The role of PD-1/PD-L1 signaling pathway in cancer pathogenesis and treatment: a systematic review
Abstract
Aim: Cancer as a complex disease poses significant challenges for both diagnosis and treatment. Researchers have been exploring various avenues to find effective therapeutic strategies, with a particular emphasis on cellular signaling pathways and immunotherapy. One such pathway that has recently been suggested is the PD-1/PD-L1 pathway, which is an immune checkpoint signaling system that plays an important role in regulating the immune system and maintaining tissue homeostasis. Cancer cells exploit this pathway by producing PD-L1, which attaches to PD-1 on T cells, thus inhibiting immune responses and enabling the cancer cells to escape detection by the immune system. This study aimed to evaluate the role of the PD-1/PD-L1 pathway in cancer pathogenesis and treatment. Method: This study was performed based on the principles of Preferred Reporting Items for Systematic Reviews and Meta-Analysis (PRISMA). All in vitro, in vivo, and clinical studies that were published in English have been considered during a thorough search of the Scopus, Web of Science, and PubMed databases without date restriction until March 2024. Results: According to the studies reviewed, the PD-1/PD-L1 signaling axis suggests promising therapeutic effects on various types of cancers such as non-small cell lung cancer, melanoma, breast cancer, hepatocellular carcinoma, squamous cell carcinoma, and colorectal cancer, among others. Additionally, research suggests that immune checkpoint inhibitors that block PD1/PD-L1, such as pembrolizumab, atezolizumab, nivolumab, durvalumab, cemiplimab, avelumab, etc., can effectively prevent tumor cells from escaping the immune system. Moreover, there might be a possible interaction between microbiome, obesity, etc. on immune mechanisms and on the immune checkpoint inhibitors (ICIs). Conclusion: Although we have gained considerable knowledge about ICIs, we are still facing challenges in effectively prescribing the appropriate ICIs for individual patients. This is largely due to the complex interactions between different intracellular pathways, which need to be thoroughly studied. To resolve this issue, it is necessary to conduct more reliable clinical trials that can produce a scientific consensus.
Keywords
INTRODUCTION
Cancer, one of the leading health issues worldwide, continues to pose significant challenges in terms of its treatment and management. Over the years, the search for effective therapeutic strategies has led researchers to explore the intricate world of cellular signaling pathways and immunotherapy[1-4]. However, the idea of using cellular signaling pathways to treat cancer was called the “Achilles heel of cancer” back in 2008. The characteristics of these signaling pathways and their considerable overlaps in different cancers leave no doubts about their therapeutic benefits[5]. Novel therapies such as cancer vaccine therapies are beneficial considering relative side effects, malignant cell cytotoxicity, and overall survival (OS)[6]. On the other hand, the PD-1/PD-L1 (programmed death Receptor/Ligand) pathway has been taken into investigation, offering novel possibilities for cancer treatment[7-9].
The PD-1/PD-L1 pathway, an immune checkpoint signaling system, plays a crucial role in regulating immune responses and maintaining tissue homeostasis[10]. Cancer cells capitalize on this defensive mechanism by producing PD-L1, which attaches to PD-1 on T cells (specifically T cells), effectively suppressing immune reactions and evading the immune system's surveillance[11]. Thus, the usage of the
The potential of PD-1/PD-L1 inhibitors to revolutionize cancer treatment approaches is a turning point in the field of oncology. However, it is crucial to continue exploring and expanding our knowledge of this pathway and its undiscovered intricacies. This comprehensive review aims to shed light on the role of the PD-1/PD-L1 pathway in cancer pathogenesis and treatment. By evaluating its impact on immune evasion, tumor progression, and therapeutic targeting, complex signaling systems can uncover new approaches for precision medicine. Moreover, we seek to bridge existing knowledge gaps by analyzing current immunotherapeutic approaches and identifying areas where further research is warranted. By combining a deep understanding of the intricate PD-1/PD-L1 pathway with the vast potential of immunotherapy, we hope that this will lead to personalized, effective, and targeted cancer treatments.
METHOD
This systematic review was performed to investigate any pathological and pharmacological relationship between the PD-1/PD-L1 pathway and cancer, based on the preferred reporting items for systematic review and meta-analysis (PRISMA) checklist as a guideline of the review process (Supplementary Material - PRISMA Checklist). Details are presented in Figure 1.
Information source and search strategy
All English publications investigating the pathological and pharmacological relationship between
First: (“Pd-L1 Pathway” OR “PDCD1” OR “CD274” OR “programmed cell death 1 ligand 1” OR “programmed death ligand 1”) AND (“Breast Cancer” OR “Hepatocellular” OR “Melanoma” OR “Non-small Cell Lung Cancer” OR “Squamous Cell Carcinoma” OR “Colon Cancer”).
Second: (“Pd-l1 Pathway” OR “PDCD1” OR “CD274” OR “programmed cell death 1” OR “programmed death 1” OR “programmed cell death 1 ligand 1” OR “programmed death ligand 1” OR “PDCD1 protein, human” OR “Programmed Cell Death 1 Receptor” OR “Immune Checkpoint Inhibitors”) AND (“Smoking” OR “Alcohols” OR “Obesity” OR “Air Pollution” OR “Life Style” OR “Diet” OR “Microbiota” OR “Nutritional Status”) AND (“drug effects” OR “Drug-Related Side Effects and Adverse Reactions” OR “Immunotherapy” OR “pembrolizumab” OR “Nivolumab” OR “durvalumab” OR “cemiplimab” OR “avelumab”).
To prevent any missing articles and select all appropriate publications, Google Scholar, related review articles, the reference list of all included articles, and conference articles were hand-searched. Finally, a total of 237 articles were included and categorized into the following seven main headings: PD-L1 Pathway, Tumorigenesis, Non-small Cell Lung Cancer, Melanoma, Breast Cancer, Hepatocellular cancer, Squamous Cell Carcinoma, Colorectal Cancer, and Other Cancers. Endnote X9 was used to remove duplications.
Eligibility criteria
Original articles with these criteria were included: (a) published in English; (b) evaluating the mechanism of PD-1/PD-L1 in cancers.
Exclusion criteria were: (a) studies with a lack of information including unconvertible or non-extractable data; (b) not being original articles or reviews (news, letters, editorials, conference abstracts, unpublished manuscripts, and book chapters); (c) low-quality publication; and (d) epidemiological and ecological studies.
Data extraction
All duplications were removed, and the remaining articles were reviewed by two authors independently. The screening process was performed in a three-phase process: firstly, the title/abstract of all publications was reviewed, and then, the full text of all studies was evaluated. Finally, we emailed the corresponding authors 3 times to get the full-text PDF of the articles that we could not find their full texts. The following data were extracted based on a pre-defined list by two independent authors: last name of the first author, publication year, the cellular mechanism of PD-1/PD-L1 in cancer, and any medication used in cancer treatment related to PD-1/PD-L1 pathway. The retrieved studies were reviewed by the two primary authors (A.S and S.S) and any disagreements were resolved by the final author (S.M).
RESULTS
PD-1/PD-L1 pathway
Immune checkpoints (ICs) are key factors for many cell functions and immunity. As a result, they play an important role in many processes such as self-tolerance, reducing peripheral tissue damage, and preventing autoimmunity[14-16]. Unfortunately, troubles can be brought up by misusing these checkpoints, as happens in cancer. It can be vividly observed that analyzing these checkpoints’ maps and pathways can be of great importance in controlling cancer[17,18], considering the increasing predicted deaths due to cancer in the future[19]. Over almost 20 years, the discovery of a new molecule proclaimed hopeful prospects. In 1992,
Function and structure of PD-1/PD-L1 pathway
PD-1 is composed of 288 amino acids encoded by Pdcd1, and is present on the surface of T cells, B cells, and NK cells. It is a transmembrane protein and composed of three parts: an IgV domain serves as the extracellular part, a transmembrane region, and an intracellular part[20,27-29]. PD-L1 and PD-L2 are two transmembrane glycoproteins with IgV and IgC domains consisting of 40% amino acid identity[30,31].
Ligation of PD-L1 and its receptor on T cells inhibits their proliferation and induces their apoptosis. First, it causes CD4+ T cells to transform into foxp3+ cells. Second, it prevents T cells from going into the S phase of the cell cycle[25]. Together, the mentioned activities suppress the immune response. Expectedly, knocking out PD-1 in mice causes autoimmune-like symptoms as what happens in lupus-like arthritis and glomerulonephritis; consequently, the importance of the PD-1 pathway in immune suppression and self-tolerance is magnified[32,33]. The complex correlation between different pathways linked to PD-L1 regulation is shown in Figure 3[24-26].
Tumorigenesis
Since the incidence of cancer in immune-compromised individuals is higher, one can readily see that appropriate immune responses are of great importance in cancer prevention. Regarding the great impact of the PD-1/PD-L1 pathway as an IC and its role in mitigating immune responses mediated by T cells, it is not far from expectation that the occurrence of cancer can be promoted by the activation of this pathway[34,35].
Upregulation of PD-L1 is found to be effective in the occurrence of most cancers. However, this upregulation and its mechanism are not well understood yet, but it is assumed that they are related to pro-survival factors in cancer cells. Knowing that PD-L1 can be expressed in almost any nucleated cell of the body, and also due to expression via the presence of IFN-γ in the aforementioned cells, it should be reminded that the more this pathway is observed and understood, the more progress in cancer therapy can be made[36-43].
The activation of T cells needs two responses from APCs. First, an interaction between antigenic major histocompatibility complex (MHC) and T cell receptor (TCR) conveys specificity from antigen recognition. Second, an antigen-independent signal that is mediated via interaction between molecules on the surface of T cells and APCs is responsible for the activation of T cells. Without this mechanism, immune responses will go in vain, and energy will be produced. Therefore, we can call the second mechanism co-stimulation[44,45]. Now, it is observable that the upregulation of PD-L1 in tumor cells is not unexpected. This upregulation is assumed to be related to oncogenic pathways; meanwhile, ongoing studies are indicating it[46-49].
NON-SMALL CELL LUNG CANCER
Despite the complicated effects of the PD-1/PD-L1 axis in tumorigenesis, many studies have observed various connections between cancer and this axis[50]. The first cancer to be analyzed in this paper is non-small cell lung cancer (NSCLC), which is known as the first cause of death globally[51]. Studying PD-L1 expression in NSCLC has two benefits. First, high expression of PD-L1 can be a prognostic factor for the development of cancer into more advanced stages, despite different findings. Additionally, silencing PD-L1 reduces the development of advanced stages and increases the survival rate[50,52,53]. Furthermore, patients with more tumor-infiltrating lymphocytes (TILs) have a higher chance of survival. Considering the role of PD-L1 in decreasing the number of TILs, it is very important to know about its characteristics to the fullest[54,55]. Moreover, PD-L1 expression is significantly affected by the activation of other cellular pathways such as EGFR. It has been proven that EGFR activation induces PD-L1 expression, preventing the encounter of immunosuppressive agents with the host’s antitumor immune response. As a result, the usage of EGFR tyrosine kinase inhibitors together with PD1 blockers can predominantly decrease the risk of resistance and lengthen the treatment duration [Figure 4][56].
Figure 4. The EGF binds with its receptor and, through a cascade of reactions via activation of 3 pathways, consequently induces transcription of the PD-L1 gene; (1) c-Cbl activates NCK and then activates JNKs, and finally, transcription of PD-L1 gene is induced via activation of c-Jun. (2) JAK2/STAT1 pathway induces transcription of PD-L1 gene. (3) Ras-BRAF-MEK-ERK induces PDL1 transcription.
Immunotherapy has been suggested as a potential treatment, considering the essence of the PD-1/PD-L1 axis. Immune checkpoint inhibitors (ICIs) have been used in the treatment of NSCLC for many years, and this has continued to be the case because of their good safety, low toxicity, and well-tolerated nature. The first drug is nivolumab, a U.S. Food and Drug Administration (FDA)-approved monoclonal antibody against PD-1, which was used as the second-line therapy for patients with advanced squamous NSCLC in 2015[57]. Pembrolizumab is another well-known member of anti-PD-1 antibodies, which was approved by the FDA in 2015 as a second-line treatment, the same as nivolumab[58]. However, the use of pembrolizumab was further expanded. In the case of metastatic tumors with greater than 50% PD-L1 expression, pembrolizumab can be used as the first-line treatment, while nivolumab is the drug of choice for second-line treatment, regardless of PD-L1 expression[59]. It is noteworthy that higher PD-L1 expression is generally linked to better results of ICI therapy[60], yet burdens always lie ahead. Low RR and survival of patients going through ICI therapy, specifically those with metastatic and advanced NSCLC, created new urges for medicine[61]. Although RR in many clinical trials revolves around 20% to 30%[62], it may be surprising to mention that the underlying reason for this low RR is not necessarily the weakness of these drugs. There are so many complications in the interaction of the PD-1/PD-L1 axis with the internal cellular mechanisms, many of which are still unknown. Therefore, it can be concluded that the reason for this low RR may be the physician’s inability to identify the right cases for therapy with ICIs[58,63].
One solution for this is to look at this axis from a new point of view and target PD-L1 with monoclonal antibodies (mAbs). Atezolizumab, durvalumab, and avelumab are some examples; fortunately, they have shown promising results up to now. On the other hand, it is proven through studies that analysis of the proliferation of CD8+ cells and expression of PD-L1+ mRNA/protein, together as a signature, tends to be a better prediction tool for evaluating the patient’s response to durvalumab, in comparison with manual PD-L1 IHC scoring[54]. Combinational therapy is the next solution. Chemotherapy, the very basic and well-known treatment strategy for cancer, has shown improved results in combination with pembrolizumab[60]. A study conducted recently reported meaningful improvement in objective RR and progression-free survival (PFS) after using tiragolumab in combination with atezolizumab compared to atezolizumab alone[64]. Some studies have tried to target other surface molecules besides PD-1. One successful example of this is the positive results received from combining ramucirumab, a VEGFR2 inhibitor, with pembrolizumab[65]. ALT-803 is a pharmacological grade IL-15/IL-15Rα complex fused to an IgG1 Fc that can be combined with nivolumab to obtain better results[66]. Platycodon grandiflorum (PG), a Chinese traditional homology, possesses immunomodulatory effects on T cells via inhibiting PD-1 expression, leading to NSCLC suppression. A combination of platycodin D and platycodin D3, molecules of the same family as PG, has also shown antitumor effects[67]. It is reported that high-dose ionizing irradiation has been effective in PD-L1 expression upregulation, and therefore, blockade of this pathway improves the effectiveness of radiotherapy through mechanisms that are mainly carried out by cytotoxic T cells. Consequently, anti-PD1 and anti-CTLA4 (Cytotoxic T lymphocyte antigen-4) therapy, together with radiotherapy, tends to cause an elevation in tumor-specific T cells[68]. In the case of combinational therapy, a recent study has indicated that NSCLC patients receiving a daily dose of Aspirin in combination with PD-L1 inhibitors (Durvalumab or Atezolizumab) showed more likelihood of regaining complete remission and indicated less chance of PD complications[69]. Finally, considering the use of new therapies, it should be mentioned that up to now, the use of small molecules and tumor vaccines has proven to be possible and valuable therapies for cancer, and NSCLC is not an exception. However, their usage requires to be further investigated[67].
Analyzing the PD-L1 axis in NSCLC has indicated that high expression of this ligand can be a prognostic factor in evaluating the development of cancer in advanced stages and its grading. Additionally, it is proven that silencing PD-L1 reduces the development of advanced stages and increases the survival rate. Furthermore, patients with more tumor-infiltrating lymphocytes (TILs) have a higher chance of survival. Considering the role of PD-L1 in decreasing the number of TILs, immune checkpoint inhibitors (ICI) functioning as anti-PD-1 antibodies such as Nivolumab and Pembrolizumab have been suggested as drugs of choice for the treatment of NSCLC due to safety, low toxicity, and also well-tolerated nature. On the other hand, the low RR and survival rate of patients undergoing ICI therapy have led us to learn more about the complications and interaction of the PD-1/PD-L1 axis with the internal cellular pathways. As a result, a solution is to target PD-L1 with monoclonal antibodies such as Atezolizumab, Durvalumab, and avelumab. In addition, it would be more effective if these mAbs were combined with chemotherapy, mirroring what has been observed in the combination of tiragolumab with atezolizumab, resulting in increased effectiveness compared to atezolizumab alone. On the other hand, some studies have targeted other surface molecules in addition to PD-1, for instance; ramucirumab, which is a VEGFR2 inhibitor, has presented positive results in combination with pembrolizumab. Other examples are Platycodon grandiflorum, a Chinese traditional homology, and ALT-803, a pharmacological complex fused to an IgG1 Fc. Finally, it should be mentioned that the usage of vaccines and other small molecules for the treatment of NSCLC should be further investigated.
MELANOMA
The complexity of regulation persists, and many signaling pathways are involved in the regulation of PD-L1 expression. BRAF mutations are present in 66% of melanomas and are the most abundant mutations in it. Their occurrence is so high that some types of melanomas are categorized based on the type of BRAF mutation causing them. Other signaling pathways like MAPK and PI3K can cause upregulation of PD-L1 through the activation of different molecular mechanisms as well. One of the most common ways for the oncogenic activation of the PI3K pathway, especially in malignant melanoma patients, is the loss of PTEN protein[70]. It has also been proved that PTEN loss is usually followed by BRAF mutations, so MAPK and AKT pathways are activated in parallel and lead to melanoma[71,72]. Based on these correlations, combination therapies may yield better results than mono-target therapies [Figure 5]. Making this complexity more complex, the presence of TILs in the tumor microenvironment (TME) appears to be a good sign, but in a study conducted in 2012, the production of inflammatory cytokines such as IFN-Y by TILs could enhance the expression of PD-L1, thus having counter effects on immune response[39,73]. NK cells from innate immunity have initial responses against tumors. Tumors can suppress NK cells by creating an immune suppressive microenvironment, upregulating ligands for inhibitory receptors, recruiting immunosuppressive cells such as Treg cells, and secreting IDO, PGE2, and anti-inflammatory cytokines[74,75]. Tumor-associated fibroblasts play a pivotal role in the inhibition of NK cells. PGE2 produced by these cells prevents IL-2-driven upregulation of triggering receptors, thus NK cell inhibition[76]. It was shown by Alavi et al. that interferon signaling was also inhibited in cases of melanoma[77]. Regulating mechanisms affecting PD-L1 resemble an iceberg and we need to dig deeper to take the upper hand against cancer[78].
Figure 5. Basic oncogenic pathways in melanoma. BRAF mutations are mostly common and, in the end, lead to the expression of PD-L1. The PI3K pathway is usually activated simultaneously and is inhibited by PTEN.
Back in 2011, the first drugs affecting the PD-1/PD-L1 axis received FDA approval. Before this time, most drugs used for different types of melanomas focused on molecules produced by immune cells, such as interleukin-2 and IFN-Y. Nivolumab, vemurafenib, ipilimumab, and pembrolizumab were among the first FDA-approved drugs to inhibit ICs, therefore earning the classification as ICIs. These drugs are currently the first line of treatment for both types of melanomas, melanoma of unknown primary (MUP) and melanoma of known primary (MKP). MUP patients exhibit comparable survival rates to MKP stage IV patients. This is attributed to the shared immunological mechanisms that contribute to the regression of the primary tumor, ultimately leading to a more favorable prognosis for MUP patients compared to MKP patients.[79] Nivolumab and pembrolizumab have 30%-33% RRs, while Vemurafenib shows a 48% RR[80-82]. Approximately low responses point out the importance of finding other therapeutic ways or improving the previous strategies in some way.
However, the whole medication is not limited to ICIs. In 2020, S-adenosylmethionine (SAM), a methylating agent, was proven to not only show anti-melanoma effects but also boost the action of anti-PD-1 drugs[80]. Cytokines are still being investigated for optimal usage[83]. Next, some researchers have gone beyond PD-1 molecules and focused on other agents with the ability to affect other molecules of cancer cells. CD155 is a ligand on the surface of melanoma cells that suppresses T cells. The combination of CD155 and PD-1 blockade is more effective in improving the ability of the immune system to take part[84]. PCC0208025 is a molecule that prevents the binding between PD-1 and PD-L1 and bears its antitumor role in this way[85]. PD-L1 should not be forgotten among all the attention given to PD-1. Alongside antibodies, other molecules can also affect PD-L1. Aptamers, which are single-stranded nucleic acids with the ability to bind to proteins and cells, can bind to PD-L1 and stop tumor growth[86]. Some other chemicals such as celastrol (CEL) are also claimed to be effective. CEL can do two important things: First, it causes cell death; second, it will downregulate PD-L1, which is helpful[87]. Nanomedicine can also be used for transferring drugs such as docetaxel with two primary benefits: First, lesser systemic toxicity, and second, more accumulation of the drug in the tumor site[88].
Yet, the capacity of PD-L1 for treatment is way more than what was discussed earlier. A combination of PD-L1 immunotherapy with other drugs can bring in better results. For instance, a combination of GK-1, a peptide derived from helminth Taenia Crassiceps, with an anti-PD-L1 therapy can increase survival time and enhance T cell function in mice models[89,90]. PV-10, a 10% solution of rose bengal disodium, is another chemical that has synergistic effects when used with an anti-PD-L1 antibody. Therapy with these two drugs can delay tumor growth in mice models[91]. Transferring the drugs to the site of the tumor is also another topic of debate. Different ways of transferring enable the usage of specific drugs or access to new points of lesion or tumor for treatment. Gels loaded with anti-PD-L1 antibodies and may be some contributing medicine can be a revolutionary idea. They can be injected or physically applied to the site of the tumor and bear preventative advantages parallel to therapeutic ones[92,93].
Due to a better understanding of intracellular pathways, there is a greater temptation every day that boosting or blocking some pathways may result in control of the PD-1/PD-L1 axis. This article focuses on PD-L1 rather than PD-1 because it is expressed and regulated by the tumor cells. As previously mentioned, BRAF mutations are important oncogenes in melanoma. Vemurafenib and dabrafenib, two BRAF inhibitors, have shown great efficacy in clinical studies. Dabrafenib is a drug with the unique ability to inhibit only mutant BRAF. MAPK and PI3K pathways are two other important signaling pathways that control the expression of PD-L1 and are being studied intensively[94]. A recent study has drawn attention to a novel regulating molecule, c-jun. c-jun is a proto-oncogene protein that contributes to the formation of AP-1, a transcription factor. It is notable to say that c-jun was the first oncogenic transcription factor discovered[95], but remained in shadows. Activation of c-jun can lead to PD-L1 expression and its consequences. Alianthone, a traditional Chinese medicine, is found to be effective in ceasing c-jun-dependent PD-L1 expression[96]. New technologies, as a big trend in medicine, have led some studies to follow the hypothesis of combining cancer vaccines and anti-PD-1 antibodies and have improved results[97-99].
While PD-L1 expression level is thought to be a good predictive marker for RR or treatment[100], Taube et al. have introduced the idea that although PD-L1 expression can be a good predictive marker, it can be a sign of immunogenic tumor and probably more aggressive ones[39,101]. Only one side effect of therapy using anit-PD-1/PD-L1 antibodies has been mentioned so far, but it is righteous to hint at numerous clinical studies that work on the side effects of these drugs and have reported different cases, from colitis to myocarditis[102].
Melanoma, as the deadliest cutaneous cancer, has a high profile of PD-L1 expression. One primary reason is the overactivity of other signaling pathways such as BRAF, PI3K, and MAPK. PD-L1 blockade has long been considered the first-line treatment. However, not very gratifying results of these drugs are forcing pharmaceutics to find alternatives. These alternatives can be immunomodulators, drugs to use alongside ICIs, chemicals affecting PD-1, or nanoparticles to carry compounds to the wanted site. Many of these innovatory modalities are still being probed in clinical trials, but up to now, they have been shown to be righteous substitutes or companions for ICIs.
Investigating several articles, we highlighted the complexity of regulating PD-L1 expression in melanoma, regarding the involvement of various signaling pathways and the impact of TILs in the tumor microenvironment. Drugs targeting the PD-1/PD-L1 axis, such as Nivolumab, vemurafenib, ipilimumab, and pembrolizumab, are used as first-line treatments for some types of melanoma. Additionally, other therapeutic approaches, including the use of S-adenosylmethionine (SAM), CD155 blockade, and molecules like PCC0208025 and celastrol, are mentioned. Furthermore, the potential of combining PD-L1 immunotherapy with other drugs for better outcomes is also discussed, along with the use of nanomedicine for drug delivery to tumor sites.
BREAST CANCER
Breast cancer is the most common cancer in women worldwide. It is also one of the most burdensome cancers, especially in developing countries[103]. More invasion and higher resistance are observed in tumors with a more active PD-L1 profile[104]. Further, the expression of PD-L1 is an advocate for creating an inhibitory TME[105]. However, there are also unexpected roles for PD-L1. For instance, higher PD-L1 expression can result in blockage of nociceptor neurons, therefore reducing the pain in BC patients[106]. Besides the comfort it brings for the patient, one can look at this feature as an obstacle in the treatment process mainly because most people seek care after the onset of symptoms.
Talking about signaling, the PTEN pathway has an inhibitory effect on PD-L1 expression, but its role in breast cancer is emphasized since 30% to 40% of primary breast tumors are accompanied by PTEN mutations[107]. Other pathways are also involved in breast cancer tumor progression that are described in Figure 6[26,108,109].
Figure 6. Signaling pathway in breast cancer. TGFβ, VEGF, and EGF receptors are involved in tumor progression of breast cancer through PI3K/AKT, Raf/ERK, and SMAD pathways.
Once again, ICIs are a common option for treatment. The well-known drug pembrolizumab has a 20% response rate, yet it is not that much. Combinational therapy can be a possible solution. Administering pembrolizumab and a PARP inhibitor (niraparib) is more effective than monotherapy with each[110]. ICIs against PD-L1 are not left intact either. Atelizumab, the first anti-PD-L1 drug approved by the FDA, has a 19% RR in BC[111]. Its combination with nab-paclitaxel is attested by the European Medicines Agency (EMA) to be effective in cases of BC with more than 1% of PD-L1 expression[112]. Avelumab is another anti-PD-L1 drug with two advantages. First, it can be useful in metastatic cases of BC, and second, it can boost immune cell activity[107].
Similar to the use of nanoparticles in melanoma treatment, the treatment of BC with nanoparticles is gaining scientific interest. These particles are instructed to inhibit PD-L1 or decrease its expression[113,114]. In 2019, a study introduced a nanomolecular formulation with 90% inhibition[115]. Nanoparticles can also be used as drug delivery systems with promising results[116]. Looking at the RRs of current drugs against ICs, this rate of inhibition is a miracle. Vaccines are another agent of modern treatment that show inhibitory effects on tumors[117], and a study observed more efficacy when anti-PD-L1 antibodies were combined with dendritic cell (DC) vaccines[118]. Several dispersed studies are working on different treatment options. These studies suggest several other choices such as olaparib with Oligo-Fucoidan[119], STM108, a conjugate antibody against PD-L1[120], Triptolide, a diterpenoid epoxide[121], and miRNAs[122] as treatment strategies for breast cancer.
Among clinical trials revolving around different types of BC, pembrolizumab has been under attention[123]. A trial conducted in 2019 scrutinized the use of niraparib in combination with pembrolizumab, a treatment strategy that was previously suggested by another study and mentioned in this paper[110]. Although this trial only included 5 patients with BC, it reported this combination to be tolerable in all patients of the study, whether with BC or ovarian cancer[124]. A global clinical trial in 2022 reported constructive results for a combination of PD-L1 inhibitors with nab-paclitaxel, a nanoparticle albumin-bound form of paclitaxel[125].
PI3K mutations and PD-L1 expression together in patients with metastatic breast cancer have shown poor prognosis associated with low overall survival (OS) and relapse-free survival (RFS)[126]. On the other hand, a study done in 2017 showed a positive correlation between PD-L1 expression, OS, and disease-free survival (DFS) rate in TNBC patients. It is also mentioned that there is a strong association between PD-L1 and the prevalence of TILs, which is an important prognostic factor for these patients. Overall, the connection between PD-L1 and OS is not demonstrated[127,128]. There is a strong correlation between PD-L1 and MDR1 protein which results in chemotherapy resistance, especially in the TNBC subtype[125]. Inhibiting BET proteins such as BRD 2, BRD3, and BRD4, which fundamentally regulate PD-L1 expression in TNBC, can be profitable[129]. The intrinsic effect of PD-L1, independently of its binding to PD-1, is proved in TNBC cell proliferation, colony formation, migration, and invasion in chick embryo chorioallantoic membrane (CAM) model in vivo[130].
We discussed the significance of PD-L1 expression in breast cancer, highlighting its role in creating an inhibitory tumor microenvironment but also noting unexpected benefits such as pain reduction. The impact of other signaling pathways like PTEN on PD-L1 expression and the prevalence of PTEN mutations in tumors are also under attention. Possible and common therapies with great outcomes include using immune checkpoint inhibitors (ICIs) like pembrolizumab and atelizumab, as well as combination therapies with drugs like niraparib. Additionally, the potential of nanoparticles in inhibiting PD-L1 expression and serving as drug delivery systems is significant. The efficacy of vaccines and other treatment options such as olaparib, STM108, Triptolide, and miRNAs in breast cancer therapy should be mentioned. Clinical trials involving pembrolizumab, niraparib, and PD-L1 inhibitors with nab-paclitaxel have shown promising results in the treatment of breast cancer.
HEPATOCELLULAR CANCER
The footprint of PD-L1 can be traced to hepatocellular cancer (HCC), a cancer with an estimated number of more than 1 million incidences by 2025 globally[131]. Logically, there are pathways to regulate PD-L1 expression. IFN-Y, IFN-c, and osteopontin can upregulate PD-L1 expression and cause immune invasion of tumor cells[132-137]. CD8-positive T cells that are recruited from the tumor microenvironment play an important role in inducing a partial tumoricidal immune response. These cells also promote upregulation of PD-L1 by secreting IFN-Y[138]. On the contrary, some molecules like WSX1, an IL-27 receptor, can downregulate PD-L1 expression and be of help in HCC treatment[139]. The role of DHA combined with EPA treatment on renal IR injury has been previously evaluated and showed that pre-ischemic exposure to a combination of DHA and EPA inhibits IR-induced oxidative stress and apoptosis[140]. This combination has shown many other benefits such as hippocampal neuron survival after ischemia[141,142].
Internal factors are not the only reason for the development of HCC viral agents, especially hepatitis; the virus can cause HCC with complications. However, the expression of PD-L1 and characteristics of the TME are not different in virus or non-virus-associated types. Once more, ICIs are a strategy that shows a 14% to 20% RR and have the same effects on different types of HCC, since different types of HCC have almost no difference in PD-L1 expression and TME. However, pembrolizumab and nivolumab exhibit positive results in patients who primarily received VEGF-targeted therapy in virus-associated HCC[143] and can be used as a treatment for patients with advanced HCC[144]. Similar to BC, the use of PARP inhibitors in combination with anti-PD-1 drugs can be more effective than monotherapy with each. Olaparib is a PARP inhibitor that is confirmed to be effective in ovarian cancer and its use in other cancer forms is being investigated[145], but it shows positive results in HCC when used in combination with anti-PD-1 drugs[143]. Dihydroartemisinin (DHA), an antimalarial medicine, enhances the effect of anti-PD-1 therapy by inhibiting YAP1 and reducing lipid droplets (LD)[146]. A new therapeutic approach for HCC patients with upregulated Gasdermin D (GSDMD) involves combining a GSDMD inhibitor with anti-PD-1 treatment. GSDMD facilitates the STAT1-induced transactivation of PD-L1 by modulating calcium (Ca2+) influx, promoting PD-L1 expression through histone deacetylases/signal transducer pathways, and influencing potassium (K+) efflux (similar to the effect of diazoxide), which leads to increased autophagy[147,148]. Adenosine 5′-triphosphate (ATP)-dependent potassium channels [K(ATP)] have been currently targeted for the treatment of different diseases using Diazoxide such as acute gastric ulcerations (EAGU)[149] and indomethacin-induced gastric ulceration (IIGU)[148]. Details of the HCC signaling pathway are presented in Figure 7[150-152].
Figure 7. Signaling pathways in HCC. Activation of WNT, VEGF, and EGFR receptors may lead to HCC tumor progression through PI3K/AKT, β-catenin, and Raf/ERK cascade.
Unfortunately, ICI therapy does not have enough RRs in many patients with HCC; therefore, finding replacements is necessary. The usage of meloxicam with anti-PD-1 therapy is observed to reflect enhanced results[153]. Androgen receptors (ARs) are shown to downregulate expression of PD-L1. Since men are more prone to HCC and dihydrotestosterone (DHT) is a ligand with high affinity for AR, this receptor can be a target against HCC specifically in men[154]. In the case of advanced HCC, nivolumab and sorafenib, a multi-kinase inhibitor, have shown promising results. Small patient cohorts have reported sunitinib, bevacizumab, epidermal growth factor receptor (EGFR) inhibitors, and mammalian target of rapamycin (mTOR) inhibitors to be effective, too[155,156].
In summary, IFN-Y, IFN-c, and osteopontin can upregulate and some molecules like WSX1 can downregulate PD-L1 expression. Moreover, many drugs showed positive results such as pembrolizumab and nivolumab in virus-associated HCC, Olaparib in ovarian cancer, and a combination of Gasdermin D (GSDMD) inhibitor and anti-PD-1 in GSDMD upregulated-HCC.
SQUAMOUS CELL CARCINOMA
Squamous cell carcinoma (SCC) is the second most common cause of skin malignancies[157], and it is not an exception to other cancers reviewed in this article in case of having correlations with PD-L1. Higher expression of PD-L1 is observed in some earlier histological changes that lead to SCC such as oral epithelium dysplasia (OED)[158]. The significance of PD-L1 expression is of great concern here, as its expression exceeding 1% can be associated with unfavorable prognostic outcomes[159]. A unique characteristic of SCC is its multiple subtypes, which are categorized based on their anatomical site of occurrence, such as oral squamous cell carcinoma (OSCC), head and neck squamous cell carcinoma (HNSCC), and so on. As a result of this variation, different patterns of PD-L1 expression are found in each type. One advantage of studying these patterns is to understand the differences in finding the optimum treatment in each case[160] [Figure 8]. The following paragraphs are dedicated to unique findings about PD-L1 expression/regulation in the most important subtypes of SCC.
Figure 8. (A) HNSCC signaling pathways. VEGFR, integrin, and EGFR/HER2 activate SRC, initiating three different regulatory pathways (STAT3, RAS/RAF, and Gab-1); eventually, all of these pathways lead to carcinogenic modifications. TKR starts the PI3K pathway and PTEN inhibits it. The function of NOTCH will also lead to cancer. (B) Esophageal SCC signaling pathway. There are about two kinds of separated pathways that trigger the cancerous gene amplification in the cell. The first one is activated by YAP1, SOX2, and SOX9. The PI3K and mTOR get activated, respectively. Activation of mTOR is responsible for genetic changes in the cell. There is also a novel regulatory pathway started by TOPK that finally triggers genetic changes by STAT3. (C) Cutaneous SCC. (D) Oral SCC. By binding the growth factors to the receptor tyrosine kinase, GEFs get activated. Due to that, some different pathways including SRC/STAT, PI3K/AKT/ERK, and RAS/RAF/ERK trigger the cancerous changes in cell DNA. (E) Lung SCC. Lung SCC signaling pathways. EGFR mutations affect two important pathways: PI3K and RAS. Additionally, FGFR1 amplifications activate STAT3 and RAS/RAF/MAPK. All of the mentioned pathways exacerbate the cancerous changes in the cell. The only inhibitory factor is PTEN by deactivating PIP2 to PKC converting reaction.
Oral squamous cell carcinoma
A fascinating finding about OSCC is the higher chance of nodal metastasis associated with high PD-L1 expression[161,162]. Progression of dysplasia to OSCC ensures the high expression of PD-L1 and its role in pre-malignancy immune tolerance[163]. PD-L1 positive group is supposed to be more severe, as it is seen in a study that lymph node metastasis is more and median disease-free survival is lower than PD-L1 negative group of patients with tongue squamous cell carcinoma. However, there is no difference in tumor size[164].
Esophageal squamous cell carcinoma
Pathology and depth of esophageal squamous cell carcinoma (ESCC) are positively related to PD-L1 expression[165]. A meta-analysis conducted in 2018 assessed data collected from almost 3,000 patients and reported that distant metastasis of ESCC has a two-sided link to PD-L1 expression, but unlike what we said about OSCC, nodal metastasis is not correlated with PD-L1 expression level[166].
Vulvar squamous cell carcinoma
Effects of high PD-L1 expression in vulvar squamous cell carcinoma (VSCC) are more serious than other types of SCC we have discussed up to now, as it is shown to be related to a higher risk of occurrence and mortality[167]. Yet, there is an interesting finding about the effects of high PD-L1 expression in VSCC. It is shown that PD-L1 expression can cause inflammation in the site of the tumor; consequently, the higher the inflammation, the higher the PD-L1 expression and the chance of successful anti-PD-L1 therapy[168].
Cervical squamous cell carcinoma
Unlike other types of SCC we have discussed until now, there are not many clinicopathological associations with high PD-L1 expression in cervical squamous cell carcinoma (CSCC)[169]. However, measuring PD-L1 expression can still be valuable as it is recognized to be a prognostic factor in studying cervical lesions[170]. It is mentioned in a study conducted in 2017 that there is a higher rate of PD-1 expression in squamous cell carcinoma in comparison to adenosquamous carcinoma (37.8% vs. 28.6%)[171]. High PD-L1 expression does not confirm the clinical presentation; however, it is notable that there was a higher expression of PD-L1 in young patients. As patients respond to chemotherapy clinically, it is more probable to see a decrease in
Head and neck squamous cell carcinoma
Several studies have reported the relevance of high PD-L1 expression and distant metastasis or lymph node metastasis. This is the third time that we have seen such a correlation in different types of SCCs reviewed in this paper. There is probably a positive connection between PD-L1 expression and invasion of the tumor cells[161,173,174]. Following the strain of metastasis, the same study[173] reported a higher rate of PD-L1 expression in patients under chemotherapy and, as a result of higher PD-L1, more chance of metastasis. Although there are many differences between the types of tumors in the HNSCC category and the patterns of involvement are diverse, generally, it can be said that the higher level of PD-L1 expression in a tumor can make this tumor more potent to respond to immunotherapy[175]. By using immune chemotherapy, which refers to a combinational therapy of immunotherapy and chemotherapy, there will be a great increase in ORR in patients with HNSCC[176-179].
Pulmonary/lung squamous cell carcinoma
In both types, high expression of PD-L1 is not shown to be correlated with clinicopathological characteristics[159,180], yet PD-L1 assessment can still be valuable since some studies have suggested its link to poor prognosis in lung squamous cell carcinoma (LSCC)[159].
Studying all the above is useless without talking about treatment. Herein, the drugs standing first in the line are ICIs. The use of anti-PD-1 mAb such as pembrolizumab and nivolumab was first approved by FDA in 2016 as a second-line treatment for recurrent and/or metastatic HNSCC[167,181,182]. Based on the positive outcomes, improved OS and quality of life in studies conducted with pembrolizumab, in 2019, the FDA approved the use of pembrolizumab as a first-line treatment in patients with recurrent, metastatic, or unresectable HNSCC with PD-L1 expression with a combined positive score (CPS) ≥ 1[160,174]. However, objective response rates (ORRs) of pembrolizumab and nivolumab are only 15%, indicating that research for more effective treatments is immensely in demand[174]. Searching in the world of ICIs, we found Cemiplimab, another anti-PD-1 mAb without the issue of low RR. A RR of almost 50% has been observed for this drug with maintenance for 6 months in some patients[183]. It is good to mention other drugs such as durvalumab and atezolizumab, which are being used in the treatment of HNSCC, yet their use is not as much as the heads of family, pembrolizumab and nivolumab[174]. PD-L1 has not been left intact either. Durvalumab is an anti-PD-L1 mAb that has shown an 18% overall response rate (ORR) in HNSCC patients with high PD-L1 expression and an 11% ORR in all patients[182]. One may interpret that most mABs against the PD-1/PD-L1 axis are used in HNSCC. Although this is true to some extent, these drugs are being tried to be used in other types of SCCs as soon as they are approved[163]. The use of pembrolizumab, nivolumab, and atezolizumab is approved in other types of SCC such as cervical, vulvar, oesophageal, and oral[163,167,168,180]. Looking at promising results of ICIs may make us overjoyed, but everything comes with a cost, and in the case of immunotherapy, toxicity and high costs are two downsides. This should make us more alert to choose the best cases for immunotherapy based on their PD-L1 profile, TME, or whatever is about to come[160,174]. It is worth knowing that immunotherapy is not limited to the PD-1/PD-L1 axis. Many other molecules like EGFR and LAG-3 are targeted in immunotherapy for SCC treatment, but these studies and ideas are inchoate and need to be thoroughly investigated[158,184]. Parallel to other cancers, intracellular pathways with regulatory effects on PD-L1 expression can be targeted for therapy. Myc-c is one of these pathways with great potential for ICIs and has shown such positive results that some consider it a prospect for treatment. Combinational therapy has also elicited good outcomes. For instance, paclitaxel is a strong candidate for combination with anti-PD-1 drugs[185].
Beyond theories, it seems that the use of pembrolizumab is the most effective therapy for patients with SCC, according to current studies and trials. However, ORRs for pembrolizumab and even nivolumab are not satisfactory yet[161,168,173,174]. In the case of pembrolizumab, an additional prognostic factor is found. PD-L2, which is mostly expressed in immune cells, can also be rarely expressed in other cells, and now the better prognosis is observed in HNSCCs with both PD-L1 and PD-L2 expression[165]. Some trials have reported positive outcomes of anti-PD-1 antibodies in cases of negative PD-L1 tumors. Herein, the PD-L1 blocker, durvalumab, has also been used and elicited an ORR of 12%, although both these studies reported their results about HNSCC[174]. As we mentioned earlier, other receptors such as EGFR are being targeted for immune therapy. Cetuximab, panitumumab, gefitinib, and dacomitinib are all anti-EGFR antibodies that showed positive results in phase II trials but have not been proven to be clinically useful[183].
SCC, as the second most common cause of skin malignancies, has correlations with PD-L1 expression. The expression of over 1% of PD-L1 shows poor prognosis and outcomes. Due to different subtypes of SCC, there are also many different patterns of PD-L1 expression. In most SCC subtypes, there is a correlation between high PD-L1 expression and tumor invasion. However, this correlation is not meaningful in CSSC. There are two drugs (pembrolizumab and nivolumab) that were approved by the FDA in 2019 and 2016, respectively. They are both anti-PD-1 mAb with an ORR of 15%. There are also other useful mAbs such as Cemiplimab, durvalumab, and atezolizumab for various SCCs. The intracellular pathway can be set as a target for therapy. There are also other targets like EGFR receptors (Cetuximab, panitumumab, gefitinib, and dacomitinib).
COLORECTAL CANCER
The role of PD-L1-expressing tumors has been investigated in PD-1 targeting therapy, which has shown increased antitumor activity due to more immune cell infiltration. However, anti-PD-L1 therapy has been proven to be more effective in combination therapies for colorectal carcinomas (CRCs). The findings have validated the involvement of STAT3 in triggering apoptosis and have shown that inhibiting STAT3 can lead to decreased PD-L1 expression and increased apoptosis. Inhibiting STAT3 has also led to a decrease in T-reg populations and function, reducing IL-10 production and immune tolerance to tumor antigens[186,187]. PD-L1 expression could serve as a novel indicator of an unfavorable outlook in CRC, since it enables tumor cells to avoid immune detection and bolster the activity of T-regulatory cells[138]. PTGS1 (cyclooxygenase-1) and PTGS2 (cyclooxygenase-2) enzymes produce prostaglandins, including PGE2, which can lead to local inflammation and enable tumor cells to escape detection by the immune system [Figures 9 and 10]. Experimental proof backs a synergistic impact of aspirin and immune checkpoint blockade in stimulating T cell-mediated antitumor immune response, suggesting that the activated state of immune checkpoint in cells within the tumor microenvironment might confer resistance to aspirin. The presence of high levels of CD3+, CD8+, and CD45RO+ cells in CRC tissue has been linked to improved patient survival, indicating that their densities, as determined by IHC, can serve as a gauge of the antitumor T cell-mediated immune response to colorectal tumors. CD8+ T cells play a critical role in PD-1 inhibitors and contribute to antitumor immunity in CRC. Thus, anti-PD-1 therapy, which relies on CD8+ cells, can impede CRC cell metastasis to the intestines, liver, and lungs, ultimately extending the survival of CRC mice. This suggests that inhibiting PD-1 with a focus on CD8+ cells could be a promising treatment for CRC[188-192].
Figure 9. Colorectal cancer overview. Some plasma membrane receptors are involved in colorectal cancer, such as tumor necrosis factor receptor, transforming growth factor receptor, LRP5/6, EP1, EP2, EP3, EP4, EGFR, etc.
Figure 10. Aspirin inhibits both COX-1 and COX-2. Arachidonic acid makes PGH2 with the help of COX-1 and COX-2. The following derivatives of PGH2 bind to their receptors and induce their specific effects.
PD-L1 expression could potentially indicate a poor prognosis in CRC. Additionally, higher levels of CD3+, CD8+, and CD45RO+ cells have been linked to improved patient survival, indicating that the density of these T cells may reflect an antitumor T cell-mediated immune response to colorectal tumors. Lastly, it is proposed that inhibiting PD-1 with CD8+ cells could be a viable treatment for CRC.
OTHER CANCERS
Bladder cancer is a prevalent medical condition that ranks as the 10th most common neoplasm worldwide and the 13th most fatal. In 90% of bladder cancer cases worldwide, the condition is primarily caused by urothelial cell bladder cancer[193,194]. Currently, four PD-1/PD-L1 inhibitors have been approved for treating locally advanced or metastatic urothelial carcinoma of the bladder and the upper urinary tract. These include Atezolizumab, Pembrolizumab (used as first-line treatments), Nivolumab, and Durvalumab. However, there are still many unclear and unfinished aspects of the PD-L1 pathway in advanced urothelial carcinoma that need to be refined[195,196]. Surprisingly, some classes of anti-PD-L1 antibodies can block the interaction of PD-L1 and B7 proteins. This additional blockage is favorable because PD-L1/B7 interaction can suppress T cells[197,198]. Anti-PD-L1 drugs have shown positive outcomes in urothelial carcinoma, NSCLC, and Merkel cell carcinoma treatment[199,200]. Again, combinational therapy is an option. Axitinib, a small molecule inhibiting tyrosine kinase, is hypothesized to be effective when used in combination with pembrolizumab in advanced renal cell carcinoma (RCC). However, evidence in this case is not enough, and bigger and more complete trials are required[201]. Therapy with ICIs is not limited to malignancies caused by endogenous mutations, but also malignancies caused by microbiological agents. In virus-associated malignancies such as Epstein-Barr virus (EBV)-associated nasopharyngeal cancer, hepatitis B virus (HBV)-related HCC and HNSCC, and T cell leukemia virus-1-associated T cell leukemia/lymphoma,
List of clinical trials assessing some cancers. Dugs such as camrelizumab, platinum irinotecan, atezolizumab, durvalumab, carboplatin. Oxaliplatin, etc. has shown potential benefits in the treatment of small cell lung cancer, squamous cell carcinoma, ductal carcinoma, ovarian cancer, rectal cancer, etc.
First author | Cancer | Drug | Dose | No. patients | Follow-up (month) | Outcome | Adverse effects | Type of study |
Ni et al., 2023[203] | ES-SCLC (Extensive-stage small cell lung cancer) | Camrelizumab & platinum irinotecan | - | 19 | 12.1 | Objective response rate of 89.6% | Neutropenia, diarrhea | Clinical trial |
Ni et al., 2023[203] | ES-SCLC | Atezolizumab, durvalumab) plus platinum etoposide | - | 34 | 12.1 | Objective response rate of 82.4% | - | Clinical trial |
Patil et al., 2023[204] | (LAHNSCC) Locally advanced head and neck squamous cell carcinoma | Radiation with concurrent docetaxel | - | 178 | 25.5 | Improved 2-year overall survival | Mucositis, odynophagia, and dysphagia | Clinical trial phase II/III |
O'Shea et al., 2023[205] | Ductal carcinoma | Nelipepimut-S peptide vaccine | - | 13 females | 6 | Adaptive immune response stimulation | - | Clinical trial |
Li et al., 2023[206] | Bone metastases from solid tumors | QL1206 (denosumab biosimilar) | 120 mg | 357 | 18.25 | Efficacy, tolerable safety, and pharmacokinetics equivalent to denosumab | - | Clinical trial |
Penson et al., 2023[207] | Recurrent ovarian cancer | Carboplatin, gemcitabine, iniparib (GCI combination) | gemcitabine | 87 | 12 | GCI tolerance | Nausea, vomiting, neutropenia, anemia, dehydration, thrombocytopenia, hypokalemia | Clinical trial phase II |
Zheng et al., 2023[208] | locally advanced rectal cancer | Capecitabine plus oxaliplatin, chemotherapy, tislelizumab, and radiotherapy | oxaliplatin | 50 | Every | High level of immune effect | - | Clinical trial phase II |
Topalian et al., 2012[209] | Advanced melanoma, colorectal cancer, NSCLC, prostate cancer | BMS-936558 | 0.1-10 mg/kg | 296 | 24 | Objective response rate of 36% | Pulmonary toxicity | Cohort |
THE EFFECT OF EXPOSURE ON IMMUNITY AND IMMUNE CHECKPOINT INHIBITORS
The effectiveness of PD-L1/PD-1 pathway inhibitors in treating Non-Small Cell Lung Cancer (NSCLC) could be impacted by environmental factors such as the patient's microbiota and smoking habits. Smoking status could be a promising alternative prognostic indicator for patients suffering from advanced NSCLC, especially in combination with TMB value[210]. A 2019 study revealed that patients with highly diverse gut microbiota tend to exhibit more memory T cell and natural killer cell aggregation, leading to a better prognosis when treated with nivolumab than patients with less diverse microbiota. This outcome is attributed to the immune regulatory function of short-chain fatty acids produced in and secreted from microbiota. Bifidobacterium is a microorganism that can positively affect the efficacy of anti-PD-L1 medications. Meanwhile, species such as Bacteroids (specifically B. fragilis and B. thetaiotaomicron) and Faecalibactirium have a significant beneficial impact on the efficacy of PD-1 and CTLA-4 blockade[211-213].
Response to immunotherapy relies on several factors. One factor to mention is gut microbiota, which is shown to be potent in cases of melanoma and other cancers such as hepatocellular carcinoma[214]. It is demonstrated that the alpha diversity of microbiota is higher in responsive patients to anti-PD-1 therapy; moreover, specific types of bacteria may be more abundant in the gut of responsive patients[215]. In non-responsive patients, one solution that sparks is changing gut microbiota. Fecal microbiota transplant is one modality to change the microbiota system; however, very few trials have been established for this modality and unpromising response rates to immunotherapy after transplant have been reported[216,217]. Other modalities such as using chemicals have been experimented. For instance, Diosgenin, a natural steroid saponin has been able to improve microbiota and antitumor response in mouse models[218,219]. A study done in a cancer center in Hoston showed that the diversity of gut microbes boosts immunotherapy with nivolumab and the number of CD8+ T cells in metastatic melanoma patients, especially when there is an abundance of Clostridium species[220].
The relationship between the gut microbiome and the response to immune checkpoint inhibitors (ICIs) is not fully understood, but it is believed that gut microbial metabolites play a crucial role in linking the gut microbiome to systemic immunity. Short-chain fatty acids (SCFAs) are the primary end products of gut microbe-mediated metabolism, generated through the anaerobic fermentation of dietary fibers in the intestine. SCFAs exhibit immunomodulatory functions and can impact CD4+ T cells and antigen-presenting cells. Specifically, butyric acid (BA) and other SCFAs have been found to enhance the expression of IFNγ and granzyme B in CD8+ cytotoxic T lymphocytes and interleukin-17-secreting CD8+ T cells. Moreover, SCFAs, particularly BA and Valeric acid (VA), can inhibit histone deacetylases, which have been found to increase the response to immunotherapy[221].
The effectiveness of anti-CTLA-4 antibodies was observed to decrease in the absence of gut commensal bacteria but was notably improved in the presence of two species from the Bacteroidales order (Bacteroidetes phylum) and one species from the Burkholderiales order (Proteobacteria phylum). These two species were also discovered to alleviate the histopathological indications of colitis, a common immune-related adverse event linked to anti-CTLA-4 antibody therapy. By stimulating the production of IL-6, these microorganisms initiate a series of signals that elevate the levels of suppressor Treg cells in the tumor microenvironment[222].
It has been proven that obesity can suppress CD8+ T cell’s antitumor activity through the leptin/PD-L1-STAT3-FAO (fatty acid oxidation) pathway in breast cancer. This pathway inhibits T cell glycolysis and releases IFNy and Th1 cytokines[223]. Thus, immunotherapy with anti-PD-L1 drugs makes a good response in obese patients[224]. Identifying the molecular mechanisms that enable microbes to communicate with cells that modulate the immune system is imperative. This is crucial to enhance our understanding of how to effectively evaluate and quantify the contribution of commensal bacterial populations in promoting the success of immunotherapy.
DISCUSSION & CONCLUSION
This paper has attempted to have an overall look at cancer and its molecular aspects to shed light on recent advances and evaluated studies with different characteristics [Table 2]. To our knowledge, there has been no systematic review to assess all the clinical and empirical data together and prepare it in a comprehensive and understandable design. Despite the existing knowledge about treatments and medications, it is always beneficial to look at the existing literature to discover gaps and opportunities to push the field forward and find better ways to alleviate symptoms such as pain and anxiety in disorders like HNSCC[225].
Summary of the main characteristics of the included studies. Studies are compared based on study design, participants and sample size, intervention, disease type, and provided results
Study detail | Study design | Participants & sample size | Intervention | Disease type | Results |
Topalian et al., 2012[209] | Clinical trial (phase I) | 296 patients | 0.1-10.0 mg/kg of anti-PD-1 antibody every 2 weeks | Advanced melanoma, colorectal cancer, NSCLC, prostate cancer | Objective response to anti-PD-1 antibody |
Cho et al., 2022[64] | Randomized trial (phase II) | Patients with chemotherapy-naive, PD-L1-positive | Tiragolumab (600 mg) once 3 weeks | NSCLC | Tiragolumab plus Atezolizumab combination for the treatment of NSCLC |
Rittmeyer et al., 2017[226] | Randomized, open-label trial (phase III) | Patients with squamous carcinoma or NSCLC (18 years or older) | Atezolizumab 1,200 mg or docetaxel 75 mg/m2 every 3 weeks | SCC, NSCLC | Improvement of overall survival with atezolizumab versus docetaxel |
Nomura et al., 2020[227] | Randomized trial (phase III) | 216 patients | Atezolizumab/nivolumab/pembrolizumab monotherapy 1,200/240/200 mg/body for 2/3/3 weeks, pembrolizumab | NSCLC | the non-inferiority of ICI discontinuation compared to continuation |
Khan et al., 2017[228] | Case report | A 3-year-old woman | Two cycles of ipilimumab and nivolumab, last administered 3 weeks before her presentation | Melanoma | autoimmune hemolytic anemia |
Kunimasa et al., 2018[229] | Case report | A 53-year-old man | Pembrolizumab | NSCLC | Skin rash due to reinvigorated CD8+ |
Zahoor et al., 2022[230] | Case report | A 52-year-old woman | Nivolumab 140 mg every two weeks (43 cycles) | SCC | Paronychia, blackish discoloration of the nails |
Cubillos-Zapata et al., 2019[231] | Cross-sectional | 476 patients (aged > 18 years) | - | Melanoma | Increased serum levels of sPD-L1 |
Huang et al., 2018[232] | Clinical trial | 13 chemotherapy patients | Chemotherapy | SCC | Activation of immune system function in SCC |
Stenmark et al., 2023[233] | Randomized clinical trial (phase III) | 1,178 patients | Radiotherapy | Breast Cancer | Identification of tumors with immunological biomarkers |
Eichhorn et al., 2019[234] | Clinical trial (phase II) | 30 patients with NSCLC | Pembrolizumab 200 mg every 3 weeks | NSCLC | Neoadjuvant immunotherapy, along with lung surgery |
Piersiala et al., 2021 [235] | Observational study | Patients with SCC | - | SCC | Lymph nodes flowcytometry as a method for PD-1 level investigation |
Miyoshi et al., 2016[236] | Case report | a 66-year-old woman with advanced melanoma | Nivolumab 2 mg/kg every 3 weeks | Melanoma | Diabetic ketoacidosis for differential diagnosis of patients receiving nivolumab |
Vienot et al., 2023[237] | Clinical trial (phase II) | Patients with HCC | Atezolizumab 1,200 mg IV, bevacizumab 15 mg/kg IV | HCC | Anti-PD-1/PD-L1 therapy plus anti-telomerase vaccine in HCC therapy |
Bahig et al., 2019[182] | Clinical trial (phase I & II) | 35 patients | durvalumab 1,500 mg IV every 4 weeks, tremelimumab 75 mg IV every 4 weeks | SCC | Local and systemic disease control by combining durvalumab and tremelimumab |
Yun et al., 2020[238] | Case report | A 63-year-old man | Pembrolizumab 2 mg/kg for 14 months | NSCLC | Vitiligo-like depigmentation |
Kunimasa et al., 2018[239] | Case report | A 48-year-old, never-smoking woman with left cervical lymphadenopathy | Heparin infusion, direct oral anticoagulants (DOAC) | NSCLC | Immediate continuous heparin infusion improved their symptoms and continuing pembrolizumab with direct oral anticoagulant successfully induced tumor shrinkage |
Cui et al., 2023[240] | Case report | a 64-year-old man | Two cycles of neoadjuvant tislelizumab treatment coupled with chemotherapy | NSCLC | Neoadjuvant immunotherapy for PD-L1-negative NSCLC patients before surgical intervention |
Althammer et al., 2019[54] | Clinical trial (phase I & II) | 163 patients | Durvalumab | NSCLC | An automated CD8xPD-L1 signature to identify NSCLC patients with durvalumab therapy |
Merhi et al., 2018[241] | Case report | A 71-year-old man | Nivolumab | SCC | The presence of anti-NY-ESO-1 integrated immunity to identify PD-1 blockade response in HNSCC patients |
Ribas et al., 2016[242] | Clinical trials (phase I) | Patients (> 18 years) with advanced or metastatic melanoma | Pembrolizumab 10 mg/kg every 2 weeks | Melanoma | Overall objective response rate of 33% |
Pazoki-Toroudi et al., 2009[243] | Comparative study | Wistar rats (210-150 g) | Xylazine (10 mg/kg), Ketamine HCl (50 mg/kg) | I/R injury | Renal function improved |
Habibey et al., 2008[244] | Comparative study | Male wistar rats (180-240 g) | Morphine (20 mg/kg) | I/R injury | Protective effect of morphine on kidney |
Shoorei et al., 2019[245] | In vitro | 50 female mice preantral follicles (12-14-week-old) | Hesperidin (22.5, and 50 µmol/L) | - | Antifertility effects of hesperidin |
Among the clinical trials reviewed, there is a clear diversity of the medications used. It seems that after the early and successful exposure and FDA approval of pembrolizumab[58] and nivolumab[57], a consensus for the next medication is still not achieved. Another finding, which authors believe to be a scarcity, is the existence of few studies that include more than 200 patients. In addition, follow-up periods rarely break through a year. However, a positive current is that the number of studies conducted in recent years is growing rapidly. Details are presented in Table 2.
Cancer is a hot topic in medicine nowadays and there are vast opportunities for growth and innovation. The first suggestion of the authors is shifting the focus to other medications rather than pembrolizumab and nivolumab. As mentioned previously, these drugs are proven to be the most effective ones in ICI therapy, but as we found out, a major detrimental issue is the lack of compatibility between drug and disease[58,63]. The trials were mostly designed with drugs other than pembrolizumab and nivolumab, and combinational therapy was considered well but not enough. As a result, our second applicable suggestion is to consider new methods and therapies.
Standing where we are now, applying statistical analysis is currently not possible. The integrity of the data is not satisfying and the quality of studies is not high, thus preventing researchers from making more serious attempts. Due to the reasons mentioned, no one can statistically analyze the current literature at the moment, so one limitation of our study is the comprehensive nature of the final implications. Another limitation is that we could not assess subtypes of cancer separately and we presented it as a whole material. Moreover, there is not enough information about the influence of smoking, alcohol, food/drink intake, obesity, etc. on drug effects and the immune system. Thus, more research in this field is strongly recommended. The authors could not find any information about molecular pathological epidemiology of those factors in relation to molecular pathologies and clinical outcomes including effects and side effects of immunotherapies, which can be considered another limitation of this study. However, this action could not benefit the goals of this paper and its design to cover the field wholistically. The only goal of conducting this study was to find out these gaps, and if one wants to remember only one point after reading this paper, it is the necessity for clinical studies on different types and subtypes of each cancer regarding ICI therapy. Moreover, the authors visualize a very bright opportunity for analyzing, reviewing, and even proposing new therapies or guidelines for treatment.
DECLARATIONS
Acknowledgments
We would like to thank Mr. Ali Niavarani for conducting the scientific illustrations and graphic design for the manuscript figures.
Author’s contributions
Contribution to conception, investigation and interpretation: Sabaghian A, Gupta Y, Prakash A, Pazoki-Toroudi H
Writing the manuscript draft and revisions: Sabaghian A, Shamsabadi S, Momeni S, Mohammadikia M, Mohebbipour K, Sanami S, Ahmad S, Akhtar N, Sharma NR, Kushwah RBS
Availability of data and materials
Not applicable
Financial support and sponsorship
None.
Conflict of Interest
Prakash A is a Junior Editorial Board member of the Journal of Cancer Metastasis and Treatment, while the other authors have declared that they have no conflicts of interest.
Ethical Approval and Consent to Participate
Not applicable.
Consent for publication
Not applicable.
Copyright
© The Author(s) 2024.
Supplementary Materials
REFERENCES
1. Smyth EC, Nilsson M, Grabsch HI, van Grieken NC, Lordick F. Gastric cancer. Lancet. 2020;396:635-48.
2. Loibl S, Poortmans P, Morrow M, Denkert C, Curigliano G. Breast cancer. Lancet. 2021;397:1750-69.
5. Kroemer G, Pouyssegur J. Tumor cell metabolism: cancer’s Achilles’ heel. Cancer Cell. 2008;13:472-82.
6. Sharma P, Jhawat V, Mathur P, Dutt R. Innovation in cancer therapeutics and regulatory perspectives. Med Oncol. 2022;39:76.
7. Ai L, Xu A, Xu J. Roles of PD-1/PD-L1 pathway: signaling, cancer, and beyond. Adv Exp Med Biol. 2020;1248:33-59.
8. Chen G, Huang AC, Zhang W, et al. Exosomal PD-L1 contributes to immunosuppression and is associated with anti-PD-1 response. Nature. 2018;560:382-6.
9. Gordon SR, Maute RL, Dulken BW, et al. PD-1 expression by tumour-associated macrophages inhibits phagocytosis and tumour immunity. Nature. 2017;545:495-9.
10. Kim MJ, Kim K, Park HJ, et al. Deletion of PD-1 destabilizes the lineage identity and metabolic fitness of tumor-infiltrating regulatory T cells. Nat Immunol. 2023;24:148-61.
11. Wartewig T, Daniels J, Schulz M, et al. PD-1 instructs a tumor-suppressive metabolic program that restricts glycolysis and restrains AP-1 activity in T cell lymphoma. Nat Cancer. 2023;4:1508-25.
12. Topalian SL, Taube JM, Pardoll DM. Neoadjuvant checkpoint blockade for cancer immunotherapy. Science. 2020;367:eaax0182.
13. Doroshow DB, Bhalla S, Beasley MB, et al. PD-L1 as a biomarker of response to immune-checkpoint inhibitors. Nat Rev Clin Oncol. 2021;18:345-62.
14. Pardoll DM. The blockade of immune checkpoints in cancer immunotherapy. Nat Rev Cancer. 2012;12:252-64.
15. Topalian SL, Drake CG, Pardoll DM. Immune checkpoint blockade: a common denominator approach to cancer therapy. Cancer Cell. 2015;27:450-61.
16. Ferlay J, Soerjomataram I, Dikshit R, et al. Cancer incidence and mortality worldwide: sources, methods and major patterns in GLOBOCAN 2012. Int J Cancer. 2015;136:E359-86.
17. Marin-Acevedo JA, Dholaria B, Soyano AE, Knutson KL, Chumsri S, Lou Y. Next generation of immune checkpoint therapy in cancer: new developments and challenges. J Hematol Oncol. 2018;11:39.
18. Leemans CR, Snijders PJF, Brakenhoff RH. The molecular landscape of head and neck cancer. Nat Rev Cancer. 2018;18:269-82.
19. Siegel RL, Miller KD, Fuchs HE, Jemal A. Cancer statistics, 2021. CA Cancer J Clin. 2021;71:7-33.
20. Ishida Y, Agata Y, Shibahara K, Honjo T. Induced expression of PD-1, a novel member of the immunoglobulin gene superfamily, upon programmed cell death. EMBO J. 1992;11:3887-95.
21. Dong H, Zhu G, Tamada K, Chen L. B7-H1, a third member of the B7 family, co-stimulates T-cell proliferation and interleukin-10 secretion. Nat Med. 1999;5:1365-9.
22. Freeman GJ, Long AJ, Iwai Y, et al. Engagement of the PD-1 immunoinhibitory receptor by a novel B7 family member leads to negative regulation of lymphocyte activation. J Exp Med. 2000;192:1027-34.
23. Bretscher PA. A two-step, two-signal model for the primary activation of precursor helper T cells. Proc Natl Acad Sci USA. 1999;96:185-90.
24. Oyer JL, Gitto SB, Altomare DA, Copik AJ. PD-L1 blockade enhances anti-tumor efficacy of NK cells. Oncoimmunology. 2018;7:e1509819.
26. Yi M, Niu M, Xu L, Luo S, Wu K. Regulation of PD-L1 expression in the tumor microenvironment. J Hematol Oncol. 2021;14:10.
27. Fanoni D, Tavecchio S, Recalcati S, et al. New monoclonal antibodies against B-cell antigens: possible new strategies for diagnosis of primary cutaneous B-cell lymphomas. Immunol Lett. 2011;134:157-60.
28. Terme M, Ullrich E, Aymeric L, et al. IL-18 induces PD-1-dependent immunosuppression in cancer. Cancer Res. 2011;71:5393-9.
29. Bally AP, Austin JW, Boss JM. Genetic and epigenetic regulation of PD-1 expression. J Immunol. 2016;196:2431-7.
30. Zhang X, Schwartz JC, Guo X, et al. Structural and functional analysis of the costimulatory receptor programmed death-1. Immunity. 2004;20:337-47.
31. Lázár-Molnár E, Yan Q, Cao E, Ramagopal U, Nathenson SG, Almo SC. Crystal structure of the complex between programmed death-1 (PD-1) and its ligand PD-L2. Proc Natl Acad Sci USA. 2008;105:10483-8.
32. Wolchok JD, Kluger H, Callahan MK, et al. Nivolumab plus ipilimumab in advanced melanoma. N Engl J Med. 2013;369:122-33.
33. Nishimura H, Nose M, Hiai H, Minato N, Honjo T. Development of lupus-like autoimmune diseases by disruption of the PD-1 gene encoding an ITIM motif-carrying immunoreceptor. Immunity. 1999;11:141-51.
34. Lake RA, Robinson BW. Immunotherapy and chemotherapy-a practical partnership. Nat Rev Cancer. 2005;5:397-405.
35. Rabinovich GA, Gabrilovich D, Sotomayor EM. Immunosuppressive strategies that are mediated by tumor cells. Annu Rev Immunol. 2007;25:267-96.
36. Chen L. Co-inhibitory molecules of the B7-CD28 family in the control of T-cell immunity. Nat Rev Immunol. 2004;4:336-47.
37. Zou W, Chen L. Inhibitory B7-family molecules in the tumour microenvironment. Nat Rev Immunol. 2008;8:467-77.
38. Sznol M. Blockade of the B7-H1/PD-1 pathway as a basis for combination anticancer therapy. Cancer J. 2014;20:290-5.
39. Taube JM, Anders RA, Young GD, et al. Colocalization of inflammatory response with B7-h1 expression in human melanocytic lesions supports an adaptive resistance mechanism of immune escape. Sci Transl Med. 2012;4:127ra37.
40. Jiang X, Zhou J, Giobbie-Hurder A, Wargo J, Hodi FS. The activation of MAPK in melanoma cells resistant to BRAF inhibition promotes PD-L1 expression that is reversible by MEK and PI3K inhibition. Clin Cancer Res. 2013;19:598-609.
41. Mittendorf EA, Philips AV, Meric-Bernstam F, et al. PD-L1 expression in triple-negative breast cancer. Cancer Immunol Res. 2014;2:361-70.
42. Song M, Chen D, Lu B, et al. PTEN loss increases PD-L1 protein expression and affects the correlation between PD-L1 expression and clinical parameters in colorectal cancer. PLoS One. 2013;8:e65821.
43. Amani H, Ajami M, Nasseri Maleki S, et al. Targeting signal transducers and activators of transcription (STAT) in human cancer by dietary polyphenolic antioxidants. Biochimie. 2017;142:63-79.
44. Podojil JR, Turley DM, Miller SD. Therapeutic blockade of T-cell antigen receptor signal transduction and costimulation in autoimmune disease. Adv Exp Med Biol. 2008;640:234-51.
46. Spranger S, Spaapen RM, Zha Y, et al. Up-regulation of PD-L1, IDO, and T(regs) in the melanoma tumor microenvironment is driven by CD8+ T cells. Sci Transl Med. 2013;5:200ra116.
47. Massi D, Brusa D, Merelli B, et al. PD-L1 marks a subset of melanomas with a shorter overall survival and distinct genetic and morphological characteristics. Ann Oncol. 2014;25:2433-42.
48. Abiko K, Mandai M, Hamanishi J, et al. PD-L1 on tumor cells is induced in ascites and promotes peritoneal dissemination of ovarian cancer through CTL dysfunction. Clin Cancer Res. 2013;19:1363-74.
49. Wang L, Liu H, Chen X, Zhang M, Xie K, Ma Q. Immune sculpting of norepinephrine on MHC-I, B7-1, IDO and B7-H1 expression and regulation of proliferation and invasion in pancreatic carcinoma cells. PLoS One. 2012;7:e45491.
50. Kim DH, Kim H, Choi YJ, et al. Exosomal PD-L1 promotes tumor growth through immune escape in non-small cell lung cancer. Exp Mol Med. 2019;51:1-13.
51. Al Bakir M, Huebner A, Martínez-Ruiz C, et al. The evolution of non-small cell lung cancer metastases in TRACERx. Nature. 2023;616:534-42.
52. Mignon S, Willard-Gallo K, Van den Eynden G, et al. The relationship between tumor-infiltrating lymphocytes, PD-L1 expression, driver mutations and clinical outcome parameters in non-small cell lung cancer adenocarcinoma in patients with a limited to no smoking history. Pathol Oncol Res. 2020;26:1221-8.
53. Zhang N, Zeng Y, Du W, et al. The EGFR pathway is involved in the regulation of PD-L1 expression via the IL-6/JAK/STAT3 signaling pathway in EGFR-mutated non-small cell lung cancer. Int J Oncol. 2016;49:1360-8.
54. Althammer S, Tan TH, Spitzmüller A, et al. Automated image analysis of NSCLC biopsies to predict response to anti-PD-L1 therapy. J Immunother Cancer. 2019;7:121.
55. Zhang Y, Huang S, Gong D, Qin Y, Shen Q. Programmed death-1 upregulation is correlated with dysfunction of tumor-infiltrating CD8+ T lymphocytes in human non-small cell lung cancer. Cell Mol Immunol. 2010;7:389-95.
56. Akbay EA, Koyama S, Carretero J, et al. Activation of the PD-1 pathway contributes to immune escape in EGFR-driven lung tumors. Cancer Discov. 2013;3:1355-63.
57. Huang Z, Su W, Lu T, et al. First-line immune-checkpoint inhibitors in non-small cell lung cancer: current landscape and future progress. Front Pharmacol. 2020;11:578091.
58. Villaruz LC, Socinski MA. The clinical utility of PD-L1 testing in selecting non-small cell lung cancer patients for PD1/PD-L1-directed therapy. Clin Pharmacol Ther. 2016;100:212-4.
59. Sepesi B, Nelson DB, Mitchell KG, et al. Prognostic value of PD-L1 mRNA sequencing expression profile in non-small cell lung cancer. Ann Thorac Surg. 2018;105:1621-6.
60. Tseng JS, Yang TY, Wu CY, et al. Characteristics and predictive value of PD-L1 status in real-world non-small cell lung cancer patients. J Immunother. 2018;41:292-9.
61. Bozinovski S, Vannitamby A, Rangamuwa K, et al. Integrating endobronchial ultrasound bronchoscopy with molecular testing of immunotherapy biomarkers in non-small cell lung cancer. Transl Lung Cancer Res. 2021;10:2779-87.
62. Zhang J, Gao J, Li Y, et al. Circulating PD-L1 in NSCLC patients and the correlation between the level of PD-L1 expression and the clinical characteristics. Thorac Cancer. 2015;6:534-8.
63. Zhao S, Gao G, Li W, et al. Antibiotics are associated with attenuated efficacy of anti-PD-1/PD-L1 therapies in Chinese patients with advanced non-small cell lung cancer. Lung Cancer. 2019;130:10-7.
64. Cho BC, Abreu DR, Hussein M, et al. Tiragolumab plus atezolizumab versus placebo plus atezolizumab as a first-line treatment for PD-L1-selected non-small-cell lung cancer (CITYSCAPE): primary and follow-up analyses of a randomised, double-blind, phase 2 study. Lancet Oncol. 2022;23:781-92.
65. Herbst RS, Arkenau HT, Santana-Davila R, et al. Ramucirumab plus pembrolizumab in patients with previously treated advanced non-small-cell lung cancer, gastro-oesophageal cancer, or urothelial carcinomas (JVDF): a multicohort, non-randomised, open-label, phase 1a/b trial. Lancet Oncol. 2019;20:1109-23.
66. Wrangle JM, Velcheti V, Patel MR, et al. ALT-803, an IL-15 superagonist, in combination with nivolumab in patients with metastatic non-small cell lung cancer: a non-randomised, open-label, phase 1b trial. Lancet Oncol. 2018;19:694-704.
67. Yang R, Pei T, Huang R, et al. Platycodon grandiflorum Triggers antitumor immunity by restricting PD-1 expression of CD8+ T cells in local tumor microenvironment. Front Pharmacol. 2022;13:774440.
68. Anagnostou VK, Brahmer JR. Cancer immunotherapy: a future paradigm shift in the treatment of non-small cell lung cancer. Clin Cancer Res. 2015;21:976-84.
69. Aiad M, Tahir A, Fresco K, et al. Does the combined use of aspirin and immunotherapy result in better outcomes in non-small cell lung cancer than immunotherapy alone? Cureus. 2022;14:e25891.
70. Cabrita R, Mitra S, Sanna A, et al. The role of PTEN loss in immune escape, melanoma prognosis and therapy response. Cancers. 2020;12:742.
71. Tsao H, Goel V, Wu H, Yang G, Haluska FG. Genetic interaction between NRAS and BRAF mutations and PTEN/MMAC1 inactivation in melanoma. J Invest Dermatol. 2004;122:337-41.
72. Adeleke S, Okoli S, Augustine A, et al. Melanoma-the therapeutic considerations in the clinical practice. Ann Palliat Med. 2023;12:1355-72.
73. Davis EJ, Johnson DB, Sosman JA, Chandra S. Melanoma: what do all the mutations mean? Cancer. 2018;124:3490-9.
74. Pietra G, Vitale M, Moretta L, Mingari MC. How melanoma cells inactivate NK cells. Oncoimmunology. 2012;1:974-5.
75. Lee H, Da Silva IP, Palendira U, Scolyer RA, Long GV, Wilmott JS. Targeting NK cells to enhance melanoma response to immunotherapies. Cancers. 2021;13:1363.
76. Balsamo M, Scordamaglia F, Pietra G, et al. Melanoma-associated fibroblasts modulate NK cell phenotype and antitumor cytotoxicity. Proc Natl Acad Sci USA. 2009;106:20847-52.
77. Alavi S, Stewart AJ, Kefford RF, Lim SY, Shklovskaya E, Rizos H. Interferon signaling is frequently downregulated in melanoma. Front Immunol. 2018;9:1414.
78. Taube JM, Young GD, McMiller TL, et al. Differential expression of immune-regulatory genes associated with PD-L1 display in melanoma: implications for PD-1 pathway blockade. Clin Cancer Res. 2015;21:3969-76.
79. de Waal AC, Aben KK, van Rossum MM, Kiemeney LA. Melanoma of unknown primary origin: a population-based study in the Netherlands. Eur J Cancer. 2013;49:676-83.
80. Mehdi A, Attias M, Mahmood N, et al. Enhanced anticancer effect of a combination of S-adenosylmethionine (SAM) and immune checkpoint inhibitor (ICPi) in a syngeneic mouse model of advanced melanoma. Front Oncol. 2020;10:1361.
81. Ascierto PA, Grimaldi AM, Anderson AC, et al. Future perspectives in melanoma research: meeting report from the “Melanoma Bridge”, Napoli, December 5th-8th 2013. J Transl Med. 2014;12:277.
82. Chapman PB, Hauschild A, Robert C, et al. Improved survival with vemurafenib in melanoma with BRAF V600E mutation. N Engl J Med. 2011;364:2507-16.
83. Algazi A, Bhatia S, Agarwala S, et al. Intratumoral delivery of tavokinogene telseplasmid yields systemic immune responses in metastatic melanoma patients. Ann Oncol. 2020;31:532-40.
84. Mahnke K, Enk AH. TIGIT-CD155 interactions in melanoma: a novel Co-inhibitory pathway with potential for clinical intervention. J Invest Dermatol. 2016;136:9-11.
85. Hu Z, Yu P, Du G, et al. PCC0208025 (BMS202), a small molecule inhibitor of PD-L1, produces an antitumor effect in B16-F10 melanoma-bearing mice. PLoS One. 2020;15:e0228339.
86. Zhang R, Zhong L, Liu X, et al. Microfluidic PLGA microcapsules with PD-L1 aptamers and docetaxel encapsulation for enhancing tumor immunity. Appl Mater Today. 2022;27:101484.
87. Qiu N, Liu Y, Liu Q, et al. Celastrol nanoemulsion induces immunogenicity and downregulates PD-L1 to boost abscopal effect in melanoma therapy. Biomaterials. 2021;269:120604.
88. Gu Z, Wang Q, Shi Y, et al. Nanotechnology-mediated immunochemotherapy combined with docetaxel and PD-L1 antibody increase therapeutic effects and decrease systemic toxicity. J Control Release. 2018;286:369-80.
89. Vera-Aguilera J, Perez-Torres A, Beltran D, et al. Novel treatment of melanoma: combined parasite-derived peptide GK-1 and anti-programmed death ligand 1 therapy. Cancer Biother Radiopharm. 2017;32:49-56.
90. Rodríguez-Rodríguez N, Madera-Salcedo IK, Bugarin-Estrada E, et al. The helminth-derived peptide GK-1 induces an anti-tumoral CD8 T cell response associated with downregulation of the PD-1/PD-L1 pathway. Clin Immunol. 2020;212:108240.
91. Liu H, Weber A, Morse J, et al. T cell mediated immunity after combination therapy with intralesional PV-10 and blockade of the PD-1/PD-L1 pathway in a murine melanoma model. PLoS One. 2018;13:e0196033.
92. Shi Y, Li D, He C, Chen X. Design of an injectable polypeptide hydrogel depot containing the immune checkpoint blocker anti-PD-L1 and doxorubicin to enhance antitumor combination therapy. Macromol Biosci. 2021;21:e2100049.
93. Zhao J, Ye H, Lu Q, et al. Inhibition of post-surgery tumour recurrence via a sprayable chemo-immunotherapy gel releasing PD-L1 antibody and platelet-derived small EVs. J Nanobiotechnol. 2022;20:62.
94. Wang X, Si L, Guo J. Treatment algorithm of metastatic mucosal melanoma. Chin Clin Oncol. 2014;3:38.
96. Yu P, Wei H, Li K, et al. The traditional chinese medicine monomer Ailanthone improves the therapeutic efficacy of anti-PD-L1 in melanoma cells by targeting c-Jun. J Exp Clin Cancer Res. 2022;41:346.
97. Yazdani M, Hatamipour M, Alani B, et al. Liposomal gp100 vaccine combined with CpG ODN sensitizes established B16F10 melanoma tumors to anti PD-1 therapy. Iran J Basic Med Sci. 2020;23:1065-77.
98. Chowdhury PS, Chamoto K, Honjo T. Combination therapy strategies for improving PD-1 blockade efficacy: a new era in cancer immunotherapy. J Intern Med. 2018;283:110-20.
99. Tian H, Shi G, Wang Q, et al. A novel cancer vaccine with the ability to simultaneously produce anti-PD-1 antibody and GM-CSF in cancer cells and enhance Th1-biased antitumor immunity. Signal Transduct Target Ther. 2016;1:16025.
100. Cheng B, Ren Y, Niu X, et al. Discovery of novel resorcinol dibenzyl ethers targeting the programmed cell death-1/programmed cell death-ligand 1 interaction as potential anticancer agents. J Med Chem. 2020;63:8338-58.
101. Frydenlund N, Leone D, Yang S, et al. Tumoral PD-L1 expression in desmoplastic melanoma is associated with depth of invasion, tumor-infiltrating CD8 cytotoxic lymphocytes and the mixed cytomorphological variant. Mod Pathol. 2017;30:357-69.
102. Brahmer JR, Drake CG, Wollner I, et al. Phase I study of single-agent anti-programmed death-1 (MDX-1106) in refractory solid tumors: safety, clinical activity, pharmacodynamics, and immunologic correlates. J Clin Oncol. 2010;28:3167-75.
103. Azadnajafabad S, Saeedi Moghaddam S, Mohammadi E, et al. Burden of breast cancer and attributable risk factors in the North Africa and Middle East region, 1990-2019: a systematic analysis for the global burden of disease study 2019. Front Oncol. 2023;13:1132816.
104. Hanamura T, Kitano S, Kagamu H, et al. Immunological profiles of the breast cancer microenvironment represented by tumor-infiltrating lymphocytes and PD-L1 expression. Sci Rep. 2022;12:8098.
105. Shi F, Chang H, Zhou Q, Zhao YJ, Wu GJ, Song QK. Distribution of CD4+ and CD8+ exhausted tumor-infiltrating lymphocytes in molecular subtypes of Chinese breast cancer patients. Onco Targets Ther. 2018;11:6139-45.
106. Zhang J, Zhang H, Luo Y. Association between activation of the programmed cell death-1 (PD-1)/programmed death-ligand 1 (PD-L1) pathway and pain in patients with cancer. Med Sci Monit. 2019;25:1275-82.
107. Juliá EP, Amante A, Pampena MB, Mordoh J, Levy EM. Avelumab, an IgG1 anti-PD-L1 immune checkpoint inhibitor, triggers NK cell-mediated cytotoxicity and cytokine production against triple negative breast cancer cells. Front Immunol. 2018;9:2140.
108. Lamberti G, Sisi M, Andrini E, et al. The mechanisms of PD-L1 regulation in non-small-cell lung cancer (NSCLC): which are the involved players? Cancers. 2020;12:3129.
109. Florent L, Saby C, Slimano F, Morjani H. BRAF V600-mutated metastatic melanoma and targeted therapy resistance: an update of the current knowledge. Cancers. 2023;15:2607.
110. Ofori S, Awuah SG. Small-molecule poly(ADP-ribose) polymerase and PD-L1 inhibitor conjugates as dual-action anticancer agents. ACS Omega. 2019;4:12584-97.
111. Tie Y, Yang H, Zhao R, et al. Safety and efficacy of atezolizumab in the treatment of cancers: a systematic review and pooled-analysis. Drug Des Devel Ther. 2019;13:523-38.
112. Krämer S, Rogmans C, Saylan D, et al. Treatment progress in triple negative breast cancer. Eur J Gynaecol Oncol. 2022;43:341-52.
113. Luo X, Wang H, Ji D. Carbon nanotubes (CNT)-loaded ginsenosides Rb3 suppresses the PD-1/PD-L1 pathway in triple-negative breast cancer. Aging. 2021;13:17177-89.
114. Zhao W, Hu X, Li W, et al. M2-like TAMs function reversal contributes to breast cancer eradication by combination dual immune checkpoint blockade and photothermal therapy. Small. 2021;17:e2007051.
115. Zhang R, Zhu Z, Lv H, et al. Immune checkpoint blockade mediated by a small-molecule nanoinhibitor targeting the PD-1/PD-L1 pathway synergizes with photodynamic therapy to elicit antitumor immunity and antimetastatic effects on breast cancer. Small. 2019;15:e1903881.
116. Mirzaei-Parsa MJ, Najafabadi MRH, Haeri A, et al. Preparation, characterization, and evaluation of the anticancer activity of artemether-loaded nano-niosomes against breast cancer. Breast Cancer. 2020;27:243-51.
117. Chen Z, Hu K, Feng L, et al. Senescent cells re-engineered to express soluble programmed death receptor-1 for inhibiting programmed death receptor-1/programmed death ligand-1 as a vaccination approach against breast cancer. Cancer Sci. 2018;109:1753-63.
118. Ge Y, Xi H, Ju S, Zhang X. Blockade of PD-1/PD-L1 immune checkpoint during DC vaccination induces potent protective immunity against breast cancer in hu-SCID mice. Cancer Lett. 2013;336:253-9.
119. Chen LM, Yang PP, Al Haq AT, et al. Oligo-fucoidan supplementation enhances the effect of olaparib on preventing metastasis and recurrence of triple-negative breast cancer in mice. J Biomed Sci. 2022;29:70.
120. Salatino M, Girotti MR, Rabinovich GA. Glycans pave the way for immunotherapy in triple-negative breast cancer. Cancer Cell. 2018;33:155-7.
121. Liang M, Fu J. Triptolide inhibits interferon-gamma-induced programmed death-1-ligand 1 surface expression in breast cancer cells. Cancer Lett. 2008;270:337-41.
122. Zhou Y, Yamamoto Y, Takeshita F, Yamamoto T, Xiao Z, Ochiya T. Delivery of miR-424-5p via extracellular vesicles promotes the apoptosis of MDA-MB-231 TNBC cells in the tumor microenvironment. Int J Mol Sci. 2021;22:844.
123. Fertal SA, Poterala JE, Ponik SM, Wisinski KB. Stromal characteristics and impact on new therapies for metastatic triple-negative breast cancer. Cancers. 2022;14:1238.
124. Konstantinopoulos PA, Waggoner S, Vidal GA, et al. Single-arm phases 1 and 2 trial of niraparib in combination with pembrolizumab in patients with recurrent platinum-resistant ovarian carcinoma. JAMA Oncol. 2019;5:1141-9.
125. Antony GR, Augustine P, Parambil ST, et al. Immunohistochemical expression of PD-L1 and MDR1 in breast tumors: association with clinico-pathological parameters and treatment outcome. Clin Exp Med. 2023;23:859-69.
126. Afkhami M, Schmolze D, Yost SE, et al. Mutation and immune profiling of metaplastic breast cancer: correlation with survival. PLoS One. 2019;14:e0224726.
127. AiErken N, Shi HJ, Zhou Y, et al. High PD-L1 expression is closely associated with tumor-infiltrating lymphocytes and leads to good clinical outcomes in Chinese triple negative breast cancer patients. Int J Biol Sci. 2017;13:1172-9.
128. Ali HR, Glont SE, Blows FM, et al. PD-L1 protein expression in breast cancer is rare, enriched in basal-like tumours and associated with infiltrating lymphocytes. Ann Oncol. 2015;26:1488-93.
129. Andrieu GP, Shafran JS, Smith CL, et al. BET protein targeting suppresses the PD-1/PD-L1 pathway in triple-negative breast cancer and elicits anti-tumor immune response. Cancer Lett. 2019;465:45-58.
130. Alkaabi D, Arafat K, Sulaiman S, Al-Azawi AM, Attoub S. PD-1 independent role of PD-L1 in triple-negative breast cancer progression. Int J Mol Sci. 2023;24:6420.
131. Llovet JM, Kelley RK, Villanueva A, et al. Hepatocellular carcinoma. Nat Rev Dis Primers. 2021;7:6.
132. Chen Z, Chen Y, Peng L, Wang X, Tang N. 2,5-dimethylcelecoxib improves immune microenvironment of hepatocellular carcinoma by promoting ubiquitination of HBx-induced PD-L1. J Immunother Cancer. 2020;8:e001377.
133. Peña-Asensio J, Calvo H, Torralba M, Miquel J, Sanz-de-Villalobos E, Larrubia JR. Anti-PD-1/PD-L1 based combination immunotherapy to boost antigen-specific CD8+ T cell response in hepatocellular carcinoma. Cancers. 2021;13:1922.
134. Pan Z, Di S, Shi B, et al. Increased antitumor activities of glypican-3-specific chimeric antigen receptor-modified T cells by coexpression of a soluble PD1-CH3 fusion protein. Cancer Immunol Immunother. 2018;67:1621-34.
135. Sawada Y, Yoshikawa T, Shimomura M, Iwama T, Endo I, Nakatsura T. Programmed death-1 blockade enhances the antitumor effects of peptide vaccine-induced peptide-specific cytotoxic T lymphocytes. Int J Oncol. 2015;46:28-36.
136. Shi F, Shi M, Zeng Z, et al. PD-1 and PD-L1 upregulation promotes CD8+ T-cell apoptosis and postoperative recurrence in hepatocellular carcinoma patients. Int J Cancer. 2011;128:887-96.
137. Zeng Z, Shi F, Zhou L, et al. Upregulation of circulating PD-L1/PD-1 is associated with poor post-cryoablation prognosis in patients with HBV-related hepatocellular carcinoma. PLoS One. 2011;6:e23621.
138. An J, Kang HJ, Yu E, Lee HC, Shim JH. The effects of immune checkpoint modulators on the clinical course of patients with resectable hepatocellular carcinoma. J Liver Cancer. 2022;22:40-50.
139. Wu M, Xia X, Hu J, Fowlkes NW, Li S. WSX1 act as a tumor suppressor in hepatocellular carcinoma by downregulating neoplastic PD-L1 expression. Nat Commun. 2021;12:3500.
140. Ajami M, Davoodi SH, Habibey R, Namazi N, Soleimani M, Pazoki-Toroudi H. Effect of DHA+EPA on oxidative stress and apoptosis induced by ischemia-reperfusion in rat kidneys. Fundam Clin Pharmacol. 2013;27:593-602.
141. Ajami M, Eghtesadi S, Razaz JM, et al. Expression of Bcl-2 and Bax after hippocampal ischemia in DHA + EPA treated rats. Neurol Sci. 2011;32:811-8.
142. Zarch AV, Toroudi HP, Soleimani M, Bakhtiarian A, Katebi M, Djahanguiri B. Neuroprotective effects of diazoxide and its antagonism by glibenclamide in pyramidal neurons of rat hippocampus subjected to ischemia-reperfusion-induced injury. Int J Neurosci. 2009;119:1346-61.
143. Yang H, Zhou X, Sun L, Mao Y. Correlation between PD-L2 expression and clinical outcome in solid cancer patients: a meta-analysis. Front Oncol. 2019;9:47.
144. Ahmed Z, Lee SS, Victor DW, Kodali S. Evidence to date: clinical utility of tremelimumab in the treatment of unresectable hepatocellular carcinoma. J Hepatocell Carcinoma. 2023;10:1911-22.
145. Tattersall A, Ryan N, Wiggans AJ, Rogozińska E, Morrison J. Poly(ADP-ribose) polymerase (PARP) inhibitors for the treatment of ovarian cancer. Cochrane Database Syst Rev. 2022;2:CD007929.
146. Hao L, Guo Y, Peng Q, et al. Dihydroartemisinin reduced lipid droplet deposition by YAP1 to promote the anti-PD-1 effect in hepatocellular carcinoma. Phytomedicine. 2022;96:153913.
147. Lv T, Xiong X, Yan W, Liu M, Xu H, He Q. Targeting of GSDMD sensitizes HCC to anti-PD-1 by activating cGAS pathway and downregulating PD-L1 expression. J Immunother Cancer. 2022;10:e004763.
148. Toroudi HP, Rahgozar M, Bakhtiarian A, Djahanguiri B. Potassium channel modulators and indomethacin-induced gastric ulceration in rats. Scand J Gastroenterol. 1999;34:962-6.
149. Rahgozar M, Pazokitoroudi H, Bakhtiarian A, Djahanguiri B. Diazoxide, a K(ATP) opener, accelerates restitution of ethanol or indomethacin-induced gastric ulceration in rats independent of polyamines. J Gastroenterol Hepatol. 2001;16:290-6.
150. O'Shea JJ, Schwartz DM, Villarino AV, Gadina M, McInnes IB, Laurence A. The JAK-STAT pathway: impact on human disease and therapeutic intervention. Annu Rev Med. 2015;66:311-28.
151. Tümen D, Heumann P, Gülow K, et al. Pathogenesis and current treatment strategies of hepatocellular carcinoma. Biomedicines. 2022;10:3202.
152. Hammarén HM, Virtanen AT, Raivola J, Silvennoinen O. The regulation of JAKs in cytokine signaling and its breakdown in disease. Cytokine. 2019;118:48-63.
153. Guangshun S, Guoqiang S, Xin C, et al. Meloxicam inhibits hepatocellular carcinoma progression and enhances the sensitivity of immunotherapy via the MicroRNA-200/PD-L1 pathway. J Oncol. 2022;2022:4598573.
154. Starley BQ, Calcagno CJ, Harrison SA. Nonalcoholic fatty liver disease and hepatocellular carcinoma: a weighty connection. Hepatology. 2010;51:1820-32.
155. Xing R, Gao J, Cui Q, Wang Q. Strategies to improve the antitumor effect of immunotherapy for hepatocellular carcinoma. Front Immunol. 2021;12:783236.
156. Giglia JL, Antonia SJ, Berk LB, Bruno S, Dessureault S, Finkelstein SE. Systemic therapy for advanced hepatocellular carcinoma: past, present, and future. Cancer Control. 2010;17:120-9.
157. Combalia A, Carrera C. Squamous cell carcinoma: an update on diagnosis and treatment. Dermatol Pract Concept. 2020;10:e2020066.
158. Kujan O, Agag M, Smaga M, et al. PD-1/PD-L1, Treg-related proteins, and tumour-infiltrating lymphocytes are associated with the development of oral squamous cell carcinoma. Pathology. 2022;54:409-16.
159. Takada K, Okamoto T, Toyokawa G, et al. The expression of PD-L1 protein as a prognostic factor in lung squamous cell carcinoma. Lung Cancer. 2017;104:7-15.
160. Liu Z, Williams M, Stewart J, Glisson BS, Fuller C, Roy-Chowdhuri S. Evaluation of programmed death ligand 1 expression in cytology to determine eligibility for immune checkpoint inhibitor therapy in patients with head and neck squamous cell carcinoma. Cancer Cytopathol. 2022;130:110-9.
161. Okada S, Itoh K, Ishihara S, et al. Significance of PD-L1 expression in pulmonary metastases from head and neck squamous cell carcinoma. Surg Oncol. 2018;27:259-65.
162. Troeltzsch M, Woodlock T, Pianka A, et al. Is there evidence for the presence and relevance of the PD-1/PD-L1 pathway in oral squamous cell carcinoma? Hints from an immunohistochemical study. J Oral Maxillofac Surg. 2017;75:969-77.
163. Monteiro de Oliveira Novaes JA, Hirz T, Guijarro I, et al. Targeting of CD40 and PD-L1 pathways inhibits progression of oral premalignant lesions in a carcinogen-induced model of oral squamous cell carcinoma. Cancer Prev Res. 2021;14:313-24.
164. Akisada N, Nishimoto K, Takao S, et al. PD-L1 expression in tongue squamous cell carcinoma. Med Mol Morphol. 2021;54:52-9.
165. Okadome K, Baba Y, Nomoto D, et al. Prognostic and clinical impact of PD-L2 and PD-L1 expression in a cohort of 437 oesophageal cancers. Br J Cancer. 2020;122:1535-43.
166. Guo W, Wang P, Li N, et al. Prognostic value of PD-L1 in esophageal squamous cell carcinoma: a meta-analysis. Oncotarget. 2018;9:13920-33.
167. Cocks M, Chaux A, Jenson EG, et al. Immune checkpoint status and tumor microenvironment in vulvar squamous cell carcinoma. Virchows Arch. 2020;477:93-102.
168. Curley J, Conaway MR, Chinn Z, Duska L, Stoler M, Mills AM. Looking past PD-L1: expression of immune checkpoint TIM-3 and its ligand galectin-9 in cervical and vulvar squamous neoplasia. Mod Pathol. 2020;33:1182-92.
169. Heeren AM, Punt S, Bleeker MC, et al. Prognostic effect of different PD-L1 expression patterns in squamous cell carcinoma and adenocarcinoma of the cervix. Mod Pathol. 2016;29:753-63.
170. Yang W, Lu YP, Yang YZ, Kang JR, Jin YD, Wang HW. Expressions of programmed death (PD)-1 and PD-1 ligand (PD-L1) in cervical intraepithelial neoplasia and cervical squamous cell carcinomas are of prognostic value and associated with human papillomavirus status. J Obstet Gynaecol Res. 2017;43:1602-12.
171. Reddy OL, Shintaku PI, Moatamed NA. Programmed death-ligand 1 (PD-L1) is expressed in a significant number of the uterine cervical carcinomas. Diagn Pathol. 2017;12:45.
172. Liang Y, Yu M, Zhou C, Zhu X. Variation of PD-L1 expression in locally advanced cervical cancer following neoadjuvant chemotherapy. Diagn Pathol. 2020;15:67.
173. Müller T, Braun M, Dietrich D, et al. PD-L1: a novel prognostic biomarker in head and neck squamous cell carcinoma. Oncotarget. 2017;8:52889-900.
174. Qiao XW, Jiang J, Pang X, et al. The evolving landscape of PD-1/PD-L1 pathway in head and neck cancer. Front Immunol. 2020;11:1721.
175. Affolter A, Liebel K, Tengler L, et al. Modulation of PD-L1 expression by standard therapy in head and neck cancer cell lines and exosomes. Int J Oncol. 2023;63:102.
176. Zhang Z, Wu B, Peng G, et al. Neoadjuvant chemoimmunotherapy for the treatment of locally advanced head and neck squamous cell carcinoma: a single-arm phase 2 clinical trial. Clin Cancer Res. 2022;28:3268-76.
177. Wu D, Li Y, Xu P, et al. Neoadjuvant chemo-immunotherapy with camrelizumab plus nab-paclitaxel and cisplatin in resectable locally advanced squamous cell carcinoma of the head and neck: a pilot phase II trial. Nat Commun. 2024;15:2177.
178. Huang X, Liu Q, Zhong G, et al. Neoadjuvant toripalimab combined with gemcitabine and cisplatin in resectable locally advanced head and neck squamous cell carcinoma (NeoTGP01): an open label, single-arm, phase Ib clinical trial. J Exp Clin Cancer Res. 2022;41:300.
179. Li X, Fang Q, Du W, Zhang X, Dai L, Qiao Y. Induction chemotherapy combined with immunotherapy in locally advanced head and neck squamous cell carcinoma. BMC Cancer. 2021;21:622.
180. Kim MY, Koh J, Kim S, Go H, Jeon YK, Chung DH. Clinicopathological analysis of PD-L1 and PD-L2 expression in pulmonary squamous cell carcinoma: comparison with tumor-infiltrating T cells and the status of oncogenic drivers. Lung Cancer. 2015;88:24-33.
181. Quan H, Yan L, Wang S, Wang S. Clinical relevance and significance of programmed death-ligand 1 expression, tumor-infiltrating lymphocytes, and p16 status in sinonasal squamous cell carcinoma. Cancer Manag Res. 2019;11:4335-45.
182. Bahig H, Aubin F, Stagg J, et al. Phase I/II trial of Durvalumab plus tremelimumab and stereotactic body radiotherapy for metastatic head and neck carcinoma. BMC Cancer. 2019;19:68.
183. Cavalieri S, Perrone F, Milione M, et al. PD-L1 expression in unresectable locally advanced or metastatic skin squamous cell carcinoma treated with anti-epidermal growth factor receptor agents. Oncology. 2019;97:112-8.
184. Yanik EL, Kaunitz GJ, Cottrell TR, et al. Association of HIV status with local immune response to anal squamous cell carcinoma: implications for immunotherapy. JAMA Oncol. 2017;3:974-8.
185. Hira-Miyazawa M, Nakamura H, Hirai M, et al. Regulation of programmed-death ligand in the human head and neck squamous cell carcinoma microenvironment is mediated through matrix metalloproteinase-mediated proteolytic cleavage. Int J Oncol. 2018;52:379-88.
186. Yaghoubi N, Soltani A, Ghazvini K, Hassanian SM, Hashemy SI. PD-1/PD-L1 blockade as a novel treatment for colorectal cancer. Biomed Pharmacother. 2019;110:312-8.
187. Jahangiri A, Dadmanesh M, Ghorban K. STAT3 inhibition reduced PD-L1 expression and enhanced antitumor immune responses. J Cell Physiol. 2020;235:9457-63.
188. Li Y, He M, Zhou Y, et al. The prognostic and clinicopathological roles of PD-L1 expression in colorectal cancer: a systematic review and meta-analysis. Front Pharmacol. 2019;10:139.
189. Hamada T, Cao Y, Qian ZR, et al. Aspirin use and colorectal cancer survival according to tumor CD274 (programmed cell death 1 ligand 1) expression status. J Clin Oncol. 2017;35:1836-44.
190. Inaguma S, Lasota J, Felisiak-Golabek A, et al. Histopathological and genotypic characterization of metastatic colorectal carcinoma with PD-L1 (CD274)-expression: possible roles of tumour micro environmental factors for CD274 expression. J Pathol Clin Res. 2017;3:268-78.
191. Masugi Y, Nishihara R, Yang J, et al. Tumour CD274 (PD-L1) expression and T cells in colorectal cancer. Gut. 2017;66:1463-73.
192. Gao CE, Zhang M, Song Q, Dong J. PD-1 inhibitors dependent CD8+ T cells inhibit mouse colon cancer cell metastasis. Onco Targets Ther. 2019;12:6961-71.
193. Bray F, Ferlay J, Soerjomataram I, Siegel RL, Torre LA, Jemal A. Global cancer statistics 2018: GLOBOCAN estimates of incidence and mortality worldwide for 36 cancers in 185 countries. CA Cancer J Clin. 2018;68:394-424.
195. van der Heijden MS, Sonpavde G, Powles T, et al. Nivolumab plus gemcitabine-cisplatin in advanced urothelial carcinoma. N Engl J Med. 2023;389:1778-89.
196. Cathomas R, Rothschild SI, Hayoz S, et al. Perioperative chemoimmunotherapy with durvalumab for muscle-invasive urothelial carcinoma: primary analysis of the single-arm phase II trial SAKK 06/17. J Clin Oncol. 2023;41:5131-9.
197. Huang YD, Zhang SD, McCrudden C, Chan KW, Lin Y, Kwok HF. The prognostic significance of PD-L1 in bladder cancer. Oncol Rep. 2015;33:3075-84.
198. Butte MJ, Peña-Cruz V, Kim MJ, Freeman GJ, Sharpe AH. Interaction of human PD-L1 and B7-1. Mol Immunol. 2008;45:3567-72.
199. Donin NM, Lenis AT, Holden S, et al. Immunotherapy for the treatment of urothelial carcinoma. J Urol. 2017;197:14-22.
200. Kaufman HL, Russell J, Hamid O, et al. Avelumab in patients with chemotherapy-refractory metastatic Merkel cell carcinoma: a multicentre, single-group, open-label, phase 2 trial. Lancet Oncol. 2016;17:1374-85.
201. Atkins MB, Plimack ER, Puzanov I, et al. Axitinib in combination with pembrolizumab in patients with advanced renal cell cancer: a non-randomised, open-label, dose-finding, and dose-expansion phase 1b trial. Lancet Oncol. 2018;19:405-15.
202. Lipson EJ, Vincent JG, Loyo M, et al. PD-L1 expression in the Merkel cell carcinoma microenvironment: association with inflammation, Merkel cell polyomavirus and overall survival. Cancer Immunol Res. 2013;1:54-63.
203. Ni J, Si X, Wang H, Zhang X, Zhang L. Camrelizumab plus platinum-irinotecan followed by maintenance camrelizumab plus apatinib in untreated extensive-stage small-cell lung cancer: a nonrandomized clinical trial. Front Immunol. 2023;14:1168879.
204. Patil VM, Noronha V, Menon N, et al. Results of phase III randomized trial for use of docetaxel as a radiosensitizer in patients with head and neck cancer, unsuitable for cisplatin-based chemoradiation. J Clin Oncol. 2023;41:2350-61.
205. O'Shea AE, Clifton GT, Qiao N, et al. Phase II trial of nelipepimut-S peptide vaccine in women with ductal carcinoma in situ. Cancer Prev Res. 2023;16:333-41.
206. Li H, Huang Y, Chen Z, et al. Efficacy and safety of denosumab biosimilar QL1206 versus denosumab in patients with bone metastases from solid tumors: a randomized phase III trial. BioDrugs. 2023;37:259-69.
207. Penson RT, Ambrosio AJ, Whalen CA, et al. Phase II trials of iniparib (BSI-201) in combination with gemcitabine and carboplatin in patients with recurrent ovarian cancer. Oncologist. 2023;28:252-7.
208. Zheng R, Wang BS, Li Z, Chi P, Xu B. Combining chemotherapy and tislelizumab with preoperative split-course hypofraction radiotherapy for locally advanced rectal cancer: study protocol of a prospective, single-arm, phase II trial. BMJ Open. 2023;13:e066976.
209. Topalian SL, Hodi FS, Brahmer JR, et al. Safety, activity, and immune correlates of anti-PD-1 antibody in cancer. N Engl J Med. 2012;366:2443-54.
210. Kato T, Masuda N, Nakanishi Y, et al. Nivolumab-induced interstitial lung disease analysis of two phase II studies patients with recurrent or advanced non-small-cell lung cancer. Lung Cancer. 2017;104:111-8.
211. Jin Y, Dong H, Xia L, et al. The diversity of gut microbiome is associated with favorable responses to anti-programmed death 1 immunotherapy in Chinese patients with NSCLC. J Thorac Oncol. 2019;14:1378-89.
212. Ochi N, Ichihara E, Takigawa N, et al. The effects of antibiotics on the efficacy of immune checkpoint inhibitors in patients with non-small-cell lung cancer differ based on PD-L1 expression. Eur J Cancer. 2021;149:73-81.
213. Sun LY, Cen WJ, Tang WT, et al. Smoking status combined with tumor mutational burden as a prognosis predictor for combination immune checkpoint inhibitor therapy in non-small cell lung cancer. Cancer Med. 2021;10:6610-7.
214. Zheng Y, Wang T, Tu X, et al. Gut microbiome affects the response to anti-PD-1 immunotherapy in patients with hepatocellular carcinoma. J Immunother Cancer. 2019;7:193.
215. Gopalakrishnan V, Spencer CN, Nezi L, et al. Gut microbiome modulates response to anti-PD-1 immunotherapy in melanoma patients. Science. 2018;359:97-103.
216. Davar D, Dzutsev AK, McCulloch JA, et al. Fecal microbiota transplant overcomes resistance to anti-PD-1 therapy in melanoma patients. Science. 2021;371:595-602.
217. Baruch EN, Youngster I, Ben-Betzalel G, et al. Fecal microbiota transplant promotes response in immunotherapy-refractory melanoma patients. Science. 2021;371:602-9.
218. Dong M, Meng Z, Kuerban K, et al. Diosgenin promotes antitumor immunity and PD-1 antibody efficacy against melanoma by regulating intestinal microbiota. Cell Death Dis. 2018;9:1039.
221. Nomura M, Nagatomo R, Doi K, et al. Association of short-chain fatty acids in the gut microbiome with clinical response to treatment with nivolumab or pembrolizumab in patients with solid cancer tumors. JAMA Netw Open. 2020;3:e202895.
222. Humphries A, Daud A. The gut microbiota and immune checkpoint inhibitors. Hum Vaccin Immunother. 2018;14:2178-82.
223. Zhang C, Yue C, Herrmann A, et al. STAT3 activation-induced fatty acid oxidation in CD8+ T effector cells is critical for obesity-promoted breast tumor growth. Cell Metab. 2020;31:148-61.e5.
224. Pingili AK, Chaib M, Sipe LM, et al. Immune checkpoint blockade reprograms systemic immune landscape and tumor microenvironment in obesity-associated breast cancer. Cell Rep. 2021;35:109285.
225. Eden D, Ghose A, Moschetta M, Pérez-Fidalgo JA, Rassy E, Boussios S. Immunotherapy combined with standard therapies in head and neck squamous cell carcinoma - a meta-analysis. Anticancer Res. 2024;44:861-78.
226. Rittmeyer A, Barlesi F, Waterkamp D, et al. Atezolizumab versus docetaxel in patients with previously treated non-small-cell lung cancer (OAK): a phase 3, open-label, multicentre randomised controlled trial. Lancet. 2017;389:255-65.
227. Nomura S, Goto Y, Mizutani T, et al. A randomized phase III study comparing continuation and discontinuation of PD-1 pathway inhibitors for patients with advanced non-small-cell lung cancer (JCOG1701, SAVE study). Jpn J Clin Oncol. 2020;50:821-5.
228. Khan U, Ali F, Khurram MS, Zaka A, Hadid T. Immunotherapy-associated autoimmune hemolytic anemia. J Immunother Cancer. 2017;5:15.
229. Kunimasa K, Isei T, Nakamura H, et al. Proliferative CD8+ PD-1+ T-cell infiltration in a pembrolizumab-induced cutaneous adverse reaction. Invest New Drugs. 2018;36:1138-42.
230. Zahoor F, Ahmed N, Afzal G. Onychopathy induced by nivolumab: a targeted immunotherapy. Cureus. 2022;14:e26950.
231. Cubillos-Zapata C, Martínez-García MÁ, Campos-Rodríguez F, et al. Soluble PD-L1 is a potential biomarker of cutaneous melanoma aggressiveness and metastasis in obstructive sleep apnoea patients. Eur Respir J. 2019;53:1801298.
232. Huang X, Zhang J, Li X, et al. Rescue of iCIKs transfer from PD-1/PD-L1 immune inhibition in patients with resectable tongue squamous cell carcinoma (TSCC). Int Immunopharmacol. 2018;59:127-33.
233. Stenmark Tullberg A, Sjöström M, Tran L, et al. Combining histological grade, TILs, and the PD-1/PD-L1 pathway to identify immunogenic tumors and de-escalate radiotherapy in early breast cancer: a secondary analysis of a randomized clinical trial. J Immunother Cancer. 2023;11:e006618.
234. Eichhorn F, Klotz LV, Bischoff H, et al. Neoadjuvant anti-programmed Death-1 immunotherapy by Pembrolizumab in resectable nodal positive stage II/IIIa non-small-cell lung cancer (NSCLC): the NEOMUN trial. BMC Cancer. 2019;19:413.
235. Piersiala K, Farrajota Neves da Silva P, Hjalmarsson E, et al. CD4+ and CD8+ T cells in sentinel nodes exhibit distinct pattern of
236. Miyoshi Y, Ogawa O, Oyama Y. Nivolumab, an anti-programmed cell death-1 antibody, induces fulminant type 1 diabetes. Tohoku J Exp Med. 2016;239:155-8.
237. Vienot A, Jacquin M, Rebucci-Peixoto M, et al. Evaluation of the interest to combine a CD4 Th1-inducer cancer vaccine derived from telomerase and atezolizumab plus bevacizumab in unresectable hepatocellular carcinoma: a randomized non-comparative phase II study (TERTIO - PRODIGE 82). BMC Cancer. 2023;23:710.
238. Yun SJ, Oh IJ, Park CK, et al. Vitiligo-like depigmentation after pembrolizumab treatment in patients with non-small cell lung cancer: a case report. Transl Lung Cancer Res. 2020;9:1585-90.
239. Kunimasa K, Nishino K, Kimura M, et al. Pembrolizumab-induced acute thrombosis: a case report. Medicine. 2018;97:e10772.
240. Cui G, Qu D, Bai Y, Sun X, Li Y, Yang Y. Postoperative pathological complete response in a patient with PD-L1-negative stage IIIB lung squamous cell carcinoma following neoadjuvant tislelizumab treatment combined with chemotherapy: a case report and literature review. Oncol Lett. 2023;26:388.
241. Merhi M, Raza A, Inchakalody VP, et al. Squamous cell carcinomas of the head and neck cancer response to programmed cell death protein-1 targeting and differential expression of immunological markers: a case report. Front Immunol. 2018;9:1769.
242. Ribas A, Hamid O, Daud A, et al. Association of pembrolizumab with tumor response and survival among patients with advanced melanoma. JAMA. 2016;315:1600-9.
243. Pazoki-Toroudi HR, Ajami M, Habibey R. Pre-medication and renal pre-conditioning: a role for alprazolam, atropine, morphine and promethazine. Fundam Clin Pharmacol. 2010;24:189-98.
244. Habibey R, Pazoki-Toroudi H. Morphine dependence protects rat kidney against ischaemia-reperfusion injury. Clin Exp Pharmacol Physiol. 2008;35:1209-14.
Cite This Article

How to Cite
Download Citation
Export Citation File:
Type of Import
Tips on Downloading Citation
Citation Manager File Format
Type of Import
Direct Import: When the Direct Import option is selected (the default state), a dialogue box will give you the option to Save or Open the downloaded citation data. Choosing Open will either launch your citation manager or give you a choice of applications with which to use the metadata. The Save option saves the file locally for later use.
Indirect Import: When the Indirect Import option is selected, the metadata is displayed and may be copied and pasted as needed.
About This Article
Copyright
Data & Comments
Data
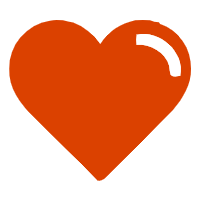
Comments
Comments must be written in English. Spam, offensive content, impersonation, and private information will not be permitted. If any comment is reported and identified as inappropriate content by OAE staff, the comment will be removed without notice. If you have any queries or need any help, please contact us at [email protected].