The role of melatonin as an adjuvant therapeutic strategy in the modulation of carcinogenesis. A narrative review
Abstract
Melatonin (N-acetyl-5 methoxytryptamine) is an indolic compound present in almost all fungi, plants, and animals. This neurohormone is synthesized and secreted into the internal environment mainly by the pineal gland, present in most vertebrates. Non-endocrine extrapineal locations have not been documented. This molecule with pleiotropic bioactions regulates the circadian rhythm, antioxidant, anti-inflammatory, immunostimulant, cardioprotective, antidiabetic, antiobesity, neuroprotective, and antiaging actions. Furthermore, in recent years, many studies have described the key role of melatonin in the prevention and development of cancer. The objective of this narrative review is to describe the different mechanisms through which melatonin exerts its action as an adjuvant in the modulation of carcinogenesis. The general anticarcinogenic mechanisms include epigenetic control, modulation of cell proliferation, regulation of cell cycle, induction of apoptosis, and telomerase inhibition. Melatonin also exerts antiestrogenic activity, which is particularly significant in hormone-dependent tumors, regulating the expression and transactivation of the estrogen receptor, and modulating the enzymes involved in the local synthesis of estrogens. Modulation of metastasis by melatonin includes increased expression of cell adhesion molecules such as E-cadherin and β1-integrin, inhibition of angiogenesis, and control of fat metabolism by inhibiting the uptake of fatty acids by membrane transporters. Finally, immunomodulatory properties include enhanced production of anti-inflammatory interleukins and other cytokines in lymphocytes and monocytes and modulation of antioxidant activity by neutralizing free radicals. Despite all the mentioned properties, the use of melatonin in daily clinical practice is very limited, and additional studies are needed to better establish the role of this hormone in oncological clinical applications against different types of cancer.
Keywords
INTRODUCTION
Melatonin (N-acetyl-5 methoxy-tryptamine; C13H16N2O2) is an indolic compound that is present in almost all species, such as fungi, plants, and animals, which was isolated for the first time in 1958 by Aaron Lerner[1]. The neurohormone melatonin is synthesized and secreted into the internal environment mainly by the pineal gland, a neuroendocrine photosensor that receives cyclic light stimulus and transforms it into humoral signals[2]. Melatonin modulates a variety of physiological functions, such as the regulation of sleep/wake and circadian rhythm. In addition, it exhibits neuro- and cardioprotective properties, along with antitumor and anti-aging effects, and enhances mitochondrial activity. All these properties are attributed to its antioxidant, anti-inflammatory, and antitumoral effects[3,4]. Altogether, this suggests that melatonin plays a crucial role in the etiopathogenic process of aging-related chronic non-communicable diseases (NCDs)[5].
Biosynthesis
The night (darkness)-day (light) cycle stimulates neuronal signals that connect the retina and the central nervous system (CNS) with the pineal gland. Progression to obscurity stimulates the release of norepinephrine from the nerve endings, which induces the transformation of serotonin into melatonin[6]. This biosynthetic and secretory process occurs in the specialized cells of the pineal gland (located in the brain behind the third ventricle) and the pinealocytes, increasing melatonin levels in the blood and cerebrospinal fluid[1]. Melatonin is an indolic compound with lipophilic properties derived from the amino acid tryptophan, which is taken up by pinealocytes from the peripheral circulation and transformed into melatonin through the action of four participating enzymes [Figure 1]; the non-enzymatic transformation of melatonin is still deficient. Enzymatic activity is regulated by the neuro-endocrine system following the circadian rhythm that responds to the light/dark cycle[1]. Therefore, melatonin has a production rate proportional to the nocturnal noradrenergic stimulus, with minimum daytime and maximum nighttime values of up to 80%, and biosynthesis can be altered by artificial light[7].
Figure 1. Biosynthetic pathway of melatonin synthesis. TPH: Tryptophan hydroxylase; AAAD: aromatic amino acid decarboxylase; SNAT: serotonin N-acetyltransferase; ASMT: N-acetyl-serotonin O-methyltransferase.
Melatonin is also synthesized alternatively in different organs and its effect does not have a specific target tissue[4]. The extrapineal biosynthetic locations include the retina, harderian gland (complementary to the lacrimal), bone marrow, skin, cerebellum, cells of the immune system (lymphocytes), and particularly cells of the gastrointestinal tract that produce serotonin. In this context, gastrointestinal cells produce the highest concentrations of melatonin after the neuroendocrine system[8]. The different biosynthetic tissues allow melatonin to act as an intracellular mediator or paracrine signal, in addition to the described endocrine effects[4,9].
Recently, it has been proposed that melatonin would be synthesized as well in mitochondria due to the presence of the enzyme aralkylamine N-acetyltransferase/serotonin N-acetyltransferase (AANAT/SNAT)
However, in physiological situations where AANAT is inactive, alternative pathways for melatonin production could exist. In this sense, in C57BL/6 mice with genetic defects in AANAT, serotonin can be acetylated to N-acetylserotonin as an alternative to the arylamine transferase of AANAT[13]. This would indicate that there are alternative routes or mechanisms to produce and metabolize serotonin and N-acetylserotonin.
Approximately 30 µg/day of melatonin is secreted in adult humans[1]. Basal concentrations of melatonin are reached 4 to 8 h after secretion, although melatonin biosynthesis varies substantially depending on chronological age. After birth, children are not able to produce melatonin, acquiring it only from breast milk (27.3 ± 5.4 pg/mL). After three months of age, melatonin synthesis begins, and it is between 4 and 7 years when it reaches its maximum concentration (329.5 ± 42.0 pg/mL). From this moment, the synthesis decreases considerably, establishing a concentration of 62.5 ± 9.0 pg/mL between 15-20 years). Afterward, melatonin synthesis progressively decreases until reaching values of 29.2 ± 6.1 pg/mL in older adults. These low melatonin concentrations could contribute to the progressive worsening of organ function associated with aging[1,7,14].
Secretion and transport
The strategic anatomical location of the pineal gland and its high adjacent vascularization allow melatonin to be secreted and have rapid access to the bloodstream and cerebrospinal fluid. This favors infiltration to the deep tissues of the CNS as well as the rest of the peripheral tissues[1,6]. Due to its high solubility, amphipathic structure, and low molecular weight, melatonin displays a high intracellular and extracellular diffusion capacity, being able to cross cell membranes and the blood-brain barrier[6,15]. In blood, melatonin is transported bound to albumin (≈ 80%) or bound to hemoglobin; it is excreted in the urine (≈ 1%) and is not stored[16]. Some transporters that allow melatonin to cross the cell membrane into the cytosol are the glucose transporters (GLUT-1) and oligopeptide transporters (PepT 1 and 2). These transporters work by active transport against the gradient, enabling melatonin to reach the mitochondria, where it is found abundant[11].
Metabolism
The rapid absorption of melatonin allows it to reach its maximum plasma concentration after 40 min, with linear kinetics after oral administration at doses between 1-5 mg. Bioavailability is low, between 3%-33%, with a half-life ranging between 45-65 min after oral administration, and 0.5 to 6 min after parenteral administration. This difference is due to an important hepatic first-pass effect. Melatonin is metabolized by hepatic cytochrome P450 (CP450) into 6-hydroxymelatonin. This byproduct after the hepatic sulfoconjugation reaction (Phase II), with sulfate ion, is excreted by the renal system[6,17]. In the CNS, melatonin is metabolized mainly by the enzyme indoleamine 2,3-dioxygenase (IDO), giving rise to the metabolite N1-acetyl-N2-formyl-5-methoxykynuramine (AFMK), which is subsequently transformed into N-acetyl-5-methoxykynuramine (AMK)[1,6]. The presence of several isoforms of CP450 and AFMK has also been observed in mitochondria. However, in this case, AFMK is not the product of an interaction of melatonin with IDO, but with cytochrome C[8]. Melatonin is also metabolized by non-enzymatic processes induced by ultraviolet radiation (UVR) or free radicals. In this particular situation, melatonin is not enzymatically transformed into AFMK and AMK. In addition, melatonin is differently metabolized by antioxidant activity after interactions with ROS[8,18].
MECHANISMS OF ACTION OF MELATONIN
Melatonin actions can be classified as mediated and non-mediated by receptors[7].
Melatonin receptor-mediated actions
Membrane receptors
Melatonin can also act through specific cellular receptors located in the cell membrane, called MT1 and MT2. These are seven transmembrane-spanning proteins belonging to the G-protein-coupled receptor (GPCR) superfamily, by the cytosolic enzyme quinone reductase (QR2)[7] [Figure 2].
Figure 2. Melatonin-specific cellular receptors. AhR: Aryl hydrocarbon receptor; MT: melatonin receptor; QR2: quinine reductase.
Two MT subtypes (MT1 and MT2) have been described, differing in affinity and location. Melatonin receptors are widespread in the retina, brain, kidneys, gastrointestinal tract, skin, immune, endocrine, reproductive, and cardiovascular systems[19].
MT1 (350 amino acids) and MT2 (362 amino acids) are 60% homologous proteins that belong to the class of GPCRs, essentially inhibitory G proteins, with intracellular and extracellular domains and 7 transmembrane helices[20]. MT1 and MT2 modulate adenylyl cyclase and guanylyl cyclase responsible for cAMP and cGMP biosynthesis, as well as phospholipase C (PLC) activity. This allows the control of intracellular messengers such as inositol triphosphate (IP3) and subsequently, the calcium and potassium fluxes through Ca2+ and Na+/K+ channels, respectively[21,22]. MT1 has a 3 times greater affinity than MT2 for melatonin. MT1 receptors in the CNS allow melatonin to inhibit the activation of CNS neurons during the night, contributing to promoting sleep, modulating signal transduction in the reproductive system, and regulating peripheral vasoconstriction[16]. CNS MT2 receptors regulate the effects of melatonin on circadian rhythms. Both MT1 and MT2 receptors are highly susceptible to “desensitization”, their activity markedly decreases after exposure to supraphysiological concentrations of the hormone[23].
The binding of melatonin to MT1 and MT2 receptors results in several physiological responses activating different biological pathways[19,20,23] such as:
(a) cAMP/PKA/CREB signaling pathway, leading to the activation of calcium signaling by
(b) Raf/MEK1/2/ERK1/2 signaling pathway, involved in the regulation of cell proliferation.
(c) Activation of the ERK-MAPK/JNK signaling pathway and modulation of oxidative stress.
(d) Activation of the PI3K/Akt (PKB) signaling pathway, involved in the cardioprotective effect.
(e) Inhibition of the PKB pathway (target of the rapamycin complex in mammalian cells or mammalian Target of Rapamycin (mTOR)) and the GC/cGMP /PKG pathway to decrease proliferation and increase apoptosis of tumor cells.
Cytosolic receptors
MT3 receptors correspond to melatonin cytoplasmic receptors. Although MT3 is not considered a receptor per se, it is roughly a detoxifying enzyme, QR2, which inhibits the electron transfer reactions of quinones, allowing them to modulate and neutralize ROS[21]. Also, the aryl hydrocarbon receptor (AhR) has been identified as a receptor for melatonin and metabolites[24]. The AhR resides in the cytoplasm and, upon ligand binding, moves to the nucleus, where it heterodimerizes with the AhR nuclear translocator. Melatonin and its metabolites can act as AhR agonists and can interact with peroxisome proliferator-activated receptor-γ (PPARγ) at high concentrations. This would induce cytoprotective mechanisms against oxidative stress[24].
Actions non-mediated by cellular receptors
ROR/RZR is not a receptor for melatonin or its metabolites. It is a receptor for sterols and secosteroids. However, melatonin or its metabolites can indirectly modulate RORα and other ROR activities[25]. It has been hypothesized that the effects of melatonin on the transcriptional activities of RORα are through indirect actions. Thus, three indirect mechanisms have been proposed by Slominski et al., such as through MT1 or MT2 or other receptors/regulatory proteins, changes induced by melatonin or its metabolites in mitochondrial activity that affect the activity of RORα, and indirectly affecting the activity of the nuclear receptor, REV-ERBα, which also interacts with the RORE, thus influencing the expression of the same target genes as the RORα[25].
Additionally, melatonin can exert its effects by acting through receptor-independent pathways[6], which involve the direct interaction of melatonin and other molecules, mainly related to its antioxidant and radical scavenging action[22,26]. The amphipathic structure of melatonin allows easy access to different tissue cells and their organelles, interacting with intracellular molecules with no interaction with receptors, exerting in this way an antioxidant action. Furthermore, melatonin plays an important regulatory role in the ubiquitin-proteasome pathway, responsible for controlling protein degradation. In the Calcium-Calmodulin complex, melatonin inhibits the Ca2+/Calmodulin-dependent protein kinase, as well as influencing the transcription and expression of the so-called clock genes. In this context, melatonin acts as an inhibitor or stimulator of proteins (Period 1 and Period 2) that control the transcription and expression of other genes responsible for most of the cellular circadian functions[6,22,26].
ANTITUMOR MECHANISMS OF MELATONIN
Cancer is a set of diseases characterized by the rapid and uncontrolled multiplication of anomalous cells displaying alterations in cell growth, division, and apoptosis. This allows cancerous cells to spread and invade adjacent and distal tissues and organs. As a whole, cancer affects physiology homeostasis and the proper functioning of the organism[27]. Despite the diversity of cancer types, malignant cells share certain characteristics [Figure 3].
Figure 3. Main characteristics of cancer cells. ↑: Activation; ↓: inhibition; ATP: adenosine triphosphate; LDH: lactate dehydrogenase; PDH: pyruvate dehydrogenase; VEGF: vascular endothelial growth factor; ECM: extracellular matrix; MMP: metalloproteases; EMT: epithelial-mesenchymal transition; TNF-α: tumor necrosis factor alpha.
The potential effects of melatonin as an anticancer agent with multimodal oncostatic action have been reported[22], showing its ability to reduce tumor progression in monotherapy or combination with other treatments[28]. Furthermore, epidemiological studies have reported an association between cancer risk and melatonin deficiency. In this context, breast cancer patients undergoing oncological treatments and with low levels of melatonin present more resistance to oncologic treatments[29]. Cancer inhibition mechanisms associated with the effect of melatonin have been described in various types of neoplasms both in vitro and in vivo, including breast, endometrial, prostate, ovarian, laryngeal, liver, kidney, lung, gastric, pancreatic, and colorectal, among others[30]. Figure 4 shows the different proposed antitumor mechanisms of melatonin interacting with malignant cells[30].
Figure 4. Anticancer action mechanisms of melatonin. →: Promote; −ǀ: inhibit; −: modulate; NF-κβ: nuclear factor kappa B; TGF-β: transforming growth factor beta; PGE2: prostaglandin E2; EGFR: Epidermal growth factor receptor; PI3K: phosphoinositol 3-kinases; mTOR: mammalian Target of Rapamycin; MAPK/ERK: mitogen-activated protein kinases; JNK: c-Jun N-terminal kinase; IGF-1: insulin grow factor 1; CDKs: cyclin-dependent kinases; hTERT: human telomerase reverse transcriptase; SEEM: selective estrogen enzyme modulator; SERM: selective estrogen receptor modulator; ER: estrogen receptor; CaM: Calmodulin; AIF: apoptosis-inducing factor; XIAP: X-linked inhibitor of apoptosis; PAIs: inhibit the apoptosis process; MMP: metalloproteinases; EMT: epithelial-mesenchymal transition; VEGF: vascular endothelial growth factor; VEGFR2: vascular endothelial growth factor receptor 2; HIF: hypoxia-inducing factor; STAT3: signal transducer and activator of transcription 3; ET1: Endothelin-1; PDKs: phosphoinositide-dependent protein kinase; LA: lactic acid; 13-HODE: 13-hydroxyoctadecadienoic acid; Nrf2: nuclear factor erythroid 2; SIRT3: sirtuin 3; miRNA: microRNAs; SOD2: superoxide dismutase; GPX3: glutathione peroxidase.
Epigenetic factors
Epigenetic factors play a key role in tumor development. Epigenetic mechanisms include DNA methylation, histone modification, and the presence of small non-coding ribonucleic acid (RNA) molecules such as micro-RNA (miRNA) that regulate the post-transcriptional expression of target genes in the oncogenic process[29]. In general, the biological process of cancer begins with DNA mutation, modulating the genetic response towards carcinogenesis development[29].
micro-RNA
Melatonin could modulate/alter miRNA expression in various types of tumors, particularly in the breast, head and neck, liver, stomach, prostate, central nervous system, colon, and rectum. In these tumors, 46 micro-RNAs have been found with altered expression. These miRNAs regulate the expression of target genes related to biological processes such as regulation of the cell cycle, cell death and migration, and senescence. Melatonin seems to have a greater action in breast, oral, and gastric tumors, while this action is modest in prostate, colorectal tumors, and glioblastomas, through changes induced in miRNAs[31]. The expression changes in miRNAs alter the expression of their target genes, potentially decreasing the activity of oncogenes as well as increasing that of tumor suppressor and DNA repair genes[32].
Histones
Likewise, histone acetylation and/or methylation patterns induced by melatonin modulate the coding DNA sequences, modifying the balance of the genes to be transcribed, decreasing the transcription of proto-oncogenes and increasing that of tumor suppressor genes[33]. The interaction of melatonin with various isoforms of histone deacetylase (HDAC) alters its activity and can increase or decrease acetylation, favoring in all cases the suppression of proliferation and the induction of cell apoptosis.
At the same time, indolamine can inhibit histone lysine methylase, whose excessive accumulation results in a decrease in methylation, which leads to reduced expression of tumor suppressor genes[32]. Melatonin can act on HDACs by blocking their translocation to the nucleus by inhibiting calmodulin-dependent protein kinase II alpha (CaMKIIα)[28,34]. In particular, indolamine acts by promoting the dephosphorylation and nuclear import of HDAC4, responsible for the inactivation of CaMKII α[30,32].
Regulation of proliferation, cell cycle and apoptosis
The control of cell proliferation is mediated by the balance between proliferation and cell death. Otherwise said, the regulation of the cell cycle and the induction of apoptotic pathways are instrumental in the control of tumor growth[27]. Melatonin interacts with its membrane receptors, activating cascades of intracellular signals that regulate the transcription factors of key enzymes that act in the processes of cancer development, altering DNA and generating changes in cancer cells and their microenvironment, reducing carcinogenic development[28,33].
Proliferation
The first report regarding melatonin effects on cell proliferation was in vitro studies on the MCF-7 breast cell line[35]. Melatonin inhibits cell growth depending on the concentration, time and mode of exposure, the number of estrogen receptors, and the concentration of hormones and/or growth factors in the tumor microenvironment[36]. Therefore, melatonin can inhibit the growth of breast cancer cells in the MCF-7 and MDA-MB-231 cell lines by inducing cell death by increasing the expression of the apoptotic markers TUNEL and Bax and the autophagy Beclin-1, LC3, and p62[37].
Cellular cycle
Melatonin blocks the progression of cells from the G0/G1 phases to the S phase, causing an increase in the number of cells in the G0/G1 phase and a decrease in cells in the S phase, retaining cells in G0 (quiescent phase)[38]. The accumulation in G0 allows tumor cells to acquire a higher degree of differentiation. In general, cancer disease has a better prognosis and response to treatment when neoplastic cells display higher degrees of differentiation. Simultaneously, melatonin reduces the DNA replication rate in cells in transition to the G2/M phase[28,39].
The proposed mechanisms could lie in the ability of melatonin to regulate, through its receptor MT1, the transcription of the tumor suppressor gene p53, increasing the cell cycle inhibitor p27 (kip1) and the consequent activation of protein kinases (PK) A (PKA), and C (PKC)[28,39]. Melatonin induces an increase in the expression of p53 and p21/WAF, which act as negative regulators by inhibiting cyclin-dependent kinases and preventing the phosphorylation of the Rb (retinoblastoma protein), which is part of the last checkpoint of the G1 phase. Otherwise said, entry into the replication phase is blocked by the action of melatonin on cyclins and cyclin-dependent kinases that regulate the progression of the cycle through the S and G2/M phases. All these actions stop progression through the cell cycle[29,40].
Furthermore, melatonin could downregulate the transcription of cyclin D1, mediated by c-Jun and ATF-2 proteins[41], or reduce epidermal growth factor (EGF)[33]. It has also been suggested that the accumulation in the G1 phase of ovarian cancer cells (OVCAR-429 and PA-1) treated with melatonin is due to the regulation of CDK 2 and CDK4[41]. Under these conditions, the progression into the cell cycle is blocked. The importance of the ubiquitin-proteasome system in breast cancer has also been considered, due to its possible involvement in the regulation of the cell cycle. In the G1 to S transition, the ubiquitin ligase SCFskp2 (ubiquitin-proteasome system) is responsible for downregulating p21 and p27 to ubiquitination before degradation. Low levels of p27 in breast tumor cells are an indication of poor prognosis, which has led to SCFskp2 being considered a potential therapeutic target of melatonin[42].
Apoptosis
Regarding the apoptotic effect of melatonin, published reports present controversial results. On one hand, melatonin could protect normal cells against ROS, preventing apoptosis. On the other hand, melatonin has an apoptotic cytotoxic effect on myeloid cell lines HL-60, B lymphoma, MCF-7, prostate cancer cells LNCaP, or hepatocarcinoma HepG2[43]. Melatonin can trigger apoptosis by acting on several cytosolic pathways such as the PI3K/AKT/mTOR, the p38 kinase pathway of mitogen-activated protein kinases (MAPK/ERK or MAPK pathway), and the c-Jun N-terminal pathway (JNKs) JNK/c-jun[32].
The phosphatidylinositol 3-kinase (PI3K/Akt) pathway is a family of lipid kinases that modulate metabolic pathways that favor cell growth and proliferation and decrease apoptosis. Inhibition of the PI3K/Akt pathway by melatonin reduces Akt signaling, a precursor metabolite of the mTOR target, blocking proliferation, growth stimulation, and cycle progression of cancer cells[33,44].
The p38MAPK pathway contributes to cancer development. However, in this pathway, melatonin increases the concentration and activation of ERK, a molecule that regulates protein transcription in the cell nucleus, by acting on PKA and decreasing the formation of cAMP that induces negative regulation of the expression of mitogenic proteins and the antiapoptotic gene Bcl-2[28,30]. Furthermore, the influence of melatonin on the JNK/c-jun pathway is based on the activation of c-Jun N-terminal kinase (JNK), contributing to cell death through MT1[28,32].
The tumor suppressor p53 is a key transcription factor that regulates cellular pathways such as DNA repair, cell cycle, apoptosis, angiogenesis, and senescence. It acts as an important defense mechanism against the onset and progression of cancer. p53 is negatively regulated by the interaction with the oncoprotein murine double minute 2 (MDM2)[45]. In this line, melatonin exerts its therapeutic action by inhibiting MDM2/p53 complex formation. This complex induces an increase in the expression of pro-apoptotic proteins such as Bax and Bad, and decreases other anti-apoptotic proteins such as Bcl-2 and Bax[46]. Furthermore, a second mechanism involved would be related to TGF-β-dependent apoptotic processes, producing an increase in caspase 8, which in turn acts as an inducer of caspases 3, 6 and 7, giving rise to apoptosis[29,46].
Melatonin can also influence mitochondria-dependent apoptotic processes by interfering with the release of ROS, cytochrome c, and cell death regulatory proteins[47]. The resistance of cancer cells to apoptosis depends largely on the action of proteins that inhibit the apoptosis process, such as XIAP, cIAP-1, cIAP-2, survivin, and levin. These proteins limit the activity of initiator and effector caspases[28]. Intramitochondrial melatonin can be associated with XIAP and survivin deactivation using the COX-2/PI3K/Akt pathway. This pathway is involved in mitochondrial dynamics by decreasing cyclooxygenase 2 (COX-2) concentration and ROS-dependent Akt activation[28,34,48] Other antiapoptotic proteins that intervene in the process of mitochondrial apoptosis and whose activity can be regulated by melatonin are Bcl-2. These proteins prevent the release of cytochrome c by inactivating Bax proteins, and thus the formation of the apoptosome, a complex formed by cytochrome c when it binds to apoptosis protease-activating factor 1(Apaf-1). This complex allows the activation of caspase-9, which subsequently activates the effector caspases (caspases-3) that initiate DNA degradation[47]. Melatonin is responsible for increasing the expression of the mediator that interacts with Bcl-2 (Bim), activating the transcription of the FoxO3a factor, and increasing its concentration in the nucleus[30]. Additionally, melatonin can promote, in mitochondria, the formation of the apoptosis-inducing factor (AIF) precursor protein that promotes cell death by triggering chromatin condensation and DNA fragmentation[47].
Antiestrogenic action
Among the oncostatic effects of melatonin, antiestrogenic actions are instrumental, especially relevant in hormone-dependent tumors, such as breast, prostate, and ovarian cancer[49]. Two possible mechanisms have been described that would explain how melatonin reduces the development of hormone-dependent cancer through its binding to MT1 receptors[50]. First, an indirect mechanism of melatonin through an inhibitory action on the hypothalamus-pituitary-gonadal reproductive axis should be considered. This would decrease circulating levels of estrogen, gonadotropin-releasing hormone (GnRH), prolactin, and gonadotropins and the ability of melatonin to modulate the activity of enzymes involved in the synthesis of estrogens, both gonadal and extragonadal. These properties of melatonin enable it to function as a selective estrogen enzyme modulator (SEEM)[50].
Melatonin, like SEEM, can intervene through the MT1 receptor in enzymatic pathways that collaborate in the transformation of weak estrogens into potent and biologically active estrogens, promoting their synthesis[51]. Melatonin inhibits the expression and activity of enzymes that positively collaborate in this process, such as aromatase or steroid sulfatase, and 17β-hydroxysteroid dehydrogenase. In addition, it stimulates those that perform the reverse function, such as estrogen sulfotransferase, regulating the local synthesis of estrogens towards levels similar to those found in normal breast tissue[46,50].
The actions of melatonin with selective estrogen receptor modulator (SERM) activity are capable of decreasing estrogen production by intervening in the estradiol (E2) signaling pathway, by reducing the expression of estrogen receptors (ER) alpha Erα and blocking its transcriptional activity[28,46]. The activation of ERα by E2 can occur through increased concentrations of cAMP, enhancing ER transcription. Melatonin, through the membrane receptor MT1, can decrease the activity of PKA by inhibiting the enzyme adenylate cyclase, thus being able to counteract the effect of estrogens[50]. In turn, melatonin reduces estrogen concentration by inhibiting the binding of the E2-ER complex to the estrogen response element (ERE) in DNA[52,53]. The association of this complex with calmodulin (CaM) induces its binding to the ERE, with CaM being considered a regulator of E2 transcription[54]. This calcium-regulatory protein can be inhibited by melatonin by binding directly to it[53]. This also allows melatonin to acquire the ability to improve the phosphorylation and activation of transcription factors and nuclear binding sites, involved in cell proliferation[52].
In this way, the antiestrogenic actions of melatonin can be explained both through its SERM and SEEM properties[51,52]. Altogether, this supports the therapeutic potential of this hormone for its possible use in the treatment of hormone-dependent cancer[50].
Other actions of melatonin such as SEEM consist of the inhibition of the desmoplastic reaction due to the ability to promote the expression of transcription factors (PPAR and c/EBP) involved in the differentiation of fibroblasts into mature adipocytes. This occurs through an indirect mechanism by reducing the synthesis and secretion of antiadipogenic cytokines by malignant epithelial cells and fibroblasts. Furthermore, (melatonin) reduces aromatase activity in both cell types, thus decreasing local estrogen production[55].
Another activity of melatonin linked to SERM is its inhibitory effect on the stimulation of overexpression of the retinoic acid receptor (RORα) on the transcriptional activity of ERα[55]. Melatonin can mimic the action of proteasome inhibitors, causing a decrease in estrogen-induced gene transcription in breast MCF-7 cells[55].
Inhibition of telomerase activity
Reducing the ability of tumor cells to divide unlimitedly is a therapeutic target to reduce cancer progression. Melatonin acts on this process by mediating the PI3K-Akt/ mTOR pathways and cyclin-dependent kinases (CDKs), in the activity of telomerase[32]. Telomerases prevent the natural shortening of chromosomes by adding specialized ribonuclear protein sequences to telomeres, which maintain DNA stability despite frequent cell divisions[34]. Melatonin collaborates in the inhibition of human telomerase reverse transcriptase (hTERT), a subunit of telomerase that is mainly responsible for the activation of the enzyme[56]. It can do this by reducing the expression of hTERT mRNA, but also by its - anti-estrogenic capacity. The presence of the imperfect ERE in the hTERT promoter explains the ability of estrogens to regulate the expression of hTERT and, thereby, the activity of telomerase[57].
Modulation of metastasis
The mechanisms of action of melatonin in the modulation of metastasis are targeted in controlling their development induced by tumor cells as well as by microenvironmental tumoral factors[28]. In general, the actions are mediated by MT1 receptors to minimize the process and slow the progression of cancer[34], such as acting on the expression of cell adhesion molecules, modulation of the expression of metalloproteinases (MMP) and integrins, control of cytoskeletal movements and regulation of epithelial-mesenchymal transition (EMT)[33,34,58].
Cell adhesion is achieved through a variety of cell junctions, such as occlusive (tight junctions), anchoring (adherent, desmosomes, and hemidesmosomes), and communicating (gap junctions)[59]. The molecules responsible for cell adhesion are cadherins and integrins, which are necessary to keep cells together. In tumor cells, these molecules are missing, favoring increased tumor invasion. On the other hand, adhesion to the extracellular matrix allows cells to survive and proliferate. If a cell loses its ability to adhere to the extracellular matrix, it will be prone to detach from the original tissue and invade surrounding tissues[27,60]. Melatonin increases the expression of cadherins (E-cadherin) by preventing the interaction of NF-κß with the junctional enhancer protein CCAAT (C-EBPβ), integrins (β1-integrin) by reducing the expression of αvβ3 integrin, occludin (tight junctions)[28,29] and vimentin, proteins involved in cell adhesion, migration, and invasiveness. Altogether, these proteins may contribute to modulating the metastatic potential of cancer cells[61,62].
Regarding the modulation of the expression of MMPs, proteins that promote the remodeling of the extracellular matrix (ECM)[59], melatonin achieves this repressive effect by inhibiting the p38 MAPK signaling pathway through PKA, exerting a negative effect on transcription by NF-kB, and even by docking directly at the MMP-9 activation site[28,62].
Melatonin is associated with a decrease in the expression of Rho-associated protein 1 (ROCK1) and myosin light chain kinase (MLCK), in the presence of Ca2+/calmodulin and ATP MLCK catalyzes the phosphorylation of myosin light chain (MLC). All of them are molecules that modulate the dynamics of the cytoskeleton, and in particular, MLC collaborates in the interactions of actin and myosin filaments[63], enabling cellular movement of the cytoskeleton.
Cell mobility can also increase through EMT, a process controlled by external transcription and signaling stimuli such as NF-κß, Wnt, AP-1, and growth factors, among others. Melatonin could intervene in the activity of NF-κß, a molecule that suppresses the epithelial phenotype by inhibiting the transcription of proteins that negatively regulate E-cadherin. In turn, NF-κß represents an obstacle to EMT by contributing to the induction of vimentin, a protein responsible for maintaining the mesenchymal phenotype, as well as increasing cell migration[33,62]. Another EMT regulatory pathway in which melatonin is involved is Caterin Wnt /β. As the cell's invasiveness progresses, low concentrations of E-cadherin can favor the release of β-catenin from cell junctions to the nucleus. In this process, melatonin promotes the elimination of β-catenin by interfering with the phosphorylation of Akt[28,62].
Inhibition of angiogenesis
Angiogenesis is a physiological process that allows tumors to progress rapidly by creating new blood vessels that provide oxygen and nutrients[59]. Vascular endothelial growth factor (VEGF) is key in this process[33]. The antiangiogenic actions of melatonin are centered on reducing the mediators that participate in the formation of extensive capillary structures or decreasing the density of blood vessels[23,32]. In this line, the blood vessels formed during angiogenesis are highly disorganized and can increase tissue hypoxia, a biological signal that favors the action of pro-angiogenic factors and the activation of their receptors[11].
VEGF accumulation can be decreased by suppressing its expression through regulation of mRNA transcription or by inhibiting the nuclear receptor RZR/RORγ[31]. Furthermore, another mechanism by which this inhibition of VEGF occurs seems to be related to the destabilization of the heterodimer hypoxia-inducing factor (HIF) one alpha (HIF-1α). HIF-1α in a cellular microenvironment with low oxygen concentrations avoids being hydroxylated by prolyl 4-hydroxylases (PHDs) and subsequently degraded by the Von - Hippel -Lindau tumor suppressor protein (pVHL)[62]. The antioxidant action of melatonin allows the elimination of ROS that interfere with the activity of PHD, allowing HIF-1α to be destabilized during hypoxia[62,63]. On the other hand, the HIF-1α/HIF-1β dimer, together with the signal transducer and activator of transcription 3 (STAT3), an inducer of HIF-1α stability, participate in the genetic transcription of VEGF by binding to its activator CBP/p300. To avoid this, melatonin can act not only by promoting the degradation of the molecule but also by intervening in the translation of HIF-1α associated with p70S6K or in the activity of STAT3[30,62,63].
The antiangiogenic activity of melatonin also occurs through a series of indirect mechanisms. One of them is based on the inhibition of endothelin 1 (ET-1), which, in addition to functioning as a mitogen, also has pro-angiogenic properties. ET-1 is a vasoconstrictor that collaborates in the growth and invasion of cancer cells by intervening in tumor angiogenesis by stimulating the development of blood vessels[59]. The ability of melatonin as an inhibitor of ET-1 synthesis has been demonstrated by reducing the expression of its corresponding mRNA, as well as by inactivating FoxO1 and NF-κß[28,30]. Inhibition of NF-κB allows the activity of MMP-9 to be reduced, inhibiting the angiogenic process and controlling vascular endothelial permeability[62].
Antioxidant action against carcinogenesis
The excess of free radicals in carcinogenic processes induces an oxidative state (OS) that exceeds the body's ability to neutralize them. OS contributes to carcinogenesis, cell growth, differentiation, and apoptosis. For these reasons, it has been suggested that the enhancement of the endogenous antioxidant mechanisms in the body can protect against cancer[60]. Melatonin can reduce ROS, thereby preventing genetic damage and aiding in reducing carcinogenesis in different phases such as initiation, progression, and metastasis[26,64]. However, it can also increase the production of ROS, promoting the apoptosis of malignant cells[47,64]. Therefore, melatonin has a dual effect as an antioxidant acting as an antiproliferative, and as a prooxidant acting as cytotoxic[30]. Melatonin as an antioxidant, among other actions, directly neutralizes free radicals and ROS, stimulates the synthesis of antioxidant substances such as nitric oxide and glutathione, increases the activity of antioxidant enzymes such as glutathione peroxidase, superoxide dismutase (SOD2) and catalase, inhibits the activity of oxidative enzymes, and protects the function of mitochondria by increasing the activity of the transport chain and preventing depolarization of the mitochondrial membrane[30,33]. Due to the activation of these antioxidant enzymes, melatonin favors the phosphorylation and translocation to the nucleus of nuclear factor erythroid 2 (Nrf2) by raising intracellular Ca2+ and the subsequent activation of PKC[32]. Nrf2, once in the nucleus, can bind to the DNA sequence known as the antioxidant response element (ARE) and control the expression of genes coding for antioxidant enzymes. Melatonin is also responsible for the stimulation of the enzyme sirtuin 3 (SIRT3), which causes the deacetylation and activation of SOD2[12,47].
Actions on cellular metabolism
The effects of melatonin in regulating the function of mitochondria allow it to have anticancer properties. (melatonin) can repress aerobic glycolysis and increase mitochondrial oxidative phosphorylation, reversing the Warburg effect produced in cancer cells, an action that reduces proliferation and increases cell apoptosis[48]. The transformation of metabolism in cancer cells depends on the intervention of several transcription factors. Among them, HIF-1 stands out, which increases the expression of numerous glycolytic enzymes and glucose transporters. In addition, it promotes the activity of pyruvate dehydrogenase kinases (PDKs), which inactivates pyruvate dehydrogenase (PDH)[33]. The action of melatonin allows it to inhibit HIF-1, reducing the formation of pyruvate and increasing the concentration of Acetyl CoA. Acetyl CoA is an important metabolite that intervenes in the Krebs cycle of the oxidative metabolic pathway carried out in the mitochondria, and in turn, a cofactor in the production of the N-acetyltransferase/serotonin (AANAT/SNAT), essential in the synthesis of melatonin[64].
On the other hand, in the process of cancer formation and development, it is essential to have some n-6 fatty acids, such as linoleic acid, which is used in the biosynthesis of prostaglandins and cell membrane phospholipids[34]. Melatonin can intervene in lipid metabolism by limiting the intracellular uptake of these fatty acids by reducing the formation of cAMP, as well as its transformation to 13-hydroxyoctadecadienoic acid (13-HODE) in the presence of 15-lipoxygenase. 13-HODE participates in cell proliferation by acting on the EGF pathway as an amplifying signal of mitogenesis, and on the metabolites of the MAPK/ERK pathway[15,28].
Immunomodulatory properties
Modulation of the immune system has appeared in recent times as a possible strategy in cancer treatment, inducing a powerful antitumor response[65]. Melatonin can influence both specific and non-specific immune factors, acting as an effective immune enhancer and anti-inflammatory agent[34].
The NF-κß factor is a protein complex that controls cytokine production, cell survival, and DNA transcription[30,34]. The increase in this transcription factor drives the formation of inducible nitric oxide synthase (iNOS) and COX-2, the latter an important mediator of inflammation and a key enzyme in prostaglandin synthesis[33,48]. Therefore, inhibition of NF-κß promotes an anti-inflammatory response, which could influence the redox status and immune microenvironment of the tumor[28,33]. Melatonin, through the MT1 receptor, inhibits NF-κß, modulating its translocation to the nucleus and binding to DNA, which leads to the negative regulation of COX-2[28,30]. The hormone can also reduce COX-2 synthesis directly by blocking the transcription of the COX-2 activator (p52) by inhibiting the activity of p300 histone acetyltransferase (HAT)[33,48].
Transforming growth factor beta (TGF-β) and prostaglandin E2 (PGE2) are instrumental target molecules released by cancer-associated fibroblasts (CAFs) that contribute to tumor proliferation. These metabolites decrease the body's antitumor capacity by attenuating the activity of NK cells, dendritic cells, and CD8+ cytotoxic T lymphocytes, the main immunological defense against cancer development[48]. PGE2 inhibits the release of IL-2, an interleukin that intervenes in the proliferation and activity of both NK cells and CD8+ cytotoxic T lymphocytes. Melatonin can reduce the action of PGE-2, allowing the secretion of IL-2, stimulating at the same time Th1 lymphocytes and monocytes to increase the release of other cytokines, such as IL-6, IL-12, IL-27, and TNF-α, also regulators of NK cell proliferation[33,48]. On the other hand, TGF-β, together with other molecules such as IL-4, are considered inducers of regulatory T cells (Tregs), capable of blocking the antitumor effect of the immune system. Melatonin acts by reducing the synthesis and gene expression of Forkp3, increasing the presence of Treg inhibitors such as IFN-γ[26,48]. These can be produced by NK cells, and act by promoting the activity of macrophages and improving the antigen presentation of tumor cells[26].
APPLICATIONS OF MELATONIN IN CLINICAL TRIALS AS AN ADJUVANT TO MEDICAL ONCOLOGY THERAPIES
Melatonin is considered a dietary supplement in the USA. However, in Australia, melatonin is a drug that requires a medical prescription. Nevertheless, in Europe, in 2007, the European Medicines Evaluation Agency (EMA) authorized melatonin to be marketed in both forms, as a drug and as a supplement for the treatment of circadian rhythm sleep disorders[7].
In any case, clinical trials are essential to evaluate the effects of melatonin in patients with various types of cancer, and in this sense, Lissoni et al. carried out the first clinical trial in humans with substantial benefits on quality of life and functional status after the administration of melatonin during chemo- and radiotherapy[66]. Most of the clinical studies[67-69] in humans where melatonin has been administered as a therapeutic adjuvant have been carried out on patients with an advanced stage of cancer, with metastasis and without response to conventional treatments. Taking into account the situation of these patients who had no complete response to treatment, their partial response was notably better in patients supplemented with melatonin[67-69].
In patients with non-small cell lung cancer, melatonin treatment as an adjuvant to conventional chemotherapy increased 5-year survival, and increased tumor regression in a notable number of patients[70]. In a cohort study[69] with 250 patients with various types of cancer in advanced stages of different tumor types and with melatonin supplementation during a period of one year, they presented a higher survival rate and had fewer statistically significant side effects associated with chemotherapy (thrombocytopenia, asthenia, stomatitis, and neuropathies), compared to the non-supplemented group[69]. Melatonin could also have protective effects against cardio- and neurotoxicity associated with chemotherapy drugs, favoring survival and tumor regression[68]. Furthermore, it has been reported that melatonin would safeguard hematopoietic stem cells from toxicity induced by chemo- and radiotherapy[71]. However, some side effects such as weight or appetite loss associated with chemo- and radiotherapy were not reversed with melatonin supplementation in patients with advanced lung, breast[72], and brain tumors[73].
Ten trials updated in recent years on Clinicaltrials.gov showed melatonin supplementation in cancer therapy between 1 and 20 mg/day for periods ranging from 10 days to 12 months [Table 1]. Those that present results directly (NCT01805089) or through related publications[73-78] showed heterogeneous results. The beneficial effects of melatonin (3 mg/day) have been demonstrated in mood, sleep quality, and menopause symptoms (NCT01805089). Also, adjuvant 20 mg/day melatonin treatment following resection of non-small cell lung cancer (NSCLC) increased five-year disease-free survival in patients with late-stage disease[78], reduced the expression of miR-210 and CD44 in squamous cell carcinoma of the oral cavity[78], and counteracted the dysfunction in the inhibitory pain modulatory system and improved pain perception measures and neuroplasticity state in women with breast cancer[75]. However, supplementation with
Clinical trials with melatonin registered at https://clinicaltrials.gov/ for the treatment of adult patients with cancer
Registry number, last update year, country, and publication about study results | Study design | Participants | Intervention | Objectives | Outcomes |
NCT06125353, 2023, Greece, NR, and without results | Randomized placebo-controlled study | Women (≥ 18 years) diagnosed with breast cancer who underwent chemotherapy | 1 mg/day + Mediterranean diet 3 months | Evaluate whether adherence to the Mediterranean diet, together with melatonin oral treatment or placebo, would ameliorate Cancer related fatigue in breast cancer patients receiving chemotherapy treatment | Cancer-related fatigue By functional assessment of chronic illness therapy - fatigue questionnaire. MD adherence by the MedDiet score |
NCT00031967, 2022, USA, Berk et al.[73], and without results | Randomized phase II trial (RTOG 0119) | Brain metastasis patients (≥ 65 years) undergo whole brain irradiation once a day 5 days a week for 2 weeks | 20 mg/day a.m. or p.m. intakes 6 months | Determine the effect of melatonin, used as radiosensitization/radioprotection, on overall survival and clinical deterioration in patients with brain metastases who are undergoing radiotherapy | Survival |
NCT01805089, 2023, USA, NR, and, with results | Triple-blind placebo-controlled randomized clinical trial | Women postmenopausal with a history of ductal carcinoma in situ, lobular carcinoma in situ, or breast cancer in stages 1-3. Patients are not currently receiving chemotherapy or hormonal therapy | 3 mg/day p.m. intakes 4 months | Melatonin's effects on sleep, hot flashes, mood, estradiol blood levels, and insulin-growth factor blood levels | Mood, sleep quality and menopausal symptoms, estradiol blood levels, and insulin-growth factor blood levels |
NCT00668707, 2021, Canada, Seely et al.[78], and with results | Parallel blind quadruple randomized controlled trial | Adults (≥ 18 years) with a clinical diagnosis of non-small cell lung cancer, eligible for surgical resection | 20 mg/day a.m. or p.m. intakes 12 months | To evaluate the potential of melatonin as an additional aid for the treatment of patients with lung cancer, improving survival and parameters related to quality of life | Quality of life, pain, fatigue, anxiety, sleep and depression, and incidence of lung cancer recurrence and/or death |
NCT00513357, 2022, USA, | Triple-blind placebo-controlled randomized clinical trial | Adults (≥ 18 years) with solid gastrointestinal tumors, or lung cancer referred to palliative care and an unintentional weight loss of 5% or more in the last 6 months with anorexia (> 3 on a visual analog scale such as ESAS) | 20 mg/day Before sleep 8 weeks | To evaluate the effectiveness of melatonin on weight gain, maintenance/gain of lean muscle mass, improvement of appetite, and side effects in patients with advanced cancer | Appetite, weight, or quality of life |
NCT00925899, 2021, Denmark, Lund Rasmussen et al.[76], and with results | Blinded Crossover Quadruple Randomized Trial | Adults (≥ 18 years) with cancer in the palliative phase or phase IV; answered "quite a bit" or "very much" to the question “ Were you tired?” (from EORTC QLQ-C15-PAL) | 20 mg/day 1 week washout period 48 h | To investigate if a supplement of melatonin affects (a) fatigue (the primary outcome of the trial); (b) the symptoms of insomnia; appetite loss, depression, and pain; and (c) overall quality of life | Fatigue, symptoms of insomnia, appetite loss, depression, pain, and quality of life |
NCT01706627, 2022, Thailand, NR, and without results | Multicenter, randomized, double-blind, placebo-controlled trial | Adults (≥ 18 years) with histologically proven advanced non-small cell lung, breast, head, and neck or sarcoma cancer | 10 or 20 mg/day after 9.00 p.m. 6 months | To evaluate the effect of melatonin in improving quality of life and reducing chemotherapy-induced toxicity in advanced cancer patients | Quality of life (FACT), oxidative stress status, melatonin level, overall survival, Number of participants with adverse events |
NCT01965522, 2021, Canada, NR, and without results | Blinded crossover quadruple randomized trial | 36 women (≥ 18 years) with histologically confirmed invasive breast cancer (ductal, lobular, or mixed) before definitive surgery (lumpectomy or mastectomy) in 3 or more weeks | 20 mg/day 4 weeks | To evaluate the anticancer activity of melatonin | Ki67 proliferation rate, change in serum micro-RNA profile |
NCT03205033, 2021, Brazil, | Randomized, double-blind, parallel, placebo-controlled clinical trial | Women (≥ 18 years) with breast cancer treated surgically and with an indication for adjuvant chemotherapy, aged between 18 and 75 years | 20 mg/day 10 days | To evaluate the response to melatonin as a synchronizer of the sleep-wake rhythm, neuromodulator, and genoprotective myeloprotective in the effects induced by chemotherapy in women with breast cancer | Sleep-wake rhythm (actigraphy, sleep diary), depressive symptoms, pain threshold pressure, effect on platelets and white blood cell series, pain response to conditioned stimuli and quality of life, serum markers of neuroplasticity (BDNF and calcium-binding protein S100 β) and effect of the micronucleus will be quantified using the cellular material of the oral mucosa. |
NCT04137627, 2022, Indonesia, | Randomized, double-blind, placebo-controlled trial | Child, adults, and older adults with locally advanced oral squamous cell carcinoma who are planned with neoadjuvant chemotherapy | 20 mg/day 12 months | To demonstrate the efficacy of melatonin in increasing clinical response in patients with locally advanced OSCC when treated with NC | Clinical Response is measured using RECIST 1.1. criteria, change in expression of HIF-1⍺, miR-210, CD44, CD133 |
In this review, the potential mechanisms of the complex and diverse antineoplastic properties of melatonin have been described. However, there may be relatively weak experimental evidence as to why it is not more widely used as an adjunct in cancer therapy or generates little interest from pharmaceutical companies. Perhaps the dose and duration of melatonin are not adequate. The recommended range in humans
POTENTIAL ROLE OF MELATONIN ANALOGS AS THERAPEUTICS IN CANCER THERAPY
One of the biggest obstacles to the use of melatonin as a clinically effective anticancer is its short half-life (between 20 and 40 min). This problem could be solved by developing new formulations with melatoninergic agonists with a longer half-life and greater power, such as agomelatine, ramelteon, and tasimelteon, among others. Additionally, Neu-P11, is an agonist of MLT receptors MT1, MT2, MT3 receptors[83], and serotonin receptors: 5-HT1a, 5-HT1b and 5-HT2b[84]. Currently, synthetic melatonin agonists have the potential to act in the treatment of insomnia disorders and depression[85]. Melatonin receptors would influence cancer pathology. In this sense, the MT1 melatonin receptor has oncostatic potential in breast and prostate cancer. In breast cancer, the activation of MT1 receptors inhibits the expression and/or phospho-activation of numerous kinases, transcription factors, and co-regulators, which are responsible for the progression of this type of cancer[86]. Therefore, MT1 receptor agonists could be useful tools in cancer treatment, in monotherapy, or as adjuvants to medical oncology therapies. Melatonin analogs UCM 976, UCM 1032, UCM 1033, UCM 1037) that target membrane receptors MT1 and MT2 showed an ability to inhibit the proliferation of DX3 melanoma cells and MCF-7 breast cancer cells, and MDA-MB231 in a dose- and time-dependent manner in viability assays. Furthermore, UCM 1037 at concentrations of 0.1 mM exhibited antiproliferative and cytotoxic activity in vitro on breast cancer cells. In vivo testing in xenograft mouse models confirmed that UCM 1037 suppressed DX3 tumor growth and demonstrated greater inhibitory activity compared to melatonin alone at the same dose (16 mg/kg)[87]. Future studies of the synergistic relationships between melatonin analogs and anticancer drugs are a promising field, and these compounds could significantly strengthen the cytostatic and cytotoxic effects triggered by conventional antineoplastic drugs.
POTENTIAL EFFECTS OF MELATONIN METABOLITES
The metabolites AFMK and AMK can limit oxidative stress by eliminating toxic radicals and inhibiting their generation, especially at the mitochondrial level[88]. AMK showed higher efficiency in scavenging ROS and preventing protein oxidation relative to AFMK. In this sense, AMK is a better OH • - and NO eliminator than melatonin and AFMK[89]. Regarding AFMK, it is a less effective free radical scavenger than AMK and melatonin. However, unlike vitamin C and vitamin E, AMFK can donate more than one electron[90].
CONCLUSION
Melatonin is marketed as a dietary supplement and as a medicine, the latter for the indication of insomnia. The participation of the molecule in the activation of various anticancer actions mediated by its receptors [Figure 5] that act on the regulation of the different characteristics of neoplastic cells, could establish this molecule as an important anticancer agent.
In summary, the main mechanisms that allow melatonin to have anticancer properties are induction of epigenetic processes, regulation of the cell cycle, modulation of proliferation, induction of apoptosis, inhibitory actions of telomerase, and pro-inflammatory activities, and antioxidants, anti-estrogenic action, reversal of the Warburg effect, antiangiogenesis, and anti-inflammatory and immunomodulatory processes. Furthermore, others such as the inhibition or activation of enzymes that participate in the carcinogenesis process could reduce the proliferative and metastatic capacity of tumor cells. The low toxicity of melatonin, its low cost, and its easy availability, since it can be synthesized endogenously by the body, or easily acquired exogenously using pharmacological sources, and even non-pharmacological sources through diet and healthy lifestyle habits, make it a good candidate for widespread use as an adjuvant in cancer therapies. Melatonin is an endogenously synthesized indoleamine well known for its potent antioxidant effect that exerts a beneficial action in many pathological conditions, although studies on its clinical application in humans as a potential anticancer agent are limited.
Due to the diversity in the types of treatment, melatonin has been combined with chemotherapy agents, radiotherapy, or alternative therapies. Furthermore, the dose of melatonin used is still a matter of study. In most cancer intervention studies, melatonin was administered in a range of 20-40 mg, always orally and several hours before the onset of sleep. This dose is much higher than that used for the treatment of sleep disorders. This raises the question of toxicity and whether there are significant side effects at these levels. For these reasons, it is necessary to continue research to better know and understand the anticancer effects that melatonin can produce in patients, the effective dose, bioavailability, and the safety that it represents in the long term. Altogether, they come to consider melatonin as a standardized therapeutic option in the treatment of cancer.
DECLARATIONS
Acknowledgments
The authors would like to thank the Neurobiology Research Group of the University of Valladolid, and the Department of Cellular Biology, Genetics, Histology and Pharmacology, Faculty of Medicine, University of Valladolid (Spain) for their collaboration in carrying out the review.
Author’s contributions
Conceived and designed the study, analyzed, and interpreted the data, drafted the paper, writing - original draft, prepared figures and/or tables, and approved the final version submitted for publication:
Conceptualized and planned, revised manuscript, critically reviewed the paper and prepared figures and/or tables: Celorrio San Miguel AM
Writing, analyzed, and interpreted the data and critically reviewed the paper: Roche E
All authors read and approved the final manuscript.
Availability of data and materials
Not applicable.
Financial support and sponsorship
This study has been financed by the Department of Education of the Junta de Castilla - León and the European Regional Development Fund (FEDER) by TCUE Plan 2021-2023, 134/2021, within the research project “Application of Genomics through the Study of Genetic polymorphisms in the treatment and prevention of chronic diseases in older adult patients”; (grant nos. SO002P23).
Conflicts of interest
All authors declared that there are no conflicts of interest.
Ethical approval and consent to participate
Not applicable.
Consent for publication
Not applicable.
Copyright
© The Author(s) 2024.
REFERENCES
1. Amaral FGD, Cipolla-Neto J. A brief review about melatonin, a pineal hormone. Arch Endocrinol Metab 2018;62:472-9.
2. Tan DX, Manchester LC, Hardeland R, et al. Melatonin: a hormone, a tissue factor, an autocoid, a paracoid, and an antioxidant vitamin. J Pineal Res 2003;34:75-8.
3. Castroviejo D, López LC, Escames G, López A, García JA, Reiter RJ. Melatonin-mitochondria interplay in health and disease. Curr Top Med Chem 2011;11:221-40.
4. Acuña-Castroviejo D, Escames G, Venegas C, et al. Extrapineal melatonin: sources, regulation, and potential functions. Cell Mol Life Sci 2014;71:2997-3025.
5. Majidinia M, Reiter RJ, Shakouri SK, et al. The multiple functions of melatonin in regenerative medicine. Ageing Res Rev 2018;45:33-52.
6. Cipolla-Neto J, Amaral FGD. Melatonin as a hormone: new physiological and clinical insights. Endocr Rev 2018;39:990-1028.
7. Tordjman S, Chokron S, Delorme R, et al. Melatonin: pharmacology, functions and therapeutic benefits. Curr Neuropharmacol 2017;15:434-43.
8. Tan D, Reiter RJ. Mitochondria: the birth place, battle ground and the site of melatonin metabolism in cells. Melatonin Res 2019;2:44-66.
9. Stefulj J, Hörtner M, Ghosh M, et al. Gene expression of the key enzymes of melatonin synthesis in extrapineal tissues of the rat. J Pineal Res 2001;30:243-7.
10. Tan DX, Manchester LC, Qin L, Reiter RJ. Melatonin: a mitochondrial targeting molecule involving mitochondrial protection and dynamics. Int J Mol Sci 2016;17:2124.
11. Reiter RJ, Tan DX, Rosales-Corral S, Galano A, Zhou XJ, Xu B. Mitochondria: central organelles for melatonin's antioxidant and anti-aging actions. Molecules 2018;23:509.
12. Reiter RJ, Sharma R, Pires de Campos Zuccari DA, de Almeida Chuffa LG, Manucha W, Rodriguez C. Melatonin synthesis in and uptake by mitochondria: implications for diseased cells with dysfunctional mitochondria. Future Med Chem 2021;13:335-9.
13. Slominski A, Pisarchik A, Semak I, Sweatman T, Wortsman J. Characterization of the serotoninergic system in the C57BL/6 mouse skin. Eur J Biochem 2003;270:3335-44.
14. Meng X, Li Y, Li S, et al. Dietary sources and bioactivities of melatonin. Nutrients 2017;9:367.
15. Ahmad SB, Ali A, Bilal M, et al. Melatonin and health: insights of melatonin action, biological functions, and associated disorders. Cell Mol Neurobiol 2023;43:2437-58.
16. Kopustinskiene DM, Bernatoniene J. Molecular mechanisms of melatonin-mediated cell protection and signaling in health and disease. Pharmaceutics 2021;13:129.
17. Poza JJ, Pujol M, Ortega-Albás JJ, Romero O. Insomnia Study Group of the Spanish Sleep Society (SES). Melatonin in sleep disorders. Neurologia 2022;37:575-85.
18. Tan DX, Manchester LC, Esteban-Zubero E, Zhou Z, Reiter RJ. Melatonin as a potent and inducible endogenous antioxidant: synthesis and metabolism. Molecules 2015;20:18886-906.
19. Dubocovich ML, Markowska M. Functional MT1 and MT2 melatonin receptors in mammals. Endocrine 2005;27:101-10.
20. Stauch B, Johansson LC, Cherezov V. Structural insights into melatonin receptors. FEBS J 2020;287:1496-510.
21. von Gall C, Stehle JH, Weaver DR. Mammalian melatonin receptors: molecular biology and signal transduction. Cell Tissue Res 2002;309:151-62.
23. Liu J, Clough SJ, Hutchinson AJ, Adamah-Biassi EB, Popovska-Gorevski M, Dubocovich ML. MT1 and MT2 melatonin receptors: a therapeutic perspective. Annu Rev Pharmacol Toxicol 2016;56:361-83.
24. Slominski AT, Kim TK, Slominski RM, et al. Melatonin and its metabolites can serve as agonists on the aryl hydrocarbon receptor and peroxisome proliferator-activated receptor gamma. Int J Mol Sci 2023;24:15496.
25. Slominski AT, Zmijewski MA, Jetten AM. RORα is not a receptor for melatonin. Bioessays 2016;38:1193-4.
26. Vinther AG, Claësson MH. The influence of melatonin on immune system and cancer. Ugeskr Laeger 2015;177:V10140568.
27. Fernández-Lázaro D, Hernández JLG, García AC, del Castillo AC, Hueso MV, Cruz-Hernández JJ. Clinical perspective and translational oncology of liquid biopsy. Diagnostics 2020;10:443.
28. Reiter RJ, Rosales-Corral SA, Tan DX, et al. Melatonin, a Full service anti-cancer agent: inhibition of initiation, progression and metastasis. Int J Mol Sci 2017;18:843.
29. Hill SM, Belancio VP, Dauchy RT, et al. Melatonin: an inhibitor of breast cancer. Endocr Relat Cancer 2015;22:R183-204.
30. Li Y, Li S, Zhou Y, et al. Melatonin for the prevention and treatment of cancer. Oncotarget 2017;8:39896-921.
31. de Almeida Chuffa LG, Carvalho RF, Justulin LA, et al. A meta-analysis of microRNA networks regulated by melatonin in cancer: Portrait of potential candidates for breast cancer treatment. J Pineal Res 2020;69:e12693.
32. Bondy SC, Campbell A. Mechanisms underlying tumor suppressive properties of melatonin. Int J Mol Sci 2018;19:2205.
34. Bhattacharya S, Patel KK, Dehari D, Agrawal AK, Singh S. Melatonin and its ubiquitous anticancer effects. Mol Cell Biochem 2019;462:133-55.
35. Hill SM, Blask DE. Effects of the pineal hormone melatonin on the proliferation and morphological characteristics of human breast cancer cells (MCF-7) in culture. Cancer Res 1988;48:6121-6.
36. Blask DE, Hill SM. Effects of melatonin on cancer: studies on MCF-7 human breast cancer cells in culture. J Neural Transm Suppl 1986;21:433-49.
37. Önder GÖ, Sezer G, Özdamar S, Yay A. Melatonin has an inhibitory effect on MCF-7 and MDA-MB-231 human breast cancer cell lines by inducing autophagy and apoptosis. Fundam Clin Pharmacol 2022;36:1038-56.
38. Liu L, Zhu Y, Xu Y, et al. Melatonin delays cell proliferation by inducing G1 and G2 /M phase arrest in a human osteoblastic cell line hFOB 1.19. J Pineal Res 2011;50:222-31.
39. Gil-Perotin S, Haines JD, Kaur J, et al. Roles of p53 and p27(Kip1) in the regulation of neurogenesis in the murine adult subventricular zone. Eur J Neurosci 2011;34:1040-52.
40. Mediavilla MD, Cos S, Sánchez-Barceló EJ. Melatonin increases p53 and p21WAF1 expression in MCF-7 human breast cancer cells in vitro. Life Sci 1999;65:415-20.
41. Blask DE, Sauer LA, Dauchy RT. Melatonin as a chronobiotic/anticancer agent: cellular, biochemical, and molecular mechanisms of action and their implications for circadian-based cancer therapy. Curr Top Med Chem 2002;2:113-32.
42. Vriend J, Reiter RJ. Breast cancer cells: modulation by melatonin and the ubiquitin-proteasome system - a review. Mol Cell Endocrinol 2015;417:1-9.
43. Rodriguez C, Martín V, Herrera F, et al. Mechanisms involved in the pro-apoptotic effect of melatonin in cancer cells. Int J Mol Sci 2013;14:6597-613.
44. Morsali S, Sabahi Z, Kakaei J, et al. Clinical efficacy and safety of melatonin supplementation in multiple sclerosis: a systematic review. Inflammopharmacology 2023;31:2213-20.
45. Nag S, Qin J, Srivenugopal KS, Wang M, Zhang R. The MDM2-p53 pathway revisited. J Biomed Res 2013;27:254-71.
46. Mediavilla MD, Sanchez-Barcelo EJ, Tan DX, Manchester L, Reiter RJ. Basic mechanisms involved in the anti-cancer effects of melatonin. Curr Med Chem 2010;17:4462-81.
47. Proietti S, Cucina A, Minini M, Bizzarri M. Melatonin, mitochondria, and the cancer cell. Cell Mol Life Sci 2017;74:4015-25.
48. Mortezaee K, Potes Y, Mirtavoos-Mahyari H, et al. Boosting immune system against cancer by melatonin: a mechanistic viewpoint. Life Sci 2019;238:116960.
49. Anisimov VN, Popovich IG, Zabezhinski MA, Anisimov SV, Vesnushkin GM, Vinogradova IA. Melatonin as antioxidant, geroprotector and anticarcinogen. Biochim Biophys Acta 2006;1757:573-89.
50. Gonzalez A, Cos S, Martinez-Campa C, et al. Selective estrogen enzyme modulator actions of melatonin in human breast cancer cells. J Pineal Res 2008;45:86-92.
51. Cos S, González A, Martínez-Campa C, Mediavilla MD, Alonso-González C, Sánchez-Barceló EJ. Estrogen-signaling pathway: a link between breast cancer and melatonin oncostatic actions. Cancer Detect Prev 2006;30:118-28.
52. Menéndez-Menéndez J, Martínez-Campa C. Melatonin: an anti-tumor agent in hormone-dependent cancers. Int J Endocrinol 2018;2018:3271948.
53. del Río B, García Pedrero JM, Martínez-Campa C, Zuazua P, Lazo PS, Ramos S. Melatonin, an endogenous-specific inhibitor of estrogen receptor alpha via calmodulin. J Biol Chem 2004;279:38294-302.
54. Pedrero JM, Del Rio B, Martínez-Campa C, Muramatsu M, Lazo PS, Ramos S. Calmodulin is a selective modulator of estrogen receptors. Mol Endocrinol 2002;16:947-60.
55. Alvarez-García V, González A, Alonso-González C, Martínez-Campa C, Cos S. Melatonin interferes in the desmoplastic reaction in breast cancer by regulating cytokine production. J Pineal Res 2012;52:282-90.
56. Leon-Blanco MM, Guerrero JM, Reiter RJ, Calvo JR, Pozo D. Melatonin inhibits telomerase activity in the MCF-7 tumor cell line both in vivo and in vitro. J Pineal Res 2003;35:204-11.
57. Martínez-Campa CM, Alonso-González C, Mediavilla MD, Cos S, González A, Sanchez-Barcelo EJ. Melatonin down-regulates hTERT expression induced by either natural estrogens (17beta-estradiol) or metalloestrogens (cadmium) in MCF-7 human breast cancer cells. Cancer Lett 2008;268:272-7.
58. Rodríguez-Santana C, Florido J, Martínez-Ruiz L, López-Rodríguez A, Acuña-Castroviejo D, Escames G. Role of melatonin in cancer: effect on clock genes. Int J Mol Sci 2023;24:1919.
59. Alberts B, Johnson A, Lewis J, et al. Cell junctions. In: Molecular biology of the cell. 4th edition. New York: Garland Science; 2002. Available from: https://www.ncbi.nlm.nih.gov/books/NBK26857/ [Last accessed on 2 Apr 2024].
60. Fernández-Lázaro D, García Hernández JL, García AC, Córdova Martínez A, Mielgo-Ayuso J, Cruz-Hernández JJ. Liquid biopsy as novel tool in precision medicine: origins, properties, identification and clinical perspective of cancer's biomarkers. Diagnostics 2020;10:215.
61. Cos S, Fernandez R, Güezmes A, Sánchez-Barceló EJ. Influence of melatonin on invasive and metastatic properties of MCF-7 human breast cancer cells. Cancer Res 1998;58:4383-90.
62. Su SC, Hsieh MJ, Yang WE, Chung WH, Reiter RJ, Yang SF. Cancer metastasis: mechanisms of inhibition by melatonin. J Pineal Res 2017;62:e12370.
63. Goradel NH, Asghari MH, Moloudizargari M, Negahdari B, Haghi-Aminjan H, Abdollahi M. Melatonin as an angiogenesis inhibitor to combat cancer: mechanistic evidence. Toxicol Appl Pharmacol 2017;335:56-63.
64. Reiter RJ, Sharma R, Ma Q, Rorsales-Corral S, de Almeida Chuffa LG. Melatonin inhibits Warburg-dependent cancer by redirecting glucose oxidation to the mitochondria: a mechanistic hypothesis. Cell Mol Life Sci 2020;77:2527-42.
65. Fernández-Lázaro D, Fernández-Lázaro CI, Caballero García A, Córdova Martínez A. [Immunomodulator drugs for the treatment of multiple myeloma]. Rev Med Chil 2018;146:1444-51.
66. Lissoni P, Barni S, Tancini G, et al. Clinical study of melatonin in untreatable advanced cancer patients. Tumori 1987;73:475-80.
68. Kim C, Kim N, Joo H, et al. Modulation by melatonin of the cardiotoxic and antitumor activities of adriamycin. J Cardiovasc Pharmacol 2005;46:200-10.
69. Lissoni P, Barni S, Mandalà M, et al. Decreased toxicity and increased efficacy of cancer chemotherapy using the pineal hormone melatonin in metastatic solid tumour patients with poor clinical status. Eur J Cancer 1999;35:1688-92.
70. Lissoni P, Chilelli M, Villa S, Cerizza L, Tancini G. Five years survival in metastatic non-small cell lung cancer patients treated with chemotherapy alone or chemotherapy and melatonin: a randomized trial. J Pineal Res 2003;35:12-5.
71. Thomas CR Jr, Reiter RJ, Herman TS. Melatonin: from basic research to cancer treatment clinics. J Clin Oncol 2002;20:2575-601.
72. Mukhopadhyay ND, Khorasanchi A, Pandey S, et al. Melatonin supplementation for cancer-related fatigue in patients with early stage breast cancer receiving radiotherapy: a double-blind placebo-controlled trial. Oncologist 2024;29:e206-12.
73. Berk L, Berkey B, Rich T, et al. Randomized phase II trial of high-dose melatonin and radiation therapy for RPA class 2 patients with brain metastases (RTOG 0119). Int J Radiat Oncol Biol Phys 2007;68:852-7.
74. Del Fabbro E, Dev R, Hui D, Palmer L, Bruera E. Effects of melatonin on appetite and other symptoms in patients with advanced cancer and cachexia: a double-blind placebo-controlled trial. J Clin Oncol 2013;31:1271-6.
75. Kartini D, Taher A, Panigoro SS, et al. Effect of melatonin supplementation in combination with neoadjuvant chemotherapy to miR-210 and CD44 expression and clinical response improvement in locally advanced oral squamous cell carcinoma: a randomized controlled trial. J Egypt Natl Canc Inst 2020;32:12.
76. Lund Rasmussen C, Klee Olsen M, Thit Johnsen A, et al. Effects of melatonin on physical fatigue and other symptoms in patients with advanced cancer receiving palliative care: a double-blind placebo-controlled crossover trial. Cancer 2015;121:3727-36.
77. Palmer ACS, Souza A, Dos Santos VS, et al. The effects of melatonin on the descending pain inhibitory system and neural plasticity markers in breast cancer patients receiving chemotherapy: randomized, double-blinded, placebo-controlled trial. Front Pharmacol 2019;10:1382.
78. Seely D, Legacy M, Auer RC, et al. Adjuvant melatonin for the prevention of recurrence and mortality following lung cancer resection (AMPLCaRe): a randomized placebo controlled clinical trial. EClinicalMedicine 2021;33:100763.
79. Farjallah MA, Hammouda O, Ben Mahmoud L, et al. Melatonin supplementation ameliorates oxidative stress, antioxidant status and physical performances recovery during a soccer training camp. Biol Rhythm Res 2020;51:441-52.
80. Leonardo-Mendonça RC, Ocaña-Wilhelmi J, de Haro T, et al. The benefit of a supplement with the antioxidant melatonin on redox status and muscle damage in resistance-trained athletes. Appl Physiol Nutr Metab 2017; 42:700-7.
81. European Food Safety Authority. Scientific opinion on the supplement of a health claim related to melatonin and reduction of sleep onset latency (ID 1698, 1780, 4080) pursuant to Article 13(1) of Regulation (EC) No 1924/2006. Available from: https://www.efsa.europa.eu/en/efsajournal/pub/2241 [Last accessed on 2 Apr 2024].
82. Spanish Agency for Medicines and Health Products. The Spanish agency for medicines and health products withdraws the food supplement melatonin 3 mg capsules. Available from: https://www.aemps.gob.es/informa/la-aemps-retira-el-complemento-alimenticio-melatonin-3-mg-capsulas/ [Last accessed on 2 Apr 2024].
83. Williams WP 3rd, McLin DE 3rd, Dressman MA, Neubauer DN. Comparative review of approved melatonin agonists for the treatment of circadian rhythm sleep-wake disorders. Pharmacotherapy 2016;36:1028-41.
84. Li X, He J, Li X, Li Y, Zhou Y, Cai S. Neu-P11 - a novel melatonin receptor agonist, could improve the features of type-2 diabetes mellitus in rats. Endokrynol Pol 2021;72:634-42.
85. Zlotos DP, Jockers R, Cecon E, Rivara S, Witt-Enderby PA. MT1 and MT2 melatonin receptors: ligands, models, oligomers, and therapeutic potential. J Med Chem 2014;57:3161-85.
86. Anim-Koranteng C, Shah HE, Bhawnani N, et al. Melatonin-a new prospect in prostate and breast cancer management. Cureus 2021;13:e18124.
87. Gatti G, Lucini V, Dugnani S, et al. Antiproliferative and pro-apoptotic activity of melatonin analogues on melanoma and breast cancer cells. Oncotarget 2017;8:68338-53.
88. Slominski AT, Semak I, Fischer TW, et al. Metabolism of melatonin in the skin: why is it important? Exp Dermatol 2017;26:563-8.
89. Bocheva G, Slominski RM, Janjetovic Z, et al. Protective role of melatonin and its metabolites in skin aging. Int J Mol Sci 2022;23:1238.
Cite This Article
Export citation file: BibTeX | EndNote | RIS
OAE Style
Fernández-Lázaro D, Celorrio San Miguel AM, Roche E. The role of melatonin as an adjuvant therapeutic strategy in the modulation of carcinogenesis. A narrative review. J Cancer Metastasis Treat 2024;10:15. http://dx.doi.org/10.20517/2394-4722.2024.05
AMA Style
Fernández-Lázaro D, Celorrio San Miguel AM, Roche E. The role of melatonin as an adjuvant therapeutic strategy in the modulation of carcinogenesis. A narrative review. Journal of Cancer Metastasis and Treatment. 2024; 10: 15. http://dx.doi.org/10.20517/2394-4722.2024.05
Chicago/Turabian Style
Diego Fernández-Lázaro, Ana M. Celorrio San Miguel, Enrique Roche. 2024. "The role of melatonin as an adjuvant therapeutic strategy in the modulation of carcinogenesis. A narrative review" Journal of Cancer Metastasis and Treatment. 10: 15. http://dx.doi.org/10.20517/2394-4722.2024.05
ACS Style
Fernández-Lázaro, D.; Celorrio San Miguel AM.; Roche E. The role of melatonin as an adjuvant therapeutic strategy in the modulation of carcinogenesis. A narrative review. J. Cancer. Metastasis. Treat. 2024, 10, 15. http://dx.doi.org/10.20517/2394-4722.2024.05
About This Article
Special Issue
Copyright
Data & Comments
Data
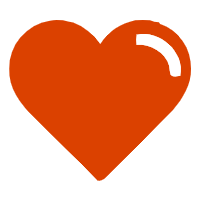
Comments
Comments must be written in English. Spam, offensive content, impersonation, and private information will not be permitted. If any comment is reported and identified as inappropriate content by OAE staff, the comment will be removed without notice. If you have any queries or need any help, please contact us at support@oaepublish.com.