Targeting cerebrospinal fluid for discovery of brain cancer biomarkers
Abstract
Central nervous system (CNS) cancer is a devastating illness with unmet therapeutic needs. Establishing biomarkers that have the potential to guide accurate CNS cancer diagnosis or are helpful in predicting disease progression or therapy response is of great interest. Cerebrospinal fluid (CSF) has been extensively targeted for the detection of molecules that might be useful markers for cancer detection. However, so far very few of such markers have found a standardized routine clinical application. This review examines the current scientific knowledge about the biochemical elements in the CSF that have been reported in the literature as brain cancer biomarkers and highlight reasons why the role of most markers is not yet established in the managment of CNS tumors.
Keywords
Introduction
Brain cancers are the leading cause of death by solid tumors in children and the cause of morbidity and mortality across a wide range of adult individuals.[1,2] The identification of biomarkers that could allow diagnosis of brain neoplasms and could be informative for cancer spread or monitor therapy response is in great demand. Blood analysis for novel biomarkers has facilitated the timely diagnosis for patients with several malignancies such as prostate and breast cancers.[3] However, one of the challenges that contributes to the paucity of biomarkers in the serum for central nervous system (CNS) malignancies is the blood-brain barrier, which is thought to prevent the release of tumor-specific molecules into the blood circulation. Cerebrospinal fluid (CSF) has thus been investigated in the search for brain tumor markers.
CSF is a readily accessible body fluid that is reflective of the underlying pathological state of the CNS, hence it has been widely targeted for biomarker discovery for a variety of neurological disorders. The CSF is continuously produced and recycled much like blood or lymph.[3] The majority of CSF is produced by the choroid plexus located on the lateral, third and fourth ventricles. The rate of CSF production in humans is 0.3-0.4 mL/min and the total CSF volume is 90-150 mL in adults and 65-150 mL in children.[4-6] CSF circulates through the ventricles, the cisterns, and the subarachnoidal space at the base of the brain, then flows over the convexities of the brain and down the length of the spinal cord.[5-7] Therefore, CSF is in contact with brain tissue and in proximity to most tumor bulks, making it an ideal reservoir of tumor-related/secreted molecules.[8] It is accessible through lumbar puncture, a little invasive procedure. Any cancer cells released by brain cancer bulk or molecules that are actively secreted or passively diffused by cancer cells are likely to disperse into the CSF and therefore can be detected. Hence CSF analysis is considered to be an important tool in the evaluation of CNS malignancies. This review discusses potential and limitations of CSF analyses in brain cancer patients.
Detection of cancer cells in the CSF
Primary CNS cancers and metastases are often located in close proximity to ventricular surfaces or CSF cisterns.[9-11] Malignant cells derived from brain cancers reach the leptomeninges by CSF spread or by direct extension from the primary tumor itself e.g. medulloblastoma, primitive neuroectodermal tumors, germ cell tumors, ependymoma, and glioma may be disseminated throughout the neuroaxis by the flow of the CSF.[12-14]Table 1 shows the particular incidence of malignant leptomeningeal involvement in selected primary brain cancers. Currently microscopic evaluation of CSF is routinely performed in CNS malignancies with frequent leptomeningeal spread, such as medulloblastomas, PNET, pineoblastomas, germ-cell tumors and CNS lymphoma.[15] Cancer therapy and prognosis of these groups of brain cancer are crucially determined by positive CSF cytology.[13,14]
Association between primary brain tumors localisation and LS incidence
Disease | Localisation | Incidence LS | Source |
---|---|---|---|
Medulloblastoma | Fossa posterior possible extension to fourth ventricle, brainstem, cisterna magna | 30-40% | [17,85] |
Supratentorial PNETs | Frontal lobes, parietal, temporal and occipital lobes | 25-40% | [17,86] |
CNS AT/RT | The exact incidence of CNS AT/RT is difficult to determine because the tumor has been widely recognized for only the last decade | 29% | [87-89] |
Retinoblastoma | Retina, possible optic nerve invasion and choroidal involvement | 3-23% | [90,91] |
Germ-cell tumors | Pineal-region, possible extension to third ventricle, suprasellar | 22% | [92] |
Primary CNS lymphoma | Cerebral hemisphers, basal ganglia, corpus callosum, cerebellum | 10-20% | [93] |
Brainstem glioma | Tectal plate to medullary cervical junction, possible extension to prepontine cistern and fourth ventricle | 3-13% | [94,95] |
Pinealoblastoma | Pineal-region | 10% | [96] |
LGG hypothalamic | LGG can occur anywhere in the CNS | 7% | [97-100] |
Ependymoma | Infratentorial intraventricular (fourth ventricle’s floor, lateral walls, roof) or supratentorial within the brain parenchyma | 5% | [17] |
CSF cytoanalysis
CSF cytology, in which CSF is prepared and examined under a microscope to look for cells, is currently considered the gold standard for diagnosis of brain cancer with leptomeningeal spread and metastatic cancer to the brain.[14] To achieve CSF cytology a sample can be obtained at the time of tumor surgery or by lumbar or intracerebroventricular (ICV) reservoir puncture.[3] However, lumbar CSF remains the specimen of choice to detect malignant cells of primary CNS tumors.[12,16] To avoid false positive results due to sloughing of tumor cells at the time of surgery, a recovering interval of one to two weeks is currently suggested before performing diagnostic postoperative CSF cytologic evaluation.[9,16,17] Accurate cytopreparatory techniques are essential criteria for successful CSF microscopic evaluations. 7.5 mL of CSF are usually withdrawn and immediately processed, as the cell counts can diminish by up to 50% within 2 h of collection.[4,18] CSF samples are then processed by centrifugation (CytospinÒ) at 800 g for 3-5 min, air-dried for 10-15 min and stained with May-Grunwald Giemsa (MGG) stain solution for 10-15 min.[19] Thin-layer preparation (ThinPrep) is a relatively new liquid-based cytology method which has been suggested to better detect malignant cells in CSF from solid tumors by performing good preservation of cell morphologic features. During the ThinPrep analysis, the CSF cells are collected through high-precision filtration driven by fluid mechanics and gently absorbed onto a glass slide by using electrochemical forces. The collected samples need to be added to 10 mL preservation solution, mixed and stood for 15 min. Slides are fixed in 95% ethanol for 15 min and stained by standard Papanicolaou method.[19]
CSF cytology, although indispensable, has many limitations Table 2. To start with it involves the pathological identification of abnormal cells in the CSF by Giemsa stain and clinicians must make judgments on the presence or absence of malignant cells. Hence, CSF cytological analysis is a pure qualitative test that bears no quantification and lacks validation.[3,20] Another weakness is that because the shedding of malignant cells into the CSF may occur intermittently and in low numbers, inconsistent presence of cancer cells in the CSF should be expected. CSF specimens may, therefore, fail to capture malignant cells representing one of the major weaknesses of CSF cytology. It is therefore recommended that CSF analysis should be repeated if initially negative.[21] One of the drawbacks is while CSF cytology is highly specific in detection of cancer cells, it suffers from a lack of sensitivity. A retrospective meta-analysis[22] reported that CSF cytology sensitivity could be as low as 45% depending on how many times the lumbar puncture was repeated. False negative cytopathology is common (10-20% of patients) because of the paucity of cells in the CSF and morphological similarities between benign and malignant cells.[13,23,24] The lack of standardized techniques for obtaining and evaluating CSF cytology specimens and the absence of molecular analysis of tumor cells certainly contributes to the wide sensitivity range.[3] Hence although it is currently used in the clinic, CSF cytology remains a poor surrogate marker for disease response in brain cancer/metastasis involvement.[9,13]
Advantages and disadvantages of different methods for brain tumors biomarkers detection in the CSF
Approach | Method | Pros | Cons |
---|---|---|---|
Detection of cancer cells in the CSF | CSF cytoanalysis: CSF is examined under a microscope to look for cancer cells | Highly specific[12-14,16] | Low sensitivity and false negative results are common[3,13,20,23,24] |
Flow cytometry analysis: Have the potential to provide information about cell surface protein expression | Automated method that allows rapid analysis[14,25] | False negative and false positive results can occur (especially at low cell counts, < 25 cells/uL). Poor differential ability between mitoses and neoplastic cells is reported[14,23,25] | |
Smaller CSF volume is needed[9,23] | |||
Other tools: Measuring chromosomal content of cancer cells in the CSF using DNA single cell cytometry techniques or fluorescence in-situ hybridization | These techniques have the ability to detect genetic aberrations as a sign of malignancy location[26] | Low sensitivity[26] | |
Detection of biochemical molecules secreted by cancers to the CSF | CSF proteomic analysis: Systematic identification and quantification of the complete complement of proteins in the CSF | Specific proteomic patterns can differentiate subtypes or grades of specific brain tumors[27-30] | Limited sensitivity and specificity[31] |
CSF microRNAs analysis: Measuring microRNA Profiling of CSF | High specificity and chemical stability[60,101]. Only small amounts of CSF samples are required for the detection of miRNAs in the CSF offers the advantage of convenient repetitive monitoring of molecular events happening in cancer in the response to treatment[76] | The unknown origin and factors influence their level of expression might impact their specificity as biomarkers[76,102-106] |
Flow cytometry analysis
CSF fluid flow cytometry is a useful addition to CSF cytology. Cytology examines morphologic patterns, and flow cytometry has the potential to provide information about cell surface protein expression. It is an additional highly sensitive cytological technique capable of accurately detecting malignant CSF cells, especially in comparatively smaller CSF volume and in samples with very low cell counts when combined with multicolor fluorescent antibody labelling.[9,23] In this method CSF must be processed similar to cytology within 1 hour of sampling however centrifugation should be minimized.[14,23] Automated methods allow rapid flow cytometry data analysis and thereby reduce the significant time expenditures used in conventional cytology routine.[14,25] Flow cytometry seems to provide a higher sensitivity. However the cell count and the percentage of neoplastic cells reported in the CSF by both cytology and flow cytometry were significantly higher compared with those found to be positive by flow cytometry alone.[23]
It has to be said that both false negative and false positive results (especially at low cell counts, < 25 cells/uL) can occur with flow cytometry too, a poor differential ability between mitoses and neoplastic cells is also reported Table 2. Therefore before flow cytometry can be recommended in a routine CSF examination in combination with the conventional cytology, standardized protocols are needed to uniform definitions of positivity and procedure.[14,23,25] Rare cell capture technology, for example, CellSearch® is a recent technique using molecular markers to detect and enumerate circulating tumor cells in the CSF. This method is established to detect prognostic marker on different cancer cells circulating in the peripheral blood such as breast cancer and has recently attracted the interest of CSF cancer researcher.[3,9] However, the application of CellSearch® technology for detecting primary CNS cancer cells in CSF has not been published yet.
Other tools for cancer cell detection in the CSF
Measuring the chromosomal content of cancer cells in the CSF, using DNA single cell cytometry techniques or fluorescence in-situ hybridization that detects genetic aberrations as a sign of malignancy, can also give additional diagnostic information to CSF analysis, but still has a low sensitivity Table 2. PCR can also establish cancer diagnosis when cytology is inconclusive, but the genetic alteration of the neoplasia must be known for it to be amplified with this technique, and this is generally not the case.[26]
Proteomic analysis of CSF
Proteomic profiling has become an active area of research for the biomarker discovery and the identification of new targets for therapeutic strategies. Recent studies have shown that specific proteomic patterns can differentiate subtypes or grades of human brain tumors.[27-30] Modern technological advancements in protein quantification which provide rapid screening, low sample consumption, and accurate protein identification, have enhanced the precision of proteomic analyses and are anticipated to accelerate brain tumor biomarker discovery.[31]
Research work on traditional sampling sources for proteomic profiling, such as blood[31,32] and tissue lysates,[33] have yielded asubstantial amount of information on potential brain cancer biomarkers. However, the majority of these markers exhibited limited value in a clinical setting, justifying the need for the exploration of more clinically relevant sampling sources. One such a promising source for protein biomarker discovery is the CSF where protein presences might result from either secretion/leaking by tumor tissues or abnormal blood brain barrier function.[8]
CSF proteomic analysis for detection of brain cancer markers
In the search for accurate biomarkers a number of reports have emerged over the past decade describing the analysis of different brain cancer proteome using CSF. For example the CSF level of carcinoembryonic antigen (CEA), is a protein tumor marker that is commonly increased in several human malignances, was found recently to play an important role in differential diagnosis of primary and metastatic brain tumors[34,35] and useful auxiliary marker in diagnosis of meningeal carcinomas.[36-38] In a study by Khwaja et al.,[39]the authors reported that proteomic analysis of CSF can discriminate malignant and non-malignant disease of the CNS and identified carbonic anhydrase protein (known to be overexpressed in many malignancies including high-grade gliomas) as a prognostic marker of brain cancer.
The most significant example of how analysis of CSF proteins has impacted the clinical management of CNS cancer is in the case of intracranial malignant germ cell tumors.[40] Germ cell tumors are heterogeneous group of gonadal or extragonadal tumors that thought to arise from the aberrant migration and differentiation of primordial germ cells during embryogenesis. Extragonadal germ cell tumors can occur intracranial in the pineal and suprasellar regions and comprise approximately 3% of all pediatric brain tumors. Germ cell tumors retain the molecular characteristics of their primordial lineage as they maintain the expression of embryonic proteins, such as beta human chorionic gonadotropin (bHCG) and alpha-fetoprotein (AFP).[41] bHCG is a 36 kDa glycoprotein normally secreted by placental tissues while AFP is a 70 kDa glycoprotein normally secreted by the foetus primarily in the yolk sac, gastrointestinal tract, and liver. AFP is elevated in wide range of cancers, including colon adenocarcinoma, liver and gastric cancers while bHCG and AFP were found to be markedly elevated in the CSF of intracranial malignant germ cell tumor patients.[42] Both markers are currently utilized clinically as diagnostic and accurate indicators of response to therapy. Assessment of AFP and total bHCG in both serum and CSF is mandatory in order to distinguish between germinoma and NGGCT non-germinoma germ cell tumors. CSF AFP > 1000 ng/mL at diagnosis, or age < 6 years, intracranial malignant germ cell tumor patients are stratified as high risk and are treated more intensively. Moreover, the verification of bHCG and AFP levels prior to surgical resection provides a reference point that can be used to assess recurrence during follow-up however their absence does not rule out a germ cell tumor. Additional CSF protein markers such as placental alkaline phosphatase (PLAP) and lactate dehydrogenase isoenzymes have been shown to be clinically useful in the diagnosis and monitoring of pediatric intracranial germinomas, however such markers are less specific.[43] Elevated levels of s-kit, the soluble form of the c-kit receptor, a transmembrane tyrosine kinase receptor, was found to be a reliable marker for germ cell tumor diagnosis that can differentiate germ cell tumors from other CNS cancers. Miyanohara et al.[44] also reported that s-kit expression is able to detect recurrence of germ cell tumors and subarachnoid dissemination.
Gliomas are the most common primary brain tumors in adults. Glioblastoma multiforme (GBM) is the deadliest glioma with a median survival of only 14 months despite the recent advances in intensive therapeutic strategies.[45] Hence more effort was applied to study whether specific CSF proteomic profile can be generated to evaluate gliomas prognosis. Fang Shen et al.[8] conducted a review of the literature on the proteomic screening for glioma-related protein biomarkers in CSF. They were able to identify 19 differentially expressed proteins, the majority exhibited increased concentrations (B2M, CA2, CA12, CALD1, DDAH1, MYCN, PPIA, SPP1, VEGFB, ALB, MAPT, SERPINA3, SPARCL1) while (GSN) was downregulated in the glioma CSF. Further functional assessments revealed several important protein networks (e.g., IL6/STAT-3) and four novel focus proteins (IL-6, galanin (GAL), HSPA5 and WNT4) and the authors reported that these proteins might be involved in glioma pathogenesis. On the same theme, Khwaja et al.[46] used two proteomic techniques, two-dimensional gel electrophoresis and cleavable Isotope-Coded Affinity Tag to compare CSF proteomes in order to identify tumor- and grade-specific biomarkers in patients bearing brain tumors of different histology and grades. By performing retrospective analyses on 60 samples derived from astrocytomas WHO grade II, III, and IV, schwannomas, metastastic brain tumors, inflammatory samples, and non-neoplastic controls, the group identified 103 potential tumor-specific markers of which 20 were high-grade astrocytoma-specific. SPARCL1, FGF14, VEGF-B, tau, b2M, bdefensin and Attractin were found as an upregulated marker in the CSF of patients with malignant astrocytoma and mediates glioma cell migration.[47] Sampath et al.[48] assessed whether vascular endothelial growth factor (VEGF) could be measured in the CSF of patients with cerebral neoplasms and used as a marker of particular brain cancer tumors. They investigated CSF samples from 27 patients with high-grade astrocytomas, 39 patients with nonastrocytic CNS neoplasms, and 14 patients with no known CNS neoplasm. In their study, VEGF was detectable in 89% of samples with malignant astrocytoma and not normal CSF samples. The levels of VEGF were significantly higher in high-grade astrocytomas than in nonastrocytic tumors indicating that detection of VEGF in CSF could be a potential marker for differentiating astrocytic from nonastrocytic tumors.
Another group applied mass spectrometry based technology to identify possible CSF peptide markers of GBM.[49] Out of 2,000 detected CSF peptides four peptides which significantly distinguished GBM from controls were identified. They were specific C-terminal fragments of alpha-1-antichymotrypsin, osteopontin, and transthyretin as well as N-terminal residue of albumin. Interestingly the identified four molecules are constituents of normal CSF, but this group are the first to report their significant elevation in CSF of GBM patients. To detect biomarkers in high-grade astrocytomas, Ohnishi et al.[50] analysed the differential expression of proteins in the CSF from two cases each of diffuse astrocytoma (grade II), and glioblastoma (grade IV) using agarose 2-D gel electrophoresis. The authors found that the expression of gelsolin protein is decreased with histological grade. To examine whether gelsolin is a useful indicator of tumor aggressiveness the group further analysed the gelsolin expression in 41FFPE astrocytomas. Gelsolin expression was found to be significantly lower in high-grade than in low-grade astrocytomas. Moreover the overall survival of patients in the low-gelsolin expression was significantly poorer than in the high expression group highlighting the usefulness of gelsolin as a potential prognostic factor in astrocytoma.
Diffuse intrinsic pontine glioma (DIPG) is not surgically resectable, resulting in a paucity of tissue available for molecular studies and, currently, there are no effective treatments. Saratsis et al.[51] investigated 15 CSF specimens from patients with DIPG for proteomic analysis. Protein profiling was generated by mass spectrometry. CSF proteomic analysis revealed selective upregulation of Cyclophillin A (CypA) and dimethylarginase 1 (DDAH1) in DIPG, compared with controls. Protein expression was further validated with Western blot analysis and immunohistochemical assays using CSF and brain tissue as well as in blood samples from DIPG. Immunohistochemical staining showed selective upregulation of secreted but not cytosolic CypA and DDAH1 in patients with DIPG. Their study indicated that detection of secreted CypA and DDAH1 in CSF and serum has potential clinical application, with implications for assessing treatment response and detecting tumor recurrence in patients with DIPG.
Primary central nervous system lymphoma (PCNSL) is another highly aggressive tumor that can lead to quick death if not diagnosed in time. The diagnosis of PCNSL can present a diagnostic challenge. It relies on histopathology of brain biopsies to the same extent as most brain tumors, while less invasive tests to detect early tumor pathogens with sufficient diagnostic accuracy are not available yet. Proteomic analysis of CSF has revealed various proteins that are differentially expressed in CNS lymphoma.[52-54] Among these, antithrombin III (ATIII), a serine protease inhibitor that is associated with neovascularization in CNS lymphoma, has been prospectively validated.[26] ATIII expression was reported by Roy et al.[55] to be elevated in the CSF of patients with CNS lymphoma compared to those patients with control. ATIII levels higher than 1.2 g/mL made the detection of CNS lymphoma possible with >70% sensitivity and 99% specificity.[26] Elevated antithrombin III levels significantly correlated with shorter survival rates and less response to chemotherapy. However and on the contrary a recent study from Finland, by Kuusisto et al.[56] declared that ATIII is not a suitable biomarker for diagnosis of PCNSL and increased concentrations of ATIII in CSF might be due to leakage of the blood-brain barrier.[57]
CXCL13 protein that is known to mediate chemotaxis of CNS lymphoma cells was detected within biopsy specimens from PCNSL patients[58] raising the possibility that this chemokine may contribute to CNS tropism. Rubenstein et al.[55] investigated the concentration of CXCL13 in CSF of CNS lymphoma patients and control cohorts in a multicenter study involving 220 patients. Their result demonstrated that elevated CXCL13 concentration in CSF is a highly specific marker for the detection of CNS lymphoma and can be helpful as an adjunctive diagnostic test and response to treatment assessment. Following their steps in studying chemokine in PCNSL, Sasagawa et al.[59] investigated CSF from 19 patients with CNS lymphoma (15 and 26 non-lymphoma patients with various brain tumors) and reported that CSF IL-10 is a superior biomarker for initial screening for patients with CNS lymphoma.
Medulloblastoma (MB) is the most common malignant brain tumor in children. It includes various subtypes with group 3 and 4 subtypes being clinically distinct with regard to metastasis and prognosis, which may also manifest in a difference in their proteomic spectra. With the aim to identify putative biomarkers for MB in CSF, Rajagopal et al.[60] investigated the CSF proteome from 33 children with MB and compared it against the CSF proteome from 25 age-matched controls using two-dimensional gel electrophoresis. In their study levels of prostaglandin D2 synthase (PGD2S) were found to be six-fold significantly decreased in the CSF of tumor samples most likely representing a host response to the presence of the tumor.[61] Usually biomarkers are often thought to be elevated in a disease state compared to normal levels however candidate negative diagnostic marker such as PGD2S could be useful for detecting MB as well as recurrence of the disease. On the other hand it has to be said that while negative biomarkers are potentially useful, their relationship to tumor biology is less direct and more highly complex in comparison to proteins that are over-expressed in tumor associated samples.[40] Desiderio et al.[62] investigated CSF from 14 children with posterior fossa tumors (6 Pilocytic astrocytoma, 5 Medulloblastoma, 3 Ependymoma and 5 nontumoral control). In their study the CSF proteomics demonstrated the potential biomarker role of the hemoglobin subunit beta fragments (peptides LVV- and VV-hemorphin-7) in posterior cranial fossa pediatric brain tumors. Both LVV- and VV-h7 were detectable in control-CSFs but absent in the patient CSFs collected before surgery (i.e. in presence of tumor). Interestingly both LVV- and VV-h7 were also absent in the CSF collected 6 days after the resection tumor in patients with tumor relapse. Their data suggest that analysis in post-surgery CSF could be used to predict patient prognosis. However, it will be interesting to evaluate the cancer specificity of LVV- and VV-h7 in relation to other forms of CNS pediatric tumors. Finally levels of polysialic-neural cell adhesion molecule (PSANCAM), considered a marker of developing neuron, were found to be significantly higher in CSF from MB patients that are refractory to treatment or those who relapsed, than patients in remission.[63]
Atypical teratoid/rhabdoid (AT/RT) tumor is a rare, highly malignant tumor of the CNS most commonly found in children less than 5 years of age. Osteopontin (OPN) a bone matrix glycoprotein levels were found to be significantly elevated in patients with AT/RT. Clinical studies identified OPN as a potential diagnostic marker in ovarian, breast, colon, prostate, and lung cancers.[64] Using enzyme-linked immunosorbent assay and immunohistochemical analysis, Kao et al.[65] investigated plasma, CSF, and brain tissue specimens from 39 patients MB, 16; AT/RT, 8; epilepsy, 6; hydrocephalus, 9) and found that patients with AT/RT have higher plasma and CSF OPN levels in comparison with patients with MB, hydrocephalus, or epilepsy. Interestingly significant correlation between OPN levels and the risk of tumor relapse in patients with AT/RT was identified while OPN levels in the CSF were found to decrease with treatment.
Other biochemical markers
Malignant brain tumors may show an increased fraction of anaerobic LDH concentrations (LD4 and LD5) in CSF.[52] A number of other potential CSF protein biomarkers for CNS cancers have been reported in the literature such as Insulin-like growth factor binding protein 2 (IGFBP2), Insulin-like growth factor binding protein 3 (IGFBP3),[66] Polysialic-neural cell adhesion molecule (PSANCAM),[63] Total Tau (t-Tau),[67] Tumor necrosis factor (TNF) alpha[68] and CSF , S-100,[69] Neuron-specific (NSE),[70] neuron growth factor, HCG.[71] Apolipoprotein A-II,[72] MIC-1/GDF15,[73] Elevated expression of such markers in the CSF was found to be relatively specific for brain cancer[74,75] however sensitivities and specificities have widely varied.[26]
MicroRNAs
MicroRNAs (miRNA) are short, non translated fragments of RNA that bind to 3’ untranslated regions of messenger RNA and repress protein translation in several molecular pathways.[26] The discovery of miRNAs role in controlling essential regulators of key pathways implicated in development of CNS tumors make them a powerful tool for detection of cancer, risk assessment and prognosis. During the past decades, great efforts have been made in conducting research evaluating the diagnostic value of miRNAs in CNS cancer’s tissue.[76] However, a major drawback of the tissue-based approach centers on the need for invasive surgical procedures in sample collection. MiRNAs have been found to stably coexist in several body fluids including CSF which can be collected with minimal invasiveness and permit following the disease over time.[26] In this context several reports have described that deregulated miRNAs in CSF are closely associated with the clinical course of CNS malignant tumors.[2,77-82]
For example Baraniskin et al.[77] found that combined expression analyses of miR-21 and miR-15b were able to distinguish patients with glioma from controls with various neurologic disorders, including patients with carcinomatous brain metastases and primary CNS lymphoma with accuracy of 90% sensitivity and 100% specificity. While Teplyuk et al.[2] reported that combined analysis of a group of seven CSF miRNAs enabled the discrimination between GBM and metastatic brain cancers with more than 90% accuracy. miRNA-21 and miR-10b expression levels were significantly increased only in brain tumor lesions (in patients with GBM or brain metastases) compared to nonneoplastic conditions while members of the miR-200 family were found solely in CSF of patients with brain metastases, indicating that CSF miRNAs could be used to discriminate between glioblastoma and metastatic brain tumors, an important consideration for cancer treatment.[2] GBM is the deadliest glioma with median survival of only 14 months despite the recent advances in intensive therapeutic strategies.[80] Due to their anatomic location and infiltrative nature, these tumors are not amenable to surgical resection or even to biopsy in some cases. The paucity of biomarkers represents a sizable gap in improving the clinical management of these patients. Analysis of CSF miRNA could therefore be advantageous for identifying putative disease markers for DIPGs.
An earlier work by Baraniskin et al.[77] demonstrated that combined miRNA analysis of miR-19, miR-21, and miR-92a in CSF accurately discriminate patients with PCNSL from other neurologic disorders controls with diagnostic accuracy of 95.7% sensitivity and 96.7% specificity indicating significant diagnostic value. In the same theme, Scott et al.[79] conducted a review of the literature on CNS lymphoma diagnosis (1966 to 2011) and extracted data regarding the usefulness of CSF cytology, proteomics and miRNAs in the diagnosis of CNS lymphoma. The authors reported low sensitivity for CSF cytology (2-32%) which is increased when combined with flow cytometry. CSF lactate dehydrogenase isozyme 5, β2-microglobulin, and immunoglobulin heavy chain rearrangement studies have improved sensitivity over CSF cytology (58-85%) but have only moderate specificity (85%). Interestingly miRNA analysis has more than 95% specificity in the diagnosis of CNS lymphoma.
Twenty three studies with a total of 299 CNS cancer patients and 418 controls were analyzed by Wei et al.[81] through systematic meta-analysis for articles in the topic diagnostic value of miRNAs for CNS cancers and comparing sensitivity of on blood-and CSF based miRNAs assays for the diagnosis of CNS malignancies. Thirteen out of the 23 studies they analyzed focused on miRNAs as diagnostic biomarkers for glioma and 10 for PCNSL detection. The performance of miRNAs in CSF for CNS cancers detection showed more correctness in sensitivity suggesting a relatively high diagnostic accuracy. By the end of the study the authors concluded that miRNAs may be suitable as biomarkers for CNS cancers detection and that the CSF based miRNAs assays could be considered more reliable for clinical application. However, further validation based on a larger sample of patients and controls is still required.[81]
The presence and biological role of miRNAs in the extracellular environment of meddulloblastoma MB was examined recently by our lab and we found that more than one thousand miRNAs were released in the culture-medium in each of the MB cell lines tested.[82] Among them a panel of miRNAs were specific to the culture-medium of metastasis-related cell lines (D341 and D283) which represents the aggressive group 3 and group 4 MB subtypes. Interestingly, three metastasis-associated miRNAs were over-represented in culture-medium of metastasis-related MB cell lines were found to be significantly enriched in the CSF of the MB patient. Although more samples are required to fully verify these results, our work presented the first evidence for the presence of miRNAs excreted extracellularly by MB cells and raises the possibility that investigations, using larger sets of MB samples, could lead in the near future to the discovery of CSF-derived miRNA markers, with diagnostic and prognostic significance.
How near are we to using CSF molecular markers for brain cancer diagnosis in the clinic?
The promise of CSF biochemical markers Table 3, such as proteins and miRNA, to detect and monitor brain cancer has swept through the oncology research area in recent years leading to ample publications. However, most putative markers did not progress beyond their initial discovery. A striking discrepancy exists between the effort directed toward CSF biomarker, whether it is protein or miRNA, discovery and the number of markers that made it into clinical practice.[83] Understanding the reasons why the role of these markers is not yet established in the diagnosis of CNS tumors can help accelerate the conduit between their discovery and clinical implementation. One of the confounding issues that participate in the failure of potential markers to reach the clinic is the lack of reproducibility between similar studies or low correlation of results. The most significant source for such inter-laboratory discrepancies is mainly due to differences in protein/miRNA preparation, in the analytical methods or the use of different technologies which may bias the analysis. There are various platforms/techniques which exist each with specific biases that can greatly influence the relative expression of certain molecules in the tested CSF sample and may lead to foregone conclusions. No wonder there is often a low correlation of results obtained from different platforms or even from the same labs using kits and reagents from different vendors. Yet there are no universally implemented guidelines. Hence standardization of these assays including CSF handling (collection, storage and preparation) is a challenge for the near future. Teuniseen et al.[84] have proposed protocols for the standardization of CSF collection to minimizing blood contamination of CSF and protocols for the standardization of CSF storage to prevent sample degradation and global proteome changes.[40]
CSF biomarkers for the detection of brain cancer
Brain cancer | Marker | Method of detection | References |
---|---|---|---|
Medulloblastoma, primitive neuroectodermal tumors, germ cell tumors, ependymoma and glioma | Cancer cells | CSF cytology | [12-14] |
Intracranial malignant germ cell tumors | bHCG and AFP | CSF proteomic analysis | [41] |
Pediatric brain tumors (medulloblastoma , high-grade glioma, atypical rhabdoid tumor, astrocytoma, plexus carcinoma and anaplastic ependymoma, germ cell tumor) | Apolipoprotein A-II | CSF proteomic analysis | [72] |
CNS lymphoma | CD27, AT III, chemoattractant, CXCL13, CXCL12 and IL10 | CSF proteomic analysis | [55,57,107-111] |
Cerebral low-grade lymphoma | Immunoglobulin G IgG | CSF proteomic analysis | [112] |
Brain metastases from lung adenocarcinoma | Epidermal growth factor receptor EGFR | CSF proteomic analysis | [113] |
Brain metastases from lung and breast cancers | VEGF and stromal cell derived factor (SDF)-1 | CSF proteomic analysis | [73] |
Medulloblastoma | PGD2 | CSF proteomic analysis | [60] |
Meningeal carcinomas | CYFRA 21-1, NSE and CEA | CSF proteomic analysis | [70] |
Glioblastoma | MIC-1 GDF15 | CSF proteomic analysis | [114] |
Glioblastoma | miR-21 and miR-15b | CSF microRNA analysis | [115] |
PCNSL | miR-19, miR-21, and miR-92a | CSF microRNA analysis | [115] |
Glioblastoma and brain metastasis | miR-10b and miR-21 | CSF microRNA analysis | [116-117] |
Brain metastases from lung and breast cancers | Members of miR-200 family | CSF microRNA analysis | [116] |
Glioblastoma, medulloblastoma, brain metastasis and lymphoma | miR-935, miR-451, miR-711, miR-223 and miR-125b | CSF microRNA analysis | [118] |
Another critically important consideration is that despite the fact that several advanced platforms are available the analysis of secreted proteins/miRNA in the CSF is still a very challenging task due to technical difficulties. Often the scientists working on CSF biomarker discovery have limited knowledge of the protein/miRNA isolation/detection new platforms and or the analytical requirements which may hamper the subsequent markers analysis.
Together with low sample numbers that usually result in inadequate statistical power is another general weakness and might explain why not many of these markers have been validated for clinical use.[31,40] Taking together the successful translation of CSF biomarkers from basic research to clinical applications will likely require multi-centre standardized and coordinated efforts to facilitate biomarkers discovery and implementation. Finally there are some other limitations to the interpretation of CSF cancer related molecules studies as biomarker. Protein/miRNA composition of CSF is dependent on patient attributes such as age, gender, the specific site of CSF access.[40]
Conclusion
CSF is an invaluable diagnostic window to the pathological state of CNS. It is easily accessible by minimally-invasive standard clinical methods and can provide the necessary biological information for the diagnosis of neurological diseases. Biochemical molecules secreted by brain cancers to the CSF hold great promise as diagnostic markers for a wide range of brain malignancies owing to the significant differences that have been reported between their expression profiles in healthy individuals and those of patients. However, significant concerns remain. Despite the sizeable published number of potential diagnostic and prognostic CSF biochemical markers their reproducibility between studies is unclear, and none have been validated for clinical use. The reported sample size in the literature is small. Most data were generated by a limited number of research groups using different protocols or technologies. No universally implemented guidelines are available yet for the CSF sample collection and preparation or for protein profiling or miRNA extraction from CSF and importantly for data analysis. It is therefore premature to make specific recommendations for their clinical implementation. More research that includes multi-institutional research and longitudinal studies of large patient cohorts to validate the clinical value of putative CSF markers, as demonstrated for the field of cancer genomics, is certainly warranted. The road from CSF biomarker discovery, validation, until the translation into the clinical setting could be long and difficult however, the reward for patients, clinicians and scientists could be rather significant.
This project was supported by the Swiss Research Foundation Child and Cancer.
Financial support and sponsorshipNil.
Conflicts of interestThere are no conflicts of interest.
REFERENCES
2. Teplyuk NM, Mollenhauer B, Gabriely G, Giese A, Kim E, Smolsky M, Kim RY, Saria MG, Pastorino S, Kesari S, Krichevsky AM. MicroRNAs in cerebrospinal fluid identify glioblastoma and metastatic brain cancers and reflect disease activity. Neuro Oncol 2012;14:689-700.
3. Patel AS, Allen JE, Dicker DT, Peters KL, Sheehan JM, Glantz MJ, El-Deiry WS. Identification and enumeration of circulating tumor cells in the cerebrospinal fluid of breast cancer patients with central nervous system metastases. Oncotarget 2011;2:752-60.
4. Bonadio W. Pediatric lumbar puncture and cerebrospinal fluid analysis. J Emerg Med 2014;46:141-50.
5. Brinker T, Stopa E, Morrison J, Klinge P. A new look at cerebrospinal fluid circulation. Fluids Barriers CNS 2014;11:10.
6. Johanson CE, Duncan JA, 3rd, Klinge PM, Brinker T, Stopa EG, Silverberg GD. Multiplicity of cerebrospinal fluid functions: New challenges in health and disease. Cerebrospinal Fluid Res 2008;5:10.
7. Machida A, Ohkubo T, Yokota T. Circulating microRNAs in the cerebrospinal fluid of patients with brain diseases. Methods Mol Biol 2013;1024:203-9.
8. Shen F, Zhang Y, Yao Y, Hua W, Zhang HS, Wu JS, Zhong P, Zhou LF. Proteomic analysis of cerebrospinal fluid: toward the identification of biomarkers for gliomas. Neurosurg Rev 2014;37:367-80; discussion 80.
9. Chamberlain M, Soffietti R, Raizer J, Ruda R, Brandsma D, Boogerd W, Taillibert S, Groves MD, Le Rhun E, Junck L, van den Bent M, Wen PY, Jaeckle KA. Leptomeningeal metastasis: a Response Assessment in Neuro-Oncology critical review of endpoints and response criteria of published randomized clinical trials. Neuro Oncol 2014;16:1176-85.
11. Patel AS, Allen JE, Dicker DT, Peters KL, Sheehan JM, Glantz MJ, El-Deiry WS. Identification and enumeration of circulating tumor cells in the cerebrospinal fluid of breast cancer patients with central nervous system metastases. Oncotarget 2014;2:752-60.
12. Gajjar A, Fouladi M, Walter AW, Thompson SJ, Reardon DA, Merchant TE, Jenkins JJ, Liu A, Boyett JM, Kun LE, Heideman RL. Comparison of lumbar and shunt cerebrospinal fluid specimens for cytologic detection of leptomeningeal disease in pediatric patients with brain tumors. J Clin Oncol 1999;17:1825-8.
14. Weston CL, Glantz MJ, Connor JR. Detection of cancer cells in the cerebrospinal fluid: current methods and future directions. Fluids Barriers CNS 2011;8:14.
15. Singh G, Mathur SR, Iyer VK, Jain D. Cytopathology of neoplastic meningitis: A series of 66 cases from a tertiary care center. Cytojournal 2013;10:13.
16. Fouladi M, Gajjar A, Boyett JM, Walter AW, Thompson SJ, Merchant TE, Jenkins JJ, Langston JW, Liu A, Kun LE, Heideman RL. Comparison of CSF cytology and spinal magnetic resonance imaging in the detection of leptomeningeal disease in pediatric medulloblastoma or primitive neuroectodermal tumor. J Clin Oncol 1999;17:3234-7.
17. Terterov S, Krieger MD, Bowen I, McComb JG. Evaluation of intracranial cerebrospinal fluid cytology in staging pediatric medulloblastomas, supratentorial primitive neuroectodermal tumors, and ependymomas. J Neurosurg Pediatr 2010;6:131-6.
18. Nayak L, Fleisher M, Gonzalez-Espinoza R, Lin O, Panageas K, Reiner A, Liu CM, Deangelis LM, Omuro A. Rare cell capture technology for the diagnosis of leptomeningeal metastasis in solid tumors. Neurology 2013;80:1598-605; discussion 603.
19. Pan Z, Yang G, Wang Y, He H, Pang X, Gao Y, Shi W, Li Y, Dong L, Song Y. Thinprep plus Papanicolaou stain method is more sensitive than cytospin-coupled Wright Giems stain method in cerebrospinal fluid cytology for diagnosis of leptomeningeal metastasis from solid tumors. PLoS One 2015;10:e0122016.
20. Bach F, Bjerregaard B, Soletormos G, Bach FW, Horn T. Diagnostic value of cerebrospinal fluid cytology in comparison with tumor marker activity in central nervous system metastases secondary to breast cancer. Cancer 1993;72:2376-82.
21. Chamberlain MC, Glantz M, Groves MD, Wilson WH. Diagnostic tools for neoplastic meningitis: detecting disease, identifying patient risk, and determining benefit of treatment. Semin Oncol 2009;36:S35-45.
22. Enting RH. Leptomeningeal neoplasia: epidemiology, clinical presentation, CSF analysis and diagnostic imaging. Cancer Treat Res 2005;125:17-30.
23. Ahluwalia MS, Wallace PK, Peereboom DM. Flow cytometry as a diagnostic tool in lymphomatous or leukemic meningitis: ready for prime time? Cancer 2012;118:1747-53.
24. Glantz MJ, Cole BF, Glantz LK, Cobb J, Mills P, Lekos A, Walters BC, Recht LD. Cerebrospinal fluid cytology in patients with cancer. Cancer 1998;82:733-9.
25. Strik H, Luthe H, Nagel I, Ehrlich B, Bahr M. Automated cerebrospinal fluid cytology: limitations and reasonable applications. Anal Quant Cytol Histol 2005;27:167-73.
27. Kim JH, Lee SK, Yoo YC, Park NH, Park DB, Yoo JS, An HJ, Park YM, Cho KG. Proteome analysis of human cerebrospinal fluid as a diagnostic biomarker in patients with meningioma. Med Sci Monit 2011;18:BR450-60.
28. Okamoto H, Li J, Vortmeyer AO, Jaffe H, Lee YS, Glasker S, Sohn TS, Zeng W, Ikejiri B, Proescholdt MA, Mayer C, Weil RJ, Oldfield EH, Zhuang Z. Comparative proteomic profiles of meningioma subtypes. Cancer Res 2006;66:10199-204.
29. Vankalakunti M, Vasishta RK, Das Radotra B, Khosla VK. MIB-1 immunolabeling: a valuable marker in prediction of benign recurring meningiomas. Neuropathology 2007;27:407-12.
30. Wulfkuhle JD, Paweletz CP, Steeg PS, Petricoin EF, 3rd, Liotta L. Proteomic approaches to the diagnosis, treatment, and monitoring of cancer. Adv Exp Med Biol 2003;532:59-68.
31. Kalinina J, Peng J, Ritchie JC, Van Meir EG. Proteomics of gliomas: initial biomarker discovery and evolution of technology. Neuro Oncol 2011;13:926-42.
32. Gollapalli K, Ray S, Srivastava R, Renu D, Singh P, Dhali S, Bajpai Dikshit J, Srikanth R, Moiyadi A, Srivastava S. Investigation of serum proteome alterations in human glioblastoma multiforme. Proteomics 2012;12:2378-90.
33. Iwadate Y, Sakaida T, Hiwasa T, Nagai Y, Ishikura H, Takiguchi M, Yamaura A. Molecular classification and survival prediction in human gliomas based on proteome analysis. Cancer Res 2004;64:2496-501.
34. Batabyal SK, Ghosh B, Sengupta S, Ghosh SN, Chatterjee R. Cerebrospinal fluid and serum carcinoembryonic antigen in brain tumors. Neoplasma 2003;50:377-9.
35. Moldrich G, Lange P, Strik H. Carcinoembryonic antigen in the CSF of cancer patients--the value of intrathecal synthesis and correlation with IgA-diffusion dynamics. Acta Neurol Belg 2010;110:314-20.
36. Nakagawa H, Kubo S, Murasawa A, Nakajima S, Nakajima Y, Izumoto S, Hayakawa T. Measurements of CSF biochemical tumor markers in patients with meningeal carcinomatosis. No Shinkei Geka 1991;19:1135-41. (in Japanese)
37. Nakagawa H, Kubo S, Murasawa A, Nakajima S, Nakajima Y, Izumoto S, Hayakawa T. Measurements of CSF biochemical tumor markers in patients with meningeal carcinomatosis and brain tumors. J Neurooncol 1992;12:111-20.
38. Wang P, Piao Y, Zhang X, Li W, Hao X. The concentration of CYFRA 21-1, NSE and CEA in cerebro-spinal fluid can be useful indicators for diagnosis of meningeal carcinomatosis of lung cancer. Cancer Biomark 2013;13:123-30.
39. Khwaja FW, Nolen JD, Mendrinos SE, Lewis MM, Olson JJ, Pohl J, Van Meir EG, Ritchie JC, Brat DJ. Proteomic analysis of cerebrospinal fluid discriminates malignant and nonmalignant disease of the central nervous system and identifies specific protein markers. Proteomics 2006;6:6277-87.
40. Samuel N, Remke M, Rutka JT, Raught B, Malkin D. Proteomic analyses of CSF aimed at biomarker development for pediatric brain tumors. J Neurooncol 2014;118:225-38.
41. Qaddoumi I, Sane M, Li S, Kocak M, Pai-Panandiker A, Harreld J, Klimo P, Wright K, Broniscer A, Gajjar A. Diagnostic utility and correlation of tumor markers in the serum and cerebrospinal fluid of children with intracranial germ cell tumors. Childs Nerv Syst 2012;28:1017-24.
42. Nishizaki T, Kajiwara K, Adachi N, Tsuha M, Nakayama H, Ohshita N, Ikeda N, Ito H, Suzuki M. Detection of craniospinal dissemination of intracranial germ cell tumours based on serum and cerebrospinal fluid levels of tumour markers. J Clin Neurosci 2001;8:27-30.
43. Watanabe S, Aihara Y, Kikuno A, Sato T, Komoda T, Kubo O, Amano K, Okada Y, Koyamaishi Y. A highly sensitive and specific chemiluminescent enzyme immunoassay for placental alkaline phosphatase in the cerebrospinal fluid of patients with intracranial germinomas. Pediatr Neurosurg 2012;48:141-5.
44. Miyanohara O, Takeshima H, Kaji M, Hirano H, Sawamura Y, Kochi M, Kuratsu J. Diagnostic significance of soluble c-kit in the cerebrospinal fluid of patients with germ cell tumors. J Neurosurg 2002;97:177-83.
45. Ilhan-Mutlu A, Wagner L, Wohrer A, Jungwirth S, Marosi C, Fischer P, Preusser M. Blood alterations preceding clinical manifestation of glioblastoma. Cancer Invest 2012;30:625-9.
46. Khwaja FW, Reed MS, Olson JJ, Schmotzer BJ, Gillespie GY, Guha A, Groves MD, Kesari S, Pohl J, Van Meir EG. Proteomic identification of biomarkers in the cerebrospinal fluid (CSF) of astrocytoma patients. J Proteome Res 2007;6:559-70.
47. Khwaja FW, Duke-Cohan JS, Brat DJ, Van Meir EG. Attractin is elevated in the cerebrospinal fluid of patients with malignant astrocytoma and mediates glioma cell migration. Clin Cancer Res 2006;12:6331-6.
48. Sampath P, Weaver CE, Sungarian A, Cortez S, Alderson L, Stopa EG. Cerebrospinal fluid (vascular endothelial growth factor) and serologic (recoverin) tumor markers for malignant glioma. Cancer Control 2004;11:174-80.
49. Schuhmann MU, Zucht HD, Nassimi R, Heine G, Schneekloth CG, Stuerenburg HJ, Selle H. Peptide screening of cerebrospinal fluid in patients with glioblastoma multiforme. Eur J Surg Oncol 2010;36:201-7.
50. Ohnishi M, Matsumoto T, Nagashio R, Kageyama T, Utsuki S, Oka H, Okayasu I, Sato Y. Proteomics of tumor-specific proteins in cerebrospinal fluid of patients with astrocytoma: usefulness of gelsolin protein. Pathol Int 2009;59:797-803.
51. Saratsis AM, Yadavilli S, Magge S, Rood BR, Perez J, Hill DA, Hwang E, Kilburn L, Packer RJ, Nazarian J. Insights into pediatric diffuse intrinsic pontine glioma through proteomic analysis of cerebrospinal fluid. Neuro Oncol 2012;14:547-60.
52. Galati D, Di Noto R, Del Vecchio L. Diagnostic strategies to investigate cerebrospinal fluid involvement in haematological malignancies. Leuk Res 2013;37:231-7.
53. Huttner A. Overview of primary brain tumors: pathologic classification, epidemiology, molecular biology, and prognostic markers. Hematol Oncol Clin North Am 2012;26:715-32.
54. Le Rhun E, Taillibert S, Chamberlain MC. Carcinomatous meningitis: Leptomeningeal metastases in solid tumors. Surg Neurol Int 2013;4:S265-88.
55. Roy S, Josephson SA, Fridlyand J, Karch J, Kadoch C, Karrim J, Damon L, Treseler P, Kunwar S, Shuman MA, Jones T, Becker CH, Schulman H, Rubenstein JL. Protein biomarker identification in the CSF of patients with CNS lymphoma. J Clin Oncol 2008;26:96-105.
56. Kuusisto ME, Haapasaari KM, Remes AM, Bloigu R, Karihtala P, Turpeenniemi-Hujanen T, Kuittinen O. Antithrombin III is probably not a suitable biomarker for diagnosis of primary central nervous system lymphoma. Ann Hematol 2015;94:1167-74.
57. Zetterberg H, Andreasson U, Blennow K. CSF antithrombin III and disruption of the blood-brain barrier. J Clin Oncol 2009;27:2302-3; author reply 3-4.
58. Brunn A, Montesinos-Rongen M, Strack A, Reifenberger G, Mawrin C, Schaller C, Deckert M. Expression pattern and cellular sources of chemokines in primary central nervous system lymphoma. Acta Neuropathol 2007;114:271-6.
59. Sasagawa Y, Akai T, Tachibana O, Iizuka H. Diagnostic value of interleukin-10 in cerebrospinal fluid for diffuse large B-cell lymphoma of the central nervous system. J Neurooncol 2015;121:177-83.
60. Rajagopal MU, Hathout Y, MacDonald TJ, Kieran MW, Gururangan S, Blaney SM, Phillips P, Packer R, Gordish-Dressman H, Rood BR. Proteomic profiling of cerebrospinal fluid identifies prostaglandin D2 synthase as a putative biomarker for pediatric medulloblastoma: A pediatric brain tumor consortium study. Proteomics 2011;11:935-43.
61. Saso L, Leone MG, Sorrentino C, Giacomelli S, Silvestrini B, Grima J, Li JC, Samy E, Mruk D, Cheng CY. Quantification of prostaglandin D synthetase in cerebrospinal fluid: a potential marker for brain tumor. Biochem Mol Biol Int 1998;46:643-56.
62. Desiderio C, D'Angelo L, Rossetti DV, Iavarone F, Giardina B, Castagnola M, Massimi L, Tamburrini G, Di Rocco C. Cerebrospinal fluid top-down proteomics evidenced the potential biomarker role of LVV- and VV-hemorphin-7 in posterior cranial fossa pediatric brain tumors. Proteomics 2012;12:2158-66.
63. Figarella-Branger D, Dubois C, Chavin P, De Victor B, Gentet JC, Rougon G. Correlation between polysialic-neural cell adhesion meolecule levels in CSF and medulloblastoma outcomes. J Clin Oncol 1996;14:2066-72.
64. Fedarko NS, Jain A, Karadag A, Van Eman MR, Fisher LW. Elevated serum bone sialoprotein and osteopontin in colon, breast, prostate, and lung cancer. Clin Cancer Res 2001;7:4060-6.
65. Kao CL, Chiou SH, Ho DM, Chen YJ, Liu RS, Lo CW, Tsai FT, Lin CH, Ku HH, Yu SM, Wong TT. Elevation of plasma and cerebrospinal fluid osteopontin levels in patients with atypical teratoid/rhabdoid tumor. Am J Clin Pathol 2005;123:297-304.
66. de Bont JM, van Doorn J, Reddingius RE, Graat GH, Passier MM, den Boer ML, Pieters R. Various components of the insulin-like growth factor system in tumor tissue, cerebrospinal fluid and peripheral blood of pediatric medulloblastoma and ependymoma patients. Int J Cancer 2008;123:594-600.
67. de Bont JM, Vanderstichele H, Reddingius RE, Pieters R, van Gool SW. Increased total-Tau levels in cerebrospinal fluid of pediatric hydrocephalus and brain tumor patients. Eur J Paediatr Neurol 2008;12:334-41.
68. Albulescu R, Codrici E, Popescu ID, Mihai S, Necula LG, Petrescu D, Teodoru M, Tanase CP. Cytokine patterns in brain tumour progression. Mediators Inflamm 2013;2013:979748.
69. Zhao G, Yang Y, Su X. Determination of S-100 beta protein in cerebrospinal fluid of patients with metastatic carcinoma to central nervous system. Zhonghua Nei Ke Za Zhi 2000;39:732-4. (in Chinese)
70. Wang P, Piao Y, Zhang X, Li W, Hao X. The concentration of CYFRA 21-1, NSE and CEA in cerebro-spinal fluid can be useful indicators for diagnosis of meningeal carcinomatosis of lung cancer. Cancer Biomark 2013;13:123-30.
71. Bakri Y, al-Hawashim N, Berkowitz R. CSF/serum beta-hCG ratio in patients with brain metastases of gestational trophoblastic tumor. J Reprod Med 2000;45:94-6.
72. de Bont JM, den Boer ML, Reddingius RE, Jansen J, Passier M, van Schaik RH, Kros JM, Sillevis Smitt PA, Luider TH, Pieters R. Identification of apolipoprotein A-II in cerebrospinal fluid of pediatric brain tumor patients by protein expression profiling. Clin Chem 2006;52:1501-9.
73. Groves MD, Hess KR, Puduvalli VK, Colman H, Conrad CA, Gilbert MR, Weinberg J, Cristofanilli M, Yung WK, Liu TJ. Biomarkers of disease: cerebrospinal fluid vascular endothelial growth factor (VEGF) and stromal cell derived factor (SDF)-1 levels in patients with neoplastic meningitis (NM) due to breast cancer, lung cancer and melanoma. J Neurooncol 2009;94:229-34.
74. Bougel S, Lhermitte B, Gallagher G, de Flaugergues JC, Janzer RC, Benhattar J. Methylation of the hTERT promoter: a novel cancer biomarker for leptomeningeal metastasis detection in cerebrospinal fluids. Clin Cancer Res 2013;19:2216-23.
75. Chamberlain MC, Johnston SK. Neoplastic meningitis: survival as a function of cerebrospinal fluid cytology. Cancer 2009;115:1941-6.
76. Shalaby T, Fiaschetti G, Baumgartner M, Grotzer MA. MicroRNA signatures as biomarkers and therapeutic target for CNS embryonal tumors: the pros and the cons. Int J Mol Sci 2014;15:21554-86.
77. Baraniskin A, Kuhnhenn J, Schlegel U, Chan A, Deckert M, Gold R, Maghnouj A, Zollner H, Reinacher-Schick A, Schmiegel W, Hahn SA, Schroers R. Identification of microRNAs in the cerebrospinal fluid as marker for primary diffuse large B-cell lymphoma of the central nervous system. Blood 2011;117:3140-6.
78. Baraniskin A, Kuhnhenn J, Schlegel U, Maghnouj A, Zollner H, Schmiegel W, Hahn S, Schroers R. Identification of microRNAs in the cerebrospinal fluid as biomarker for the diagnosis of glioma. Neuro Oncol 2012;14:29-33.
79. Scott BJ, Douglas VC, Tihan T, Rubenstein JL, Josephson SA. A systematic approach to the diagnosis of suspected central nervous system lymphoma. JAMA Neurol 2013;70:311-9.
80. Turner JD, Williamson R, Almefty KK, Nakaji P, Porter R, Tse V, Kalani MY. The many roles of microRNAs in brain tumor biology. Neurosurg Focus 2010;28:E3.
81. Wei D, Wan Q, Li L, Jin H, Liu Y, Wang Y, Zhang G. MicroRNAs as Potential Biomarkers for Diagnosing Cancers of Central Nervous System: a Meta-analysis. Mol Neurobiol 2015;51:1452-61.
82. Shalaby T FG, Baulande S, Gerber NU, Baumgartner M, Grotzer MA. Detection and quantification of extracellular microRNAs in medulloblastoma. J Cancer Metastasis Treat 2015;1:67-75.
83. Fuzery AK, Levin J, Chan MM, Chan DW. Translation of proteomic biomarkers into FDA approved cancer diagnostics: issues and challenges. Clin Proteomics 2013;10:13.
84. Teunissen CE, Petzold A, Bennett JL, Berven FS, Brundin L, Comabella M, Franciotta D, Frederiksen JL, Fleming JO, Furlan R, Hintzen RQ, Hughes SG, Johnson MH, Krasulova E, Kuhle J, Magnone MC, Rajda C, Rejdak K, Schmidt HK, van Pesch V, Waubant E, Wolf C, Giovannoni G, Hemmer B, Tumani H, Deisenhammer F. A consensus protocol for the standardization of cerebrospinal fluid collection and biobanking. Neurology 2009;73:1914-22.
85. Phi JH, Lee J, Wang KC, Cho BK, Kim IO, Park CK, Kim CY, Ahn HS, Kim IH, Kim SK. Cerebrospinal fluid M staging for medulloblastoma: reappraisal of Chang's M staging based on the CSF flow. Neuro Oncol 2011;13:334-44.
86. Ho CY, VandenBussche CJ, Huppman AR, Chaudhry R, Ali SZ. Cytomorphologic and clinicoradiologic analysis of primary nonhematologic central nervous system tumors with positive cerebrospinal fluid. Cancer Cytopathol 2015;123:123-35.
87. Parmar H, Hawkins C, Bouffet E, Rutka J, Shroff M. Imaging findings in primary intracranial atypical teratoid/rhabdoid tumors. Pediatr Radiol 2006;36:126-32.
88. Zarovnaya EL, Pallatroni HF, Hug EB, Ball PA, Cromwell LD, Pipas JM, Fadul CE, Meyer LP, Park JP, Biegel JA, Perry A, Rhodes CH. Atypical teratoid/rhabdoid tumor of the spine in an adult: case report and review of the literature. J Neurooncol 2007;84:49-55.
89. Hilden JM, Meerbaum S, Burger P, Finlay J, Janss A, Scheithauer BW, Walter AW, Rorke LB, Biegel JA. Central nervous system atypical teratoid/rhabdoid tumor: results of therapy in children enrolled in a registry. J Clin Oncol 2004;22:2877-84.
90. Laurent VE, Sampor C, Solernou V, Rossi J, Gabri M, Eandi-Eberle S, de Davila MT, Alonso DF, Chantada GL. Detection of minimally disseminated disease in the cerebrospinal fluid of children with high-risk retinoblastoma by reverse transcriptase-polymerase chain reaction for GD2 synthase mRNA. Eur J Cancer 2013;49:2892-9.
91. Bakhshi S, Meel R, Kashyap S, Sharma S. Bone marrow aspirations and lumbar punctures in retinoblastoma at diagnosis: correlation with IRSS staging. J Pediatr Hematol Oncol 2011;33:e182-5.
92. Jennings MT, Gelman R, Hochberg F. Intracranial germ-cell tumors: natural history and pathogenesis. J Neurosurg 1985;63:155-67.
93. Chang SM GA, Newton HB, Vogelbaum MA. Principles and practice of neuro-oncology. A multidisciplinary approach. Demos Medical Publishing, LLC, New York 2011.
94. Benesch M, Wagner S, Berthold F, Wolff JE. Primary dissemination of high-grade gliomas in children: experiences from four studies of the Pediatric Oncology and Hematology Society of the German Language Group (GPOH). J Neurooncol 2005;72:179-83.
95. Wagner S, Benesch M, Berthold F, Gnekow AK, Rutkowski S, Strater R, Warmuth-Metz M, Kortmann RD, Pietsch T, Wolff JE. Secondary dissemination in children with high-grade malignant gliomas and diffuse intrinsic pontine gliomas. Br J Cancer 2006;95:991-7.
96. Walker DA PG, Punt JAG, Taylor RE. Brain and spinal tumors of childhood. Abingdon, Oxon: CRC Press 2004 .
99. Moon JH, Jung TY, Jung S, Jang WY. Leptomeningeal Dissemination of a Low-Grade Brainstem Glioma without Local Recurrence. J Korean Neurosurg Soc 2012;51:109-12.
100. Hukin J, Siffert J, Velasquez L, Zagzag D, Allen J. Leptomeningeal dissemination in children with progressive low-grade neuroepithelial tumors. Neuro Oncol 2002;4:253-60.
101. Leach PA, Estlin EJ, Coope DJ, Thorne JA, Kamaly-Asl ID. Diffuse brainstem gliomas in children: should we or shouldn't we biopsy? Br J Neurosurg 2008;22:619-24.
102. Valadi H, Ekstrom K, Bossios A, Sjostrand M, Lee JJ, Lotvall JO. Exosome-mediated transfer of mRNAs and microRNAs is a novel mechanism of genetic exchange between cells. Nat Cell Biol 2007;9:654-9.
103. Hunter MP, Ismail N, Zhang X, Aguda BD, Lee EJ, Yu L, Xiao T, Schafer J, Lee ML, Schmittgen TD, Nana-Sinkam SP, Jarjoura D, Marsh CB. Detection of microRNA expression in human peripheral blood microvesicles. PLoS One 2008;3:e3694.
104. Mathivanan S, Ji H, Simpson RJ. Exosomes: extracellular organelles important in intercellular communication. J Proteomics 2010;73:1907-20.
105. Kosaka N, Iguchi H, Ochiya T. Circulating microRNA in body fluid: a new potential biomarker for cancer diagnosis and prognosis. Cancer Sci 2010;101:2087-92.
106. Kosaka N, Iguchi H, Yoshioka Y, Takeshita F, Matsuki Y, Ochiya T. Secretory mechanisms and intercellular transfer of microRNAs in living cells. J Biol Chem 2010;285:17442-52.
107. Rubenstein JL, Wong VS, Kadoch C, Gao HX, Barajas R, Chen L, Josephson SA, Scott B, Douglas V, Maiti M, Kaplan LD, Treseler PA, Cha S, Hwang JH, Cinque P, Cyster JG, Lowell C. CXCL13 plus interleukin 10 is highly specific for the diagnosis of CNS lymphoma. Blood 2013;121:4740-8.
108. Lai NS, Wu DG, Fang XG, Lin YC, Chen SS, Li ZB, Xu SS. Serum microRNA-210 as a potential noninvasive biomarker for the diagnosis and prognosis of glioma. Br J Cancer 2015;112:1241-6.
109. Sasayama T, Nakamizo S, Nishihara M, Kawamura A, Tanaka H, Mizukawa K, Miyake S, Taniguchi M, Hosoda K, Kohmura E. Cerebrospinal fluid interleukin-10 is a potentially useful biomarker in immunocompetent primary central nervous system lymphoma (PCNSL). Neuro Oncol 2012;14:368-80.
110. Fischer L, Korfel A, Pfeiffer S, Kiewe P, Volk HD, Cakiroglu H, Widmann T, Thiel E. CXCL13 and CXCL12 in central nervous system lymphoma patients. Clin Cancer Res 2009;15:5968-73.
111. Murase S, Saio M, Andoh H, Takenaka K, Shinoda J, Nishimura Y, Sakai N, Takami T. Diagnostic utility of CSF soluble CD27 for primary central nervous system lymphoma in immunocompetent patients. Neurol Res 2000;22:434-42.
112. Pantazis G, Psaras T, Krope K, von Coelln R, Fend F, Bock T, Schittenhelm J, Melms A, Meyermann R, Bornemann A. Cerebral low-grade lymphoma and light chain deposition disease: exceedingly high IgG levels in the cerebrospinal fluid as a diagnostic clue. Clin Neuropathol 2010;29:378-83.
113. Yang H, Cai L, Zhang Y, Tan H, Deng Q, Zhao M, Xu X. Sensitive detection of EGFR mutations in cerebrospinal fluid from lung adenocarcinoma patients with brain metastases. J Mol Diagn 2014;16:558-63.
114. Shnaper S, Desbaillets I, Brown DA, Murat A, Migliavacca E, Schluep M, Ostermann S, Hamou MF, Stupp R, Breit SN, de Tribolet N, Hegi ME. Elevated levels of MIC-1/GDF15 in the cerebrospinal fluid of patients are associated with glioblastoma and worse outcome. Int J Cancer 2009;125:2624-30.
115. Baraniskin A, Kuhnhenn J, Schlegel U, Maghnouj A, Zollner H, Schmiegel W, Hahn S, Schroers R. Identification of microRNAs in the cerebrospinal fluid as biomarker for the diagnosis of glioma. Neuro Oncol 2012;14:29-33.
116. Teplyuk NM, Mollenhauer B, Gabriely G, Giese A, Kim E, Smolsky M, Kim RY, Saria MG, Pastorino S, Kesari S, Krichevsky AM. MicroRNAs in cerebrospinal fluid identify glioblastoma and metastatic brain cancers and reflect disease activity. Neuro Oncol 2012;14:689-700.
117. Shi R, Wang PY, Li XY, Chen JX, Li Y, Zhang XZ, Zhang CG, Jiang T, Li WB, Ding W, Cheng SJ. Exosomal levels of miRNA-21 from cerebrospinal fluids associated with poor prognosis and tumor recurrence of glioma patients. Oncotarget 2015;6:26971-81.
118. Drusco A, Bottoni A, Lagana A, Acunzo M, Fassan M, Cascione L, Antenucci A, Kumchala P, Vicentini C, Gardiman MP, Alder H, Carosi MA, Ammirati M, Gherardi S, Luscri M, Carapella C, Zanesi N, Croce CM. A differentially expressed set of microRNAs in cerebro-spinal fluid (CSF) can diagnose CNS malignancies. Oncotarget 2015;6:20829-39.
Cite This Article
Export citation file: BibTeX | RIS
OAE Style
Shalaby T, Achini F, Grotzer MA. Targeting cerebrospinal fluid for discovery of brain cancer biomarkers. J Cancer Metastasis Treat 2016;2:176-87. http://dx.doi.org/10.20517/2394-4722.2016.12
AMA Style
Shalaby T, Achini F, Grotzer MA. Targeting cerebrospinal fluid for discovery of brain cancer biomarkers. Journal of Cancer Metastasis and Treatment. 2016; 2: 176-87. http://dx.doi.org/10.20517/2394-4722.2016.12
Chicago/Turabian Style
Shalaby, Tarek, Federica Achini, Michael A. Grotzer. 2016. "Targeting cerebrospinal fluid for discovery of brain cancer biomarkers" Journal of Cancer Metastasis and Treatment. 2: 176-87. http://dx.doi.org/10.20517/2394-4722.2016.12
ACS Style
Shalaby, T.; Achini F.; Grotzer MA. Targeting cerebrospinal fluid for discovery of brain cancer biomarkers. J. Cancer. Metastasis. Treat. 2016, 2, 176-87. http://dx.doi.org/10.20517/2394-4722.2016.12
About This Article
Special Issue
Copyright
Author Biographies
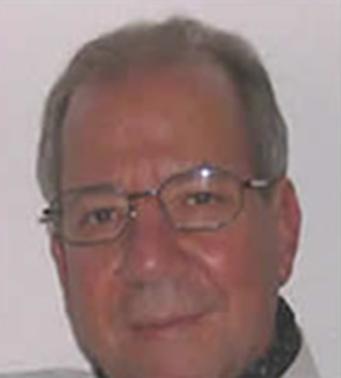
Data & Comments
Data

Comments
Comments must be written in English. Spam, offensive content, impersonation, and private information will not be permitted. If any comment is reported and identified as inappropriate content by OAE staff, the comment will be removed without notice. If you have any queries or need any help, please contact us at support@oaepublish.com.