The microbiome and ovarian cancer: insights, implications, and therapeutic opportunities
Abstract
Ovarian cancer is the leading cause of gynecologic cancer death in the United States. Most ovarian cancer patients are diagnosed with advanced-stage disease, which poses a challenge for early detection and effective treatment. At present, cytoreductive surgery and platinum-based chemotherapy are foundational for patients with newly diagnosed ovarian cancer, but unfortunately, most patients will recur and die of their disease. Therefore, there is a significant need to seek innovative, novel approaches for early detection and to overcome chemoresistance for ovarian cancer patients. The microbiome, comprising diverse microbial communities inhabiting various body sites, is vital in maintaining human health. Changes to the diversity and composition of the microbial communities impact the microbiota-host relationship and are linked to diseases, including cancer. The microbiome contributes to carcinogenesis through various mechanisms, including altered host immune response, modulation of DNA repair, upregulation of pro-inflammatory pathways, altered gene expression, and dysregulated estrogen metabolism. Translational and clinical studies have demonstrated that specific microbes contribute to ovarian cancer development and impact chemotherapy’s efficacy. The microbiome is malleable and can be altered through different approaches, including diet, exercise, medications, and fecal microbiota transplantation. This review provides an overview of the current literature regarding ovarian cancer and the microbiome of female reproductive and gastrointestinal tracts, focusing on mechanisms of carcinogenesis and options for modulating the microbiota for cancer prevention and treatment. Advancing our understanding of the complex relationship between the microbiome and ovarian cancer may provide a novel approach for prevention and therapeutic modulation in the future.
Keywords
INTRODUCTION
Ovarian cancer (OC) is the most lethal gynecologic cancer in the United States[1]. In 2022, approximately 20,000 new cases were diagnosed, with 13,000 estimated deaths[2]. The five-year survival in patients with advanced OC is estimated at 30% and has been relatively unchanged over recent years[3,4]. The poor prognosis associated with OC is because 70% of patients are diagnosed with advanced-stage disease because of limited effective screening options and often indolent or non-specific symptoms at diagnosis[1,5]. Moreover, chemotherapy resistance is one of the most significant factors contributing to the poor prognosis and increased mortality rate of patients diagnosed with ovarian cancer[6].
Notably, in recent years, the remarkable heterogeneity of OC has been increasingly realized, with significant variations in histology, genomic drivers, and molecular classification, which impact carcinogenesis, treatment selection, and disease outcomes. The vast majority of OC are of epithelial origin, and can be subdivided into several different histological subtypes, accounting for either low (30%) or high-grade (70%) tumors[7,8]. Low-grade serous OC tends to be relatively chemoresistant and harbor mutations in Kirsten rat sarcoma virus (KRAS), Phosphatase and TENsin homolog deleted on chromosome 10 (PTEN), and PIK3CA oncogenes[7]. In contrast, 90% of high-grade OC are serous carcinomas with mutations in tumor protein 53 (TP53), Breast Cancer gene 1 (BRCA1), and Breast Cancer gene 2 (BRCA2) oncogenes[7]. Notably, approximately 20% of OCs are hereditary with germline mutations, including BRCA1 and BRCA2[7].
The microbiome has been increasingly studied for its role in maintaining human health and disease. The microbiomes of the gut and the female reproductive tract have been linked to many diseases, including OC[8]. In recent years, pre-clinical and translational studies have demonstrated that specific microbes may contribute to carcinogenesis, toxicity, and efficacy of cancer therapies in patients with OC[9]. The microbiota is dynamic and may be altered through various mechanisms, including diet, exercise, medications, and fecal microbiota transplantation. This review provides an overview of the current literature detailing the relationship between OC and the microbiome, focusing on mechanisms of carcinogenesis and strategies for modulating the microbiota to improve treatment efficacy and toxicity.
REVIEW OF CURRENT TREATMENT PARADIGM FOR ADVANCED AND RECURRENT OVARIAN CANCER
While historically, most patients with OC were treated similarly in the front-line setting, the landscape of therapeutics has changed dramatically in recent years[10]. The front-line treatment paradigm for advanced OC currently includes a multimodal approach of cytoreductive surgery and chemotherapy with carboplatin and paclitaxel. Further, in patients with advanced OC, bevacizumab concurrently with platinum-doublet chemotherapy, followed by bevacizumab maintenance, is approved based on the results of GOG-0218 and ICON-7[11]. Similarly, maintenance with poly (ADP-ribose) polymerase (PARP) inhibitors is approved as monotherapy or in combination with bevacizumab, following results of the SOLO-1, PRIMA, VELIA, and PAOLA-1 trials[1,11-15]. Notably, OC tumors with homologous recombination deficiency (HRD) exhibit greater sensitivity to PARP inhibitors and have been shown to have the most significant benefit[3,10].
Despite treatment for advanced ovarian cancer with cytoreductive surgery and platinum-based chemotherapy, most patients will recur and die of their disease[16,17]. The main cause of chemoresistance in ovarian cancer is the increased antioxidant capacity of cancer cells against platinum-derived compounds[6]. Both chemotherapy drugs and cell metabolism produce significant levels of reactive oxygen species (ROS) in tumor cells, resulting in DNA damage and various cellular responses. Consequently, the nuclear factor erythroid 2-related factor 2 (Nrf2) pathway is activated to combat oxidative stress, which is associated with ovarian cancer development and resistance to platinum-based treatments[18]. Patients with recurrent, platinum-resistant ovarian cancer have limited therapeutic options, decreased chemotherapy response rates, and poor prognosis, with overall survival (OS) of 9-12 months[19]. There is a significant unmet need for innovative therapeutic strategies to improve the survival rate of patients with advanced OC.
MECHANISTIC INSIGHTS INTO THE ROLE OF THE MICROBIOME IN THE PATHOGENESIS OF OVARIAN CANCER
The microbiome as a mediator of human health and disease has been increasingly studied. The human microbiome is a collection of microorganisms, including bacteria, viruses, fungi, archaea, and protozoa, that live within and upon the body's mucosal surfaces[20,21]. Further, microbial-derived metabolites can impact immune function, cell proliferation and signaling, gene expression, and hormonal and nutrient metabolism. Each microbiota has unique properties, including the composition and diversity of microbes. The gut and the female reproductive tract microbiomes are especially relevant to gynecologic cancers, including OC [Figure 1]. The female reproductive tract has two distinct microbial environments. The lower female reproductive tract microbiota includes the vagina and cervix and is generally colonized by Lactobacillus species[9]. In contrast, the microbiota of the upper female reproductive tract, including the uterus, fallopian tubes, and ovaries, was historically considered sterile but is now understood to harbor a diverse array of anaerobes[9].
The microbiome can significantly contribute to cancer development through several mechanisms, such as chronic inflammation infections, integration into the human genome, and the production of genotoxic metabolites. Some examples, like Helicobacter pylori-mediated inflammation, are linked with mucosa-associated lymphoid tissue (MALT) lymphoma and gastric cancer[22]. Similarly, hepatocellular carcinoma was found to be more frequent in patients infected with Hepatitis B and C viruses[23] and Fusobacterium nucleatum with colorectal carcinoma[24,25]. OC is a complex and multifactorial disease with genetic, epigenetic, immunologic, and environmental risk factors. Given that significant knowledge gaps exist in OC pathogenesis, the microbiome has been increasingly studied as an environmental risk factor for several gynecologic cancers, including OC. While the pathogenesis of OC is not yet fully understood, growing evidence suggests that the microbiota may play a role in the development and progression of OC[25].
Changes in the composition of the gut microbiome and the intestinal mucosal barrier can lead to cancer development through various mechanisms, including chronic inflammation, altered immune response and barrier function, dysregulated nutrient and hormone metabolism, and upregulation of oncogenic signaling. Additionally, certain microbes can induce DNA damage and apoptosis by releasing genotoxic metabolites or indirectly by producing reactive oxygen species [Figure 2].
Figure 2. Microbiome mediated mechanisms of carcinogenesis and platinum resistance in ovarian cancer. TNF-α: Tumor necrosis factor alpha; TLR: toll-like receptor; VEGF-C: vascular endothelial growth factor-C; IL-6: interleukin 6; JAK: janus kinase; STAT: signal transducer and activator of transcription; LPS: lipopolysaccharides.
Observational studies have demonstrated a link between history of prior infections and OC, especially pelvic and sexually transmitted diseases. In a study led by Lin et al., pelvic inflammatory disease was associated with an increased risk of OC[26]. Specifically, studies have identified that a history of infection with specific pathogens, including Chlamydia trachomatis and Mycoplasma genitalium, is associated with OC development[27-30]. Prior infections may induce the activation of oncogenic pathways and increased production of inflammatory cytokines, such as disruption of the cell cycle via heightened mitogen-activated protein kinase (MAPK) signaling, degradation of p53, and the modulation of DNA damage repair proteins[31]. Specifically, studies have demonstrated that Chlamydia trachomatis contributes to carcinogenesis through multiple mechanisms, including blocking mitochondrial caspase-3 mediated cytochrome C release, inhibiting apoptosis and immune response, inducing DNA damage due to reactive oxygen species (ROS), and altering cellular tight junctions[31]. The persistence of human papillomavirus (HPV) infection is a well-established determinant for developing the majority of invasive cervical carcinomas via E6 and E7 viral oncogenes, which inactivate tumor suppressor genes p53 and Rb, respectively[31]. However, recent evidence suggests that HPV infection might also influence the pathogenesis of OC, potentially via a similar mechanism[15].
Previous studies have suggested complex interactions between the microbiota, cancer cells, and the tumor microenvironment, including upregulation of Toll-like (TLR) and nucleotide oligomerization domain (Nod)-like receptors (NLRs) signaling pathways[32-36]. Several carcinogenic signaling pathways may be activated in OC, including p53, Human epidermal growth factor receptor 2 (HER2), Epidermal growth factor receptor (EGFR), and Vascular endothelial growth factor receptor (VEGFR)[37]. Upregulation of pro-inflammatory pathways leads to increased IL-6, activation of the Janus kinase/signal transducers, and activators of the transcription 3 (JAK/STAT3) pathway, resulting in tumor proliferation[38]. IL-6 induces proliferation through increased production of matrix metalloproteinase (MMPs) and loss of E-cadherin expression, which promotes cell proliferation, increased autocrine and paracrine cytokine production, promotion of epithelial-mesenchymal transition and metastasis, and potentially development of chemoresistance [Figure 2][39]. Lactobacillus species may serve as tumor suppressors through the downregulation of Wnt/β-catenin signaling and may represent a therapeutic strategy in years to come[40].
Changes to the intestinal mucosal barrier expose the systemic circulation to bacteria and their associated by-products, leading to the production of pro-inflammatory cytokines like tumor necrosis factor-alpha
TLRs are important mediators of inflammatory pathways in the gut, which have a significant role in mediating immune responses and linking adaptive and innate immune responses [Figure 2]. TLR-4 and TLR-5/7 have been proposed as mediators in OC carcinogenesis via PI3K activation[43]. In a human-derived OC cell line (Human Epithelial Ovarian cancer cell line: CP70) study, bacterial lipopolysaccharide (LPS) binding to TLR-4 triggered multiple carcinogenesis events[44]. Similarly, TLR-4 activation in metastatic ovarian human tissue-derived cells (SKOV3 cells) resulted in NF-κB activation, p65 DNA binding, and the generation of pro-tumoral molecules (IL-6, IL-8, VEGF, and MCP-1), promoting cancer development and chemoresistance[45]. TLR-4 inhibition decreased MMP-2 and MMP-9 gene expression and enzymatic activity, preventing the epithelial-mesenchymal transition metastasis and providing antineoplastic effects[46]. TLR-5 recognition of commensal microbiota is linked to metastatic OC and systemic tumor-promoting inflammation in pre-clinical mouse models with TLR-5 deficiency treated with antibiotics to induce microbial dysbiosis[33]. TLR-5 activation leads to the production of immune-suppressive protein galectin-1, upregulation of IL-6, promotion of myeloid-derived suppressor cells (MDSCs) mobilization, and accelerated tumor growth. The importance of IL-6 production via TLR-5 activation in the first phases of ovarian cancer tumorigenesis is highlighted by the fact that TLR-5-deficient mice had much slower tumor growth. Notably, antibiotic-induced dysbiosis revokes TLR-5-dependent variations in tumor development.
The gut microbiome influences estrogen metabolism and systemically available estrogen levels, which may play a role in gynecologic cancer development [Figure 2]. Estradiol undergoes first-pass metabolism in the liver and is excreted through the bile, urine, or feces[47]. The “estrobolome” refers to microorganisms that can deconjugate estrogens excreted within the stool via beta-glucuronidase and beta-glucosidase enzymes, leading to intestinal reabsorption of estrogens and increased systemic estrogen levels[47]. In a study by Peters, shotgun metagenomic sequencing was performed on stool samples of 2,300 patients, including 295 pre-menopausal women, 1,027 post-menopausal women, and 978 men. They identified significant differences in the microbial diversity and composition between the two groups with post-menopausal women with decreased Akkermansia muciniphila, Clostridium lactatifermentans, and Parabacteroides johnsonii species. Specifically, post-menopausal women had a decreased abundance of microbial β-glucuronidase, which correlated with serum progestin metabolite levels[48]. Therefore, the interactions between estrogen, progesterone, gut microbiome, and OC warrant further investigation[49].
MICROBIAL SIGNATURES IN OVARIAN CANCER
In recent years, the gut and female reproductive tract microbiomes have been associated with OC development and treatment responses. While historically, the upper female reproductive tract was considered sterile, preliminary data also supports that microbe within the peritoneal cavity and OC tumors, referred to as the tumor microbiome, may also contribute to carcinogenesis and chemoresistance[50,51]. In a study by Banerjee et al., it was demonstrated that microbial colonization of OC tumors was frequent, with representative species including Brucella (76%), Chlamydia (60%), and Mycoplasma (74%)[52]. Additionally, they observed a significant increase in the ratio of Proteobacteria to Firmicutes in OC tumors, distinct from the composition in healthy tissues[52]. Similarly, Miao et al. evaluated the peritoneal microbiota in patients with benign ovarian masses (n = 20) or OC (n = 10)[51]. They identified that patients with OC had a distinct peritoneal microbial signature, including microorganisms responsible for estrogen metabolism
The female reproductive tract microbiome has been associated with the development of OC. Studies have identified that the absence of Lactobacillus in the microbiota of the female reproductive tract may impact OC development and oncologic outcomes. In a case-control study of 176 patients with OC compared to 115 healthy controls, 16S rRNA gene sequencing, decreased Lactobacillus within the female reproductive tract microbiota was strongly associated with increased risk for OC. Further analysis demonstrated that in age-matched women with BRCA1 mutations, those with a non-Lactobacillus dominant microbiota were more likely to have OC [Table 1][54].
Clinical studies overview
Author | Study type | Study population | Study findings |
Asangba et al., 2023[53] | Cohort | 18 years of age or older and undergoing hysterectomy for ovarian cancer | Microbial taxa were identified as differentially abundant between the benign cohort and early- and advanced-stage OC patients. The presence of mostly detrimental microbes was observed in early-stage, low-grade OC patients, with a decrease in advanced-stage, high-grade OC patients |
Nené et al., 2019[54] | Case-control | OC patients | Non-lactobacilli-dominated cervicovaginal microbiomes were more prevalent in patients with ovarian cancer and in women with BRCA1 mutations who had yet to develop cancer, compared with age-matched healthy women and women without BRCA1 or BRCA2 mutations, respectively |
Jacobson et al., 2021[56] | Retrospective | Platinum-free interval (PFI) < 6 months | Observed the inverse relationship between a Lactobacillus-dominant vaginal microbiome and ovarian cancer, with only 24% of OC patients having Lactobacillus species present compared to healthy controls (47%) |
Chambers et al., 2021[59] | Retrospective cohort | Patients with recurrent EC, CC, and OC treated with ICIs | Antibiotics given 30 days prior to ICI treatment, but not during, are linked to reduced response and worse progression-free survival (PFS) and overall survival (OS) in women with recurrent endometrial, cervical, and ovarian cancer |
Pflug et al., 2022[58] | Prospective cohort study | A potential negative impact of anti-Gram-positive antibiotics on the anticancer activity of cyclophosphamide and cisplatin in a clinical setting | |
Chambers et al., 2020[60] | Retrospective cohort | Patients diagnosed with epithelial ovarian cancer stage III/IV | Antibiotic treatment during platinum chemotherapy leads to a significant reduction in both progression-free survival (PFS) and overall survival (OS). Additionally, the use of antibiotics targeted against gram-positive bacteria is strongly associated with worse oncologic outcomes |
Wang et al., 2022[107] | Community‐based observational | Significant differences in the microbial populations and microbial-derived metabolites were observed between sarcopenic and non-sarcopenic patients |
Lastly, preliminary data has linked the gut microbiota composition with the development of OC. In a study by Chen et al., two cohorts of mice were treated with tamoxifen to induce ovarian cancer over the course of one year and were supplemented with either antibiotics for five months or a placebo[55]. Antibiotic treatment led to significant changes to the microbiota of the vagina and the gut in mice, and mice that received antibiotics had significantly fewer and less advanced tumors than control animals at the study endpoint[55]. Jacobson et al. identified an inverse relationship between Lactobacillus species and OC, with only 24% of OC patients having Lactobacillus species present compared to healthy controls [Table 1][56]. In contrast, studies have suggested that certain gut microbes may have anti-tumor activity in OC. In a study by Wang, supplementation of Akkermansia in pre-clinical mouse models of OC was associated with decreased tumor growth, increased T-cell activation, and enhanced interferon (IFNy) secretion from CD8+ T cells[57]. Further investigation is needed to understand how these microbes could be targeted for early diagnosis, therapeutic modulation, and to overcome chemoresistance[56].
IMPACT OF THE MICROBIOME ON TREATMENT RESPONSE AND DEVELOPMENT OF CHEMORESISTANCE IN OVARIAN CANCER
Over the last decade, studies across multiple cancer types suggest that the microbiome may impact the efficacy and toxicity of both systemic chemotherapy and immunotherapy[58-68]. Platinum-based chemotherapy remains the most effective chemotherapy agent for OC patients. It is utilized both in the front-line setting and in patients with recurrent, platinum-sensitive disease in combination with other agents, including paclitaxel, gemcitabine, pegylated liposomal doxorubicin with or without bevacizumab[16]. Platinum-based chemotherapy exerts its antineoplastic effects through the formation of platinum-DNA adducts or crosslinks, which block DNA replication and provoke the production of ROS, resulting in cell death[69,70].
Several studies have assessed the impact of the microbiome on platinum response in pre-clinical cancer models, including OC. A landmark study by Iida et al. administered antibiotics to mouse models of melanoma, leukemia, and colon adenocarcinoma before treatment with oxaliplatin and cisplatin[69]. Antibiotics were associated with reduced treatment response and survival compared to control animals[69]. Mice treated with antibiotics had downregulation of genes related to adaptive immune response, antigen presentation, DNA damage repair, and upregulation of genes related to cancer metabolism. Specific gram-positive microbes, such as Lactobacillus fermetum, were associated with improved anticancer activity[69]. One potential mechanism for this effect is, in prior studies, translocation of gram-positive bacteria during mucositis (characterized by epithelial barrier inflammation and cell loss), which leads to increased production of cytotoxic ROS and the infiltration of Th-17 cells into tumors, resulting in improved anticancer activity[70].
Several studies have demonstrated that in pre-clinical models of cancer, antibiotic treatment to alter the gut microbiome leads to global changes in immune response and activation of pro-inflammatory genes, which supports the gut microbiome’s impact on chemotherapy response through direct or indirect actions on the immune system[69-73]. These data suggest that the gut microbiome may have a role in modulating the immune response for platinum chemotherapy efficacy. Additionally, the gut microbiota might trigger the production of ROS in tumor-infiltrating myeloid cells, leading to increased oxidative stress and heightened cytotoxicity of platinum compounds[69,72,74].
In pre-clinical models of OC utilizing syngeneic ID-8 cells, Chambers et al. demonstrated that broad-spectrum antibiotic treatment (with neomycin, ampicillin, vancomycin, and metronidazole) resulted in increased tumor growth, cisplatin resistance, and decreased survival compared to non-antibiotic treated control mice[59]. Additionally, RNAseq analysis of OC tumors of antibiotic-treated mice demonstrated significant changes in DNA damage repair, cell death, angiogenesis, and cancer stem cell gene pathways compared to controls[59]. Notably, the chemoresistant phenotype observed in the antibiotic-treated mice was overcome through cecal microbiota transplantation of control mice stool. In this study, several gut-derived tryptophan metabolites were significantly decreased in the antibiotic-treated mice, including indole-3-propionic acid. These studies provide background to support the production of anticancer metabolites and may represent a target for intervention in women with recurrent, platinum-resistant ovarian cancer in years to come. Subsequently, clinical studies have demonstrated that antibiotics during platinum chemotherapy are associated with worsened oncologic outcomes in several cancers, including OC[58,59,68,70,75]. These studies associated anti-gram-positive antibiotics with reduced progression-free survival (PFS) and OS. In a study by Pflug et al., 800 patients with chronic lymphocytic leukemia and lymphoma were undergoing treatment with either cyclophosphamide or cisplatin, respectively, on two clinical trial protocols[Table 1][58]. Investigators assessed the use of antibiotics targeting gram-positive bacteria during treatment and demonstrated that of the patients with relapsed lymphoma, those who were treated with anti-gram-positive antibiotics
Immunotherapy is a promising treatment approach for many patients with advanced or recurrent gynecologic cancer, but unfortunately, prospective clinical trials have demonstrated limited efficacy of immunotherapy for patients with OC[19,76,77]. Notably, in patients with melanomas and various solid malignancies, the gut microbiome has been shown to modulate response to immunotherapy[64,67,77]. In a retrospective cohort study of 101 women with recurrent gynecologic cancers, including 26 patients with OC, antibiotic treatment prior to immunotherapy was associated with a significantly lower response rate, PFS, and OS[61]. Further research is needed to understand how the microbiome mediates immunotherapy response in women with gynecologic cancers and whether modulation of the microbiota may be a strategy to improve immunotherapy response in these patients.
IMPACT OF THE MICROBIOME UPON SURGICAL MANAGEMENT AND POSTOPERATIVE OUTCOMES IN OVARIAN CANCER
Cytoreductive surgery, which normally includes the removal of the uterus, cervix, fallopian tubes, ovaries, and omentum, is an essential component of the care of OC patients. Optimal cytoreduction, to remove all visible disease to less than one centimeter and ideally no gross residual disease, is consistently associated with improved PFS and OS[17,75,78-80]. Due to the often widespread nature of OC involving the peritoneal surfaces, upper abdominal surgical procedures, including diaphragm resection or splenectomy, as well as small bowel resection and large bowel resection, are often required to achieve optimal cytoreduction[79,80]. Given the extensive nature of these surgical procedures, it is not uncommon for patients to experience postoperative complications, including ileus, infection, wound separation, and anastomotic leaks[60,79]. Data on how the gut and female reproductive tract microbiome may influence a person’s risk for postoperative morbidity are limited. In a study by Tong et al., significant differences were noted between stool samples taken pre-operatively, postoperatively, and during chemotherapy[81]. Further, they appreciated that OC patients significantly increased in anaerobic species such as Bacteroides, Collinsella, and Blautia during chemotherapy[81]. Studies in patients with colorectal cancer undergoing cytoreductive surgery with bowel resection have demonstrated that probiotic administration before surgery may be protective against surgical site infection and anastomotic leaks. Still, additional data are necessary to understand the potential mechanism and whether this will similarly extend to patients with OC[82-85].
MODULATION OF THE MICROBIOME IN OVARIAN CANCER AND FUTURE DIRECTIONS
The next frontier in microbiome science is to develop therapeutic approaches to modulate the microbiome to improve response to cancer treatment in OC patients. Potential strategies include dietary modifications, exercise, fecal microbiota transplantation (FMT), and probiotic supplementation[60,68,84,86-90]. Studies in non-gynecologic cancers have demonstrated promising early responses to FMT in immunotherapy patients. Landmark phase I clinical trials by two groups gave patients with PD-1 refractory metastatic melanoma an FMT with stool from patients whose tumors responded well to treatment. FMT overcame the immunotherapy resistance in certain patients, with clinical responses restored in 30%, with one complete response. Therefore, it is plausible that these approaches may yield similar benefits in women with OC, and further study is necessary[89,91] .
In OC patients, many of whom are elderly, fatigued, malnourished, or have diminished appetite related to the stigma of advanced disease, probiotic supplementation holds potential as a practical therapeutic intervention. Studies evaluating probiotics in non-gynecologic cancers have demonstrated promising results, including decreased infectious outcomes and diminished radiation and chemotherapy-induced gastrointestinal toxicities[92-96]. Further, in vitro and early-phase clinical trials of probiotics as an oral supplement or intra-tumoral injection have demonstrated anticancer activity[92-97]. Additional studies are needed to investigate the feasibility of probiotic supplementation during chemotherapy in OC patients, evaluate the impact on the gut microbiota, patient quality of life, and symptoms, and assess the association with oncologic outcomes.
Currently, data regarding microbiome-directed dietary interventions to impact outcomes in OC patients are limited[98-100]. Diet is a key mediator of the composition and function of the microbiota. Microbial-derived metabolites, such as short-chain fatty acids (SCFA), are degradation products of fiber and can influence host immunity, either directly via changes to metabolic signaling pathways or indirectly through the regulation of gut mucosal permeability. Western-style diets, which are defined by high levels of saturated fats and low dietary fiber, have been linked to reduced SCFA levels and altered host immune response[101]. In a study of 128 patients with melanoma, those with high dietary fiber intake (defined as > 20 grams/day) had improved PFS compared to the low fiber cohort, which was most notable in those who did not use concurrent probiotics[86]. These studies provide evidence that diet modulation may be a promising strategy to improve cancer therapy outcomes.
Gynecologic cancer patients, especially the older population with advanced-stage disease, are more susceptible to malnutrition, sarcopenia, and cachexia, impacting approximately 70% of patients[102]. Malnutrition and skeletal muscle depletion have many adverse consequences, including lower quality of life, increased risk of prolonged hospitalization, heightened treatment-related toxicity, re-operations, readmission, and postoperative complications[103-105]. Several retrospective studies have indicated a strong link between malnutrition and decreased OS in OC patients compared to non-malnourished patients[103-106].
Importantly, the microbiome may influence the pathogenesis of cancer cachexia through changes in the gut microbial composition and mucosal barrier function. In a study of 1,417 participants, 10% were considered sarcopenic based on physical performance and biometric testing. Significant differences in the microbial populations and microbial-derived metabolites were observed between sarcopenic and non-sarcopenic patients [Table 1][107]. An analysis of blood samples of patients with cancer cachexia showed increased bacterial translocation, resulting in heightened inflammation and elevated concentrations of inflammatory markers such as IL-6, TNF- α, and IFN-γ[108]. Approaches focused on restoring microbial balance and enhancing gut barrier function through long-term or acute dietary changes or nutrition interventions may hold promise for addressing malnutrition and sarcopenia in cancer patients.
Prospective clinical trials are urgently needed to assess potential strategies to target the microbiome. In July 2023, we performed a systematic search strategy on the ClinicalTrials.gov website utilizing a librarian-designed search strategy [Supplementary File 1]. We compared the number of active clinical trials for microbiome-mediated interventions (prebiotics, probiotics, live biotherapeutics) for the leading cancer diagnoses in the United States according to the Surveillance, Epidemiology, and End Results (SEER) program[109]. On a review of available clinical trials on ClinicalTrials.gov in July 2023, we identified that OC and uterine cancer are remarkably underrepresented compared to other disease sites such as colorectal cancer and melanoma, and this highlights a major unmet in future research efforts [Figure 3].
CONCLUSIONS
The microbiome of the gut and female reproductive tracts serves essential functions in maintaining health. The microbiome may also impact the toxicity and efficacy of cancer therapies for OC patients. Furthermore, the microbiota is highly dynamic and may be modulated through various approaches, although significant research gaps exist for gynecologic cancer patients. The connection between the microbiome and ovarian cancer is still being investigated, but the key question of whether they are directly linked remains unanswered. Since ovarian cancer tends to occur later in life, it is challenging to conduct long-term studies that establish causality. This raises concerns about whether the vaginal microbiome contributes to its development and whether it can be leveraged for primary prevention. Moreover, establishing a causal link between the microbiome and ovarian cancer is a challenging task due to the complexity of the disease, the difficulty in conducting large longitudinal studies, and the multifaceted nature of the microbiome. Although research is still underway, there is optimism that we are making progress in the right direction. Targeting the microbiome may lead to innovative ways to treat ovarian cancer, improve outcomes, and reduce toxicity. This emerging field holds promise for developing personalized treatment approaches. Further research may lead to innovative therapies that can transform the prognosis for those affected by recurrent and chemoresistant OC. Advancing our understanding of the complex relationship between the microbiota and OC represents a novel strategy to improve future patient outcomes.
DECLARATIONS
Author’s contributions
Participated in conceptualization, methodology, investigation, data curation, writing the original draft, reviewing and editing, and visualization: Mehra Y
Participated in the methodology, investigation, data curation, writing the original draft, reviewing and editing, and visualization: Chalif J
Participated in the conceptualization, methodology, investigation, data curation, writing the original draft and figures, reviewing and editing, and visualization: Mensah-Bonsu C
Participated in project reviewing, editing of the final manuscript, and project supervision: O’Malley DM
Participated in conceptualization, methodology, formal analysis, data curation and reviewing, editing, and project supervision: Spakowicz D
Participated in conceptualization, methodology, formal analysis, data curation and reviewing, editing, and project supervision: Chambers L
Availability of data and materials
Not applicable.
Financial support and sponsorship
This work was supported by Grant 2021258 for Laura Chambers, DO from the Doris Duke Charitable Foundation (DDCF) through the COVID-19 Fund to Retain Clinical Scientists collaborative grant program and was made possible through the support of Grant 62288 from the John Templeton Foundation. The opinions expressed in this publication are those of the author(s) and do not necessarily reflect the view of the DDCF, the John Templeton Foundation, the OSU College of Medicine or Center for Clinical and Translational Science. Additionally, this project was supported by a Research Grant by a Path to K award from the Ohio State University College of Medicine Office of Research and the Center for Clinical & Translational Science through the Richard P. & Marie R. Bremer Medical Research Fund and William H. Davis Endowment for Basic Medical Research. The content is solely the responsibility of the authors and does not necessarily reflect the views of the Davis/Bremer Research Fund, the CCTS, or The Ohio State University Medical Center. Additional grant support includes The Ohio State University National Center for Advancing Translational Sciences, Grant UL1TR001070, and The Pelotonia Institute for Immuno-Oncology.
Conflicts of interest
Mehra Y, Chalif J, Mensah-Bonsu C, Spakowicz D and Chambers L have no conflicts of interest. O’Malley DM received research funding and/or personal fees (consulting and/or advisory boards) for clinical research from AstraZeneca, Tesaro/GSK, Immunogen, Ambry, Janssen/J&J, Abbvie, Regeneron, Amgen, Novocure, Genentech/Roche, Array Biopharma, EMD Serono, Ergomed, Ajinomoto Inc., Ludwig Cancer Research, Stemcentrx, Inc, CERULEAN PHARMA, GOG Foundation, Bristol-Myers Squibb Co, Serono Inc, TRACON Pharmaceuticals, Yale University, New Mexico Cancer Care Alliance, INC Research, Inc, inVentiv Health Clinical, Iovance, PRA Intl, Myriad Genetics, Eisai, Tarveda, Merck, GenMab, SeaGen, Novartis, Mersana, Clovis, Rubis, Elevar, Takeda, Toray; INXMED; SDP Oncology (BBI); Arquer Diagnostics; Roche Diagnostics MSA; Sorrento, Corcept Therapeutics, and Celsion Corp.
Ethical approval and consent to participate
Not applicable.
Consent for publication
Not applicable.
Copyright
© The Author(s) 2023.
Supplementary Materials
REFERENCES
1. Lheureux S, Braunstein M, Oza AM. Epithelial ovarian cancer: evolution of management in the era of precision medicine. CA Cancer J Clin 2019;69:280-304.
3. Boehm KM, Aherne EA, Ellenson L, et al. MSK MIND Consortium. Multimodal data integration using machine learning improves risk stratification of high-grade serous ovarian cancer. Nat Cancer 2022;3:723-33.
4. Cancer of the ovary - cancer stat facts. SEER. Available from: https://seer.cancer.gov/statfacts/html/ovary.html. [Last accessed on 15 Nov 2023].
5. Elsherif S, Javadi S, Viswanathan C, Faria S, Bhosale P. Low-grade epithelial ovarian cancer: what a radiologist should know. Br J Radiol 2019;92:20180571.
6. Tossetta G. Metformin improves ovarian cancer sensitivity to paclitaxel and platinum-based drugs: a review of in vitro findings. Int J Mol Sci 2022;23:12893.
7. Hollis RL, Gourley C. Genetic and molecular changes in ovarian cancer. Cancer Biol Med 2016;13:236-47.
8. Elkafas H, Walls M, Al-Hendy A, Ismail N. Gut and genital tract microbiomes: dysbiosis and link to gynecological disorders. Front Cell Infect Microbiol 2022;12:1059825.
9. Łaniewski P, Ilhan ZE, Herbst-Kralovetz MM. The microbiome and gynaecological cancer development, prevention and therapy. Nat Rev Urol 2020;17:232-50.
10. Mirza MR, Coleman RL, González-Martín A, et al. The forefront of ovarian cancer therapy: update on PARP inhibitors. Ann Oncol 2020;31:1148-59.
11. Haunschild CE, Tewari KS. Bevacizumab use in the frontline, maintenance and recurrent settings for ovarian cancer. Future Oncol 2020;16:225-46.
12. Pujade-Lauraine E, Banerjee S, Pignata S. Management of platinum-resistant, relapsed epithelial ovarian cancer and new drug perspectives. J Clin Oncol 2019;37:2437-48.
13. Banerjee S, Drapkin R, Richardson DL, Birrer M. Targeting NaPi2b in ovarian cancer. Cancer Treat Rev 2023;112:102489.
14. Garcia J, Hurwitz HI, Sandler AB, et al. Bevacizumab (Avastin®) in cancer treatment: a review of 15 years of clinical experience and future outlook. Cancer Treat Rev 2020;86:102017.
15. Borella F, Carosso AR, Cosma S, et al. Gut microbiota and gynecological cancers: a summary of pathogenetic mechanisms and future directions. ACS Infect Dis 2021;7:987-1009.
16. Armstrong DK, Alvarez RD, Backes FJ, et al. NCCN Guidelines® insights: ovarian cancer, version 3. 2022. J Natl Compr Canc Netw 2022;20:972-80.
17. Coleridge SL, Bryant A, Lyons TJ, Goodall RJ, Kehoe S, Morrison J. Chemotherapy versus surgery for initial treatment in advanced ovarian epithelial cancer. Cochrane Database Syst Rev 2019;2019:CD005343.
18. Yang L, Xie HJ, Li YY, Wang X, Liu XX, Mai J. Molecular mechanisms of platinum-based chemotherapy resistance in ovarian cancer (review). Oncol Rep 2022;47:82.
19. Richardson DL, Eskander RN, O'Malley DM. Advances in ovarian cancer care and unmet treatment needs for patients with platinum resistance: a narrative review. JAMA Oncol 2023;9:851-9.
20. Zhao LY, Mei JX, Yu G, et al. Role of the gut microbiota in anticancer therapy: from molecular mechanisms to clinical applications. Signal Transduct Target Ther 2023;8:201.
21. Adak A, Khan MR. An insight into gut microbiota and its functionalities. Cell Mol Life Sci 2019;76:473-93.
22. Uemura N, Okamoto S, Yamamoto S, et al. Helicobacter pylori infection and the development of gastric cancer. N Engl J Med 2001;345:784-9.
23. Zampino R, Pisaturo MA, Cirillo G, et al. Hepatocellular carcinoma in chronic HBV-HCV co-infection is correlated to fibrosis and disease duration. Ann Hepatol 2015;14:75-82.
24. Kostic AD, Gevers D, Pedamallu CS, et al. Genomic analysis identifies association of fusobacterium with colorectal carcinoma. Genome Res 2012;22:292-8.
25. Sadrekarimi H, Gardanova ZR, Bakhshesh M, et al. Emerging role of human microbiome in cancer development and response to therapy: special focus on intestinal microflora. J Transl Med 2022;20:301.
26. Lin HW, Tu YY, Lin SY, et al. Risk of ovarian cancer in women with pelvic inflammatory disease: a population-based study. Lancet Oncol 2011;12:900-4.
27. Idahl A, Lundin E, Jurstrand M, et al. Chlamydia trachomatis and mycoplasma genitalium plasma antibodies in relation to epithelial ovarian tumors. Infect Dis Obstet Gynecol 2011;2011:824627.
28. Idahl A, Le Cornet C, González Maldonado S, et al. Serologic markers of chlamydia trachomatis and other sexually transmitted infections and subsequent ovarian cancer risk: results from the EPIC cohort. Int J Cancer 2020;147:2042-52.
29. Rasmussen CB, Jensen A, Albieri V, Andersen KK, Kjaer SK. Increased risk of borderline ovarian tumors in women with a history of pelvic inflammatory disease: a nationwide population-based cohort study. Gynecol Oncol 2016;143:346-51.
30. Fortner RT, Terry KL, Bender N, et al. Sexually transmitted infections and risk of epithelial ovarian cancer: results from the Nurses' Health Studies. Br J Cancer 2019;120:855-60.
31. Shanmughapriya S, Senthilkumar G, Vinodhini K, Das BC, Vasanthi N, Natarajaseenivasan K. Viral and bacterial aetiologies of epithelial ovarian cancer. Eur J Clin Microbiol Infect Dis 2012;31:2311-7.
32. Round JL, Lee SM, Li J, et al. The toll-like receptor 2 pathway establishes colonization by a commensal of the human microbiota. Science 2011;332:974-7.
33. Rutkowski MR, Stephen TL, Svoronos N, et al. Microbially driven TLR5-dependent signaling governs distal malignant progression through tumor-promoting inflammation. Cancer Cell 2015;27:27-40.
34. Fang Y, Yan C, Zhao Q, et al. The association between gut microbiota, toll-like receptors, and colorectal cancer. Clin Med Insights Oncol 2022;16:11795549221130549.
35. Le Noci V, Bernardo G, Bianchi F, Tagliabue E, Sommariva M, Sfondrini L. Toll like receptors as sensors of the tumor microbial dysbiosis: implications in cancer progression. Front Cell Dev Biol 2021;9:732192.
36. Couturier-Maillard A, Secher T, Rehman A, et al. NOD2-mediated dysbiosis predisposes mice to transmissible colitis and colorectal cancer. J Clin Invest 2013;123:700-11.
37. Zhou B, Sun C, Huang J, et al. The biodiversity composition of microbiome in ovarian carcinoma patients. Sci Rep 2019;9:1691.
38. Browning L, Patel MR, Horvath EB, Tawara K, Jorcyk CL. IL-6 and ovarian cancer: inflammatory cytokines in promotion of metastasis. Cancer Manag Res 2018;10:6685-93.
39. Savant SS, Sriramkumar S, O'Hagan HM. The role of inflammation and inflammatory mediators in the development, progression, metastasis, and chemoresistance of epithelial ovarian cancer. Cancers 2018;10:251.
40. Ghanavati R, Akbari A, Mohammadi F, et al. Lactobacillus species inhibitory effect on colorectal cancer progression through modulating the Wnt/β-catenin signaling pathway. Mol Cell Biochem 2020;470:1-13.
41. Chung L, Thiele Orberg E, Geis AL, et al. Bacteroides fragilis toxin coordinates a pro-carcinogenic inflammatory cascade via targeting of colonic epithelial cells. Cell Host Microbe 2018;23:203-14.e5.
42. Suriano F, Nyström EEL, Sergi D, Gustafsson JK. Diet, microbiota, and the mucus layer: the guardians of our health. Front Immunol 2022;13:953196.
43. Lupi LA, Cucielo MS, Silveira HS, et al. The role of Toll-like receptor 4 signaling pathway in ovarian, cervical, and endometrial cancers. Life Sci 2020;247:117435.
44. Kelly MG, Alvero AB, Chen R, et al. TLR-4 signaling promotes tumor growth and paclitaxel chemoresistance in ovarian cancer. Cancer Res 2006;66:3859-68.
45. Szajnik M, Szczepanski MJ, Czystowska M, et al. TLR4 signaling induced by lipopolysaccharide or paclitaxel regulates tumor survival and chemoresistance in ovarian cancer. Oncogene 2009;28:4353-63.
46. Zandi Z, Kashani B, Poursani EM, et al. TLR4 blockade using TAK-242 suppresses ovarian and breast cancer cells invasion through the inhibition of extracellular matrix degradation and epithelial-mesenchymal transition. Eur J Pharmacol 2019;853:256-63.
48. Peters BA, Lin J, Qi Q, et al. Menopause is associated with an altered gut microbiome and estrobolome, with implications for adverse cardiometabolic risk in the hispanic community health study/study of latinos. mSystems 2022;7:e0027322.
49. Cheng H, Wang Z, Cui L, et al. Opportunities and challenges of the human microbiome in ovarian cancer. Front Oncol 2020;10:163.
50. Brewster WR, Burkett WC, Ko EM, Bae-Jump V, Nicole McCoy A, Keku TO. An evaluation of the microbiota of the upper reproductive tract of women with and without epithelial ovarian cancer. Gynecol Oncol Rep 2022;42:101017.
51. Miao R, Badger TC, Groesch K, et al. Assessment of peritoneal microbial features and tumor marker levels as potential diagnostic tools for ovarian cancer. PLoS One 2020;15:e0227707.
53. Asangba AE, Chen J, Goergen KM, et al. Diagnostic and prognostic potential of the microbiome in ovarian cancer treatment response. Sci Rep 2023;13:730.
54. Nené NR, Reisel D, Leimbach A, et al. Association between the cervicovaginal microbiome, BRCA1 mutation status, and risk of ovarian cancer: a case-control study. Lancet Oncol 2019;20:1171-82.
55. Chen L, Zhai Y, Wang Y, et al. Altering the microbiome inhibits tumorigenesis in a mouse model of oviductal high-grade serous carcinoma. Cancer Res 2021;81:3309-18.
56. Jacobson D, Moore K, Gunderson C, et al. Shifts in gut and vaginal microbiomes are associated with cancer recurrence time in women with ovarian cancer. PeerJ 2021;9:e11574.
57. Wang Z, Qin X, Hu D, et al. Akkermansia supplementation reverses the tumor-promoting effect of the fecal microbiota transplantation in ovarian cancer. Cell Rep 2022;41:111890.
58. Pflug N, Kluth S, Vehreschild JJ, et al. Efficacy of antineoplastic treatment is associated with the use of antibiotics that modulate intestinal microbiota. Oncoimmunology 2016;5:e1150399.
59. Chambers LM, Esakov Rhoades EL, Bharti R, et al. Disruption of the gut microbiota confers cisplatin resistance in epithelial ovarian cancer. Cancer Res 2022;82:4654-69.
60. Chambers LM, Kuznicki M, Yao M, et al. Impact of antibiotic treatment during platinum chemotherapy on survival and recurrence in women with advanced epithelial ovarian cancer. Gynecol Oncol 2020;159:699-705.
61. Chambers LM, Michener CM, Rose PG, Reizes O, Yao M, Vargas R. Impact of antibiotic treatment on immunotherapy response in women with recurrent gynecologic cancer. Gynecol Oncol 2021;161:211-20.
62. Routy B, Le Chatelier E, Derosa L, et al. Gut microbiome influences efficacy of PD-1-based immunotherapy against epithelial tumors. Science 2018;359:91-7.
63. Viaud S, Saccheri F, Mignot G, et al. The intestinal microbiota modulates the anticancer immune effects of cyclophosphamide. Science 2013;342:971-6.
64. Pinato DJ, Howlett S, Ottaviani D, et al. Association of prior antibiotic treatment with survival and response to immune checkpoint inhibitor therapy in patients with cancer. JAMA Oncol 2019;5:1774-8.
65. Gopalakrishnan V, Spencer CN, Nezi L, et al. Gut microbiome modulates response to anti-PD-1 immunotherapy in melanoma patients. Science 2018;359:97-103.
66. Abdel-rahman O, Ghosh S, Walker J. Outcomes of metastatic colorectal cancer patients in relationship to prior and concurrent antibiotics use; individual patient data analysis of three clinical trials. Clin Transl Oncol 2020;22:1651-6.
67. D’amico F, Perrone AM, Rampelli S, et al. Gut microbiota dynamics during chemotherapy in epithelial ovarian cancer patients are related to therapeutic outcome. Cancers 2021;13:3999.
68. Spakowicz D, Hoyd R, Muniak M, et al. Inferring the role of the microbiome on survival in patients treated with immune checkpoint inhibitors: causal modeling, timing, and classes of concomitant medications. BMC Cancer 2020;20:383.
69. Iida N, Dzutsev A, Stewart CA, et al. Commensal bacteria control cancer response to therapy by modulating the tumor microenvironment. Science 2013;342:967-70.
70. Yin B, Wang X, Yuan F, Li Y, Lu P. Research progress on the effect of gut and tumor microbiota on antitumor efficacy and adverse effects of chemotherapy drugs. Front Microbiol 2022;13:899111.
71. Ma W, Mao Q, Xia W, Dong G, Yu C, Jiang F. Gut microbiota shapes the efficiency of cancer therapy. Front Microbiol 2019;10:1050.
72. Rea D, Coppola G, Palma G, et al. Microbiota effects on cancer: from risks to therapies. Oncotarget 2018;9:17915-27.
73. Wilkinson EM, Ilhan ZE, Herbst-Kralovetz MM. Microbiota-drug interactions: impact on metabolism and efficacy of therapeutics. Maturitas 2018;112:53-63.
74. Lin C, Cai X, Zhang J, et al. Role of gut microbiota in the development and treatment of colorectal cancer. Digestion 2019;100:72-8.
75. Chambers LM, Bussies P, Vargas R, et al. The microbiome and gynecologic cancer: current evidence and future opportunities. Curr Oncol Rep 2021;23:92.
76. Mahdi H, Chelariu-Raicu A, Slomovitz BM. Immunotherapy in endometrial cancer. Int J Gynecol Cancer 2023;33:351-57.
77. Disis ML, Adams SF, Bajpai J, et al. Society for immunotherapy of cancer (SITC) clinical practice guideline on immunotherapy for the treatment of gynecologic cancer. J Immunother Cancer 2023;11:e006624.
78. Baek MH, Park EY, Ha HI, et al. Secondary cytoreductive surgery in platinum-sensitive recurrent ovarian cancer: a meta-analysis. J Clin Oncol 2022;40:1659-70.
79. Clair KH, Wolford J, Zell JA, Bristow RE. Surgical management of gynecologic cancers. Surg Oncol Clin N Am 2021;30:69-88.
80. Chang SJ, Hodeib M, Chang J, Bristow RE. Survival impact of complete cytoreduction to no gross residual disease for advanced-stage ovarian cancer: a meta-analysis. Gynecol Oncol 2013;130:493-98.
81. Tong J, Zhang X, Fan Y, et al. Changes of intestinal microbiota in ovarian cancer patients treated with surgery and chemotherapy. Cancer Manag Res 2020;12:8125-35.
82. Alam Z, Shang X, Effat K, et al. The potential role of prebiotics, probiotics, and synbiotics in adjuvant cancer therapy especially colorectal cancer. J Food Biochem 2022;46:e14302.
83. Huang F, Li S, Chen W, et al. Postoperative probiotics administration attenuates gastrointestinal complications and gut microbiota dysbiosis caused by chemotherapy in colorectal cancer patients. Nutrients 2023;15:356.
84. Pitsillides L, Pellino G, Tekkis P, Kontovounisios C. The effect of perioperative administration of probiotics on colorectal cancer surgery outcomes. Nutrients 2021;13:1451.
85. Zhang LM, Schuitevoerder D, White MG, et al. Combined mechanical and oral antibiotic bowel preparation is associated with prolonged recurrence-free survival following surgery for colorectal cancer. J Surg Oncol 2021;124:1106-14.
86. Spencer CN, McQuade JL, Gopalakrishnan V, et al. Dietary fiber and probiotics influence the gut microbiome and melanoma immunotherapy response. Science 2021;374:1632-40.
87. David LA, Maurice CF, Carmody RN, et al. Diet rapidly and reproducibly alters the human gut microbiome. Nature 2014;505:559-63.
88. Dizman N, Hsu J, Bergerot PG, et al. Randomized trial assessing impact of probiotic supplementation on gut microbiome and clinical outcome from targeted therapy in metastatic renal cell carcinoma. Cancer Med 2021;10:79-86.
89. Baruch EN, Youngster I, Ben-Betzalel G, et al. Fecal microbiota transplant promotes response in immunotherapy-refractory melanoma patients. Science 2021;371:602-9.
90. Ahrén IL, Bjurberg M, Steineck G, Bergmark K, Jeppsson B. Decreasing the adverse effects in pelvic radiation therapy: a randomized controlled trial evaluating the use of probiotics. Adv Radiat Oncol 2023;8:101089.
91. Davar D, Dzutsev AK, McCulloch JA, et al. Fecal microbiota transplant overcomes resistance to anti-PD-1 therapy in melanoma patients. Science 2021;371:595-602.
92. Garczyk A, Kaliciak I, Drogowski K, et al. Influence of probiotics in prevention and treatment of patients who undergo chemotherapy or/and radiotherapy and suffer from mucositis, diarrhoea, constipation, nausea and vomiting. J Clin Med 2022;11:3412.
93. Abdolalipour E, Mahooti M, Salehzadeh A, et al. Evaluation of the antitumor immune responses of probiotic bifidobacterium bifidum in human papillomavirus-induced tumor model. Microb Pathog 2020;145:104207.
94. del Giglio A, Atui FC. Fecal transplantation in patient with metastatic melanoma refractory to immunotherapy: a case report. World J Clin Cases 2023;11:5830-4.
95. Mahdy MS, Azmy AF, Dishisha T, et al. Irinotecan-gut microbiota interactions and the capability of probiotics to mitigate irinotecan-associated toxicity. BMC Microbiol 2023;23:53.
96. Gu J, Ahn-Jarvis JH, Riedl KM, Schwartz SJ, Clinton SK, Vodovotz Y. Characterization of black raspberry functional food products for cancer prevention human clinical trials. J Agric Food Chem 2014;62:3997-4006.
97. Xu M, Zhong F, Zhu J. Evaluating metabolic response to light exposure in lactobacillus species via targeted metabolic profiling. J Microbiol Methods 2017;133:14-9.
98. Chen J, Zhao KN, Vitetta L. Effects of intestinal microbial-elaborated butyrate on oncogenic signaling pathways. Nutrients 2019;11:1026.
99. Donohoe DR, Holley D, Collins LB, et al. A gnotobiotic mouse model demonstrates that dietary fiber protects against colorectal tumorigenesis in a microbiota- and butyrate-dependent manner. Cancer Discov 2014;4:1387-97.
100. Arpaia N, Campbell C, Fan X, et al. Metabolites produced by commensal bacteria promote peripheral regulatory T-cell generation. Nature 2013;504:451-5.
101. Beyaz S, Chung C, Mou H, et al. Dietary suppression of MHC class II expression in intestinal epithelial cells enhances intestinal tumorigenesis. Cell Stem Cell 2021;28:1922-35.e5.
102. Morton M, Patterson J, Sciuva J, et al. Malnutrition, sarcopenia, and cancer cachexia in gynecologic cancer. Gynecol Oncol 2023;175:142-55.
103. Polen-de C, Fadadu P, Weaver AL, et al. Quality is more important than quantity: pre-operative sarcopenia is associated with poor survival in advanced ovarian cancer. Int J Gynecol Cancer 2022;32:1289-96.
104. Seebacher V, Rockall A, Nobbenhuis M, et al. The impact of nutritional risk factors and sarcopenia on survival in patients treated with pelvic exenteration for recurrent gynaecological malignancy: a retrospective cohort study. Arch Gynecol Obstet 2022;305:1343-52.
105. Rinninella E, Fagotti A, Cintoni M, et al. Skeletal muscle mass as a prognostic indicator of outcomes in ovarian cancer: a systematic review and meta-analysis. Int J Gynecol Cancer 2020;30:654-63.
106. Bekos C, Grimm C, Gensthaler L, et al. The pretreatment controlling nutritional status score in ovarian cancer: influence on prognosis, surgical outcome, and postoperative complication rate. Geburtshilfe Frauenheilkd 2022;82:59-67.
107. Wang Y, Zhang Y, Lane NE, et al. Population-based metagenomics analysis reveals altered gut microbiome in sarcopenia: data from the Xiangya Sarcopenia Study. J Cachexia Sarcopenia Muscle 2022;13:2340-51.
108. Jiang Y, Guo C, Zhang D, Zhang J, Wang X, Geng C. The altered tight junctions: an important gateway of bacterial translocation in cachexia patients with advanced gastric cancer. J Interferon Cytokine Res 2014;34:518-25.
109. About the SEER registries. SEER. Available from: https://seer.cancer.gov/registries/index.html. [Last accessed on 15 Nov 2023].
Cite This Article
Export citation file: BibTeX | RIS
OAE Style
Mehra Y, Chalif J, Mensah-Bonsu C, Spakowicz D, O’Malley DM, Chambers L. The microbiome and ovarian cancer: insights, implications, and therapeutic opportunities. J Cancer Metastasis Treat 2023;9:38. http://dx.doi.org/10.20517/2394-4722.2023.107
AMA Style
Mehra Y, Chalif J, Mensah-Bonsu C, Spakowicz D, O’Malley DM, Chambers L. The microbiome and ovarian cancer: insights, implications, and therapeutic opportunities. Journal of Cancer Metastasis and Treatment. 2023; 9: 38. http://dx.doi.org/10.20517/2394-4722.2023.107
Chicago/Turabian Style
Mehra, Yogita, Julia Chalif, Carol Mensah-Bonsu, Daniel Spakowicz, David M. O’Malley, Laura Chambers. 2023. "The microbiome and ovarian cancer: insights, implications, and therapeutic opportunities" Journal of Cancer Metastasis and Treatment. 9: 38. http://dx.doi.org/10.20517/2394-4722.2023.107
ACS Style
Mehra, Y.; Chalif J.; Mensah-Bonsu C.; Spakowicz D.; O’Malley DM.; Chambers L. The microbiome and ovarian cancer: insights, implications, and therapeutic opportunities. J. Cancer. Metastasis. Treat. 2023, 9, 38. http://dx.doi.org/10.20517/2394-4722.2023.107
About This Article
Copyright
Data & Comments
Data
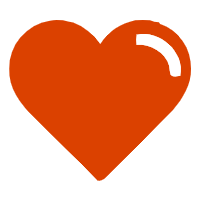

Comments
Comments must be written in English. Spam, offensive content, impersonation, and private information will not be permitted. If any comment is reported and identified as inappropriate content by OAE staff, the comment will be removed without notice. If you have any queries or need any help, please contact us at support@oaepublish.com.