Renin-angiotensin-system and clear cell renal carcinoma: research advances and future perspectives
Abstract
Hypertension is a known risk factor for clear cell renal cell carcinoma (ccRCC), yet the underlying mechanisms remain elusive. Studies have confirmed that the renin-angiotensin system (RAS) plays a role beyond regulating blood pressure, influencing various aspects of tumor development and metastasis. Generally, activation of the angiotensin-converting enzyme (ACE)/angiotensin II (Ang II)/angiotensin type 1 receptor (AT1R) axis elevates blood pressure and promotes tumor progression, while the activation of the angiotensin-converting enzyme 2 (ACE2)/[Ang-(1-7)]/Mas receptor (MasR) axis antagonizes these effects. Consequently, many cardiovascular drugs targeting the RAS may possess both hypotensive and antitumor properties. However, the role of RAS in ccRCC is controversial. To explore this, we reviewed the relevant literature. Surprisingly, apart from ACE2, the activation of RAS may facilitate the progression and metastasis of ccRCC. This unexpected finding suggests caution when using RAS inhibitors in ccRCC patients. This review provides an overview of the RAS, highlights research advances in RAS for ccRCC, elucidates the current status of RAS-targeted drugs in the treatment of ccRCC, and discusses the current challenges and future research directions in this field. In conclusion, the upregulation of other effector peptides and the activation of receptors in the RAS, apart from ACE2, may expedite ccRCC progression. Therefore, careful consideration is needed when using relevant drugs in ccRCC patients with hypertension. This synthesis of available evidence is crucial for informing the clinical management of ccRCC and guiding the development of novel therapeutic strategies.
Keywords
INTRODUCTION
Clear cell renal carcinoma (ccRCC) is the most common subtype of renal cell carcinoma, accounting for approximately 75%-85% of all cases[1]. The incidence of ccRCC has been increasing over the past few decades. The risk of developing ccRCC increases with age, with a significant increase in incidence in people over 40 years of age[2]. ccRCC is more prevalent in males than females, with a male-to-female ratio of approximately 2:1[2]. The prognosis for ccRCC patients depends on the stage and grade of the tumor at diagnosis. The 5-year overall survival rate for localized ccRCC is around 75%, while for metastatic disease, the 5-year survival rate is significantly lower, around 10%[3]. Risk factors such as smoking, obesity, and genetic predispositions contribute to a higher incidence of ccRCC. Additionally, the relative risk of ccRCC in individuals with hypertension is estimated to be 1.2 to 1.71 times higher compared to those without hypertension[4,5]. The elevated risk of ccRCC appears to be linked to long-term, chronic hypertension rather than short-term or recent-onset hypertension. Importantly, while the association between hypertension and ccRCC is well-established, the causal relationship remains unclear, necessitating further research to elucidate the underlying mechanisms.
The renin-angiotensin system (RAS) is a complex hormonal network crucial for regulating blood pressure, fluid and electrolyte balance, and other cardiovascular-related physiological and pathophysiological processes[6-9]. Two key axes of the RAS have been identified: the angiotensin-converting enzyme (ACE)/angiotensin (Ang) II/angiotensin type 1 receptor (AT1R) axis and the angiotensin-converting enzyme 2 (ACE2)/Ang-(1-7)/Mas receptor (MasR) axis. The former axis is often associated with the development of cardiovascular disease and hypertension, as it can upregulate inflammation and oxidative stress, while the latter axis is thought to counteract these effects. Given the established association between ccRCC and long-term hypertension and the central role of the RAS in blood pressure regulation, it has been hypothesized that the RAS may be implicated in the development and progression of ccRCC. Recent studies have confirmed that the RAS has been linked to the pathogenesis of various cancer types, including ccRCC. These findings present a promising avenue for investigating the potential anticancer effects of RAS inhibitors in the treatment of ccRCC. However, there remains a paucity of in-depth research elucidating the specific mechanisms by which the RAS contributes to the development and progression of ccRCC. Furthermore, there is a dearth of relevant drug testing to evaluate the efficacy of RAS-targeted therapies in the management of this malignancy. Additional research is necessary to fill these critical knowledge gaps.
To address the aforementioned questions, we conducted a search in the PubMed database for the most recent advancements in clinical, animal, and cell studies pertaining to RAS and ccRCC. This review aims to provide a concise introduction to the RAS, with a focus on the current research progress regarding its role in ccRCC, and explore the potential therapeutic implications of RAS-related drugs in the management of ccRCC. Figures and tables are included to facilitate comprehension. Additionally, the review discussed current challenges and future research directions in this field, and highlighted clinical implications of RAS for ccRCC treatment.
RAS
The classical RAS pathway ACE/Ang II/AT1R
RAS is an endocrine system expressed at multiple sites throughout the body, consisting of various effector peptides [Figure 1]. However, the expression levels of individual components within this system vary across different regions. Angiotensinogen, the precursor protein, is predominantly of hepatic origin and is cleaved by renin, a protease secreted by the juxtaglomerular cells, to generate Ang I[10]. ACE, expressed by vascular endothelial cells, then converts Ang I into Ang II, the primary effector peptide of the RAS[11].
Figure 1. Illustration of the RAS. The ACE/Ang II/AT1R axis and the ACE2/Ang-(1-7)/MasR axis represent the two most prominent and physiologically relevant pathways within the RAS. The delicate balance between the two axes is a key determinant of cardiovascular function and the regulation of inflammation and oxidative stress. ↑: To increase; RAS: renin-angiotensin system; ACE: angiotensin converting enzyme; Ang II: angiotensin II; ACE2: angiotensin converting enzyme 2; Ang: angiotensin; MasR: Mas receptor; APA: aminopeptidase; NEP: neprilysin; AT1R: Ang II type 1 receptor; AT2R: Ang II type 2 receptor; Mas: Ang-(1–7) receptor. Picture adapted from ref.[8]. In addition, we have made modifications and additions to the picture. Therefore, this picture does not involve any copyright issues.
Ang II exerts its physiological effects by activating two distinct receptor subtypes, the AT1R and the angiotensin type 2 receptor (AT2R), which exhibit different affinities for Ang II and elicit opposing functional responses[12-14]. Activation of AT1R leads to vasoconstriction, elevated blood pressure, and the promotion of inflammation and oxidative stress. Conversely, stimulation of AT2R induces vasodilation, decreased blood pressure, and anti-inflammatory, as well as antioxidant responses. Furthermore, Ang III is produced through the cleavage of Ang II by the enzyme aminopeptidase A (APA), and its physiological effects are largely equivalent to those of Ang II[15]. Similarly, Ang III binds to and activates both the AT1R and the AT2R, though it exhibits a lower affinity for the AT1R compared to Ang II, and a higher affinity for the AT2R relative to Ang II. Among the various components of the RAS, the Ang II/AT1R axis represents the most central regulatory signal, and its overactivation is implicated in the pathogenesis of numerous cardiovascular and kidney diseases.
The alternative RAS pathway ACE2/Ang-(1-7)/MasR
The ACE2/Ang-(1-7)/MasR axis represents an alternative, counter-regulatory pathway within the RAS. ACE2 is a homolog of the ACE, primarily functions to convert Ang I to Ang-(1-9) or Ang II to Ang-(1-7)[16,17]. This enzymatic conversion serves to counteract the effects of the classical RAS pathway by decreasing the levels of Ang II while increasing the levels of the vasodilatory and anti-inflammatory peptide, Ang-(1-7)[18-20]. The discovery of ACE2 has opened up new avenues for potential therapeutic interventions targeting the RAS for the treatment of cardiovascular and related diseases.
Ang-(1-7) can be generated through the cleavage of Ang II and Ang I. Upon binding to the Mas receptor, Ang-(1-7) counteracts many of the deleterious effects associated with the ACE/Ang II/AT1R axis[21,22]. In addition, ACE is the major route for the metabolism of Ang-(1-7) to Ang-(1-5)[23]. Previous studies have shown that the genetic knockout of the Mas receptor significantly impairs the ability of Ang-(1-7) to improve vascular physiology and pathophysiology, suggesting that the Mas receptor is the primary receptor through which Ang-(1-7) exerts its protective effects[9,24].
RAS IN CANCER AND ccRCC
Generally, the upregulation of ACE/Ang II/AT1R has pro-tumorigenic effects, whereas the upregulation of ACE2/Ang-(1-7)/MasR antagonizes the former[25-27]. Furthermore, the downregulation of ACE2/Ang-(1-7)/MasR may also increase tumor risk. In other words, a balance between the two axes is crucial. However, current studies do not fully endorse this opinion. In fact, both the Ang II/AT1R and the Ang-(1-7)/MasR signaling pathways may contribute to the progression of ccRCC. This section will discuss the role and putative mechanisms of RAS in the pathogenesis and progression of ccRCC. Given the paucity of studies specifically targeting RAS in ccRCC, the discussion will predominantly utilize insights from existing research on RAS’s involvement in other malignancies.
Mechanisms of RAS involvement in cancer development
The promotion of tumor growth by the Ang II/AT1R axis is associated with several key mechanisms. First, the activation of Ang II/AT1R signaling cascade upregulates the expression of pro-angiogenic factors, such as vascular endothelial growth factor (VEGF), basic fibroblast growth factor (BFGF), and angiopoietins, thereby enhancing tumor angiogenesis and increasing blood supply to the tumor[28]. Second, Ang II/AT1R activation stimulates a variety of intracellular signaling cascades, including the mitogen-activated protein kinase (MAPK)/ extracellular signal-regulated kinase (ERK), phosphoinositide 3-kinase (PI3K)/ a serine/threonine kinase (AKT), and Janus kinase (JAK)/signal transducer and activator of transcription (STAT) pathways, which collectively promote tumor cell proliferation. Third, Ang II/AT1R signaling can induce epithelial-mesenchymal transition (EMT), thereby enhancing tumor cell invasion and metastatic potential[29]. Fourth, Ang II/AT1R activation leads to the upregulation of pro-inflammatory cytokines, chemokines, and adhesion molecules, which not only creates a tumor-promoting inflammatory microenvironment but also suppresses antitumor immune responses, allowing the tumor to evade immune surveillance[30]. Finally, Ang II/AT1R signaling can promote oxidative stress within the tumor, which may contribute to genomic instability and the acquisition of additional oncogenic mutations, further driving tumor progression[31]. However, due to the limited studies specifically focused on the role of the Ang II/AT1R axis in ccRCC, the applicability of these mechanisms in fully explaining the formation and development of ccRCC may be constrained and requires further investigation.
The Ang-(1-7)/MasR axis acts oppositely to the Ang II/AT1R axis, significantly inhibiting tumor growth. In addition to the anti-angiogenic, anti-proliferative, anti-EMT, anti-inflammatory, and antioxidant effects described previously, Ang-(1-7)/MasR activation has also been shown to induce apoptosis in tumor cells. For instance, studies on hepatocellular carcinoma models have demonstrated that Ang-(1-7) inhibits tumor growth by suppressing tumor cell proliferation, promoting apoptosis, and inhibiting tumor angiogenesis[32]. These studies also revealed that Ang-(1-7) downregulates AT1R mRNA expression, upregulates AT2R and Mas mRNA levels, and inhibits p38-MAPK phosphorylation. Another study on tumor-induced cachexia has shown an antitumor effect of Mas receptor activation[33]. In vitro experiments revealed that overexpression or pharmacological activation of the Mas receptor ameliorated the muscle wasting induced by Colon-26 cancer cells. Moreover, in vivo studies demonstrated that Mas receptor activation inhibited tumor growth and alleviated muscle wasting.
Collectively, these studies have illustrated that the activated Ang II/AT1R axis promotes tumor growth, whereas the activated Ang-(1-7)/MasR axis inhibits it. Furthermore, the effects of ACE and ACE2 are realized by regulating the balance between Ang II and Ang-(1-7).
ACE and ACE2 in ccRCC
A 2010 clinical study found that ACE and ACE2 were generally downregulated in ccRCC, chromophobe renal cell carcinoma, and renal oncocytoma[34]. While this finding suggests a potential association between altered ACE and ACE2 expression and renal tumor pathogenesis, no specific correlation between ACE/ACE2 dysregulation and ccRCC was identified. This may be attributable to the lack of subgroup analysis in the study design. In contrast, another clinical investigation demonstrated that ACE, ACE2, and neprilysin (NEP) were highly expressed in ccRCC tissues and positively correlated with tumor invasiveness[35]. Moreover, NEP expression was associated with improved 15-year overall survival rates. Additionally, APA expression was inversely correlated with tumor grade in ccRCC, with the absence of APA linked to favorable 15-year survival outcomes. Subsequent blood-based assays revealed increased serum activities of ACE2, NEP, and APA in patients with renal tumors compared to healthy controls, while serum ACE activity was lower in individuals with high-grade and metastatic ccRCC. Recently, two bioinformatics analyses have implicated ACE inhibitors as potential therapeutic options for ccRCC[36,37]. Similarly, a recent pan-cancer bioinformatics study further found that high ACE2 expression was associated with improved prognosis specifically in ccRCC, suggesting a potential role for antihypertensive medications in the management of this malignancy[38]. Furthermore, a clinical study also demonstrated that higher ACE2 expression levels correlated with better overall survival in ccRCC patients[39]. Subsequent in vitro and animal studies have further indicated that ACE2 may inhibit tumor growth by upregulating Ang-(1-7) levels[39]. Collectively, these findings have suggested that ACE upregulation is associated with ccRCC development and invasiveness, whereas ACE2 upregulation is linked to antitumor effects and improved survival outcomes.
In addition, transmembrane protein 27 (TMEM27/collectrin), a homolog of ACE2 and potentially protective in the context of hypertension, has also been found to be associated with ccRCC[40,41]. Clinical studies have demonstrated that TMEM27 deficiency is linked to more aggressive ccRCC and poorer overall survival and disease-free survival in patients[42]. This may be one of the significant factors contributing to the promotion of ccRCC progression by hypertension.
Ang II/AT1R in ccRCC
The Ang II/AT1R axis has been extensively implicated in tumor development and metastasis across various cancer types, including ccRCC. However, the evidence specifically linking this pathway to ccRCC pathogenesis remains relatively limited, with only a few studies providing more indirect support. For instance, clinical investigations have shown that high expression of AT1R and AT2R in ccRCC specimens is associated with high-grade tumors and poorer progression-free survival[43]. Furthermore, in a mouse model of ccRCC, the AT1R antagonist telmisartan significantly reduced tumor growth by inducing tumor necrosis, decreasing VEGF levels, and inhibiting angiogenesis[44]. Additionally, studies utilizing the Ang II infusion model have explored the role of hypertension-associated mechanisms in ccRCC. In one such study, Wang et al. demonstrated that Ang II promoted tumor cell proliferation and migration in a mouse ccRCC model, an effect mediated by the downregulation of tissue inhibitor of metalloproteinase 3 (TIMP3)[5].
Ang-(1-7)/MasR in ccRCC
The role of the Ang-(1-7)/MasR axis has been studied less extensively in ccRCC compared to other cancer types. Only a limited number of studies have suggested that Ang-(1-7) may have potential therapeutic effects in ccRCC[39]. Interestingly, more evidence has indicated that activation of the Ang-(1-7)/MasR axis may promote the development and metastasis of ccRCC, which contradicts findings reported in other cancer models. For instance, a cellular study revealed that Ang-(1-7) enhanced the migration and invasion of 786-O and Caki-1 ccRCC cell lines, whereas antagonizing or silencing the Mas receptor abrogated the stimulatory effects of Ang-(1-7)[45]. Furthermore, the pro-migratory and pro-invasive effects of Ang-(1-7) in 786-O cells were found to be mediated through the AKT signaling pathway. Consistent with these in vitro findings, an animal study demonstrated that Ang-(1-7) dose-dependently promoted the growth and migration of Caki-1 and Caki-2 cells, and that the Mas receptor antagonist A779 did not block these pro-tumorigenic effects[46]. Notably, Ang-(1-7) did not affect cell proliferation or intratumoral vascular density in this model. Additionally, the NHERF4 has been shown to inhibit phospholipase C (PLC)/AKT signaling by directly binding to the Mas receptor, thereby suppressing the invasive potential of ccRCC[47]. Furthermore, a recent clinical study reported that the expression level of the Mas-related G protein-coupled receptor D (MrgD) in both the tumor center and the invasive front of ccRCC specimens was positively correlated with histological grade, tumor diameter, local invasion, regional lymph node involvement, and distant metastasis[48]. Collectively, these findings have suggested that, in contrast to the antitumor effects observed in other cancer types, the Ang-(1-7)/MasR axis may have pro-tumorigenic and pro-metastatic roles in the context of ccRCC.
Other effectors and metabolite
Beyond the primary bioactive peptides, the RAS encompasses a variety of effectors and metabolites, the functions of which in ccRCC remain largely unknown. A case in point is the vasoactive peptide bradykinin (BK), which serves as the optimal substrate for ACE and is quickly metabolized into its fragments, BK-(1-7) and subsequently BK-(1-5). To date, a single study has suggested a potential link between the BK receptor B2 and the prognosis of ccRCC[49]. Likewise, the (pro)renin receptor (PRR) emerges as an innovative receptor within the RAS. Ubiquitous expression of PRR is observed across kidney tumor subtypes, with particularly heightened staining intensities noted in chromophobe renal cell carcinomas (ChRCCs) and renal oncocytomas (ROs)[50]. The heightened expression of PRR at the core of the tumor and the advancing edge of the infiltrating ccRCC is significantly correlated with elevated tumor grade, increased diameter, localized invasion, and advanced staging[50]. Moreover, this elevated expression is markedly linked to an escalated risk of mortality. However, this association is not yet supported by substantial direct evidence or detailed mechanistic studies. Consequently, the involvement of RAS in ccRCC deserves further investigation to elucidate its underlying mechanisms and potential therapeutic implications.
Section summary
It has been shown that the level of Mas receptor expression in mouse arteries decreases with age, which may lead to a diminished vascular response to Ang-(1-7)[51,52]. Similarly, ACE2 and AT2R expression levels were simultaneously reduced[51]. Conversely, age is associated with increased expression of ACE, Ang II, and AT1R[51]. This suggests that the antitumor effect of ACE2 may be more closely related to the reduction of Ang II/AT1R signaling. Furthermore, the pathomechanisms and therapeutic outcomes of ccRCC may differ between younger and older patients.
Taken together, the available evidence suggests that only ACE2 has an antitumor effect and is associated with improved prognostic survival, while the remaining peptides and receptors in the RAS appear to promote tumor growth and metastasis [Figure 2 and Table 1]. This implies that ACE2 may be a critical target for the treatment of hypertension-associated ccRCC.
Figure 2. Of the components of the RAS, only ACE2 has been demonstrated to inhibit the development and metastasis of ccRCC. In contrast, activation of the remaining RAS signaling pathways is believed to promote tumor progression and metastasis. However, the underlying mechanisms driving these effects remain incompletely understood. ↑: To increase; ↓: to decrease; RAS: renin-angiotensin system; ACE2: angiotensin converting enzyme 2; ccRCC: clear cell renal cell carcinoma; ACE: angiotensin converting enzyme; Ang II: angiotensin II; AT1R: Ang II type 1 receptorplc; Ang: angiotensin; MasR: Mas receptor; TIMP3: tissue inhibitor of metalloproteinase 3; AKT: a serine/threonine kinase; PLC: phospholipase C. This picture is drawn by ourselves and there is no copyright issue involved.
The main references on the relationship between RAS and ccRCC
No. | Main research contents | Level of evidence | Ref. |
1 | ACE and ACE2 were downregulated in ccRCC. | Clinical observation | [34] |
2 | ACE, ACE2, and NEP were highly expressed in ccRCC tissues and positively correlated with tumor invasiveness. NEP expression was associated with improved 15-year overall survival rates. APA expression was inversely correlated with tumor grade in ccRCC. Increased serum activities of ACE2, NEP, and APA in patients with renal tumors compared to healthy controls, while serum ACE activity was lower in individuals with high-grade and metastatic ccRCC. | Clinical observation | [35] |
3 | Pan-cancer studies indicate that high ACE2 expression is only associated with improved prognosis in ccRCC. | Clinical observation | [38] |
4 | Higher levels of ACE2/Ang-(1-7) inhibit the proliferation of ccRCC. | Clinical observation and animal studies | [39] |
5 | TMEM27 deficiency is linked to more aggressive ccRCC and poorer overall survival and disease-free survival. | Clinical observation | [41] [42] |
6 | High expression of AT1R and AT2R in ccRCC specimens is associated with high-grade tumors and poorer progression-free survival. | Clinical observation | [43] |
7 | Telmisartan reduced tumor growth by inducing tumor necrosis, decreasing VEGF levels, and inhibiting angiogenesis. | Animal studies | [44] |
8 | Ang II promoted tumor cell proliferation and migration via downregulation of TIMP3. | Animal studies | [5] |
9 | Ang-(1-7) enhanced 786-O and Caki-1 ccRCC cell lines’ migration and invasion via mediating the AKT signaling pathway. Antagonizing or silencing Mas receptor abrogated its stimulatory effects. | Cellular studies | [45] |
10 | Ang-(1-7) dose-dependently promoted the growth and migration of Caki-1 and Caki-2 cells, and the Mas receptor antagonist A779 did not block these pro-tumorigenic effects. Ang-(1-7) did not affect cell proliferation or intratumoral vascular density. | Animal and cellular studies | [46] |
11 | The NHERF4 inhibits PLC/AKT signaling by directly binding to the Mas receptor, suppressing the invasive potential of ccRCC. | Cellular studies | [47] |
12 | The expression level of the MrgD in both the tumor center and the invasive front of ccRCC specimens was positively correlated with histological grade, tumor diameter, local invasion, regional lymph node involvement, and distant metastasis. | Clinical observation | [48] |
RAS-RELATED DRUGS IN CANCER AND ccRCC
ACE inhibitors and angiotensin receptor blockers (ARBs) are the two main RAS inhibitors, both of which are commonly used in the management of kidney and cardiovascular disease[25]. ACE inhibitors inhibit Ang II production, whereas ARBs specifically block Ang II binding to downstream receptors. Recent studies have found that ACE inhibitors and ARBs present anticancer efficacy related to inhibition of tumor metastasis, growth, and angiogenesis in some cancers. For instance, the common ARB losartan has been shown to reduce tumor growth rates in human xenograft models of pancreatic, breast, and prostate cancer[53-55]. Additionally, a study in prostate cancer demonstrated that ARBs such as telmisartan and candesartan were able to inhibit AT1R expression, induce apoptosis in tumor cells, and suppress their proliferation[56-58]. Similarly, studies on hepatocellular carcinoma have revealed that the ACE inhibitor captopril inhibits tumor angiogenesis and metastasis[59]. Furthermore, studies involving patients with rectal cancer have indicated a better response to neoadjuvant therapy when combined with ARBs or ACE inhibitors[60]. Collectively, clinical trials have generally shown that the use of RAS inhibitors significantly improves overall survival and patient outcomes across various cancer types[61,62]. Therefore, ACE inhibitors and ARBs have been proposed as potential therapeutic options for cancer.
However, the existing literature also presents divergent insights. A meta-analysis encompassing 8,818 publications found no significant effect of ARB use on cancer incidence in randomized controlled trials (OR 1.02, 95%CI 0.87-1.19; P = 0.803)[63]. Similarly, another meta-analysis based on 14 randomized controlled trials and 17 observational studies made a comparable observation[64]. Specifically, cancer incidence was lower among ARB/ACE inhibitor users in observational studies (RR = 0.82, 95%CI = 0.73-0.93); however, this association was not observed in randomized controlled trials (RR = 1.00, 95%CI = 0.92-1.08). Furthermore, the beneficial effects of ARBs/ACE inhibitors were only evident in lung cancer (RR 0.85, 95%CI 0.75-0.97). Interestingly, the relative risk of cancers associated with RAS blockade decreased with a longer duration of follow-up, and the reduction in mortality was of marginal significance with ARB/ACE inhibitor use in observational studies but not in randomized controlled trials. These findings suggest that the potential antitumor effects of RAS inhibitors may vary depending on the specific tumor type, research methodology, and duration of treatment.
Currently, there is a paucity of studies investigating the effects of RAS inhibitors in ccRCC. Drawing upon the existing findings regarding the impact of RAS inhibitors in other tumor types, it can be hypothesized that ARBs/ACE inhibitors may potentially exert inhibitory effects on ccRCC. However, further research is still warranted to substantiate this hypothesis.
CHALLENGES AND FUTURE PERSPECTIVES
Distal organ regulation and targeted drug delivery
Tumors commonly induce physiological dysfunction within the affected tissues. For instance, an imbalance in the intrarenal RAS significantly impacts patient prognosis and response to first-line immunotherapy[48]. However, the RAS is a ubiquitously expressed system across multiple organs, and locally produced RAS peptides may exert effects on distal organs through the circulatory system[65,66]. Recent studies have uncovered an intriguing phenomenon wherein overactivation of the RAS in the brain can influence chronic kidney disease (CKD)[67-69]. Furthermore, direct administration of trace amounts of RAS inhibitors into the brain can substantially ameliorate the progression of CKD[70-72]. These findings suggest that the development of hypertension-associated ccRCC may be related not only to local RAS imbalance but also to dysregulation of the RAS in distal organs. While non-targeted drug therapy can be beneficial in controlling hypertension, there is a potential risk of nonspecific side effects associated with long-term use. Therefore, precise diagnosis and targeted treatment of hypertension-associated ccRCC may help to mitigate this risk, and focused therapies could further reduce adverse effects by lowering the required drug doses.
Blood-brain barrier and engineered exosomes
When considering the role of the brain’s RAS in regulating the kidneys, a key issue that must be addressed is the blood-brain barrier (BBB)[73]. The BBB refers to the specialized barriers formed by the walls of the brain’s capillaries and the surrounding neuroglia, selectively preventing the entry of certain substances from the bloodstream into the brain tissue without interfering with the normal transport of essential nutrients. While the BBB is crucial for maintaining central nervous system (CNS) homeostasis, it also impedes the penetration of many drugs into the brain tissue, thereby limiting their therapeutic efficacy.
Exosomes are extracellular vesicles secreted by cells that serve as a means of intercellular communication by delivering their cargo of various biomolecular substrates[74]. The utilization of exosomes as drug delivery vehicles has emerged as a promising therapeutic strategy due to their inherently low immunogenicity and ability to penetrate tissue barriers. Notably, exosomes derived from macrophages, neutrophils, and natural killer (NK) cells possess membrane proteins with chemotactic properties, enabling them to localize at sites of injury and to cross the BBB[75-78]. Furthermore, biotechnological modification of exosomal membrane proteins can further enhance their brain-targeting capabilities. It has been demonstrated that incorporating specific peptides and ligands, such as c(RGDyK), AS1411 aptamer, neuropilin-1-targeting peptide, angiopep-2 peptide, T7 chimeric antigen receptor, transferrin receptor, and ANG-TRP-PK1, can significantly improve the BBB-penetrating ability of exosomes, thereby enhancing their utility as drug delivery systems targeting the CNS[78-84].
Challenges of clinical application of RAS drugs
While numerous RAS-targeted pharmacological agents have demonstrated promising results in preclinical studies for managing hypertension, their efficacy and safety profiles in the clinical control of hypertension-associated ccRCC remain uncertain. Furthermore, the sequential relationship between hypertension and the development of ccRCC is not well understood. It is plausible that the presence of tumors may lead to dysregulation of the kidney RAS, or RAS dysfunction may accelerate tumor growth. Consequently, strategically utilizing these pharmacological agents to optimize therapeutic benefits while minimizing adverse effects represents a valuable research direction for future investigation.
Tumor microenvironment
Tumor development and metastasis, including ccRCC, are closely intertwined with the tumor microenvironment and tumor-associated macrophages[85]. RAS has been shown to regulate the tumor microenvironment and modulate macrophage phenotypes through immunomodulatory mechanisms[86]. This suggests that the RAS may influence tumor progression by mediating alterations within the tumor microenvironment. While previous studies have primarily focused on the relationship between individual genes and ccRCC, investigating whether changes in the tumor microenvironment induced by the RAS can impact the trajectory of tumor progression remains a worthwhile endeavor.
Vasculogenic mimicry
Vasculogenic mimicry (VM) refers to the ability of aggressive cancer cells to form vascular-like channels that can transport blood, without the involvement of endothelial cells[87,88]. This process enables the tumor to obtain nutrients and oxygen, thereby promoting its growth and metastasis. Recent studies have explored the role of VM in ccRCC and found that its presence is often associated with poor prognosis[89-91]. Therefore, targeting VM may be a promising therapeutic approach for ccRCC.
Furthermore, 48 genes have been implicated in the formation of VM in osteosarcoma[92]. Several of these genes, such as matrix metalloproteinases (MMPs) and inflammatory factors, are also associated with RAS activation. A recent study on non-small cell lung cancer demonstrated that local RAS fluctuations can affect VM formation[88]. ACE2 and ACE inhibitors can promote VM formation through the Nodal/Notch4 signaling pathway and enhance VM structure by inhibiting VE-cadherin internalization. However, the study of the role of RAS in ccRCC-related VM is still lacking. Elucidating this relationship represents an important area for future research.
Stress and exercise
A long-term high-stress state frequently induces the body to secrete more stress hormones. This might trigger the activation of the RAS, subsequently increasing the generation of Ang II[93]. Additionally, recent studies propose that such long-term psychological stress can enhance the occurrence and development of cancer[94]. For instance, one study noticed that the levels of Ang II in serum and breast cancer genes (BRCA) tissues rose under chronic stress, resulting in accelerated BRCA growth in mouse models[95]. The AT1R inhibitor candesartan mitigated Ang II-induced cell proliferation and metastasis by suppressing the focal adhesion pathway PARP1/FN1. Subsequent clinical studies presented analogous outcomes[95]. Compared to patients in the control group, the levels of Ang II in tumor tissues and serum of stressed patients were higher, and the serum Ang II levels positively correlated with chronic stress indicators. Nevertheless, currently, there is no evidence indicating the relationship between stress and ccRCC, nor the role of RAS within it.
Conversely, moderate physical activity typically exerts a salutary regulatory influence on the RAS. It is widely acknowledged that exercise can ameliorate cardiovascular function, and enhance the elasticity and dilation capacity of blood vessels, all of which contribute to reducing the generation of Ang II and the activation of AT1R[96,97]. Furthermore, recent studies have also indicated that regular physical activities can retard the progression of cancer, including highly invasive malignant tumors[98-100]. Although the potential mechanisms related to the antitumor effect of exercise remain elusive, it is generally believed to be associated with exercise-stimulated infiltration of diverse immune cell subtypes into tumors[98]. There is also evidence suggesting that immune cells collected from the blood following exercise can be employed as adoptive cell therapy for cancer[100]. However, the role of exercise and exercise-mediated RAS in ccRCC remains indistinct.
LIMITATIONS
This study has several limitations. Firstly, the impact of RAS on ccRCC was only discovered in recent years, and the effect of RAS on ccRCC is not entirely consistent with that on other tumors. Thus, the mechanism by which RAS affects ccRCC remains uncertain. Secondly, although hypertension may accelerate the progression of ccRCC, the relationship between this mechanism and RAS is still undefined. Thirdly, it is also undetermined whether ccRCC can activate RAS. Fourthly, there are numerous drugs currently available for treating hypertension, but the efficacy of these drugs in treating ccRCC and its related hypertension is unclear. Fifthly, it remains unclear whether the current treatment plans for ccRCC will bring about blood pressure changes and RAS activation. Finally, current studies mainly focus on the influences of the two axes, namely ACE/Ang II/AT1R and ACE2/Ang-(1-7)/MasR, on ccRCC. The impacts of other effectors and metabolites in RAS on ccRCC remain under-researched. Many issues still await future resolution.
CONCLUSION
In conclusion, apart from ACE2 showing a protective effect, both the ACE/Ang II/AT1R and Ang-(1-7)/MasR axes may contribute to the progression and metastasis of ccRCC, underscoring the complex and multifaceted nature of the RAS in this malignancy. This also suggests caution in the use of hypertension medication in patients with hypertension-related ccRCC. Further research is warranted to fully elucidate the relative contributions and underlying mechanisms of these pathways in the context of ccRCC.
DECLARATIONS
Acknowledgments
We are grateful to all our colleagues in the clinical departments and laboratories.
Authors’ contributions
Writing and discussion of the article: Yang G, Wang Y, Lai ZW, Zhang H, Zhang Y, Song F
Drawing all the pictures and tables: Yang G
Agreed to the publication of the final version: Yang G, Wang Y, Lai ZW, Zhang H, Zhang Y, Song F
Availability of data and materials
Not applicable.
Financial support and sponsorship
This work was supported by Shenzhen Science and Technology Innovation Commission (JCYJ20220530150412026), Guangdong Basic and Applied Basic Research Foundation (2023A1515111112), and Shenzhen Clinical Medical Research Center for Urology and Nephrology.
Conflicts of interest
All authors declared that there are no conflicts of interest.
Ethical approval and consent to participate
Not applicable.
Consent for publication
Not applicable.
Copyright
© The Author(s) 2024.
REFERENCES
1. Moch H, Cubilla AL, Humphrey PA, Reuter VE, Ulbright TM. The 2016 WHO classification of tumours of the urinary system and male genital organs-part A: renal, penile, and testicular tumours. Eur Urol 2016;70:93-105.
2. Feng X, Zhang L, Tu W, Cang S. Frequency, incidence and survival outcomes of clear cell renal cell carcinoma in the United States from 1973 to 2014: a SEER-based analysis. Medicine (Baltimore) 2019;98:e16684.
3. Schiavoni V, Campagna R, Pozzi V, et al. Recent advances in the management of clear cell renal cell carcinoma: novel biomarkers and targeted therapies. Cancers (Basel) 2023;15:3207.
4. Kim SH, Park B, Hwang EC, et al. Retrospective multicenter long-term follow-up analysis of prognostic risk factors for recurrence-free, metastasis-free, cancer-specific, and overall survival after curative nephrectomy in non-metastatic renal cell carcinoma. Front Oncol 2019;9:859.
5. Wang C, Xu H, Liao X, et al. Hypertension promotes the proliferation and migration of ccRCC cells by downregulation of TIMP3 in tumor endothelial cells through the miR-21-5p/TGFBR2/P38/EGR1 Axis. Mol Cancer Res 2023;21:62-75.
6. Hammer A, Yang G, Friedrich J, et al. Role of the receptor Mas in macrophage-mediated inflammation in vivo. Proc Natl Acad Sci U S A 2016;113:14109-14.
7. Temme S, Yakoub M, Bouvain P, et al. Beyond vessel diameters: non-invasive monitoring of flow patterns and immune cell recruitment in murine abdominal aortic disorders by multiparametric MRI. Front Cardiovasc Med 2021;8:750251.
8. Yang G, Chu PL, Rump LC, Le TH, Stegbauer J. ACE2 and the homolog collectrin in the modulation of nitric oxide and oxidative stress in blood pressure homeostasis and vascular injury. Antioxid Redox Signal 2017;26:645-59.
9. Yang G, Istas G, Höges S, et al. Angiotensin-(1-7)-induced Mas receptor activation attenuates atherosclerosis through a nitric oxide-dependent mechanism in apolipoproteinE-KO mice. Pflugers Arch 2018;470:661-7.
10. Tan WSD, Liao W, Zhou S, Mei D, Wong WF. Targeting the renin-angiotensin system as novel therapeutic strategy for pulmonary diseases. Curr Opin Pharmacol 2018;40:9-17.
11. Perlot T, Penninger JM. ACE2 - from the renin-angiotensin system to gut microbiota and malnutrition. Microbes Infect 2013;15:866-73.
12. Bosnyak S, Jones ES, Christopoulos A, Aguilar MI, Thomas WG, Widdop RE. Relative affinity of angiotensin peptides and novel ligands at AT1 and AT2 receptors. Clin Sci (Lond) 2011;121:297-303.
13. Matysiak-Burzyńska ZE, Nowakowska M, Domińska K, Kowalska K, Płuciennik E, Piastowska-Ciesielska AW. Silencing of angiotensin receptor 1 interferes with angiotensin II oncogenic activity in endometrial cancer. J Cell Biochem 2018;119:9110-21.
14. Daugherty A, Manning MW, Cassis LA. Antagonism of AT2 receptors augments angiotensin II-induced abdominal aortic aneurysms and atherosclerosis. Br J Pharmacol 2001;134:865-70.
15. O'Connor AT, Haspula D, Alanazi AZ, Clark MA. Roles of angiotensin III in the brain and periphery. Peptides 2022;153:170802.
16. Tipnis SR, Hooper NM, Hyde R, Karran E, Christie G, Turner AJ. A human homolog of angiotensin-converting enzyme. cloning and functional expression as a captopril-insensitive carboxypeptidase. J Biol Chem 2000;275:33238-43.
17. Donoghue M, Hsieh F, Baronas E, et al. A novel angiotensin-converting enzyme-related carboxypeptidase (ACE2) converts angiotensin I to angiotensin 1-9. Circ Res 2000;87:E1-9.
18. Nakagawasai O, Takahashi K, Koyama T, Yamagata R, Nemoto W, Tan-No K. Activation of angiotensin-converting enzyme 2 produces an antidepressant-like effect via MAS receptors in mice. Mol Brain 2023;16:52.
19. Qaradakhi T, Gadanec LK, McSweeney KR, et al. The potential actions of angiotensin-converting enzyme II (ACE2) activator diminazene aceturate (DIZE) in various diseases. Clin Exp Pharmacol Physiol 2020;47:751-8.
20. Rushworth CA, Guy JL, Turner AJ. Residues affecting the chloride regulation and substrate selectivity of the angiotensin-converting enzymes (ACE and ACE2) identified by site-directed mutagenesis. FEBS J 2008;275:6033-42.
21. Santos RAS, Sampaio WO, Alzamora AC, et al. The ACE2/Angiotensin-(1-7)/MAS axis of the renin-angiotensin system: focus on Angiotensin-(1-7). Physiol Rev 2018;98:505-53.
22. Santos RA, Ferreira AJ, Verano-Braga T, Bader M. Angiotensin-converting enzyme 2, angiotensin-(1-7) and Mas: new players of the renin-angiotensin system. J Endocrinol 2013;216:R1-R17.
23. Chappell MC. Biochemical evaluation of the renin-angiotensin system: the good, bad, and absolute? Am J Physiol Heart Circ Physiol 2016;310:H137-52.
24. Santos RA, Simoes e Silva AC, Maric C, et al. Angiotensin-(1-7) is an endogenous ligand for the G protein-coupled receptor Mas. Proc Natl Acad Sci U S A 2003;100:8258-63.
25. Hassani B, Attar Z, Firouzabadi N. The renin-angiotensin-aldosterone system (RAAS) signaling pathways and cancer: foes versus allies. Cancer Cell Int 2023;23:254.
26. O'Rawe M, Kilmister EJ, Mantamadiotis T, Kaye AH, Tan ST, Wickremesekera AC. The renin-angiotensin system in the tumor microenvironment of glioblastoma. Cancers (Basel) 2021;13:4004.
27. Perini MV, Dmello RS, Nero TL, Chand AL. Evaluating the benefits of renin-angiotensin system inhibitors as cancer treatments. Pharmacol Ther 2020;211:107527.
28. Imai N, Hashimoto T, Kihara M, et al. Roles for host and tumor angiotensin II type 1 receptor in tumor growth and tumor-associated angiogenesis. Lab Invest 2007;87:189-98.
29. Nguyen L, Ager EI, Neo J, Christophi C. Regulation of colorectal cancer cell epithelial to mesenchymal transition by the renin angiotensin system. J Gastroenterol Hepatol 2016;31:1773-82.
30. Singh N, Baby D, Rajguru JP, Patil PB, Thakkannavar SS, Pujari VB. Inflammation and cancer. Ann Afr Med 2019;18:121-6.
32. Liu Y, Li B, Wang X, et al. Angiotensin-(1-7) suppresses hepatocellular carcinoma growth and angiogenesis via complex interactions of angiotensin II Type 1 Receptor, angiotensin II type 2 receptor and Mas receptor. Mol Med 2015;21:626-36.
33. Murphy KT, Hossain MI, Swiderski K, et al. Mas receptor activation slows tumor growth and attenuates muscle wasting in cancer. Cancer Res 2019;79:706-19.
34. Larrinaga G, Pérez I, Sanz B, et al. Angiotensin-converting enzymes (ACE and ACE2) are downregulated in renal tumors. Regul Pept 2010;165:218-23.
35. Errarte P, Beitia M, Perez I, et al. Expression and activity of angiotensin-regulating enzymes is associated with prognostic outcome in clear cell renal cell carcinoma patients. PLoS One 2017;12:e0181711.
36. Bai S, Wu Y, Yan Y, et al. Construct a circRNA/miRNA/mRNA regulatory network to explore potential pathogenesis and therapy options of clear cell renal cell carcinoma. Sci Rep 2020;10:13659.
37. Rehman A, Fatima I, Wang Y, et al. Unveiling the multi-target compounds of Rhazya stricta: discovery and inhibition of novel target genes for the treatment of clear cell renal cell carcinoma. Comput Biol Med 2023;165:107424.
38. Kim K, Ko Y, Ko DS, Kim YH. Prognostic significance of COVID-19 receptor ACE2 and recommendation for antihypertensive drug in renal cell carcinoma. Biomed Res Int 2020;2020:2054376.
39. Khanna P, Soh HJ, Chen CH, et al. ACE2 abrogates tumor resistance to VEGFR inhibitors suggesting angiotensin-(1-7) as a therapy for clear cell renal cell carcinoma. Sci Transl Med 2021;13:eabc0170.
40. Uhlen M, Zhang C, Lee S, et al. A pathology atlas of the human cancer transcriptome. Science 2017;357:eaan2507.
41. Javorhazy A, Farkas N, Beothe T, Pusztai C, Szanto A, Kovacs G. Lack of TMEM27 expression is associated with postoperative progression of clinically localized conventional renal cell carcinoma. J Cancer Res Clin Oncol 2016;142:1947-53.
42. Grewal R, Choung HYG, Roberts LL, et al. TMEM27 expression and clinical characteristics and survival in clear cell renal cell carcinoma. Acta Oncol 2022;61:1507-11.
43. Dolley-Hitze T, Jouan F, Martin B, et al. Angiotensin-2 receptors (AT1-R and AT2-R), new prognostic factors for renal clear-cell carcinoma? Br J Cancer 2010;103:1698-705.
44. Verhoest G, Dolley-Hitze T, Jouan F, et al. Sunitinib combined with angiotensin-2 type-1 receptor antagonists induces more necrosis: a murine xenograft model of renal cell carcinoma. Biomed Res Int 2014;2014:901371.
45. Zheng S, Yang Y, Song R, et al. Ang-(1-7) promotes the migration and invasion of human renal cell carcinoma cells via Mas-mediated AKT signaling pathway. Biochem Biophys Res Commun 2015;460:333-40.
46. Sobczuk P, Trzcinska-Danielewicz J, Koperski L, Girstun A, Cudnoch-Jedrzejewska A. Angiotensin-(1-7) can promote cell migration and tumor growth of clear cell renal cell carcinoma. J Physiol Pharmacol 2022:73.
47. Yang Y, Liang J, Zhao C, et al. NHERF4 hijacks Mas-mediated PLC/AKT signaling to suppress the invasive potential of clear cell renal cell carcinoma cells. Cancer Lett 2021;519:130-40.
48. Larrinaga G, Valdivia A, Arrieta-Aguirre I, et al. The expression of Alamandine receptor MrgD in clear cell renal cell carcinoma is associated with a worse prognosis and unfavorable response to antiangiogenic therapy. Int J Mol Sci 2024;25:1499.
49. Cui H, Xu L, Li Z, et al. Integrated bioinformatics analysis for the identification of potential key genes affecting the pathogenesis of clear cell renal cell carcinoma. Oncol Lett 2020;20:1573-84.
50. Solano-Iturri JD, Echevarría E, Unda M, et al. Clinical implications of (Pro)renin receptor (PRR) expression in renal tumours. Diagnostics (Basel) 2021;11:272.
51. Yoon HE, Kim EN, Kim MY, et al. Age-associated changes in the vascular renin-angiotensin system in mice. Oxid Med Cell Longev 2016;2016:6731093.
52. Costa-Fraga FP, Goncalves GK, Souza-Neto FP, et al. Age-related changes in vascular responses to angiotensin-(1-7) in female mice. J Renin Angiotensin Aldosterone Syst 2018;19:1470320318789332.
53. Chauhan VP, Martin JD, Liu H, et al. Angiotensin inhibition enhances drug delivery and potentiates chemotherapy by decompressing tumour blood vessels. Nat Commun 2013;4:2516.
54. Diop-Frimpong B, Chauhan VP, Krane S, Boucher Y, Jain RK. Losartan inhibits collagen I synthesis and improves the distribution and efficacy of nanotherapeutics in tumors. Proc Natl Acad Sci U S A 2011;108:2909-14.
55. Rhodes DR, Ateeq B, Cao Q, et al. AGTR1 overexpression defines a subset of breast cancer and confers sensitivity to losartan, an AGTR1 antagonist. Proc Natl Acad Sci U S A 2009;106:10284-9.
56. Alhusban A, Al-Azayzih A, Goc A, Gao F, Fagan SC, Somanath PR. Clinically relevant doses of candesartan inhibit growth of prostate tumor xenografts in vivo through modulation of tumor angiogenesis. J Pharmacol Exp Ther 2014;350:635-45.
57. Ishiguro H, Ishiguro Y, Kubota Y, Uemura H. Regulation of prostate cancer cell growth and PSA expression by angiotensin II receptor blocker with peroxisome proliferator-activated receptor gamma ligand like action. Prostate 2007;67:924-32.
58. Takahashi S, Uemura H, Seeni A, et al. Therapeutic targeting of angiotensin II receptor type 1 to regulate androgen receptor in prostate cancer. Prostate 2012;72:1559-72.
59. Neo JH, Malcontenti-Wilson C, Muralidharan V, Christophi C. Effect of ACE inhibitors and angiotensin II receptor antagonists in a mouse model of colorectal cancer liver metastases. J Gastroenterol Hepatol 2007;22:577-84.
60. Morris ZS, Saha S, Magnuson WJ, et al. Increased tumor response to neoadjuvant therapy among rectal cancer patients taking angiotensin-converting enzyme inhibitors or angiotensin receptor blockers. Cancer 2016;122:2487-95.
61. Li XY, Sun JF, Hu SQ. The renin-angiotensin system blockers as adjunctive therapy for cancer: a meta-analysis of survival outcome. Eur Rev Med Pharmacol Sci 2017; 21:1375-83.
62. Sun H, Li T, Zhuang R, Cai W, Zheng Y. Do renin-angiotensin system inhibitors influence the recurrence, metastasis, and survival in cancer patients? Medicine (Baltimore) 2017;96:e6394.
63. Datzmann T, Fuchs S, Andree D, Hohenstein B, Schmitt J, Schindler C. Systematic review and meta-analysis of randomised controlled clinical trial evidence refutes relationship between pharmacotherapy with angiotensin-receptor blockers and an increased risk of cancer. Eur J Intern Med 2019;64:1-9.
64. Shen J, Huang YM, Wang M, et al. Renin-angiotensin system blockade for the risk of cancer and death. J Renin Angiotensin Aldosterone Syst 2016;17:1470320316656679.
65. Reichhart N, Figura A, Skosyrski S, Strauß O. Control of the retinal local RAS by the RPE: an interface to systemic RAS activity. Exp Eye Res 2019;189:107838.
66. Cruz-López EO, Uijl E, Danser AHJ. Perivascular adipose tissue in vascular function: does locally synthesized angiotensinogen play a role? J Cardiovasc Pharmacol 2021;78:S53-62.
67. Cao W, Li A, Wang L, et al. A salt-induced reno-cerebral reflex activates renin-angiotensin systems and promotes CKD progression. J Am Soc Nephrol 2015;26:1619-33.
68. Liu Y, Li L, Qiu M, et al. Renal and cerebral RAS interaction contributes to diabetic kidney disease. Am J Transl Res 2019; 11:2925-39.
69. Ren L, Lu X, Danser AHJ. Revisiting the brain renin-angiotensin system-focus on novel therapies. Curr Hypertens Rep 2019;21:28.
70. Li J, Liang M, Zeng T, et al. Silencing of central (Pro)renin receptor ameliorates salt-induced renal injury in chronic kidney disease. Antioxid Redox Signal 2021;35:93-112.
71. Atkinson AJ Jr. Intracerebroventricular drug administration. Transl Clin Pharmacol 2017;25:117-24.
72. Cohen-Pfeffer JL, Gururangan S, Lester T, et al. Intracerebroventricular delivery as a safe, long-term route of drug administration. Pediatr Neurol 2017;67:23-35.
73. Chen J, Zhang D, Zhang J, Wang Y. Pathological changes in the brain after peripheral burns. Burns Trauma 2023;11:tkac061.
74. Yang G, Waheed S, Wang C, Shekh M, Li Z, Wu J. Exosomes and their bioengineering strategies in the cutaneous wound healing and related complications: current knowledge and future perspectives. Int J Biol Sci 2023;19:1430-54.
75. Wang J, Tang W, Yang M, et al. Inflammatory tumor microenvironment responsive neutrophil exosomes-based drug delivery system for targeted glioma therapy. Biomaterials 2021;273:120784.
76. Huang Z, Guo L, Huang L, Shi Y, Liang J, Zhao L. Baicalin-loaded macrophage-derived exosomes ameliorate ischemic brain injury via the antioxidative pathway. Mater Sci Eng C Mater Biol Appl 2021;126:112123.
77. Li F, Zhao L, Shi Y, Liang J. Edaravone-loaded macrophage-derived exosomes enhance neuroprotection in the rat permanent middle cerebral artery occlusion model of stroke. Mol Pharm 2020;17:3192-201.
78. Tao B, Du R, Zhang X, et al. Engineering CAR-NK cell derived exosome disguised nano-bombs for enhanced HER2 positive breast cancer brain metastasis therapy. J Control Release 2023;363:692-706.
79. Tian T, Zhang HX, He CP, et al. Surface functionalized exosomes as targeted drug delivery vehicles for cerebral ischemia therapy. Biomaterials 2018;150:137-49.
80. Wu T, Liu Y, Cao Y, Liu Z. Engineering macrophage exosome disguised biodegradable nanoplatform for enhanced sonodynamic therapy of glioblastoma. Adv Mater 2022;34:e2110364.
81. Jia G, Han Y, An Y, et al. NRP-1 targeted and cargo-loaded exosomes facilitate simultaneous imaging and therapy of glioma in vitro and in vivo. Biomaterials 2018;178:302-16.
82. Li B, Chen X, Qiu W, et al. Synchronous disintegration of ferroptosis defense axis via engineered exosome-conjugated magnetic nanoparticles for glioblastoma therapy. Adv Sci (Weinh) 2022;9:e2105451.
83. Liu J, Sun Y, Zeng X, et al. Engineering and characterization of an artificial drug-carrying vesicles nanoplatform for enhanced specifically targeted therapy of glioblastoma. Adv Mater 2023;35:e2303660.
84. Wang R, Wang X, Zhao H, et al. Targeted delivery of hybrid nanovesicles for enhanced brain penetration to achieve synergistic therapy of glioma. J Control Release 2024;365:331-47.
85. Xu W, Wu Y, Liu W, et al. Tumor-associated macrophage-derived chemokine CCL5 facilitates the progression and immunosuppressive tumor microenvironment of clear cell renal cell carcinoma. Int J Biol Sci 2022;18:4884-900.
86. Vallejo-Ardila DL, Fifis T, Burrell LM, Walsh K, Christophi C. Renin-angiotensin inhibitors reprogram tumor immune microenvironment: a comprehensive view of the influences on anti-tumor immunity. Oncotarget 2018;9:35500-11.
87. Maniotis AJ, Folberg R, Hess A, et al. Vascular channel formation by human melanoma cells in vivo and in vitro: vasculogenic mimicry. Am J Pathol 1999;155:739-52.
88. Xu K, Han H, Luo Y, Ye H, Lin H, Ni L. The angiotensin-converting enzyme inhibitory state promotes the transformation of non-small cell lung cancer blood supply pattern toward vasculogenic mimicry formation. Front Oncol 2021;11:663671.
89. Geng B, Liu W, Wang J, et al. The categorizations of vasculogenic mimicry in clear cell renal cell carcinoma unveil inherent connections with clinical and immune features. Front Pharmacol 2023;14:1333507.
90. Wang X, Yang R, Wang Q, Wang Y, Ci H, Wu S. Aberrant expression of vasculogenic mimicry, PRRX1, and CIP2A in clear cell renal cell carcinoma and its clinicopathological significance. Medicine (Baltimore) 2019;98:e17028.
91. Liu Q, Zhao E, Geng B, et al. Tumor-associated macrophage-derived exosomes transmitting miR-193a-5p promote the progression of renal cell carcinoma via TIMP2-dependent vasculogenic mimicry. Cell Death Dis 2022;13:382.
92. Yan L, Li R, Li D, Zhu Y, Lv Z, Wang B. Development of a novel vasculogenic mimicry-associated gene signature for the prognostic assessment of osteosarcoma patients. Clin Transl Oncol 2023;25:3501-18.
93. Peliky Fontes MA, Kangussu LM, Simões-e-Silva AC. Chapter 24 - the renin-angiotensin system, emotional stress and anxiety. In: Pilowsky PM, editors. Angiotensin from the kidney to coronavirus. Academic Press; 2023. p. 623-40.
94. Eckerling A, Ricon-Becker I, Sorski L, Sandbank E, Ben-Eliyahu S. Stress and cancer: mechanisms, significance and future directions. Nat Rev Cancer 2021;21:767-85.
95. Cui Y, Zhuang M, Huang Z, et al. An antihypertensive drug-AT1 inhibitor attenuated BRCA development promoted by chronic psychological stress via Ang II/PARP1/FN1 pathway. Biochim Biophys Acta Mol Basis Dis 2024;1870:167031.
96. Baffour-Awuah B, Man M, Goessler KF, et al. Effect of exercise training on the renin-angiotensin-aldosterone system: a meta-analysis. J Hum Hypertens 2024;38:89-101.
97. Kaschina E. Cross-talk between exercises and renin-angiotensin-aldosterone-system blockade in hypertension. Hypertens Res 2024;47:1981-3.
98. Wang Q, Zhou W. Roles and molecular mechanisms of physical exercise in cancer prevention and treatment. J Sport Health Sci 2021;10:201-10.
99. McTiernan A, Friedenreich CM, Katzmarzyk PT, et al. 2018 PHYSICAL ACTIVITY GUIDELINES ADVISORY COMMITTEE*. Physical activity in cancer prevention and survival: a systematic review. Med Sci Sports Exerc 2019;51:1252-61.
Cite This Article
How to Cite
Download Citation
Export Citation File:
Type of Import
Tips on Downloading Citation
Citation Manager File Format
Type of Import
Direct Import: When the Direct Import option is selected (the default state), a dialogue box will give you the option to Save or Open the downloaded citation data. Choosing Open will either launch your citation manager or give you a choice of applications with which to use the metadata. The Save option saves the file locally for later use.
Indirect Import: When the Indirect Import option is selected, the metadata is displayed and may be copied and pasted as needed.
About This Article
Copyright
Data & Comments
Data
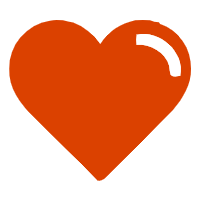
Comments
Comments must be written in English. Spam, offensive content, impersonation, and private information will not be permitted. If any comment is reported and identified as inappropriate content by OAE staff, the comment will be removed without notice. If you have any queries or need any help, please contact us at [email protected].