Optimal approach to stem cell mobilization in transplant-eligible multiple myeloma patients
Abstract
Multiple myeloma is a neoplastic disorder of plasma cells typically afflicting older individuals. Despite significant improvements in induction therapy and outcomes for transplant-ineligible newly diagnosed multiple myeloma (NDMM) patients, autologous stem cell transplant (ASCT) remains the standard of care for consolidation in transplant-eligible NDMM. Consequently, the optimal approach to stem cell mobilization remains critical. In this manuscript, we outline key updates to stem cell mobilization in NDMM patients. We describe the optimal number of cycles of induction prior to mobilization, emphasizing the need to balance the benefit of achieving improved depth of response by giving additional cycles of pre-transplant induction with the impact on stem cell yields. Additionally, we review ideal timing between the end of induction and initiation of mobilization to facilitate both a minimization of time off therapy as well as better communication and work flow between community providers and transplant centers. We also review different stem cell mobilization regimens with and without upfront CXCR4 inhibitors to optimize stem cell yields while considering both clinical outcomes and cost of care. Finally, we review optimal yields of CD34+ hematopoietic stem cells to minimize toxicity for ASCT and also consider optional utilization of stem cells for both salvage second ASCT and stem cell boosts for poor marrow function.
Keywords
INTRODUCTION
Multiple myeloma (MM) is a neoplastic disorder of plasma cells typically afflicting older individuals. It is the second most common hematological malignancy, with roughly 35,000 new cases diagnosed yearly, resulting in 13,000 deaths[1,2]. Consolidative autologous stem cell transplant (ASCT) has remained the standard of care for the treatment of transplant-eligible newly diagnosed multiple myeloma (NDMM) patients for over three decades. Over that time, both overall survival (OS) and progression-free rate (PFS) have dramatically improved across all age, gender, and ethnic groups[3]. However, novel therapeutics have revolutionized the treatment of MM and led to significantly improved outcomes in patients both eligible and ineligible for consolidative ASCT[4]. Allogenic stem cell transplants have also been previously used to treat MM. Although it can be a potentially curative treatment, they are rarely utilized given significant transplant-related risks and limited data supporting their use. They are currently considered investigational. Given their limited role in MM treatment, this review will not discuss allogenic stem cell transplants any further and will focus on ASCT[5,6].
Several recently completed landmark phase 3 trials have demonstrated excellent outcomes even in transplant-ineligible patients, leading some to question the ongoing role of consolidative ASCT. The MAIA trial evaluated daratumumab in addition to lenalidomide and dexamethasone (DRD), showing significantly increased OS and PFS in transplant-ineligible patients with a greater than 60% 4-year PFS[7]. In a separate phase 3 randomized trial, SWOG S0777 found that adding bortezomib to lenalidomide and dexamethasone (RVD) significantly improved PFS and OS in patients without an intent for immediate ASCT with a median PFS in the RVD arm of 41 months[8]. The phase III ENDURANCE trial compared carfilzomib, lenalidomide, and dexamethasone (KRD) and RVD, and both generated an excellent PFS with a median PFS of 34.6 months (95%CI: 28.8-37.8) in the KRd group and 34.4 months (30.1-not estimable) in the VRd group, but one approach was not superior to other with a different toxicity profile noted[9].
While the above-mentioned induction approaches in transplant-ineligible (or those without the intent for upfront ASCT) have shown promising outcomes, the utilization of upfront ASCT in combination with novel induction continues to be superior. Several recently completed randomized trials specifically evaluating the role of consolidative ASCT continue to show the improved PFS and possible OS advantage of consolidative ASCT, while other phase 2/3 trials incorporating CD38 monoclonal antibodies show deep responses with high rates of minimal residual disease (MRD) negativity and prolonged remissions in albeit shorter follow up [Table 1]. In two randomized trials, DETERMINATION and IFM 2009, RVD with upfront ASCT was compared to RVD without upfront ASCT (delayed ASCT was allowed at relapse)[10,11]. In both trials, upfront ASCT was associated with improved PFS and deeper responses. It is worth noting that OS was not improved in either trial. In a similar randomized trial, UNITO-MM-01/FORTE comparing KRD with upfront ASCT, KCD (carfilzomib with cyclophosphamide and dexamethasone) with upfront ASCT, and 12 cycles of KRD, the groups with upfront ASCT demonstrated improved PFS and recent multivariate analysis shows an OS advantage[12]. Finally, recent trials have demonstrated that quadruplet-based induction prior to ASCT significantly improves the depth of response and outcomes. In the phase 3 PERSEUS trial, it was found that adding daratumumab to RVD in transplant-eligible NDDM individuals improved minimal residual disease (MRD)-negative status (75.2% vs. 47.5%, P < 0.001) and PFS at 48 months
Outcomes of modern induction therapy and upfront autologous stem cell transplantation
Trial | Study design | CR or better rate | MRD negativity | mPFS | OS |
UNITO-MM-01/ FORTE[12] | KRd12 vs. KRd plus ASCT vs. KCd plus ASCT | KRd12 57%, KRd plus ASCT 54%, and KCd plus ASCT 42% (P = 0.016 for the overall comparison) | 56% KRd12, 62% KRd plus ASCT, and 43% KCd plus ASCT (P = 0·0032 for the overall comparison) by multiparameter flow cytometry at 10-5 sensitivity | 55.3 months for KRd12, median NR for KRd plus ASCT, and 53 months for KCd plus ASCT (KRd plus ASCT vs. KCd plus ASCT; P = 0.0008. KRd12 vs. KCd plus ASCT; P = 0.45. KRd plus ASCT vs. KRd12; P = 0.0084) | 4-year overall survival was 86% with KRd plus ASCT, 85% with KRd12, and 76% with KCd plus ASCT (no significant difference at follow-up) |
DETERMINTATION[10] | RVD vs. RVD plus ASCT | RVD 42.0% and RVD plus ASCT 46.8% (P = 0.99) | 40% RVD-alone and 54% RVD plus ASCT (OR: 0.55; unadjusted 95%CI: 0.30 to 1.01) by NGS at 10-5 sensitivity | 67.5 months for RVD plus ASCT and 46.2 months for RVD (risk of PD or death 53% higher in the RVD-alone group; HR: 1.53; 95%CI: 1.23 to 1.91; P < 0.001) | 5-year OS was 79.2% for RVD and 80.7% for RVD plus ASCT (HR: 1.10; 95%CI: 0.73 to 1.65) |
IFM 2009 study[11] | RVD vs. RVD plus ASCT | RVD 48% and RVD with ASCT 59% (P = 0.03) | 20% in the RVD-alone arm v 30% in the ASCT arm (OR for undetectable MRD 1.65; 95%CI: 1.10-2.49; P 5 .02) by NGF at 10-6 sensitivity | 36 months for RVD alone vs. 50 months for RVD plus ASCT (P < 0.001) | 4-year OS was 82% for RVD and 81% for RVD plus ASCT |
IsKia[14] | IsaKRd pre-ASCT induction and post-ASCT consolidation vs. KRd pre-ASCT induction and post-ASCT consolidation | IsaKRd 74% and KRd 72% | 77% IsaKRd and 67% KRd (OR 1.67; P = 0.049) by NGS at 10-5 sensitivity | Current follow-up (median, 20 months), no difference in PFS (95% at 1 year in both arms) | Median OS not reached for either arm. OS results pending |
Perseus[13] | D-VRd pre-ASCT induction and post-ASCT consolidation vs. VRd pre-ASCT induction and post-ASCT consolidation | D-VRd 87.9% and VRd 70.1% (P < 0.001) | 75.2% D-VRd group vs. 47.5% for VRd (P < 0.001) by NGS at 10-5 sensitivity | Median PFS not reached for either arm. At 48 months, 84.3% D-VRd group and 67.7% VRd group (HR: 0.42; 95%CI: 0.30 to 0.59; P < 0.001) | Median OS not reached for either arm. OS results pending |
Despite the advances made in non-transplant induction therapy, ASCT remains an important component in the treatment of MM and an ongoing standard of care in NDMM patients. Consequently, the optimal collection of stem cells remains critically important. In addition to utilizing stem cells for consolidative ASCT, additional uses exist, including at the time of relapse for salvage autologous stem cell transplantation (SAT) and for stem cell boosts in the setting of chimeric antigen T-cell therapy and as general support for chronic cytopenias in heavily pre-treated patients. This review will outline the optimal approach of mobilizing stem cells for upfront ASCT in MM patients in the context of the current myeloma treatment landscape.
OPTIMAL TIMING OF MOBILIZATION
The optimal timing of mobilization of stem cells is important to consider in MM patients eligible for ASCT. A key factor to consider is balancing the benefit or achieving improved depth of response by giving additional cycles of pre-transplant induction with the impact of impairing stem cell mobilization by the additional therapy. The benefit of deep pre-transplant response is clear, with multiple studies demonstrating that pre-transplant depth of response is associated with improved long-term outcomes including PFS and OS[16-19].
There is ample evidence that certain induction therapeutics such as melphalan, lenalidomide, and daratumumab can negatively impact stem cell collection. In addition, while quad-based regimens have improved outcomes as outlined above, four drug inductions such as Daratumumab-RVD can further impact stem cell yields. Frontline use of melphalan for induction therapy is discouraged and has been previously shown to increase stem cell mobilization failure[20]. Additionally, lenalidomide has been associated with increased rates of stem cell mobilization failure. In one retrospective analysis, it was found that more than three cycles of lenalidomide (P = 0.001) was significantly associated with increased rates of mobilization failure with G-CSF alone. In patients treated with greater than three cycles of lenalidomide, the failure rate was 78% (7 of 9) compared to 16% (9 of 55) in patients who received one to three cycles of lenalidomide[21]. Another retrospective analysis found that the median number of PBSCs collected in patients treated with lenalidomide was significantly less compared to patients who did not receive lenalidomide (6.34 vs. 7.52 × 106 CD34+ cells/kg; P = 0.0004), as was the median number of apheresis sessions needed for adequate collection of stem cells (2 vs. 1 sessions; P = 0.002)[22]. Daratumumab has also been associated with decreased stem cell yields. Findings from the GRIFFIN and MASTER trials demonstrated that adding daratumumab to standard triplet-based induction (KRD and RVD, respectively) had a negative impact on stem cell yields. The median total CD34 cell collection was 6.0 × 106/kg after D-KRd induction, 8.3 × 106/kg after D-RVd induction, and 9.4 × 106/kg after RVd induction. Additionally, the median days of collection required were higher in patients who received induction with D-KRd and D-RVd at two days compared to only one day for RVd. Notably, the cell yields decreased after quadruplet therapy despite increased plerixafor utilization. The plerixafor use rate was 97% in the D-KRd group, 72% in the D-RVd group, and 55% in the RVd group[23]. Similarly, another retrospective analysis found that patients who received induction with versus without daratumumab had a fewer number of stem cells collected
It is important to consider the maximum number of cycles of induction that can be given prior to collection. Old dogma recommended that no more than four cycles of induction should be given prior to mobilization of stem cells to prevent stem cell mobilization failure. Although an increased number of cycles of induction can lead to challenges with stem cell mobilization, we believe that up to six cycles can be given and adequate collection can still be achieved. In one prospective study, it was found that all patients treated with six cycles of lenalidomide-based triplet therapy were able to collect enough cells for autologous transplant without mobilization failure[25]. Another analysis found that induction therapy with up to six cycles of VRD and KRD did not lead to stem cell mobilization failure[26]. Further, relaxing requirements of induction cycles prior to transplant can facilitate easier communication and workflow with community physicians referring patients to distant transplant centers and allow ongoing deep remissions in patients prior to transplant, which, as above, has been shown to be beneficial. In addition to treatment-related factors putting patients at risk for poor mobilization, other patient-specific factors associated with poor mobilization include extensive bone marrow involvement with malignancy and older age[27,28].
An often-overlooked component of stem cell collection is the timing of stem cell mobilization after the last cycle of induction. An extended period of time between induction and mobilization should be avoided to decrease the risk of biochemical progression of disease while off therapy. Some mobilization protocols require as little as seven days off of therapy prior to stem cell mobilization[29]. Other protocols specify a maximum time of six weeks off of therapy but do not specify a minimum time[13]. It is imperative to avoid prolonged time between the end of induction and mobilization, as a shortened interval between completing induction and transplant is associated with improved outcomes. Notably, one retrospective analysis found that patients who had less than the median time (33 days) between the end of induction and transplant had a significantly improved PFS (35.6 vs. 32.1 months, P < 0.03) following transplant[30].
Unfortunately, there is no phase 3 trial evaluating the optimal number of induction cycles prior to collection or whether the optimal approach is to administer a fixed number of cycles or treat to depth of response. Given the conflicting data, lack of randomized trials specifically addressing optimal mobilization, and varying practice patterns, there is still some disagreement amongst experts regarding the optimal timing of collection, but most experts recommend 4-6 cycles of induction. The European Myeloma Network recommends that patients should not receive greater than 4-6 cycles of induction[31]. The IMWG consensus approach states that giving 3-6 cycles of induction before ASCT is a reasonable balance between minimum toxicity and maximum benefit[32]. The ASBMT does not make any recommendations about the number of cycles of induction prior to mobilization.[33]Figure 1 outlines our approach to induction and timing of mobilization.
Figure 1. Approach to stem cell mobilization in newly diagnosed multiple myeloma. CXCR4: Chemokine receptor type 4; Dara: daratumumab; DRD: daratumumab -lenalidomide-dexamethasone; G-CSF: granulocyte colony-stimulating factor; KRD: carfilzomib-lenalidomide-dexamethasone; PR: partial response; RVD: lenalidomide- bortezomib-dexamethasone; Vel: bortezomib.
OPTIMAL MOBILIZATION REGIMEN
Peripheral blood progenitor cells (PBPCs) are collected by apheresis after mobilization agents are administered. Mobilization strategies vary between institutions. Strategies for mobilization include granulocyte colony-stimulating factor (G-CSF), with or without a chemokine receptor type 4 (CXCR4) inhibitor (plerixafor, motixafortide), or chemomobilization [Table 2]. Additionally, new novel mobilization agents are currently being evaluated in trials, although they have not yet achieved widespread use[27]. Given that our institution does not recommend chemomobilization except in rare instances such as mobilization failure and it has not been permitted in recent landmark randomized trials such as the MASTER trial, we will not further address chemomobilization in this review. The typical dose of G-CSF is 10 μg/kg subcutaneously daily, with harvesting of stem cells beginning on day 5 and continuing daily until target stem cell yields are met. Mobilization with G-CSF alone typically takes a median of four days to collect a goal of 6 × 106 CD34+ cells/kg[34]. Two separate studies found that median stem cell yields with G-CSF alone after one day were 2.29 × 106 CD34+ cells/kg and 2.25 × 106 CD34+ cells/kg, respectively[35,36].
Mobilization strategies for peripheral blood progenitor cell collection
Doses | Day 1 yield (median) (all values × 106 CD34+ cells/kg) | Day 2 yield (median) (all values × 106 CD34+ cells/kg) | Total yield (median) (all values × 106 CD34+ cells/kg) | Notable toxicities | |
G-CSF[34] | 10 μg/kg | 2.29 | 1.78 × 106 CD34+ cells/kg | 6.18 | Nausea, fatigue, chest pain, cough |
G-CSF plus plerixafor[34] | 7.01 | 4.02 × 106 CD34+ cells/kg | 10.96 | Injection site reaction, nausea, diarrhea, fatigue, headache | |
G-CSF plus motixafortide[35] | Not specified | 10.8 | Not specified | Not specified | Injection site reactions, nausea, flushing, paresthesia, back pain, hypokalemia |
Risk-adapted strategy: Plerixafor 1[40] | Not specified | 2.4 | Not specified | 6.1 | As above, per standard G-CSF and plerixafor |
Risk-adapted strategy: Plerixafor 2[40] | Not specified | 3.7 | Not specified | 7.8 | As above, per standard G-CSF and plerixafor |
Several trials have demonstrated that G-CSF, in combination with plerixafor, leads to significantly improved mobilization and decreased mobilization failure compared to G-CSF alone. In a phase III randomized trial comparing G-CSF with placebo and G-CSF with plerixafor, the experimental group showed significantly improved stem cell yields. On day 1 of collection, the median stem cell yield in the plerixafor group was
Some institutions implement a risk-adapted strategy in which peripheral CD34 cell count is measured after patients receive G-CSF to identify patients who are poor mobilizers and require mobilization with plerixafor[40,41]. This strategy was designed to achieve cost savings by decreasing the use of costly CXCR4 inhibitors. One study analyzed stem cell yields and costs for two different risk-adapted algorithms. In the first algorithm (plerixafor-1), mobilization was initiated with G-CSF alone, and if peripheral blood CD34 counts on day 4 or day 5 were < 10/μL, plerixafor was administered. Furthermore, if on any day, the yield was < 0.5 × 106 CD34/kg, plerixafor was added. An additional algorithm (plerixafor-2) was also analyzed, which had lower thresholds for plerixafor administration. In this algorithm, plerixafor was added if day-4 PB CD34 < 10/μL for single or < 20/μL for multiple transplantations, or day-1 yield was < 1.5 × 106 CD34/kg, or any subsequent daily yield was < 0.5 × 106 CD34/kg. Stem cell yields were improved with the risk-adapted strategies. Median CD34 yield for G-CSF alone, plerixafor-1, and plerixafor-2 were 5.6, 6.1, and
COST OF MOBILIZATION
Mobilization of stem cells is a costly process, and although what is in the best medical interest of patients should be at the forefront of mobilization treatment plans, financial stewardship is also needed. Much of the cost of mobilization comes from the use of expensive mobilizing agents. Contracted prices of plerixafor vary between institutions, but costs typically exceed $7,000 per dose[42,43]. As previously mentioned, generic formulations of plerixafor are becoming available, so cost will hopefully decrease in the near future, but our experience with other generics shows that this is not always the case. Motixafortide is even more expensive, at around $12,000 per vial[44]. Although plerixafor and motixafortide add additional upfront costs to mobilization, some of these expenses are offset by reductions in days of additional collection and associated costs including nursing, apheresis, and additional lab work. Days of stem cell collection are expensive, with a variable reported cost per session ranging from $4,680 to $12,985[43,45]. The cost-effectiveness of plerixafor was demonstrated in a retrospective analysis comparing upfront plerixafor plus
In addition to the financial cost directly associated with additional days of collection, there are also more indirect costs such as the cost of transportation, the time spent by the patient at additional appointments, the stress and psychological cost to patients, and the additional burden on caregivers. It is important to note that improved stem cell yields with the use of CXCR4 inhibitors may also decrease post-transplant costs. As we will discuss in more detail later, improved stem cell transplant doses may lead to quicker engraftment, shorter hospitalizations, and fewer infusions, leading to decreased costs.
GOAL APHERESIS TARGETS
Research has shown that increased CD34+ autologous hematopoietic stem cell dose in ASCT is associated with improved outcomes. The international myeloma working group (IMWG) recommends that at least
Higher stem cell doses are associated with earlier engraftment. There is a known negative correlation between CD34 dose and time to both neutrophil and platelet engraftment[50] and engraftment kinetics demonstrate that infused CD34 dose and time to engraftment are negatively correlated. An early report found that if the infused CD34 dose was between 5.0 and 7.5 × 106/kg, there was a 95% chance of having neutrophil engraftment by day 15 and although a minimal threshold could not be defined, a dose
Data also support that higher doses of infused stem cells are associated with shorter hospital stays. This conclusion comes from a retrospective analysis of patients who underwent ASCT for various malignancies including non-Hodgkin lymphoma and MM. Patients infused with more than 5.0 × 106 cells/kg had 3.3 fewer hospital days. Additionally, patients infused with more than 5.0 × 106 cells/kg had lower resource utilization. In the group of patients who received more than 5.0 × 106 cells/kg, the mean number of platelet transfusions was 2.9 compared to 5.1, red blood cell transfusions were 2.6 compared to 3.4, and days of IV antibiotics were 6.5 compared to 12.0. This difference in resource utilization led to a difference in cost of care at $41,516 vs. $32,382[53].
HARVESTING ENOUGH CELLS FOR TWO TRANSPLANTS AND STEM CELL BOOSTS FOR POOR MARROW FUNCTION
An important question surrounding stem cell collection is whether enough cells should be collected to perform a salvage second transplant (SAT) if needed. SAT should be considered at the time of relapse in patients who sustained a prolonged remission after first transplant. Support for SAT comes from two randomized trials and a recently completed phase 2 trial, the CARFI study. The BSBMT/UKMF Myeloma X study compared a non-transplantation approach to SAT. In this Phase III, open-label, randomized trial conducted across multiple centers, patients who experienced a relapse following at least a 12-month progression-free survival (PFS) after upfront autologous stem cell transplantation (ASCT) underwent re-induction therapy. They were then randomized to receive either SAT or weekly cyclophosphamide for
The use of novel maintenance therapies after SAT has also improved the benefits that SAT provides. The CARFI study is a randomized phase II trial in which patients at initial relapse of MM were re-induced with four cycles of carfilzomib, cyclophosphamide, and dexamethasone and then underwent SAT. Following the transplant, they were randomized to either observation or maintenance with carfilzomib and dexamethasone. Maintenance therapy with carfilzomib and dexamethasone improved time to progression (TTP). Median TTP after randomization was 25.1 months (22.5‐NR) in the carfilzomib‐dexamethasone maintenance group and 16.7 months (14.4-21.8) in the control group (HR: 0.46, 95%CI: 0.30-0.71;
For MM patients with refractory disease and multiple relapses, chimeric antigen receptor (CAR)-T therapy should be considered. One potential complication of CAR-T therapy is prolonged cytopenias with hypoplastic bone marrow[59,60]. Retrospective analyses have reported the use of stored PBPCs for stem cell boosts is both effective in successfully recovering hematopoiesis and well tolerated[61,62]. It has also been proposed that stored PBPCs can be used as an alternative starting source for CAR-T cell manufacturing in heavily treated patients, which is being actively investigated[63].
Although harvesting additional stem cells for SAT has benefits, it also comes with significant financial costs. One analysis found that the cost per patient to harvest and store enough additional stem cells for SAT was US $16,590[64]. It is important to consider the associated costs given the low utilization rates of these cells. A retrospective analysis of a cohort of 400 MM patients who underwent ASCT found that at 6 years after transplant, only 12.0% of patients underwent SAT and an additional 1.9% of patients utilized stored cells for stem cell boost. Of note, none of the patients who were 70 years or older at the time of first transplant underwent SAT[65]. With the development of novel therapies, the number of patients undergoing SAT has been steadily decreasing since 1990[66]. Evolving treatment paradigms have decreased the utilization of stored stem cells for SAT, but utilization could potentially increase as novel uses of these cells are discovered.
CONCLUSION
ASCT remains an important therapy in the treatment of MM. Given the importance of ASCT, it is imperative to define the ideal approach to stem cell mobilization. The summary of our recommendations for optimal mobilization can be found in Figure 1. We recommend that patients receive 4-6 cycles of induction and not escalate or change therapy if patients have achieved at least PR. After completing induction, patients should be mobilized as soon as one week, and at the latest within 4 weeks to minimize delaying transplant. To optimize stem cell yields, we recommend that patients should receive G-CSF with upfront CXCR4 inhibitor. The minimum dose of CD34+ hematopoietic stem cells that should be given per transplant is at least 2 × 106 cells/kg with a goal dose of CD34+ hematopoietic stem cells of 4 × 106 cells/kg. We recommend harvesting enough stem cells for two transplants, although it is reasonable not to harvest enough cells for two transplants in older patients (i.e., older than 70) who are unlikely to utilize stored cells. As the MM landscape rapidly evolves, additional prospective trials are needed to further elucidate the optimal approach to hematopoietic stem cell mobilization, including optimal doses to store for both hematopoietic stem cell boosts in addition to salvage second transplant, cost-saving approaches to both collection and storage of hematopoietic stem cells, and potentially collecting and storing healthier t-cells early in the MM disease course for future CAR-T therapy.
DECLARATIONS
Authors’ contributions
Contributed to the manuscript and approved the final version: Fitzsimons J, Hagen PA
Availability of data and materials
Not applicable.
Financial support and sponsorship
None.
Conflicts of interest
Both authors declared that there are no conflicts of interest.
Ethical approval and consent to participate
Not applicable.
Consent for publication
Not applicable.
Copyright
© The Author(s) 2024.
REFERENCES
1. Key statistics about multiple myeloma. Available from: https://www.cancer.org/cancer/types/multiple-myeloma/about/key-statistics.html [Last accessed on 21 May 2024].
2. Cancer stat facts: myeloma. Available from: https://seer.cancer.gov/statfacts/html/mulmy.html [Last accessed on 21 May 2024].
3. Costa LJ, Brill IK, Omel J, Godby K, Kumar SK, Brown EE. Recent trends in multiple myeloma incidence and survival by age, race, and ethnicity in the United States. Blood Adv 2017;1:282-7.
4. Nishimura KK, Barlogie B, van Rhee F, et al. Long-term outcomes after autologous stem cell transplantation for multiple myeloma. Blood Adv 2020;4:422-31.
5. Martino M, Paviglianiti A, Gentile M, Martinelli G, Cerchione C. Allogenic stem cell transplantation in multiple myeloma: dead or alive and kicking? Panminerva Med 2020;62:234-43.
6. Greil C, Engelhardt M, Finke J, Wäsch R. Allogeneic stem cell transplantation in multiple myeloma. Cancers 2021;14:55.
7. Facon T, Kumar SK, Plesner T, et al. Daratumumab, lenalidomide, and dexamethasone versus lenalidomide and dexamethasone alone in newly diagnosed multiple myeloma (MAIA): overall survival results from a randomised, open-label, phase 3 trial. Lancet Oncol 2021;22:1582-96.
8. Durie BGM, Hoering A, Sexton R, et al. Longer term follow-up of the randomized phase III trial SWOG S0777: bortezomib, lenalidomide and dexamethasone vs. lenalidomide and dexamethasone in patients (Pts) with previously untreated multiple myeloma without an intent for immediate autologous stem cell transplant (ASCT). Blood Cancer J 2020;10:53.
9. Kumar SK, Jacobus SJ, Cohen AD, et al. Carfilzomib or bortezomib in combination with lenalidomide and dexamethasone for patients with newly diagnosed multiple myeloma without intention for immediate autologous stem-cell transplantation (ENDURANCE): a multicentre, open-label, phase 3, randomised, controlled trial. Lancet Oncol 2020;21:1317-30.
10. Richardson PG, Jacobus SJ, Weller EA, et al. Triplet therapy, transplantation, and maintenance until progression in myeloma. N Engl J Med 2022;387:132-47.
11. Attal M, Lauwers-Cances V, Hulin C, et al. Lenalidomide, bortezomib, and dexamethasone with transplantation for myeloma. N Engl J Med 2017;376:1311-20.
12. Gay F, Musto P, Rota-Scalabrini D, et al. Carfilzomib with cyclophosphamide and dexamethasone or lenalidomide and dexamethasone plus autologous transplantation or carfilzomib plus lenalidomide and dexamethasone, followed by maintenance with carfilzomib plus lenalidomide or lenalidomide alone for patients with newly diagnosed multiple myeloma (FORTE): a randomised, open-label, phase 2 trial. Lancet Oncol 2021;22:1705-20.
13. Sonneveld P, Dimopoulos MA, Boccadoro M, et al. Daratumumab, bortezomib, lenalidomide, and dexamethasone for multiple myeloma. N Engl J Med 2024;390:301-13.
14. Gay F, Roeloffzen W, Dimopoulos MA, et al. Results of the phase III randomized iskia trial: isatuximab-carfilzomib-lenalidomide-dexamethasone vs carfilzomib-lenalidomide-dexamethasone as pre-transplant induction and post-transplant consolidation in newly diagnosed multiple myeloma patients. Blood 2023;142:4.
15. Solimando AG, Malerba E, Leone P, et al. Drug resistance in multiple myeloma: Soldiers and weapons in the bone marrow niche. Front Oncol 2022;12:973836.
16. Gertz MA, Kumar S, Lacy MQ, et al. Stem cell transplantation in multiple myeloma: impact of response failure with thalidomide or lenalidomide induction. Blood 2010;115:2348-53.
17. Kapoor P, Kumar SK, Dispenzieri A, et al. Importance of achieving stringent complete response after autologous stem-cell transplantation in multiple myeloma. J Clin Oncol 2013;31:4529-35.
18. Moreau P, Attal M, Pégourié B, et al. Achievement of VGPR to induction therapy is an important prognostic factor for longer PFS in the IFM 2005-01 trial. Blood 2011;117:3041-4.
19. Farhan S, Lin H, Baladandayuthapani V, et al. Response before autologous hematopoietic stem cell transplantation is an important predictor of outcome in multiple myeloma. Blood 2011;118:4119.
20. Corso A, Caberlon S, Pagnucco G, et al. Blood stem cell collections in multiple myeloma: definition of a scoring system. Bone Marrow Transplant 2000;26:283-6.
21. Popat U, Saliba R, Thandi R, et al. Impairment of filgrastim-induced stem cell mobilization after prior lenalidomide in patients with multiple myeloma. Biol Blood Marrow Transplant 2009;15:718-23.
22. Bhutani D, Zonder J, Valent J, et al. Evaluating the effects of lenalidomide induction therapy on peripheral stem cells collection in patients undergoing autologous stem cell transplant for multiple myeloma. Support Care Cancer 2013;21:2437-42.
23. Chhabra S, Callander N, Watts NL, et al. Stem cell mobilization yields with daratumumab- and lenalidomide-containing quadruplet induction therapy in newly diagnosed multiple myeloma: findings from the MASTER and GRIFFIN trials. Transplant Cell Ther 2023;29:174.e1-10.
24. Oza S, Slotky R, Vissa P, et al. Effect of daratumumab on stem cell mobilization and engraftment kinetics post autologous stem cell transplantation in patients with newly diagnosed multiple myeloma. Blood 2022;140:10441-2.
25. Yoshihara S, Yoshihara K, Shimizu Y, et al. Feasibility of six cycles of lenalidomide-based triplet induction before stem cell collection for newly diagnosed transplant-eligible multiple myeloma. Hematology 2021;26:388-92.
26. Bal S, Landau HJ, Shah GL, et al. Stem cell mobilization and autograft minimal residual disease negativity with novel induction regimens in multiple myeloma. Biol Blood Marrow Transplant 2020;26:1394-401.
27. Wei X, Wei Y. Stem cell mobilization in multiple myeloma: challenges, strategies, and current developments. Ann Hematol 2023;102:995-1009.
28. Atilla P, Bakanay Ozturk SM, Demirer T. How to manage poor mobilizers for high dose chemotherapy and autologous stem cell transplantation? Transfus Apher Sci 2017;56:190-8.
29. Crees ZD, Stockerl-Goldstein K, Vainstein A, Chen H, DiPersio JF. GENESIS: phase III trial evaluating BL-8040 + G-CSF to mobilize hematopoietic cells for autologous transplant in myeloma. Future Oncol 2019;15:3555-63.
30. Charalampous C, Goel U, Gertz M, et al. Impact of the time interval between end of induction and autologous hematopoietic transplantation in newly diagnosed patients with multiple myeloma. Bone Marrow Transplant 2023;58:46-53.
31. Engelhardt M, Terpos E, Kleber M, et al. European myeloma network recommendations on the evaluation and treatment of newly diagnosed patients with multiple myeloma. Haematologica 2014;99:232-42.
32. Cavo M, Rajkumar SV, Palumbo A, et al. International myeloma working group consensus approach to the treatment of multiple myeloma patients who are candidates for autologous stem cell transplantation. Blood 2011;117:6063-73.
33. Duong HK, Savani BN, Copelan E, et al. Peripheral blood progenitor cell mobilization for autologous and allogeneic hematopoietic cell transplantation: guidelines from the American society for blood and marrow transplantation. Biol Blood Marrow Transplant 2014;20:1262-73.
34. DiPersio JF, Stadtmauer EA, Nademanee A, et al. Plerixafor and G-CSF versus placebo and G-CSF to mobilize hematopoietic stem cells for autologous stem cell transplantation in patients with multiple myeloma. Blood 2009;113:5720-6.
35. DiPersio JF, Micallef IN, Stiff PJ, et al. Phase III prospective randomized double-blind placebo-controlled trial of plerixafor plus granulocyte colony-stimulating factor compared with placebo plus granulocyte colony-stimulating factor for autologous stem-cell mobilization and transplantation for patients with non-Hodgkin's lymphoma. J Clin Oncol 2009;27:4767-73.
36. Crees ZD, Rettig MP, Jayasinghe RG, et al. Motixafortide and G-CSF to mobilize hematopoietic stem cells for autologous transplantation in multiple myeloma: a randomized phase 3 trial. Nat Med 2023;29:869-79.
37. FDA approves motixafortide plus filgrastim for stem cell mobilization in multiple myeloma. Available from: https://www.ajmc.com/view/fda-approves-motixafortide-plus-filgrastim-for-stem-cell-mobilization-in-multiple-myeloma [Last accessed on 21 May 2024].
38. Bekadja MA, Mansour B, Ouldjeriouat H, et al. First experience of the use of a generic of plerixafor in peripheral blood stem cell mobilization in multiple myeloma and lymphoma patients. Transfus Apher Sci 2021;60:103070.
39. Sevindik O, Bilgen H, Serin I, et al. Comparison of efficacy and safety of generic plerixafor vs original plerixafor in the mobilization of myeloma patients. Blood 2022;140:12803-4.
40. Micallef IN, Sinha S, Gastineau DA, et al. Cost-effectiveness analysis of a risk-adapted algorithm of plerixafor use for autologous peripheral blood stem cell mobilization. Biol Blood Marrow Transplant 2013;19:87-93.
41. Prakash VS, Malik PS, Sahoo RK, et al. Multiple myeloma: risk adapted use of plerixafor for stem cell mobilization prior to autologous stem cell transplantation is effective and cost efficient. Clin Lymphoma Myeloma Leuk 2022;22:44-51.
42. Park G, Shayani S, Stiller T, Wang S, Yuan S. Dose capping of plerixafor in patients weighing more than 100 kg at one vial led to successful mobilization outcomes and significant cost savings. Transfusion 2018;58:323-9.
43. Shah EE, Young RP, Wong SW, et al. Impact of plerixafor use at different peripheral blood CD34+ thresholds on autologous stem cell collection in patients with multiple myeloma. Biol Blood Marrow Transplant 2020;26:876-83.
44. Lamotte M, DiPersio JF, Siegel DS, Meron H, Serlin PA, Gerlier L. EE56 cost-effectiveness analysis of motixafortide on top of G-CSF for stem cell mobilization for autologous bone marrow transplantation in patients with multiple myeloma. Value Health 2022;25:S346.
45. Yassine F, Kharfan-Dabaja MA, Tsalantsanis A, et al. Trends in utilization of stored cryopreserved autologous peripheral hematopoietic cells intended for a second (or beyond) autologous hematopoietic cell transplantation in patients with multiple myeloma: a single center experience. Bone Marrow Transplant 2023;58:1130-6.
46. Afifi S, Adel NG, Devlin S, et al. Upfront plerixafor plus G-CSF versus cyclophosphamide plus G-CSF for stem cell mobilization in multiple myeloma: efficacy and cost analysis study. Bone Marrow Transplant 2016;51:546-52.
47. Norton J, Campo L, Hagen P, Tsai SB, Stiff PJ. In vivo potency of hematopoietic stem cells after long-term storage at -80C in DMSO+HES cryprotectant mixture: comparing engraftment kinetics and efficacy data in first and second autologous stem cell transplantation in myeloma. Blood 2023;142:6779.
48. Giralt S, Stadtmauer E, Harousseau J, et al. International myeloma working group (IMWG) consensus statement and guidelines regarding the current status of stem cell collection and high-dose therapy for multiple myeloma and the role of plerixafor (AMD 3100). Leukemia 2009;23:1904-12.
49. Wuchter P. Processing, cryopreserving and controlling the quality of HSCs. In: Carreras E, Dufour C, Mohty M, Kröger N, editors. The EBMT handbook. Cham: Springer International Publishing; 2019. pp. 127-30.
50. Aladağ Karakulak E, Demiroğlu H, Büyükaşik Y, et al. CD34+ hematopoietic progenitor cell dose as a predictor of engraftment and survival in multiple myeloma patients undergoing autologous stem cell transplantation. Turk J Med Sci 2020;50:1851-6.
51. Weaver CH, Hazelton B, Birch R, et al. An analysis of engraftment kinetics as a function of the CD34 content of peripheral blood progenitor cell collections in 692 patients after the administration of myeloablative chemotherapy. Blood 1995;86:3961-9.
52. Desikan KR, Tricot G, Munshi NC, et al. Preceding chemotherapy, tumour load and age influence engraftment in multiple myeloma patients mobilized with granulocyte colony-stimulating factor alone. Br J Haematol 2001;112:242-7.
53. Schulman KA, Birch R, Zhen B, Pania N, Weaver CH. Effect of CD34+ cell dose on resource utilization in patients after high-dose chemotherapy with peripheral-blood stem-cell support. J Clin Oncol 1999;17:1227.
54. Cook G, Ashcroft AJ, Cairns DA, et al. The effect of salvage autologous stem-cell transplantation on overall survival in patients with relapsed multiple myeloma (final results from BSBMT/UKMF Myeloma X Relapse [Intensive]): a randomised, open-label, phase 3 trial. Lancet Haematol 2016;3:e340-51.
55. Cook G, Williams C, Brown JM, et al. High-dose chemotherapy plus autologous stem-cell transplantation as consolidation therapy in patients with relapsed multiple myeloma after previous autologous stem-cell transplantation (NCRI Myeloma X Relapse [Intensive trial]): a randomised, open-label, phase 3 trial. Lancet Oncol 2014;15:874-85.
56. Gregersen H, Peceliunas V, Remes K, et al. Carfilzomib and dexamethasone maintenance following salvage ASCT in multiple myeloma: a randomised phase 2 trial by the Nordic Myeloma Study Group. Eur J Haematol 2022;108:34-44.
57. Hagen PA, Stiff P. The role of salvage second autologous hematopoietic cell transplantation in relapsed multiple myeloma. Biol Blood Marrow Transplant 2019;25:e98-107.
58. Michaelis LC, Saad A, Zhong X, et al. Salvage second hematopoietic cell transplantation in myeloma. Biol Blood Marrow Transplant 2013;19:760-6.
59. Bhutani M. Boosting CAR T cytopenias with backup autologous stem cells. Transplant Cell Ther 2023;29:535-6.
60. Rejeski K, Perez A, Iacoboni G, et al. Severe hematotoxicity after CD19 CAR-T therapy is associated with suppressive immune dysregulation and limited CAR-T expansion. Sci Adv 2023;9:eadg3919.
61. Davis JA, Sborov DW, Wesson W, et al. Efficacy and safety of CD34+ stem cell boost for delayed hematopoietic recovery after BCMA directed CAR T-cell therapy. Transplant Cell Ther 2023;29:567-71.
62. Mullanfiroze K, Lazareva A, Chu J, et al. CD34+-selected stem cell boost can safely improve cytopenias following CAR T-cell therapy. Blood Adv 2022;6:4715-8.
63. Künkele A, Brown C, Beebe A, et al. Manufacture of chimeric antigen receptor T cells from mobilized cyropreserved peripheral blood stem cell units depends on monocyte depletion. Biol Blood Marrow Transplant 2019;25:223-32.
64. Ahmed N, Li L, Rojas P, et al. Significant costs and low utilization of stored peripheral blood stem cells for salvage autologous transplant in multiple myeloma patients including those meeting mSMART criteria. Bone Marrow Transplant 2021;56:1458-61.
65. Chhabra S, Thapa B, Szabo A, et al. Utilization and cost implications of hematopoietic progenitor cells stored for a future salvage autologous transplantation or stem cell boost in myeloma patients. Biol Blood Marrow Transplant 2020;26:2011-7.
Cite This Article
Export citation file: BibTeX | EndNote | RIS
OAE Style
Fitzsimons J, Hagen PA. Optimal approach to stem cell mobilization in transplant-eligible multiple myeloma patients. J Cancer Metastasis Treat 2024;10:18. http://dx.doi.org/10.20517/2394-4722.2024.16
AMA Style
Fitzsimons J, Hagen PA. Optimal approach to stem cell mobilization in transplant-eligible multiple myeloma patients. Journal of Cancer Metastasis and Treatment. 2024; 10: 18. http://dx.doi.org/10.20517/2394-4722.2024.16
Chicago/Turabian Style
Jack Fitzsimons, Patrick A. Hagen. 2024. "Optimal approach to stem cell mobilization in transplant-eligible multiple myeloma patients" Journal of Cancer Metastasis and Treatment. 10: 18. http://dx.doi.org/10.20517/2394-4722.2024.16
ACS Style
Fitzsimons, J.; Hagen PA. Optimal approach to stem cell mobilization in transplant-eligible multiple myeloma patients. J. Cancer. Metastasis. Treat. 2024, 10, 18. http://dx.doi.org/10.20517/2394-4722.2024.16
About This Article
Copyright
Data & Comments
Data
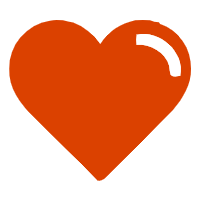
Comments
Comments must be written in English. Spam, offensive content, impersonation, and private information will not be permitted. If any comment is reported and identified as inappropriate content by OAE staff, the comment will be removed without notice. If you have any queries or need any help, please contact us at support@oaepublish.com.