Diagnostic, prognostic, and therapeutic implications of genetic profiling in pleural mesothelioma
Abstract
Pleural mesothelioma (PM) is an aggressive malignancy of the pleural lining that typically arises secondary to asbestos exposure. With the advent of next-generation sequencing, major progress has been made in the molecular characterization of pleural mesothelioma over the past three decades. However, these advances have been largely unable to identify effective targeted therapies for PM. Additionally, there remains an absence of accepted gold-standard consensus for staging and treatment, which partly explains the overall poor outcomes in patients with PM. In recent years, genetic profiling of PM tumors has proved to be an effective tool in the diagnosis and prognosis of PM. Genomic sequencing has identified several potential targets for the development of novel therapeutics in PM. This review summarizes the progress in diagnosis, prognosis, and therapeutics derived by genomics and tumor profiling of PM tumors.
Keywords
INTRODUCTION
Pleural mesothelioma (PM) is a rare but aggressive malignancy that arises from the mesothelial cells of lung pleural lining. PM is most classically associated with prior asbestos exposure, though it has also been linked to ionizing radiation, other non-asbestos fibers, and genetic factors[1,2]. PM affects approximately 3,200 new patients per year in the United States and all comers have a median survival of about 8 months depending on treatment, stage, and histology[2-4]. The global incidence rates have been reported to be between 1-30 cases per million persons, likely secondary to regional differences in industrialization, mining history, and asbestos use[5]. Clinical outcomes in PM are related to certain patient and tumor characteristics. Favorable patient factors for overall survival include female sex, histological subtype, early stage, smaller tumor volume, good nutritional status, and younger age at diagnosis[2,6,7]. At the molecular level, lower expression of specific genes such as BRCA1-associated protein (BAP1), myosin heavy chain 10 (MYH10), Ras homolog family member A (RHOA) were correlated with shorter overall survivals in non-epithelioid PM histology[6]. Germline mutations in BAP1 have been associated with a hereditary predisposition to the development of PM[2].
In the last decade, significant efforts have been made in the molecular characterization of PM. Next-generation sequencing technologies have been applied to improve diagnosis and identify patients who can benefit from specific treatments[3,8-11]. Herein, we describe the diagnostic, prognostic, and therapeutic implications of recent advances in PM genomics and tumor profiling.
PM ORIGIN AND CLASSIFICATION
The pathophysiological process for the development and progression of PM is thought to be due to chronic pleural inflammation (most often caused by asbestos exposure), which facilitates the malignant transformation of the pleura[10]. The asbestos fibers reach the pleural lining via lymphatics, where they remain for months or years. This leads to a chronic inflammatory state, associated with activation of the high mobility group protein B1 (HMGB1), the activating nuclear factor kappa-light-chain enhancer of activated B cells (NF-kB) and the phosphatidylinositol 3-kinase (PI3K) pathways[2]. These inflammatory pathways are thought to drive the proliferation of the pleural mesothelial cells, leading to their malignant transformation by the accumulation of genetic mutations[12].
Mesothelial tumors and proliferations are defined by their level of differentiation and invasiveness. Benign mesothelial tumors include adenomatoid and well-differentiated papillary mesothelial tumors. Recent WHO classification has included mesothelioma in situ within the category of benign proliferation[13]. The diagnosis of mesothelioma in situ requires BAP1 loss by immunohistochemistry (IHC) or homozygous cyclin-dependent kinase inhibitor 2A (CDKN2A) deletion by fluorescent in situ hybridization (FISH). Though progression is typically slow, it is estimated that up to 70% of mesothelioma in situ will progress to invasive malignant mesothelioma[13].
Malignant PM is classified into three major subtypes based on tumor histology. Epithelioid, the most common subtype (~60% of cases), contains ≥ 90% epithelial-shaped cells. Conversely, sarcomatoid, the rarest subtype (≤ 20% of cases), has ≥ 90% spindle-shaped cells arranged in haphazard patterns. Finally, the biphasic subtype consists of both epithelioid and spindle-shaped cells. Epithelioid PM has been associated with longer overall survival (12-27 months), whereas sarcomatoid with shorter overall survival(7-18 months)[10]. In 2018, the National Mesothelioma Audit showed one-year survival of 51%, 28%, and 14% that decreased to 3%, 1%, and 1% after three years for epithelioid, biphasic, and sarcomatoid subtypes, respectively[14]. Some rare histological patterns, including pleomorphic and transitional PM, had previously been grouped by pathologists as epithelioid. However, based on their structural characteristics and transcriptomic profiles, transitional PMs have recently been reclassified as non-epitheliod[13,15].
PM DIAGNOSIS
Clinically, the first symptom of the disease presentation of PM is typically dyspnea secondary to a pleural effusion or chest wall pain[16]. Other common symptoms include dry cough, chest pain, fatigue, and weight loss[2]. Diagnostic workup often starts with drainage and cytological evaluation of the pleural fluid; however, the reported sensitivity of cytological diagnosis ranges between 30%-75%[2,17]. Usually, pathological tissue diagnosis with biopsy via video-assisted thoracoscopic surgery (VATS) or core needle is needed to confirm a diagnosis[16,18]. A single small incision in the line of a potential future thoracotomy is recommended due to the propensity of the tumor to spread into any port sites[16,19]. Tumor invasion must be assessed histologically to definitively diagnose PM. Even so, histologic diagnosis can still present challenges, with multiple patterns described within each histologic subtype[17,18]. In addition, most PM tumors have several histological patterns within a single specimen, indicating an intra-tumor spatial heterogeneity that may not always be captured in the limited volume obtained by biopsy[17]. Sarcomatoid histotype can also pose diagnostic challenges as it can be similar in appearance to normal reactive stroma. The subjective judgment of the component of spindle cells as benign/reactive vs. malignant can make the identification of PM tumors difficult to characterize[2]. Even a surgically-obtained PM biopsy can present diagnostic challenges and require pathologic expertise to diagnose. As such, accurate, objective, and accessible diagnostic tests are still needed for PM.
GENETIC PROFILING IN PM; DIAGNOSIS AND PROGNOSIS
Genetic alterations
The advent of next-generation sequencing (NGS) has allowed for the identification of many relevant genomic, epigenomic, and transcriptomic alterations that are associated with the development of PM[20]. Many of the chromosomal abnormalities associated with PM result in aneuploidy secondary to chromosomal loss or gain mutations. Several studies have identified frequent chromosomal gains in 1q, 5p, 7p, 8q, and 17q and frequent chromosomal losses in 1p, 3p, 4q, 6q, 9p, 13q, 14q, and 22q[1,20-22]. Though nonspecific to PM, these findings have been used to differentiate benign and malignant mesothelial proliferations[20]. Common genetic alterations associated with PM are shown in Table 1.
Common genetic alterations in PM and potential therapeutic targets
Gene name | Chromosomal region | Incidence | Potential therapeutic targets |
BRCA1 Associated Protein 1 (BAP1) | 3p21.1 | 17%-45%[3,107] | EZH2; PARP |
Cyclin-dependent kinase inhibitor 2A (CDKN2A) | 9p21.3 | 48.2%-74%[39,43,107] | MDM2; p53; CDK4/6 |
Methylthioadenosine phosphorylase (MTAP) | 9p21.3 | 74%[43] | Adenylosuccinate synthetase; MAT2A; PRMT |
Tumor Protein P53 (TP53) | 17p13.1 | 7%-19%[3,22,107] | G2-checkpoint; MDM2; p53 |
Neurofibromin 2 (NF2) | 22q12.2 | 16%-32.8%[3,107] | YAP-TEAD; FAK; mTOR and PI3K |
The large tumor suppressor kinase 2 (LATS2) | 13q12.11 | 1%[3] | YAP-TEAD |
Lysine-specific demethylase 4A (KDM4A) | 1p34.2 | 100%[97] | H3K9me3 |
The loss or inactivation of tumor suppression genes, such as BAP1, neurofibromatosis type 2 (NF2), cyclin-dependent kinase inhibitor 2A (CDKN2A), cyclin-dependent kinase inhibitor 2B (CDKN2B), large tumor suppressor kinase 2 (LATS2), and tumor protein 53 (TP53) has also been implicated in the pathogenesis of PM[20,21,23]. To date, there have been no identified oncogenes in the pathogenesis of PM, making it particularly difficult to identify targets for effective therapies[21].
Diagnostic BAP1 and CDKN2A testing
The clinical tests used for the diagnosis of PM include cytological evaluation and histological classification. Though useful, these tests have variable sensitivity and cannot always confirm diagnoses. Immunohistochemistry (IHC) staining has been used to detect the presence or absence of BAP1 expression and support a diagnosis of PM. BAP1 IHC use involves binary evaluation of the presence or absence of BAP1 nuclear staining (defined as positive when tumor cell nuclei show immunoreactivity). The presence of nuclear BAP1 staining is associated with wild-type BAP1, whereas the complete absence of cellular staining correlates with BAP1 biallelic loss[24-26]. Intact nuclear BAP1 staining is more common in sarcomatoid PM[27-29]. The role of cytoplasmic BAP1 in the diagnosis of PM is still controversial and incompletely understood[25,28-38].
FISH has been used clinically in PM diagnosis by detecting homozygous CDKN2A deletion. The frequency of homozygous deletion of CDKN2A in PM tumors has been shown to be 74%[39]. Illei et al. examined cytologically suspicious and confirmed cases of PM and found that FISH was successful in confirming the diagnosis in 12 of 13 cases[40]. In a different study, the combined approach of BAP1 IHC and CDKN2A FISH on a series of 93 PM cases yielded a diagnostic tool with high sensitivity (84%) and specificity (100%)[41]. While highly diagnostic, FISH testing presents challenges to implementation in the clinical setting. It is less widely available, more expensive, and more technically challenging than immunohistochemistry. Also, the translational product of CDKN2A (p16) is not a reliable substitute for CDKN2A FISH[42]. However, deletion of the CDKN2A gene has been correlated with deletion of the methyltioadenosine phosphorylase (MTAP) gene located ~100 kb from the CDKN2A on chromosome 9p21. Since these genes are closely located, they are often simultaneously deleted. It has been shown that IHC staining for MTAP is a reliable surrogate for CDKN2A loss with a sensitivity of 75% and specificity of 95%[42,43].
Gene ratio test in diagnosis and prognosis
Our group has developed an algorithm to translate expression profiles into simple molecular tests with clinical relevancy[44]. Gene expression profiles from a group of tissues are compared with expression profiles of another group distinguishable for a single characteristic such as histology or outcome. Ratios of gene expression are then generated using genes with significantly different levels of expression between the two tissues. This method has been used to distinguish PM from adenocarcinoma using a 6-gene 3-ratios test[45] as well as in the differential diagnosis of PM, where a sequential combination of ratio tests was able to identify PM samples from other thoracic malignancies with high sensitivity and specificity[46]. In addition, the gene ratio test was also successfully applied to determine the histological subtype of PM samples[46].
In the last decade, molecular subtype stratification has proved to be a powerful tool for tumor classification and clinical prognostication. The gene ratio technique has been used to identify patients likely to benefit from tumor resection[44,47,48]. Sixty treatment-naïve frozen PM samples from patients that underwent extrapleural pneumonectomy (EPP) were used to develop a prognostic 4-gene, 3-ratio test (TM4SF1/PKM2, TM4SF1/ARHGDIA, and COBLL1/ARHGDIA). A combined ratio > 1 predicted a good outcome, whereas a combined ratio < 1 predicted a poor outcome [Table 2][47]. A later study showed that the prognostic gene ratio test has robust predictive value and technical assay performance[48]. In addition, a predictive model, the Mesothelioma Prognostic Test (MPT), was developed by investigating the associations of the gene ratio test and pathological predictive parameters (i.e., histology and lymph node status) with survival. The model assigned values of 0 or 1 to each of the three parameters (gene ratio test, histology, and lymph node status). Specifically, values of 0 were assigned to non-epithelioid histology, presence of cancer in a lymph node, and gene ratio test associated with poor prognosis, whereas values of 1 were assigned to epithelial histology, absence of cancer in a lymph node, and gene ratio test associated with good prognosis. The MPT score was calculated for each sample by adding the sum of the three values. Using this algorithm, patients were separated into three distinct risk groups: low risk (MPT = 3, survival 31.9 months), intermediate risk
Prognostic models for PM. This table summarizes the currently available clinical- and molecular-prognostic models of PM, including study cohort and size, variables analyzed, stratification of prognostic groups, and statistical outcomes
Model | Study cohort | Variables | Prognostic groups | Outcomes |
Clinical models | ||||
CALGB, 1998[7] | Treatment naïve PM Pts (n = 337) | ECOG performance status Age Hemoglobin WBC count Chest pain Weight loss | Median survival (95%CI) | |
1 | 13.9 mo (11.1-31.4) | |||
2 | 9.5 mo (CI 6.9-14.7) | |||
3 | 9.2 mo (CI 7.5-10.5) | |||
4 | 6.5 mo (3.7-9.4) | |||
5 | 4.4 mo (3.4-5.1) | |||
6 | 1.4 mo (0.5-3.6) | |||
Australian model, 2016[50] | All PM patients (n = 482) | Weight loss Hemoglobin Serum albumin Histological diagnosis ECOG performance status | Median survival (IQR) | |
1 | 34.0 mo (22.9-47.0) | |||
2 | 17.7 mo (11.6-25.9) | |||
3 | 12.0 mo (6.0-20.6) | |||
4 | 7.4 mo (3.3-11.1) | |||
Molecular models | ||||
Gordon et al., 2003[47] | Surgical EPP patients (n = 46) | Gene ratios KIAA0977/GDIA1 L6-related EST/CTHBP L6-related EST/GDIA1 | Median survival | |
Good-outcome | 36 mo | |||
Poor-outcome | 7 mo | |||
Gordon et al., 2005[108] | Surgical EPP or P/D patients (n = 75) | Gene ratios CD9/KIAA1199 CD9/ THBD DLG5/KIAA1199 DLG5/THBD | Median survival | |
Good-outcome | 12 mo | |||
Poor-outcome | 5 mo | |||
Blum et al., 2019[8] | Surgical PM patients (n = 382) | E-score S-score | Hazard ratio | |
E-score | 1.00 (0.38, 2.64) | |||
S-score | 5.08 (1.56, 16.55) | |||
MESO EMT gene signature[74] | Treatment naïve PM patients (n = 82) | EMT-high EMT-low | Hazard ratio | |
EMT-low | ||||
EMT-high | 2.9 (P = 4.4 × 10-5) | |||
Cioce et al., 2021[76] | Treatment naïve PM patients (n = 84) | 18 - Gene prognostic signature | Hazard ratio | |
Signature-low | ||||
Signature-high | 2.30 [1.71-3.09] (P = 2.8 × 10-8) | |||
Combined Clinical and Molecular Models | ||||
Mesothelioma Prognostic Test (MPT), 2009[48] | Surgical EPP or P/D patients (n = 120) | TM4SF1/PKM2 TM4SF1/ARHGDIA COBLL1/ARHGDIA Histological diagnosis lymph node involvement | Median survival (95%CI) | |
Low risk | 31.9 mo (21.9-41.7) | |||
Intermediate risk | 12.9 mo (9.9-16.4) | |||
High risk | 6.9 mo (2.6 to 8.9) | |||
Mesothelioma risk score (MRiS), 2021[11] | Surgical EPP or P/D patients (n = 384) | Surgery type (P/D vs. EPP) Mesothelioma Prognostic test (MPT) CLDN15/VIM score Tumor volume Neutrophil-to-lymphocyte ratio serum albumin ECOG performance status | Median survival | |
EPP | ||||
Low risk | 39 mo | |||
Intermediate risk | 21 mo | |||
High high | 12 mo | |||
P/D | ||||
Low risk | 36 mo | |||
Intermediate risk | 14 mo | |||
High risk | 9 mo |
MicroRNAs in diagnosis and prognosis
MicroRNAs (miRNAs) are a class of non-coding RNA with an average length of 22 nucleotides. MiRNAs have been shown to regulate gene expression[56]. They are critical for a variety of biological processes and their altered expression has been associated with cancer, where they can act as oncogenes or tumor suppressor genes[57]. A specific combination of miRNAs is associated with each cancer; therefore, specific miRNA signatures may be used for diagnosis, patient stratification, and prognosis[58-62].
Recent studies have found diagnostic miRNAs that are upregulated or downregulated in circulation, tumor tissue, and pleural effusion of patients with PM[63]. MiRNA were investigated as biomarkers to differentiate PM from reactive mesothelial proliferations (RMPs)[64]. Formalin-fixed, paraffin-embedded (FFPE) preoperative diagnostic biopsy tumor specimens and corresponding non-neoplastic pleural samples from 5 patients with PM were used to analyze an array of 742 miRNAs. A four-miRNA (miR-126, miR-143, miR-145, and miR-652) signature able to differentiate between PM and the non-neoplastic controls was identified and consequently validated in an independent cohort of 40 PMs[64]. Other studies have evaluated miRNA expression as a prognostic factor for overall survival and progression-free survival (PFS). Pass et al. found that increased hsa-miR-29c* expression predicted a favorable prognosis[65]. When examined within cell lines, they found that overexpression of hsa-miR-29c* led to decreased tumor cell characteristics associated with invasiveness and malignancy such as proliferation, migration, and colony formation[65]. Busacca et al. evaluated the differential expression of miRNA between normal human mesothelial cell lines and mesothelioma cell lines[66]. Sixty-five microRNAs were found dysregulated using miRNA expression profiles. Selected miRNAs were then investigated in 24 mesothelioma specimens. MiR-17-5p, miR-21, miR-29a, miR-30c, miR-30e-5p, miR-106a, and miR-143 were significantly correlated with PM histological subtype, whereas reduced expression of miR-17-5p and miR-30c was associated with longer survival[66].
“Liquid Biopsies” in diagnosis and prognosis
Due to the difficulties posed by PM diagnosis and the need for invasive procedures to acquire tissue biopsies for complete histopathologic diagnosis, recent efforts have been made to develop targets for “liquid biopsy” from serum, effusion, plasma, serum, or saliva specimens[67]. Typically, the term “liquid biopsy” refers to circulating free tumor DNA (ctDNA) that can be detected in plasma samples, though it can refer to the measurement of soluble biomarkers such as tumor markers and tumor-specific proteins[68].
MiRNA
Circulating serum miRNA in peripheral blood samples has also been evaluated as a diagnostic tool for PM. Cavalleri et al. evaluated circulating exosomal miRNA in peripheral blood from PM patients and found a specific miRNA signature (miR103, miR-98, miR-148b, miR-744, and miR-30e-3p) highly accurate in differentiating PM patients from asbestos-exposed, non-PM controls[69]. In another study, the TaqMan OpenArray was used to analyze 47 pleural effusion (PE) cells and supernatant from 26 patients with PM, 10 from patients with benign disease, and 11 from patients with adenocarcinoma. A three-miRNA signature (miR-143, miR-210, and miR-200c) was identified that accurately differentiated the PM samples from the others, indicating that the expression of this three-miRNA signature could be used in the diagnosis of PM[70].
Mesothelin
Serum mesothelin is a protein expressed on the cell surface of tumor cells in many malignancies, including PM[71]. Burt et al. explored serum mesothelin as a predictive biomarker of recurrence in PM[71]. They retrospectively reviewed outcomes data of 102 PM patients who underwent surgical resection of PM between 2014-2016 and who had documented preoperative mesothelin values. They found that in PM patients who underwent complete macroscopic resection, there was a significant decrease in postoperative values compared to preoperative values (3.4 ± 4.9 nmol/L vs. 0.8 ± 0.5 nmol/L). They also found that higher levels of preoperative serum mesothelin were associated with poor overall survival. Finally, they showed that in patients with epithelioid PM who underwent macroscopic complete resection, a rise in serum mesothelin was associated with the presence of recurrence (sensitivity = 90%, specificity = 93%)[71]. These findings suggest a clinical role of mesothelin surveillance in the postoperative setting as an indicator of disease progression and a possible indicator for recurrence.
Circulating tumor DNA (ctDNA)
Circulating tumor DNA has shown efficacy in detecting malignancy in both patients with lung cancer with EGFR mutations as well as patients with colorectal cancer with KRAS mutations[68]. Though ctDNA assays for other malignancies are in development, there have not been any validated ctDNA assays in PM. Preliminary work in ctDNA assays in PM has failed to show the diagnostic success of ctDNA that is seen in other malignancies. Martinson et al. performed whole exome sequencing (WES) on tumor tissue and matched germline DNA from 11 surgical PM patients[72]. They created a patient-specific ctDNA assay design using multiple tumor regions from each patient to identify variants to compare germline ctDNA. However, only 4 of 11 patients were positive for germline ctDNA using this approach[72]. Similarly,
Molecular clusters-based classification
Unsupervised consensus clustering of bulk-RNA transcriptomes from 216 PM tumors distinguished four distinct molecular clusters of PM: sarcomatoid, epithelioid, biphasic-epithelioid (biphasic-E), and biphasic-sarcomatoid (biphasic-S). These four molecular clusters are approximately correlated with the histological epithelioid to sarcomatoid histological spectrum[3]. Differential gene expression analysis between the two extreme clusters identified 189 upregulated and 241 downregulated genes related to the epithelial-to-mesenchymal transition (EMT) process[3]. In addition, the ratio of the expression of two genes, Claudin 15 and Vimentin (C/V), was able to significantly differentiate each of the four molecular clusters[3].
The Cancer Genome Atlas (TCGA) group performed comprehensive multi-omic molecular profiling on 74 primary tumor samples from treatment-naïve PM patients[22]. Four distinct prognostic clusters significantly associated with overall survival (P = 0.008) were identified[22]. Cluster 1 was associated with longer survival and correlated with epithelioid histology (P = 0.002), whereas cluster 4 was associated with short survival and with the mesenchymal part of the EMT spectrum (P < 0.001)[22].
Blum et al. conducted a comprehensive meta-analysis of mesothelioma profiles from 422 PM tumors from publicly available PM datasets, which included the previously published Bueno and TCGA datasets[8]. Through this analysis, they identified two main groups corresponding to the most extreme clusters that included epithelioid and sarcomatoid samples, respectively. The remaining tumors represented a continuum, or “histo-molecular gradient” between the two extremes. Using a deconvolution approach, they identified two molecular signatures: the E-score and the S-score, which were able to define the proportion of epithelioid-like and sarcomatoid-like components in each tumor. They determined that tumors with high S-scores were correlated with worse overall survival (HR = 6.28, P = 0.001)[8], and that S-score was more prognostic of poor survival than the E-score or histological subtype (HR = 5.08, P = 0.007) [Table 2][8]. This multi-omic meta-analysis confirmed the insights gained from the aforementioned studies, which include the presence of unique histo-molecular clusters within PM, validating the hypothesis of PM existing on an EMT spectrum[21].
Using the TCGA database, Wu et al. developed a 9-gene EMT gene signature to create the MESO EMT score[74]. This 9-gene score showed statistically significant survival differences between the “High” and “Low” groups (Logrank P = 4.4 × 10-5) [Table 2]. They then used single-cell RNA sequencing to analyze pleural effusion and tumor biopsies and found that the 9 EMT genes from their MESO EMT score were predominantly expressed specifically in cancer cells. Additionally, they found an association of high MESO EMT scores with high rates of epigenetic hypermethylation, suggesting that the mesenchymal transition that has been observed in PM may be due to epigenetic signaling[74].
Aldehyde dehydrogenase (ALDH) functions to enzymatically oxidize intracellular aldehydes in many pathological conditions. High ALDH activity has been shown to be associated with chemoresistance in MPM cell lines[75]. Using the TCGA cohort (n = 84), Cioce et al. found that patients with high ALDH1A3 (a member of the ALDH family) had significantly shorter survival compared to low ALDH1A3 patients
TREATMENT
Current clinical practice
PM is behind in terms of consensus and staging for treatments which partly explains the overall poor outcomes. Patients usually get treated regardless of staging in manners that differ among centers and specialists[19]. To define the best standards of treatment, we have identified three different categories important for treatment strategy: stage, patient’s physical status, and tumor biology[19].
To simplify staging, we divide patients into three groups: patients with ipsilateral radiographically resectable tumors without localized invasion of the chest wall and/or mediastinum or mediastinal lymph node involvement; patients with localized disease with chest wall/mediastinal or lymph node involvement, and patients with distant systemic disease[19].
As for physical status, data show that nutritional status measured by albumin, frailty, age, chest pain, chest contraction, and hematological status (platelets, WBC, and neutrophil-to-lymphocyte ratios) can correlate both with stage and survival[11]. Finally, even when all of these parameters are kept constant, specific tumor biology evident by histological subtypes, sex, mutational status, and transcriptional status predict both responses to therapies and outcomes.
Current treatment strategies employ a multimodal approach, including surgery, systemic therapy, and radiotherapy[77]. Treatment is guided by staging, histological subtype, and functional status[77]. These treatments have been shown to be beneficial only to certain populations of highly selected patients[10,77,78]. Current studies have yet to stratify patients that would most benefit from various treatment strategies.
For surgical candidates, macroscopic complete resection through pleurectomy and decortication or extrapleural pneumonectomy is pursued, and in a highly selected subset of patients with early-stage disease, pleurectomy has been associated with improved 5-year survival at 22%[79]. However, these operations cannot always completely address the local microscopic disease spillage[78]. As such, some centers employ cisplatin-based intracavitary heated chemotherapy or other modalities to enhance local control at the time of surgery. Although no randomized clinical trials have been performed, several studies suggest benefits in overall survival[80].
Recently, clinical trials have shown survival benefits of immunotherapy treatment in selected patients. Programmed cell death receptor 1 (PD-1) and its ligand (PDL-1) are expressed in activated immune cells. The expression of these proteins has been shown to play an important role in immune evasion by tumor cells[81]. The phase III CheckMate 743 trial (NCT02899299) published 3-year clinical outcomes data for immunotherapy vs. chemotherapy[82]. They enrolled 605 previously untreated and unresectable PM patients and compared immunotherapy with nivolumab (an anti-PD-1 antibody)/ipilimumab (an anti-CTLA-4 antibody) (n = 303) vs. chemotherapy with cisplatin/pemetrexed (n = 302). They found that median overall survival was greater in the immunotherapy group (18.1 vs. 14.1 months; HR: 95%CI = 0.73 (0.61-0.87)). Subgroup analysis revealed that the survival benefit was mostly seen in the non-epithelioid histotypes[82]. The results from the PrE0505 clinical trial showed that a combination of durvalumab (an anti-PD-L1 antibody) and platinum-pemetrexed chemotherapy led to superior overall survival compared to historical controls that received cisplatin/pemetrexed alone (20.4 vs. 12.1 months)[83]. Recent data has indicated a potential use for immunotherapy and anti-PD-L1 immune checkpoint blockade (ICB) via durvalumab ± tremelimumab (an anti-CTLA-4 antibody) in a neoadjuvant setting[84]. In a phase II, randomized, prospective clinical trial (MPM; NCT02592551), surgically resectable PM patients were randomized to ICB combination therapy with durvalumab + tremelimumab (n = 11), monotherapy with durvalumab alone
The key pharmaceutical trials and their therapeutic pathways for PM are shown in Table 3 and Figure 1. Though these trials show promising progress towards novel first and second-line treatments, there remain no reliable molecular or genetic biomarkers to predict optimal treatment response[77,85].
Figure 1. Commonly mutated pathways in PM and pharmaceutical targeted agents. Adapted with permission[109] and created with BioRender.com. CDKN2A: Cyclin-dependent kinase inhibitor 2A; CDK4: cyclin-dependent kinase 4; CDK6: cyclin-dependent kinase 6; Rb: retinoblastoma protein; NF2: neurofibromin 2; FAK: focal adhesion kinase; mTOR1: mammalian target of rapamycin complex 1; KDM4A: lysine-specific demethylase 4A; ERK: extracellular signal-regulated kinase; BAP1: BRCA1 associated protein 1; EZH2: enhancer of zeste 2 polycomb repressive complex 2 subunit; H3K27: lysine 27 on histone H3; PARP: poly [ADP-ribose] polymerase)
Key pharmaceutical trials and targeted pathways
Author | Phase | Design | Pathway | Drug [mechanism] | Results |
Fennell et al.[90] | II | Single-arm, open-label (n = 36) | BAP1/BRCA1 | Rucaparib [PARPi] | Disease control at 12 week = 58% [95%CI: 37-77] |
Zauderer et al.[89] | II | Single-arm, open-label (n = 74) | BAP1/EZH2 | Tazemetostat [EZH2i] | Disease control at 12 week = 51% [95%CI: 40-63] |
Fennell et al.[95] | II | Randomized, double-blind (n = 344) | NF2/Merlin | Defactinib [FAKi] | Progression-free survival = 4.1 mo [95%CI: 2.9-5.6] |
Fennell et al.[92] | II | Single-arm, open-label (n = 26) | CDKN2A | Abemaciclib [CDK4i/CDK6i] | Disease control at 12 week = 54% [95%CI: 36-71] |
Ali et al.[91] | II | Single-arm, open label | BAP1/BRCA1 | Niraparib [PARPi] | Ongoing |
Lapidot et al.[97] | Preclinical | Xenografted mouse model of PM | KDM4A | PKF118-310 [KDM4Ai] | Preclinical |
Therapeutic implications of genetic profiling
In many cancers, targeted therapy has been effective in treating highly selected patient populations by targeting known unique molecular markers. The lack of specific and feasible molecular targets presents a unique challenge for the development of targeted therapies in PM[86]. Few targeted drug trials have been conducted on genes known to be altered in PM.
BAP1 signaling pathway has been evaluated as a potential target for novel therapies. BAP1 loss has been associated with increased levels of the enhancer of zeste 2 polycomb repressive complex 2 subunit (EZH2), a methyltransferase that tri-methylates lysine 27 on histone H3 (H3K27), altering downstream gene expression[87,88]. In a phase 2 clinical trial, Zauderer et al. evaluated tazemetostat, an EZH2 inhibitor, in 74 PM patients refractory to first-line chemotherapy, 73 of whom had BAP1-inactivated tumors[89]. The absence of BAP1 expression was confirmed by DNA sequencing and IHC of tumor specimens. Results of the trial showed that the disease control rate was 51% at 12 weeks (95%CI: 40-63; 38 of 74 patients) and 28% at 24 weeks (18-39; 21 of 74)[89]. No patients reached complete response, and only two patients had confirmed partial response based on Response Evaluation Criteria in Solid Tumors (RECIST) criteria[89]. Currently, several PARP inhibitors are in early-phase clinical trials[87]. In a phase II single-armed study, patients with relapsed mesothelioma were treated with PARPi (rucaparib) monotherapy. Thirty-six patients were included in the study; 66% had confirmed BAP1 deficiency, 57% were BRCA1 deficiency, and 29% were double deficient in BAP1 and BRCA1. A total of 26 patients who had BAP1 and/or BRCA1 deficiency were eligible for treatment with rucaparib. The primary endpoint was disease control at 12 weeks, which was achieved in 15 patients (58% [95%CI: 37-77]). Six patients reached 24 weeks (23% [9-44]). None of the treated patients achieved a complete response, and only three patients (12%) achieved a partial response[90]. Niraparib, another PARPi, is currently in a phase II clinical trial to evaluate the response of PM patients with and without BAP1 mutations[91].
Similarly, the CDKN2A pathway has been evaluated as a potential target for novel therapies. P16ink4A is a transcriptional product of the CDKN2A pathway, which is an inhibitor of CDK4 and CDK6, which regulate cell cycle progression and promote tumor growth[92]. In a phase 2, single-armed clinical trial, Fennell et al. studied the effects of abemaciclib, a CDK4i/CDK6i, on p16ink4A-deficient PM patients[92]. All patients had completed at least one cycle of cisplatin-based therapy and were molecularly screened for p16ink4A deficiency prior to inclusion in this study. They found a disease control rate of 54% (14 of 26 patients) at 12 weeks (95%CI: 36-71), with 80% of patients showing a reduction in tumor volume[92].
The tumor suppressor gene NF2, located on 22q12, is a plasma membrane-associated inhibitor of proliferation[93]. Mutations in NF2 are associated with many benign and malignant tumors including PM[22,93]. Large bulk RNA-sequencing studies have shown NF2 mutation rates are particularly high in PM tumors with sarcomatoid histotype[3]. Loss of function mutations in NF2 has been shown to dysregulate the Hippo signaling pathway, which is commonly altered in PM[3,86]. NF2 encodes for moesin-ezrin-radixin-like protein (merlin), a tumor-suppressor protein that recruits LATS1 and LATS2, leading to the phosphorylation of downstream targets yes-associated protein 1 (YAP1) and WW Domain-Containing Transcription Regulator 1 (TAZ), preventing their translocation into the nucleus and, ultimately, leading to upregulation of many oncogenic genes[86,93]. The focal adhesion Kinase (FAK) is a serine/threonine of the Hippo pathway, involved in cell growth and migration. FAK is elevated in many human cancers[93]. In cell-line and in vivo mouse experiments, Shapiro et al. investigated the sensitivity of merlin-deficient PM to FAK inhibition and observed that FAK inhibition led to decreased tumor growth[94]. In the phase II double-blind placebo control COMMAND trial, the FAK inhibitor (Defactinib) was investigated as maintenance therapy to improve progression-free survival. Three hundred forty-four patients with PM who had completed one cycle of first-line chemotherapy (cisplatin/pemetrexed) were randomized to receive defactinib (n = 173,) vs. placebo (n = 171)[95]. No differences in median PFS were observed in the defactinib arm (4.1 months;
Lysine-specific demethylase 4A (KDM4A), a gene overexpressed in PM, regulates the demethylation of histone 3 lysine 9 trimethylation (H3K9me3), a post-translational modification that is involved in several biological processes, including forming transcriptionally silent heterochromatin[97]. In a preclinical model, Lapidot et al. examined KDM4A as a potential target for PM treatment[97]. Using tissue microarrays from
MicroRNAs in PM treatment
As previously described, miRNAs have shown diagnostic and prognostic utility in the management of PM. However, recent studies have begun to explore their potential role as therapeutics in PM. Several studies have targeted various miRNAs in mouse models and shown that miRNA replacement can inhibit tumor growth[98-100]. In preclinical models, Tomasetti et al. subcutaneously xenografted mice with cell lines that were transfected with miRNA-126 plasmids (Met5AMiR126 and H28MiR126) vs. empty plasmids (Met5ApCMV-MiR and H28pCMV-MiR) to show that miRNA-126 played a role in mitochondrial metabolism and tumor suppression[99]. Later, Monaco et al. developed a strategy for targeted delivery of miRNA-126 to PM cell lines using endogenous, epithelial-derived exosomal vesicles[101]. They used fibroblast (IMR-90), endothelial (HUVECs), nonmalignant mesothelial (Met-5A), and PM (H28 and MM-B1) cell lines to create an in vitro tri-culture stromal model. They then treated these models with exosomes enriched with miRNA-126. They found stromal miRNA-126 uptake via paracrine and exocrine transport, which was dependent on the miRNA-126 levels in the cellular microenvironment (miRNA-126 sensitive vs. miRNA-126 resistant). Additionally, they found that treatment with miRNA-126 enriched vesicles inhibited angiogenesis in miRNA-126 sensitive PM cells[101]. Though early in the preclinical phase, these studies show examples of novel strategies for targeted therapies in the treatment of PM[100].
Similarly, miRNA-15 and miRNA-16 have been shown to be dysregulated in many solid tumors[102,103]. They target the mRNA of many genes associated with tumor progression and are thought to act as tumor suppressor genes[104]. Reid et al. showed that miRNA-15 and miRNA-16 are downregulated in PM, suggesting these miRNAs as potential targets for novel treatments[105]. In a single-arm phase 1 clinical trial, Van Zandwijk et al. intravenously treated 26 PM patients with recurrent PM with miRNA-16 - mimic minicells[106]. They evaluated disease progression using follow-up CT scans after at least 4 weeks. Of the 22 patients who had baseline CTs, 5% had partial response (n = 1), 68% had stable disease (n = 15), and 27% had disease progression (n = 6)[104]. Because this study was aimed to evaluate the drug-related toxicities associated with this novel PM therapy, it was not powered to accurately assess treatment efficacy as measured by disease response or overall survival. Nevertheless, this trial represents the first-in-human trial of targeted miRNA therapy for PM. As new miRNA treatments and delivery strategies continue to be explored, the ultimate role of miRNA in the treatment of PM remains to be seen.
CONCLUSION
Omics-based technologies permit the identification of gene signatures based on expression and sequencing data and are essential to classify patients for precision medicine[21]. Genetic profiling has demonstrated its utility by selecting patients and guiding treatment in many malignancies[106]. In PM, the advances in the genomic and transcriptomic characterization have led to a better understanding of the heterogeneity and complexity of this disease, but progress in the treatments of patients with PM is limited[3,13,87]. Further improvements in the analysis of omics data, together with advances in computational technologies, will contribute to the validation of genetic profiling approaches for new applications in PM.
DECLARATIONS
Authors’ contributions
Wrote and revised the text: Sadek A, De Rienzo A, Bueno R
Provided comments on the initial version and approved the final draft of the manuscript: Sadek A, De Rienzo A, Bueno R
Availability of data and materials
Not applicable.
Financial support and sponsorship
Dr. Bueno reports research grants and clinical trial support from Grants from NCI, DOD, NIBIB, and the Swiss National Foundation, as well as grants from Genentech, Roche, Merck, Bicycle Therapeutics, Serum, and Bayer. In addition, Dr. Bueno has four patents through the BWH (no royalties to date) and Equity in Navigation Science and is on the scientific advisory of Novocure.
Conflicts of interest
All authors declared that there are no conflicts of interest.
Ethical approval and consent to participate:
Not applicable.
Consent for publication
Not applicable.
Copyright
© The Author(s) 2023
REFERENCES
1. Maciá S. Mesothelioma: intechopen; 2020. Available from: https://www.intechopen.com/books/10130 [Last accessed on 29 June 2023].
2. Carbone M, Adusumilli PS, Alexander HR Jr, et al. Mesothelioma: scientific clues for prevention, diagnosis, and therapy. CA Cancer J Clin 2019;69:402-29.
3. Bueno R, Stawiski EW, Goldstein LD, et al. Comprehensive genomic analysis of malignant pleural mesothelioma identifies recurrent mutations, gene fusions and splicing alterations. Nat Genet 2016;48:407-16.
4. Lapidot M, Freyaldenhoven S, Bueno R. New concepts in the treatment of malignant pleural mesothelioma. J Thorac Dis 2018;10:1283-5.
5. Bianchi C, Bianchi T. Malignant mesothelioma: global incidence and relationship with asbestos. Ind Health 2007;45:379-87.
6. De Rienzo A, Archer MA, Yeap BY, et al. Gender-specific molecular and clinical features underlie malignant pleural mesothelioma. Cancer Res 2016;76:319-28.
7. Herndon JE, Green MR, Chahinian AP, Corson JM, Suzuki Y, Vogelzang NJ. Factors predictive of survival among 337 patients with mesothelioma treated between 1984 and 1994 by the Cancer and Leukemia Group B. Chest 1998;113:723-31.
8. Blum Y, Meiller C, Quetel L, et al. Dissecting heterogeneity in malignant pleural mesothelioma through histo-molecular gradients for clinical applications. Nat Commun 2019;10:1333.
9. Borchert S, Wessolly M, Schmeller J, et al. Gene expression profiling of homologous recombination repair pathway indicates susceptibility for olaparib treatment in malignant pleural mesothelioma in vitro. BMC Cancer 2019;19:108.
10. Yap TA, Aerts JG, Popat S, Fennell DA. Novel insights into mesothelioma biology and implications for therapy. Nat Rev Cancer 2017;17:475-88.
11. Yeap BY, De Rienzo A, Gill RR, et al. Mesothelioma risk score: a new prognostic pretreatment, clinical-molecular algorithm for malignant pleural mesothelioma. J Thorac Oncol 2021;16:1925-35.
14. Physicians RCo. National mesothelioma audit report 2018 (for the audit period 2014-16). London: Royal College of Physicians; 2018.
15. Galateau Salle F, Le Stang N, Tirode F, et al. Comprehensive molecular and pathologic evaluation of transitional mesothelioma assisted by deep learning approach: a multi-institutional study of the international mesothelioma panel from the MESOPATH reference center. J Thorac Oncol 2020;15:1037-53.
16. Sugarbaker DJ. Sugarbaker’s adult chest surgery. New York: McGraw-Hill Education; 2020.
17. Husain AN, Colby TV, Ordóñez NG, et al. Guidelines for pathologic diagnosis of malignant mesothelioma 2017 update of the consensus statement from the international mesothelioma interest group. Arch Pathol Lab Med 2018;142:89-108.
18. Chirieac LR, Hung YP, Foo WC, et al. Diagnostic value of biopsy sampling in predicting histology in patients with diffuse malignant pleural mesothelioma. Cancer 2019;125:4164-71.
19. Paajanen J, Jaklitsch MT, Bueno R. Contemporary issues in the surgical management of pleural mesothelioma. J Surg Oncol 2023;127:343-54.
20. Jean D, Daubriac J, Le Pimpec-Barthes F, Galateau-Salle F, Jaurand MC. Molecular changes in mesothelioma with an impact on prognosis and treatment. Arch Pathol Lab Med 2012;136:277-93.
21. Severson DT, De Rienzo A, Bueno R. Mesothelioma in the age of “Omics”: before and after The Cancer Genome Atlas. J Thorac Cardiovasc Surg 2020;160:1078-83.e2.
22. Hmeljak J, Sanchez-Vega F, Hoadley KA, et al. Integrative molecular characterization of malignant pleural mesothelioma. Cancer Discov 2018;8:1548-65.
23. Murakami H, Mizuno T, Taniguchi T, et al. LATS2 is a tumor suppressor gene of malignant mesothelioma. Cancer Res 2011;71:873-83.
24. Bott M, Brevet M, Taylor BS, et al. The nuclear deubiquitinase BAP1 is commonly inactivated by somatic mutations and 3p21.1 losses in malignant pleural mesothelioma. Nat Genet 2011;43:668-72.
25. Nasu M, Emi M, Pastorino S, et al. High incidence of somatic BAP1 alterations in sporadic malignant mesothelioma. J Thorac Oncol 2015;10:565-76.
26. Wang LM, Shi ZW, Wang JL, et al. Diagnostic accuracy of BRCA1-associated protein 1 in malignant mesothelioma: a meta-analysis. Oncotarget 2017;8:68863-72.
27. Ascoli V, Cozzi I, Vatrano S, et al. Mesothelioma families without inheritance of a BAP1 predisposing mutation. Cancer Genet 2016;209:381-7.
28. Guo Z, Carbone M, Zhang X, et al. Improving the accuracy of mesothelioma diagnosis in china. J Thorac Oncol 2017;12:714-23.
29. Righi L, Duregon E, Vatrano S, et al. BRCA1-associated protein 1 (BAP1) immunohistochemical expression as a diagnostic tool in malignant pleural mesothelioma classification: a large retrospective study. J Thorac Oncol 2016;11:2006-17.
30. Bhattacharya S, Hanpude P, Maiti TK. Cancer associated missense mutations in BAP1 catalytic domain induce amyloidogenic aggregation: a new insight in enzymatic inactivation. Sci Rep 2015;5:18462.
31. Ventii KH, Devi NS, Friedrich KL, et al. BRCA1-associated protein-1 is a tumor suppressor that requires deubiquitinating activity and nuclear localization. Cancer Res 2008;68:6953-62.
32. Carbone M, Yang H, Pass HI, Krausz T, Testa JR, Gaudino G. BAP1 and cancer. Nat Rev Cancer 2013;13:153-9.
33. Cigognetti M, Lonardi S, Fisogni S, et al. BAP1 (BRCA1-associated protein 1) is a highly specific marker for differentiating mesothelioma from reactive mesothelial proliferations. Mod Pathol 2015;28:1043-57.
34. Cozzi I, Oprescu FA, Rullo E, Ascoli V. Loss of BRCA1-associated protein 1 (BAP1) expression is useful in diagnostic cytopathology of malignant mesothelioma in effusions. Diagn Cytopathol 2018;46:9-14.
35. Farzin M, Toon CW, Clarkson A, et al. Loss of expression of BAP1 predicts longer survival in mesothelioma. Pathology 2015;47:302-7.
36. Hida T, Hamasaki M, Matsumoto S, et al. BAP1 immunohistochemistry and p16 FISH results in combination provide higher confidence in malignant pleural mesothelioma diagnosis: ROC analysis of the two tests. Pathol Int 2016;66:563-70.
37. Hida T, Hamasaki M, Matsumoto S, et al. Immunohistochemical detection of MTAP and BAP1 protein loss for mesothelioma diagnosis: Comparison with 9p21 FISH and BAP1 immunohistochemistry. Lung Cancer 2017;104:98-105.
38. De Rienzo A, Chirieac LR, Hung YP, et al. Large-scale analysis of BAP1 expression reveals novel associations with clinical and molecular features of malignant pleural mesothelioma. J Pathol 2021;253:68-79.
39. Illei PB, Rusch VW, Zakowski MF, Ladanyi M. Homozygous deletion of CDKN2A and codeletion of the methylthioadenosine phosphorylase gene in the majority of pleural mesotheliomas. Clin Cancer Res 2003;9:2108-13.
40. Illei PB, Ladanyi M, Rusch VW, Zakowski MF. The use of CDKN2A deletion as a diagnostic marker for malignant mesothelioma in body cavity effusions. Cancer 2003;99:51-6.
41. McGregor SM, McElherne J, Minor A, et al. BAP1 immunohistochemistry has limited prognostic utility as a complement of CDKN2A (p16) fluorescence in situ hybridization in malignant pleural mesothelioma. Hum Pathol 2017;60:86-94.
42. Chapel DB, Schulte JJ, Berg K, et al. MTAP immunohistochemistry is an accurate and reproducible surrogate for CDKN2A fluorescence in situ hybridization in diagnosis of malignant pleural mesothelioma. Mod Pathol 2020;33:245-54.
43. Chapel DB, Dubuc AM, Hornick JL, Sholl LM. Correlation of methylthioadenosine phosphorylase (MTAP) protein expression with MTAP and CDKN2A copy number in malignant pleural mesothelioma. Histopathology 2021;78:1032-42.
44. Gordon GJ, Jensen RV, Hsiao LL, et al. Translation of microarray data into clinically relevant cancer diagnostic tests using gene expression ratios in lung cancer and mesothelioma. Cancer Res 2002;62:4963-7.
45. Gordon GJ. Transcriptional profiling of mesothelioma using microarrays. Lung Cancer 2005;49 Suppl 1:S99-103.
46. De Rienzo A, Richards WG, Yeap BY, et al. Sequential binary gene ratio tests define a novel molecular diagnostic strategy for malignant pleural mesothelioma. Clin Cancer Res 2013;19:2493-502.
47. Gordon GJ, Jensen RV, Hsiao LL, et al. Using gene expression ratios to predict outcome among patients with mesothelioma. J Natl Cancer Inst 2003;95:598-605.
48. Gordon GJ, Dong L, Yeap BY, et al. Four-gene expression ratio test for survival in patients undergoing surgery for mesothelioma. J Natl Cancer Inst 2009;101:678-86.
49. Abakay O, Tanrikulu AC, Palanci Y, Abakay A. The value of inflammatory parameters in the prognosis of malignant mesothelioma. J Int Med Res 2014;42:554-65.
50. Brims FJ, Meniawy TM, Duffus I, et al. A novel clinical prediction model for prognosis in malignant pleural mesothelioma using decision tree analysis. J Thorac Oncol 2016;11:573-82.
51. Curran D, Sahmoud T, Therasse P, van Meerbeeck J, Postmus PE, Giaccone G. Prognostic factors in patients with pleural mesothelioma: the European Organization for Research and Treatment of Cancer experience. J Clin Oncol 1998;16:145-52.
52. Kao SC, Klebe S, Henderson DW, et al. Low calretinin expression and high neutrophil-to-lymphocyte ratio are poor prognostic factors in patients with malignant mesothelioma undergoing extrapleural pneumonectomy. J Thorac Oncol 2011;6:1923-9.
53. Kao SC, Pavlakis N, Harvie R, et al. High blood neutrophil-to-lymphocyte ratio is an indicator of poor prognosis in malignant mesothelioma patients undergoing systemic therapy. Clin Cancer Res 2010;16:5805-13.
54. Kao SC, Vardy J, Chatfield M, et al. Validation of prognostic factors in malignant pleural mesothelioma: a retrospective analysis of data from patients seeking compensation from the New South Wales Dust Diseases Board. Clin Lung Cancer 2013;14:70-7.
55. Özyürek BA, Özmen Ö, Özdemirel TŞ, Erdoğan Y, Kaplan B, Kaplan T. Relation between neutrophil/lymphocyte ratio and primary tumor metabolic activity in patients with malign pleural mesothelioma. Clin Respir J 2018;12:646-51.
56. O'Brien J, Hayder H, Zayed Y, Peng C. Overview of MicroRNA biogenesis, mechanisms of actions, and circulation. Front Endocrinol 2018;9:402.
57. Sethi S, Ali S, Sethi S, Sarkar FH. MicroRNAs in personalized cancer therapy. Clin Genet 2014;86:68-73.
58. Goto Y, Shinjo K, Kondo Y, et al. Epigenetic profiles distinguish malignant pleural mesothelioma from lung adenocarcinoma. Cancer Res 2009;69:9073-82.
59. Shin VY, Chu KM. MiRNA as potential biomarkers and therapeutic targets for gastric cancer. World J Gastroenterol 2014;20:10432-9.
60. Chandra V, Kim JJ, Mittal B, Rai R. MicroRNA aberrations: an emerging field for gallbladder cancer management. World J Gastroenterol 2016;22:1787-99.
61. Guo J, Miao Y, Xiao B, et al. Differential expression of microRNA species in human gastric cancer versus non-tumorous tissues. J Gastroenterol Hepatol 2009;24:652-7.
62. Guo Y, Chen Z, Zhang L, et al. Distinctive microRNA profiles relating to patient survival in esophageal squamous cell carcinoma. Cancer Res 2008;68:26-33.
63. Han YQ, Xu SC, Zheng WQ, Hu ZD. Diagnostic value of microRNAs for malignant pleural mesothelioma: a mini-review. Thorac Cancer 2021;12:8-12.
64. Andersen M, Grauslund M, Ravn J, Sørensen JB, Andersen CB, Santoni-Rugiu E. Diagnostic potential of miR-126, miR-143, miR-145, and miR-652 in malignant pleural mesothelioma. J Mol Diagn 2014;16:418-30.
65. Pass HI, Goparaju C, Ivanov S, et al. hsa-miR-29c* is linked to the prognosis of malignant pleural mesothelioma. Cancer Res 2010;70:1916-24.
66. Busacca S, Germano S, De Cecco L, et al. MicroRNA signature of malignant mesothelioma with potential diagnostic and prognostic implications. Am J Respir Cell Mol Biol 2010;42:312-9.
67. Cavallari I, Urso L, Sharova E, Pasello G, Ciminale V. Liquid biopsy in malignant pleural mesothelioma: state of the art, pitfalls, and perspectives. Front Oncol 2019;9:740.
68. Merker JD, Oxnard GR, Compton C, et al. Circulating tumor DNA analysis in patients with cancer: american society of clinical oncology and college of american pathologists joint review. Arch Pathol Lab Med 2018;142:1242-53.
69. Cavalleri T, Angelici L, Favero C, et al. Plasmatic extracellular vesicle microRNAs in malignant pleural mesothelioma and asbestos-exposed subjects suggest a 2-miRNA signature as potential biomarker of disease. PLoS One 2017;12:e0176680.
70. Birnie KA, Prêle CM, Musk AWB, et al. MicroRNA signatures in malignant pleural mesothelioma effusions. Dis Markers 2019;2019:8628612.
71. Burt BM, Lee HS, Lenge De Rosen V, et al. Soluble mesothelin-related peptides to monitor recurrence after resection of pleural mesothelioma. Ann Thorac Surg 2017;104:1679-87.
72. Martinson LJ, Sharkey AJ, Dawson AG, et al. Abstract 1349: personalized circulating tumor DNA profiling in malignant pleural mesothelioma. Cancer Res 2019;79:1349.
73. Hylebos M, Op de Beeck K, Pauwels P, Zwaenepoel K, van Meerbeeck JP, Van Camp G. Tumor-specific genetic variants can be detected in circulating cell-free DNA of malignant pleural mesothelioma patients. Lung Cancer 2018;124:19-22.
74. Wu L, Yoshihara K, Yun H, et al. Prognostic value of EMT gene signature in malignant mesothelioma. Int J Mol Sci 2023;24:4264.
75. Canino C, Luo Y, Marcato P, Blandino G, Pass HI, Cioce M. A STAT3-NFkB/DDIT3/CEBPβ axis modulates ALDH1A3 expression in chemoresistant cell subpopulations. Oncotarget 2015;6:12637-53.
76. Cioce M, Sacconi A, Pass HI, et al. Insights into intra-tumoral heterogeneity: transcriptional profiling of chemoresistant MPM cell subpopulations reveals involvement of NFkB and DNA repair pathways and contributes a prognostic signature. Int J Mol Sci 2021;22:12071.
77. Janes SM, Alrifai D, Fennell DA. Perspectives on the treatment of malignant pleural mesothelioma. N Engl J Med 2021;385:1207-18.
78. Friedberg JS, Culligan MJ, Tsao AS, et al. A Proposed system toward standardizing surgical-based treatments for malignant pleural mesothelioma, from the joint national cancer institute-international association for the study of lung cancer-mesothelioma applied research foundation taskforce. J Thorac Oncol 2019;14:1343-53.
79. Lapidot M, Gill RR, Mazzola E, et al. Pleurectomy decortication in the treatment of malignant pleural mesothelioma: encouraging results and novel prognostic implications based on experience in 355 consecutive patients. Ann Surg 2022;275:1212-20.
80. Järvinen T, Paajanen J, Ilonen I, Räsänen J. Hyperthermic intrathoracic chemoperfusion for malignant pleural mesothelioma: systematic review and meta-analysis. Cancers 2021;13:3637.
81. Sanmamed MF, Chen L. Inducible expression of B7-H1 (PD-L1) and its selective role in tumor site immune modulation. Cancer J 2014;20:256-61.
82. Peters S, Scherpereel A, Cornelissen R, et al. First-line nivolumab plus ipilimumab versus chemotherapy in patients with unresectable malignant pleural mesothelioma: 3-year outcomes from CheckMate 743. Ann Oncol 2022;33:488-99.
83. Forde PM, Anagnostou V, Sun Z, et al. Durvalumab with platinum-pemetrexed for unresectable pleural mesothelioma: survival, genomic and immunologic analyses from the phase 2 PrE0505 trial. Nat Med 2021;27:1910-20.
84. Lee HS, Jang HJ, Ramineni M, et al. A phase II window of opportunity study of neoadjuvant PD-L1 versus PD-L1 plus CTLA-4 blockade for patients with malignant pleural mesothelioma. Clin Cancer Res 2023;29:548-59.
85. Baas P, Scherpereel A, Nowak AK, et al. First-line nivolumab plus ipilimumab in unresectable malignant pleural mesothelioma (CheckMate 743): a multicentre, randomised, open-label, phase 3 trial. Lancet 2021;397:375-86.
86. Hinz TK, Heasley LE. Translating mesothelioma molecular genomics and dependencies into precision oncology-based therapies. Semin Cancer Biol 2020;61:11-22.
87. Paajanen J, Bueno R, De Rienzo A. The rocky road from preclinical findings to successful targeted therapy in pleural mesothelioma. Int J Mol Sci 2022;23:13422.
89. Zauderer MG, Szlosarek PW, Le Moulec S, et al. EZH2 inhibitor tazemetostat in patients with relapsed or refractory, BAP1-inactivated malignant pleural mesothelioma: a multicentre, open-label, phase 2 study. Lancet Oncol 2022;23:758-67.
90. Fennell DA, King A, Mohammed S, et al. Rucaparib in patients with BAP1-deficient or BRCA1-deficient mesothelioma (MiST1): an open-label, single-arm, phase 2a clinical trial. Lancet Respir Med 2021;9:593-600.
91. ali A, Deremer DL, Lee J, et al. Phase II trial of the PARP inhibitor, niraparib, in BAP1 and other DNA damage response (DDR) pathway deficient neoplasms (NCT03207347). J Clin Oncol 2020;38:e22061.
92. Fennell DA, King A, Mohammed S, et al. Abemaciclib in patients with p16ink4A-deficient mesothelioma (MiST2): a single-arm, open-label, phase 2 trial. Lancet Oncol 2022;23:374-81.
93. Sato T, Sekido Y. NF2/merlin inactivation and potential therapeutic targets in mesothelioma. Int J Mol Sci 2018;19:988.
94. Shapiro IM, Kolev VN, Vidal CM, et al. Merlin deficiency predicts FAK inhibitor sensitivity: a synthetic lethal relationship. Sci Transl Med 2014;6:237ra68.
95. Fennell DA, Baas P, Taylor P, et al. Maintenance defactinib versus placebo after first-line chemotherapy in patients with merlin-stratified pleural mesothelioma: COMMAND-a double-blind, randomized, phase II study. J Clin Oncol 2019;37:790-8.
96. Knelson EH, Ivanova EV, Tarannum M, et al. Activation of tumor-cell STING primes NK-cell therapy. Cancer Immunol Res 2022;10:947-61.
97. Lapidot M, Case AE, Weisberg EL, et al. Essential role of the histone lysine demethylase KDM4A in the biology of malignant pleural mesothelioma (MPM). Br J Cancer 2021;125:582-92.
98. Birnie KA, Prêle CM, Thompson PJ, Badrian B, Mutsaers SE. Targeting microRNA to improve diagnostic and therapeutic approaches for malignant mesothelioma. Oncotarget 2017;8:78193-207.
99. Tomasetti M, Nocchi L, Staffolani S, et al. MicroRNA-126 suppresses mesothelioma malignancy by targeting IRS1 and interfering with the mitochondrial function. Antioxid Redox Signal 2014;21:2109-25.
100. Monaco F, De Conti L, Vodret S, et al. Force-feeding malignant mesothelioma stem-cell like with exosome-delivered miR-126 induces tumour cell killing. Transl Oncol 2022;20:101400.
101. Monaco F, Gaetani S, Alessandrini F, et al. Exosomal transfer of miR-126 promotes the anti-tumour response in malignant mesothelioma: Role of miR-126 in cancer-stroma communication. Cancer Lett 2019;463:27-36.
102. Bandi N, Zbinden S, Gugger M, et al. miR-15a and miR-16 are implicated in cell cycle regulation in a Rb-dependent manner and are frequently deleted or down-regulated in non-small cell lung cancer. Cancer Res 2009;69:5553-9.
103. Bonci D, Coppola V, Musumeci M, et al. The miR-15a-miR-16-1 cluster controls prostate cancer by targeting multiple oncogenic activities. Nat Med 2008;14:1271-7.
104. van Zandwijk N, Pavlakis N, Kao SC, et al. Safety and activity of microRNA-loaded minicells in patients with recurrent malignant pleural mesothelioma: a first-in-man, phase 1, open-label, dose-escalation study. Lancet Oncol 2017;18:1386-96.
105. Reid G, Pel ME, Kirschner MB, et al. Restoring expression of miR-16: a novel approach to therapy for malignant pleural mesothelioma. Ann Oncol 2013;24:3128-35.
106. El-Deiry WS, Goldberg RM, Lenz HJ, et al. The current state of molecular testing in the treatment of patients with solid tumors, 2019. CA Cancer J Clin 2019;69:305-43.
107. Hiltbrunner S, Fleischmann Z, Sokol ES, Zoche M, Felley-Bosco E, Curioni-Fontecedro A. Genomic landscape of pleural and peritoneal mesothelioma tumours. Br J Cancer 2022;127:1997-2005.
108. Gordon GJ, Rockwell GN, Godfrey PA, et al. Validation of genomics-based prognostic tests in malignant pleural mesothelioma. Clin Cancer Res 2005;11:4406-14.
Cite This Article
Export citation file: BibTeX | RIS
OAE Style
Sadek A, De Rienzo A, Bueno R. Diagnostic, prognostic, and therapeutic implications of genetic profiling in pleural mesothelioma. J Cancer Metastasis Treat 2023;9:26. http://dx.doi.org/10.20517/2394-4722.2023.17
AMA Style
Sadek A, De Rienzo A, Bueno R. Diagnostic, prognostic, and therapeutic implications of genetic profiling in pleural mesothelioma. Journal of Cancer Metastasis and Treatment. 2023; 9: 26. http://dx.doi.org/10.20517/2394-4722.2023.17
Chicago/Turabian Style
Sadek, Ahmed, Assunta De Rienzo, Raphael Bueno. 2023. "Diagnostic, prognostic, and therapeutic implications of genetic profiling in pleural mesothelioma" Journal of Cancer Metastasis and Treatment. 9: 26. http://dx.doi.org/10.20517/2394-4722.2023.17
ACS Style
Sadek, A.; De Rienzo A.; Bueno R. Diagnostic, prognostic, and therapeutic implications of genetic profiling in pleural mesothelioma. J. Cancer. Metastasis. Treat. 2023, 9, 26. http://dx.doi.org/10.20517/2394-4722.2023.17
About This Article
Copyright
Data & Comments
Data
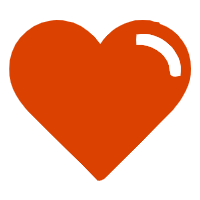

Comments
Comments must be written in English. Spam, offensive content, impersonation, and private information will not be permitted. If any comment is reported and identified as inappropriate content by OAE staff, the comment will be removed without notice. If you have any queries or need any help, please contact us at support@oaepublish.com.