, Li S, Zhao X, Yuan Y, Zhang B, Guan Y. Knockdown of circular RNA Hsa_circ_0000714 can regulate RAB17 by sponging miR-370-3p to reduce paclitaxel resistance of ovarian cancer through CDK6/RB pathway. Onco Targets Ther 2020;13:13211-24.
230. Zhao Z, Ji M, Wang Q, He N, Li Y. Circular RNA Cdr1as upregulates SCAI to suppress cisplatin resistance in ovarian cancer via miR-1270 suppression. Mol Ther Nucleic Acids 2019;18:24-33.
231. Zhang S, Cheng J, Quan C, et al. circCELSR1 (hsa_circ_0063809) contributes to paclitaxel resistance of ovarian cancer cells by regulating FOXR2 expression via miR-1252. Mol Ther Nucleic Acids 2020;19:718-30.
232. Luo Y, Gui R. Circulating exosomal circFoxp1 confers cisplatin resistance in epithelial ovarian cancer cells. J Gynecol Oncol 2020;31:e75.
233. Zheng Y, Li Z, Yang S, Wang Y, Luan Z. CircEXOC6B suppresses the proliferation and motility and sensitizes ovarian cancer cells to paclitaxel through miR-376c-3p/FOXO3 axis. Cancer Biother Radiopharm 2022;37:802-14.
234. Liu J, Wang H, Xiao S, Zhang S, Qi Y, Wang M. Circ_0000231 promotes paclitaxel resistance in ovarian cancer by regulating miR-140/RAP1B. Am J Cancer Res 2023;13:872-85.
235. Ying H, Zhao R, Yu Q, Zhang K, Deng Q. CircATL2 enhances paclitaxel resistance of ovarian cancer via impacting miR-506-3p/NFIB axis. Drug Dev Res 2022;83:512-24.
236. Wang S, Li Z, Zhu G, et al. RNA-binding protein IGF2BP2 enhances circ_0000745 abundancy and promotes aggressiveness and stemness of ovarian cancer cells via the microRNA-3187-3p/ERBB4/PI3K/AKT axis. J Ovarian Res 2021;14:154.
237. Sun C, Li N, Yang Z, et al. miR-9 regulation of BRCA1 and ovarian cancer sensitivity to cisplatin and PARP inhibition. J Natl Cancer Inst 2013;105:1750-8.
238. Chan JK, Blansit K, Kiet T, et al. The inhibition of miR-21 promotes apoptosis and chemosensitivity in ovarian cancer. Gynecol Oncol 2014;132:739-44.
239. Chung WM, Chang WC, Chen L, et al. MicroRNA-21 promotes the ovarian teratocarcinoma PA1 cell line by sustaining cancer stem/progenitor populations in vitro. Stem Cell Res Ther 2013;4:88.
240. Gao Z, Ye X, Bordeaux A, et al. miR-26b regulates cell proliferation and apoptosis of CD117+CD44+ ovarian cancer stem cells by targeting PTEN. Eur J Histochem 2021;65:3186.
241. Li Z, Hu S, Wang J, et al. MiR-27a modulates MDR1/P-glycoprotein expression by targeting HIPK2 in human ovarian cancer cells. Gynecol Oncol 2010;119:125-30.
242. Sugio A, Iwasaki M, Habata S, et al. BAG3 upregulates Mcl-1 through downregulation of miR-29b to induce anticancer drug resistance in ovarian cancer. Gynecol Oncol 2014;134:615-23.
243. Yao W, Wang Y, Huang M, et al. MiR-30a-5p enhances cisplatin sensitivity by downregulating RIF1 in ovarian cancer. Ann Clin Lab Sci 2023;53:418-26.
244. Tung SL, Huang WC, Hsu FC, et al. miRNA-34c-5p inhibits amphiregulin-induced ovarian cancer stemness and drug resistance via downregulation of the AREG-EGFR-ERK pathway. Oncogenesis 2017;6:e326.
245. Qian S, Wang W, Li M. Transcriptional factor Yin Yang 1 facilitates the stemness of ovarian cancer via suppressing miR-99a activity through enhancing its deacetylation level. Biomed Pharmacother 2020;126:110085.
246. Huh JH, Kim TH, Kim K, et al. Dysregulation of miR-106a and miR-591 confers paclitaxel resistance to ovarian cancer. Br J Cancer 2013;109:452-61.
247. Kong F, Sun C, Wang Z, et al. miR-125b confers resistance of ovarian cancer cells to cisplatin by targeting pro-apoptotic Bcl-2 antagonist killer 1. J Huazhong Univ Sci Technolog Med Sci 2011;31:543-9.
248. Zhang X, Huang L, Zhao Y, Tan W. Downregulation of miR-130a contributes to cisplatin resistance in ovarian cancer cells by targeting X-linked inhibitor of apoptosis (XIAP) directly. Acta Biochim Biophys Sin 2013;45:995-1001.
249. Li X, Chen W, Zeng W, Wan C, Duan S, Jiang S. microRNA-137 promotes apoptosis in ovarian cancer cells via the regulation of XIAP. Br J Cancer 2017;116:66-76.
250. Xiao F, Li Y, Wan Y, Xue M. MircroRNA-139 sensitizes ovarian cancer cell to cisplatin-based chemotherapy through regulation of ATP7A/B. Cancer Chemother Pharmacol 2018;81:935-47.
251. Li X, Chen W, Jin Y, et al. miR-142-5p enhances cisplatin-induced apoptosis in ovarian cancer cells by targeting multiple anti-apoptotic genes. Biochem Pharmacol 2019;161:98-112.
252. Belur Nagaraj A, Knarr M, Sekhar S, et al. The miR-181a-SFRP4 axis regulates wnt activation to drive stemness and platinum resistance in ovarian cancer. Cancer Res 2021;81:2044-55.
253. Kwon JE, Jang Y, Yun BS, et al. MET overexpression in ovarian cancer via CD24-induced downregulation of miR-181a: a signalling for cellular quiescence-like state and chemoresistance in ovarian CSCs. Cell Prolif 2023:e13582.
254. Wang L, Zhi X, Lu Y, et al. Identification of microRNA expression profiles of CD44+ ovarian cancer stem cells. Arch Gynecol Obstet 2022;306:461-72.
255. Wang YQ, Guo RD, Guo RM, Sheng W, Yin LR. MicroRNA-182 promotes cell growth, invasion, and chemoresistance by targeting programmed cell death 4 (PDCD4) in human ovarian carcinomas. J Cell Biochem 2013;114:1464-73.
256. Fang Z, Li T, Chen W, et al. Gab2 promotes cancer stem cell like properties and metastatic growth of ovarian cancer via downregulation of miR-200c. Exp Cell Res 2019;382:111462.
257. Shi X, Xiao L, Mao X, et al. miR-205-5p mediated downregulation of PTEN contributes to cisplatin resistance in C13K human ovarian cancer cells. Front Genet 2018;9:555.
258. Ge G, Zhang W, Niu L, Yan Y, Ren Y, Zou Y. miR-215 functions as a tumor suppressor in epithelial ovarian cancer through regulation of the X-chromosome-linked inhibitor of apoptosis. Oncol Rep 2016;35:1816-22.
259. Jin P, Liu Y, Wang R. STAT3 regulated miR-216a promotes ovarian cancer proliferation and cisplatin resistance. Biosci Rep 2018;38:BSR20180547.
260. Liu Y, Niu Z, Lin X, Tian Y. MiR-216b increases cisplatin sensitivity in ovarian cancer cells by targeting PARP1. Cancer Gene Ther 2017;24:208-14.
261. Liu R, Guo H, Lu S. MiR-335-5p restores cisplatin sensitivity in ovarian cancer cells through targeting BCL2L2. Cancer Med 2018;7:4598-609.
262. Cao L, Wan Q, Li F, Tang CE. MiR-363 inhibits cisplatin chemoresistance of epithelial ovarian cancer by regulating snail-induced epithelial-mesenchymal transition. BMB Rep 2018;51:456-61.
263. Chen FD, Chen HH, Ke SC, Zheng LR, Zheng XY. SLC27A2 regulates miR-411 to affect chemo-resistance in ovarian cancer. Neoplasma 2018;65:915-24.
264. Zou J, Liu L, Wang Q, et al. Downregulation of miR-429 contributes to the development of drug resistance in epithelial ovarian cancer by targeting ZEB1. Am J Transl Res 2017;9:1357-68.
265. Arrighetti N, Cossa G, De Cecco L, et al. PKC-alpha modulation by miR-483-3p in platinum-resistant ovarian carcinoma cells. Toxicol Appl Pharmacol 2016;310:9-19.
266. Tian J, Xu YY, Li L, Hao Q. MiR-490-3p sensitizes ovarian cancer cells to cisplatin by directly targeting ABCC2. Am J Transl Res 2017;9:1127-38.
267. Meghani K, Fuchs W, Detappe A, et al. Multifaceted impact of microRNA 493-5p on genome-stabilizing pathways induces platinum and PARP inhibitor resistance in BRCA2-mutated carcinomas. Cell Rep 2018;23:100-11.
268. Wu D, Lu P, Mi X, Miao J. Downregulation of miR-503 contributes to the development of drug resistance in ovarian cancer by targeting PI3K p85. Arch Gynecol Obstet 2018;297:699-707.
269. Liu G, Yang D, Rupaimoole R, et al. Augmentation of response to chemotherapy by microRNA-506 through regulation of RAD51 in serous ovarian cancers. J Natl Cancer Inst 2015;107:djv108.
270. Bagnoli M, Nicoletti R, Valitutti M, et al. Impairment of RAD17 functions by miR-506-3p as a novel synthetic lethal approach targeting DNA repair pathways in ovarian cancer. Front Oncol 2022;12:923508.
271. Xiao S, Zhang M, Liu C, Wang D. MiR-514 attenuates proliferation and increases chemoresistance by targeting ATP binding cassette subfamily in ovarian cancer. Mol Genet Genomics 2018;293:1159-67.
272. Shan L, Song P, Zhao Y, et al. miR-600 promotes ovarian cancer cells stemness, proliferation and metastasis via targeting KLF9. J Ovarian Res 2022;15:52.
273. Choi YE, Meghani K, Brault ME, et al. Platinum and PARP inhibitor resistance due to overexpression of microRNA-622 in BRCA1-mutant ovarian cancer. Cell Rep 2016;14:429-39.
274. Zhao H, Yu X, Ding Y, et al. MiR-770-5p inhibits cisplatin chemoresistance in human ovarian cancer by targeting ERCC2. Oncotarget 2016;7:53254-68.
275. Wu DD, Li XS, Meng XN, Yan J, Zong ZH. MicroRNA-873 mediates multidrug resistance in ovarian cancer cells by targeting ABCB1. Tumour Biol 2016;37:10499-506.
276. Yu JL, Gao X. MicroRNA 1301 inhibits cisplatin resistance in human ovarian cancer cells by regulating EMT and autophagy. Eur Rev Med Pharmacol Sci 2020;24:1688-96.
277. García-Vázquez R, Gallardo Rincón D, Ruiz-García E, et al. let-7d-3p is associated with apoptosis and response to neoadjuvant chemotherapy in ovarian cancer. Oncol Rep 2018;39:3086-94.
278. Xiao M, Cai J, Cai L, et al. Let-7e sensitizes epithelial ovarian cancer to cisplatin through repressing DNA double strand break repair. J Ovarian Res 2017;10:24.
Cite This Article
Export citation file: BibTeX | RIS
OAE Style
Alam S, Giri PK. Novel players in the development of chemoresistance in ovarian cancer: ovarian cancer stem cells, non-coding RNA and nuclear receptors. Cancer Drug Resist 2024;7:6. http://dx.doi.org/10.20517/cdr.2023.152
AMA Style
Alam S, Giri PK. Novel players in the development of chemoresistance in ovarian cancer: ovarian cancer stem cells, non-coding RNA and nuclear receptors. Cancer Drug Resistance. 2024; 7: 6. http://dx.doi.org/10.20517/cdr.2023.152
Chicago/Turabian Style
Alam, Shahil, Pankaj Kumar Giri. 2024. "Novel players in the development of chemoresistance in ovarian cancer: ovarian cancer stem cells, non-coding RNA and nuclear receptors" Cancer Drug Resistance. 7: 6. http://dx.doi.org/10.20517/cdr.2023.152
ACS Style
Alam, S.; Giri PK. Novel players in the development of chemoresistance in ovarian cancer: ovarian cancer stem cells, non-coding RNA and nuclear receptors. Cancer Drug Resist. 2024, 7, 6. http://dx.doi.org/10.20517/cdr.2023.152
About This Article
Special Issue
Copyright
Data & Comments
Data
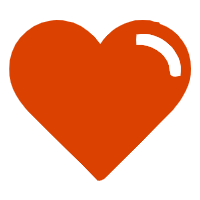

Comments
Comments must be written in English. Spam, offensive content, impersonation, and private information will not be permitted. If any comment is reported and identified as inappropriate content by OAE staff, the comment will be removed without notice. If you have any queries or need any help, please contact us at support@oaepublish.com.