Long non-coding RNAs as key regulators of cancer metastasis
Abstract
The recent advances in functional genomics have discovered that a large number of long non-coding RNAs (lncRNAs) are pervasively transcribed from the human genome. Increasing evidence further indicates that lncRNAs are important for gene expression during cell differentiation and development through various mechanisms such as nuclear organization, post-transcription regulation, alternative splicing, and epigenetic regulation. Thus, aberrant expression of lncRNAs can cause abnormality in those cellular functions and lead to various pathological conditions. One of such fatal consequences is cancer metastasis which is responsible for more than 90% of cancer-related deaths. A good understanding of how lncRNAs regulate different genetic and epigenetic changes during different stages of cancer metastasis is important not only for general cancer biology but also for identifi cation of novel biomarkers and therapeutic targets for treatment of metastatic cancer. A significant progress has been made regarding the role of lncRNAs in cancer for past several years. In this study, we first discuss general functions of lncRNAs and then highlight recent fi ndings of how lncRNAs impact cancer metastasis, and finally we provide our perspectives on clinical implications of lncRNAs.
Keywords
Introduction
It is well-known now that protein-coding genes account only about 2% of the human genome,[1] whereas the vast majority of the transcripts do not code for protein.[2] Although these non-coding RNAs were considered “transcriptional noise”, their functions are increasingly valued for defining the cellular complexity of organisms. For instance, the number of protein-coding genes in humans is only a 2-fold more than that in worms such as Caenorhabditis elegans do,[1] implying that the protein alone is not sufficient to determine the complexity of organisms. Instead, this complexity may be achieved by efficient programming, which helps in handy expression and functioning of protein in a different context. The versatility and plasticity of non-coding RNAs help in such programming of protein function by regulating their expression and assembly in contextual cues.[3]
Non-coding RNAs include a broad category of RNA molecules. Some of them are constitutively expressed in the cells, and they may play a housekeeping role such as ribosomal RNA, transfer RNA, small nuclear RNA, and small nucleolar RNA (snoRNA). In contrast, other non-coding RNAs may be spatiotemporally expressed, and they often play a regulatory role.
These regulatory RNAs, on the basis of their size, are arbitrarily classifi ed into two groups. The first group is small non-coding RNAs (< 200 bp in length) such as short interfering RNA, microRNA (miRNA), and piwiRNA. The second group of non-coding regulatory RNAs is long non-coding RNAs (lncRNAs) (> 200 bp in length).[4,5] Like protein-coding genes, most lncRNAs are polyadenylated and capped.[6] Both protein-coding genes [messenger RNA (mRNA)] and lncRNAs carry genetic information [Figure 1]; however, their functions can be very different. Based on their locations in the genome, lncRNAs can be derived through the following means:[7] (1) intergenic lncRNAs which are located between two genes; (2) sense or antisense lncRNAs which may overlap with an exon of another transcript in the same or opposite direction; (3) intronic lncRNAs which reside within an intron and do not overlap with any exon; (4) processed transcripts which reside in a locus where none of the transcript has an open reading frame and thus, do not fit into any other categories because of structural complexity.
To date, an overwhelming number of lncRNAs have been reported. For example, the non-code database lists over 56,000 human lncRNAs (http://www.noncode.org) whereas LNCipedia (http://www.lncipedia.org/) lists over 110,000 human lncRNAs. Although no unified source for categorizing and annotating lncRNAs is available yet, evidently, the number of lncRNAs is much larger than the number of protein-coding genes. Since lncRNA research is still at a very early stage and the majority of lncRNAs are poorly characterized, there is a critical need for a better understanding of their functions and role in cancer, and especially, how they impact metastasis.
LncRNAs as master gene regulators
Given that they can interact with RNA, DNA, and protein, lncRNAs have been shown to have an impact on almost every aspect of gene regulation. We list a few of examples as follows [Table 1].
Function of lncRNAs
LncRNA | Function |
---|---|
ANRIL, XIST, HOTAIR, H-19 | Transcription control by chromatin modifi cations |
LincRNA p21 | Transcriptional regulation |
H-19 | Precursor for miRNA |
Loc285194, Gas 5, lncRNA-ATB, CCAT1 | Regulators of miRNA function |
PTENP1, KRAS1P, Gas 5 | Decoy |
RoR, NEAT1, TER | Scaffold |
Transcriptional regulation
Histone modifications such as acetylation and methylation impact chromatin structure and subsequent transcriptional activity. A large number of lncRNAs have been shown to play a role in regulation of chromatin structure. Polycomb repressive complex (PRC1 and PRC2) consists of several enzymes, including enhancer of zest homolog 2 (EZH2), and is essential for histone methylation.[8] Antisense non-coding RNA in the INK4 locus (ANRIL) is one of the lncRNAs that can suppress transcription by remodeling chromatin structure. In this regard, the human chromosome 9p21 harbors INK4b/ARF/INK4a locus which has 3 coding genes, p14/ARF, p15/CDKN2B, and p16/CDKN2A along with ANRIL. ANRIL is an antisense lncRNA, overlapping with p15/CDKN2B and p16/CDKN2A.[9] Binding of ANRIL to PRC1 and PRC2 facilitates the recruitment of PRC1 and PRC2 into the INK4a/ARF locus, which causes trimethylation of the histone and reduces transcription activity of the locus.[10] Similarly, X-inactive specific transcript (XIST) is a key regulator of X chromosome activity by chromatin structure modifications during embryonic development. XIST recruits EZH2 in X chromosome and then causes the trimethylation of histone, leading to a factual heterochromatin structure and silencing of one of the two X chromosomes.[11] Besides, recruitment of different proteins in a gene promoter region can also change the transcription activity. For example, the enrichment of hnRNP-K in the promoters of p53 regulated genes represses the transcription of those genes.[12] However, lincRNA-p21 interacts with hnRNP-K and helps enrichment of hnRNP-K into these promoters, resulting in transcription suppression.[13] On the other hand, p53 enhances transcription of lincRNA-p21, thus forming an auto-regulatory feedback loop.
LncRNA as a progenitor of small RNAs, regulating their functions
Although there is still no concrete evidence yet, lncRNAs may be post-transcriptionally processed into the small RNAs. For instance, the computational analysis indicated that exons of lncRNAs are highly enriched with small RNAs. In fact, snoRNAs are enriched 6-fold higher in their exons than any other genomic loci.[14] Similarly, many miRNAs are derived from transcripts which are capped and polyadenylated including lncRNAs. About 20% miRNAs are overlapped with either introns or exons of lncRNAs.[15] LncRNA H19 is one such prominent example which serves as the precursor of miRNA. The pri- and pre-miR-675 resides in H19 and expression of miR-675 coincides with H19 in murine embryo. However, miR-675 is not expressed in NIH3T3 cells that lack H19 expression. The digestion of 32P-labeled H19 clone with Drosha: DGCR8 (enzyme for miRNA biogenesis) releases 57 nucleotide long pre-miRNA 675, indicating that H19 is the parental transcript of miR-675.[16]
LncRNAs not only serve as the precursor for the small RNAs but also regulate the expression and the function of miRNAs. The lncRNAs provide putative binding sites for miRNAs. Such interactions alter the expression and the function of mature miRNAs. For example, loc285194 is a tumor suppressor in colon cancer and it carries two binding sites for oncogenic miR-211. This interaction does not affect the pri- and pre-miR-211 level but alters the mature miR-211.[17] Similarly, growth arrest-specific 5 (Gas5) regulates the level of miR-21 through their interaction. Apparently, Gas5 does not affect the pre- and pri-miR-21. Moreover, both miR-21 and Gas5 are found in RNA-induced silencing complex (RISC), suggesting that Gas5 regulates miR-21 through RNA interference (RNAi) mechanism.[18]
LncRNAs as a decoy
A pseudogene is a class of lncRNAs, derived from mutations in protein-coding genes. They usually have similar sequences to their parental gene with few mismatches. This resemblance in structure could entice different cellular entities as lncRNAs rather than mRNAs, impacting the cellular function. For example, PTENP1 is a mutated form of PTEN and their sequences differ by only 18 mismatches. PTEN carries a number of different sites for miRNA in its untranslated region (3’-UTR). Although PTENP1 is 1 kb shorter in the 3’-UTR than PTEN, most of the miRNA binding sites are conserved. This can trap many miRNAs to PTENP1 to compete with PTEN.[19] A similar relationship was also observed between Kras and its pseudogene KRAS1P. KRAS1P is amplified in most of cancers with activated Kras, indicating a positive correlation between them. Although how KRAS1P regulates KRAS level is not well-understood, it may act as a sponge for miRNAs that bind to the 3’-UTR of Kras and prevents degradation of Kras transcript.[19] The lncRNA decoy function is not limited to miRNAs, and it can also be applied to DNA. For instance, Gas5, which is enriched in growth-arrested cells,[20] inhibits the function of glucocorticoid receptor (GR) by competing with glucocorticoid response element (GRE) to bind GR. GR is a transcription factor and is activated by ligand and subsequently the activated GR binds to GRE to initiate transcription of downstream genes. A part of Gas5 sequence is capable of forming 6 hairpin structures; among them, hairpin structure 5 has two GRE-like structures that mimic GRE. Therefore, GR could bind Gas5 instead of GRE, and as a result, this interaction hinders the GR-mediated transcription activity.[21]
LncRNAs as a scaffolding and structural support
Physical association between cellular entities is critically important for coordination of a variety of cellular functions. It is well-known that specific binding between two different cellular components can control the reprogramming of cellular signaling, leading to alternations of cell phenotype or function. Apparently, proteins can serve such function as a scaffolding and structural support.[22] Recent studies suggest that lncRNAs can also have a similar function because they can interact with different proteins, through which lncRNAs provide a platform for the assembly of various proteins. Such interactions may affect protein localization, protein function, transcriptional activity, gene splicing, etc. Linc-ROR is lncRNA as a regulator of induced pluripotent cell reprogramming.[23] Of interest, linc-ROR plays an important role in repression of p53 translation by interaction with phosphorylated hnRNP I in the cytoplasm. The physical association between linc-ROR and hnRNP I controls p53 translation and deletion of hnRNP I binding motif in linc-ROR abolishes its repression capability.[24] The scaffolding function of lncRNAs is also essential for the formation of special architect-like paraspeckles, a nuclear body structure that appears during interphase of cell cycle. Paraspeckles are primarily composed of proteins such as paraspeckle protein (PSP1, PSP2) and p54/nrb.[25] Although the function of paraspeckles is still not clear, components within paraspeckles are known to play an important role for transcription and alternative splicing.[26,27] Since paraspeckles do not have any membrane structure, lncRNAs within the paraspeckle may help to establish this compartment.[28] NEAT1 is one of the lncRNAs that interact with PSP1 and together, they may help to form paraspeckles. Importantly, the number of paraspeckles increases in vivo and in vitro with the increase in NEAT 1 expression and deletion of NEAT1 eliminates the paraspeckles, suggesting an important role of this lncRNA in the formation of paraspeckles.[29] Another lncRNA that provides a platform for the binding of protein is telomerase RNA (TER). TER along with telomerase reverse transcriptase (TERT) is essential for telomere synthesis. A telomere is nucleotide repeat at the end of DNA which is required for the genomic stability.[30] TER is a template RNA for the synthesis of telomere.[31] TER consists of various motifs; core domain is essential for template activity and CR4/CR5 domain binds with TERT.[32] The mutation in core domain of TER disintegrates proper structure of this RNA, losing the binding capability to TERT, which leads to aplastic anemia.[33] This indicates that a comprehensive structure of TER functions as a scaffold for telomerase to bind and work properly.
Cancer metastasis is a multistep process
More than 90% of cancer death is attributed to metastasis. Cancer metastasis is a process in which cancer cells migrate from its origin to a distant site and then proliferate. The two major steps of metastasis are physical dissociation from the origin of the primary tumor and migration to distant sites and colonization of those migrating cells in the distant sites.[34]
This multistep process is usually inefficient and only very few cells that start migrating from the origin would be able to colonize in distant organs. The disaggregation of cells from the primary tumor is the first event during the metastasis. This process is greatly enhanced by loss of E-cadherin, a protein, that helps to adhere epithelial cells together.[35,36] The next barrier that prevents migration of the cancer cell is basement membrane. The disintegrated cancer cells can induce stroma to secrete proteolytic enzyme-like matrix metalloproteinases that dissolve the basal lamina.[37] Detached cells also require motility to move from one place to another. Several changes in cytoskeleton, interactions between cell and matrix, and induced Rho, cdc42, and Rac signaling are important for mobility. Furthermore, epithelial-mesenchymal transition (EMT) is critical for dissemination of the cancer cell to distant site as it helps in effective motility and invasiveness and survival of the cells.[38] To reach the distant organs, the cells also require a traveling path. The hematogenous route works as highway for this process.[39] Although it is still unclear how the altered cells invade into the blood vessels, the high invasive capacity of metastasizing cells and chemoattractive factors in the blood may help their intravasation. Inside the blood vessels, the migrating cells endure a constant physical pressure as well as immune responses, an inclement condition for tumor cells. However, the chance of survival can be increased by adhering tumor cells to different factors such as thrombin.[40] Tumor cells may also be able to attach to endothelial cells via protein-protein interaction (integrin α3β1 of tumor and laminin 5 of endothelial cells) to protect themselves from harsh condition.[41] The adherence of cells not only prevent their possible elimination, but also help their exit from the capillaries (extravasation). Extravasation is primarily supported by enhanced permeability of capillary. Vascular endothelial growth factor secreted by tumor cells can increase the permeability of blood vessels. In addition, other factors such as alterations in receptor expression and physical bursting may also help in the exit of tumor cells from blood vessels.[42]
Once the cells depart the capillary, they try to colonize in distant organs. However, few of circulating tumor cells can colonize to establish micro-metastases. The colonization is dictated by the microenvironment of primary site and distant site. A number of cytokines both from tumors and the site of colonization provide mitogenic signals for the successful proliferation, survival and resist to apoptosis in alien environment, finally to develop macro-metastases.[43]
LncRNAs and cancer metastasis
In the previous section, we highlighted the steps during metastasis. Several genetic and epigenetic modifications during this multistep process make cells sturdy to survive the foreign ambience. Although we still do not have a clear picture as to what causes those change, increasing evidence suggests that lncRNAs play a crucial role in different stages of metastasis [Figure 1]. We list the following lncRNAs as examples.
Metastasis-associated lung adenocarcinoma transcript 1
Metastasis-associated lung adenocarcinoma transcript 1 (MALAT1) is located at chromosome 11q13 with 8.7 kb in length. It is expressed in most tissues with the highest level in pancreas and lung. The elevated expression of MALAT1 was found in metastatic cases of non-small cell lung carcinoma, and as such, it was named MALAT1. The high expression of MALAT1 in metastatic tumors predicts poor prognosis.[44] As a nuclear lncRNA, MALAT1 plays an important role in alternative splicing.[45] However, loss of MALAT1 does not seem to affect alternative splicing in the lung cancer tissue; rather it affects expression of different genes, including those involved in EMT (LPHN2, ABC1) and others in regulation of metastasis formation (GPC6, MCAM, PRCKE). Furthermore, tail vein injection of xenograft mice with A549 cells overexpressing MALAT1 shows diffused growth in the lung. Knockdown of MALAT1 results in either fewer tumor nodules or the cells cannot exit out of endothelial cells.[46] Similarly, loss of MALAT1 in A549 cells inhibits expression of those genes (CTHRC1, CCT4, HMMR) that regulate folding of cytoskeleton and migration of cells.[47] These lines of evidence indicate that MALAT1 is critically important for the cell motility and extravasation of cells from capillaries.
In addition to lung cancer, MALAT1 is also important for pancreatic and cervical cancer metastasis.[48] In pancreatic cancer, MALAT1 enhances expression of EMT markers (loss of E-cadherin and gain of N-cadherin, vimentin, and slug) dependent on transforming growth factor-β (TGF-β). Similarly, MALAT1 was found highly expressed in cancer stem cells (CSCs) of pancreatic cancer and knockdown of MALAT1 in those cells greatly reduces CD133+ population and sphere formation,[49] suggesting an important role of CSC formation which may help in migration and survival of cells during metastasis.
HOX transcript antisense RNA
HOTAIR stands for HOX transcript antisense RNA with 2.2 kb in length. It is derived from HOX C gene (which determines anterior and posterior plane during embryonic development). High expression of HOTAIR is correlated with metastasis, and it signifi cantly decreases the chance of survival of those patients. Overexpression of HOTAIR in different cell lines increases cell invasion and transforms non-invasive cells into invasive cells in vivo. Similarly, overexpression of HOTAIR in breast cancer MDA-MB-231 cells increases metastatic lung nodules by a 10-fold as compared to control.[50]
Like many other lncRNAs, HOTAIR impacts metastasis via chromatin remodeling. HOTAIR can directly bind to PRC2 and LSD1, a demethylase that flanks HOXD. This binding coordinates enrichment of EZH2 in HOXD promoter which causes methylation of H3K27, leading to silencing of transcription through HOXD gene.[51] A similar mechanism was also observed in the metastatic breast cancer cell lines, where HOTAIR helps in PRC2 occupancy on promoter of hundreds of genes and silences them by trimethylation of H3K27. Many of those genes are involved in breast cancer progression, cell adhesion, and metastasis.[50]
In addition to breast cancer metastasis, HOTAIR has been attributed to enhance metastasis in oral squamous cell carcinoma by suppressing the level of E-cadherin.[52] Moreover, HOTAIR along with miR-196a is also associated with high-risk metastasis and poor survival of a patient with gastrointestinal stromal tumors.[53]
H19
H19 is one of the first lncRNAs identified in early 1980 and its expression is in accordance to expression of α-fetoprotein.[54] This gene represents a maternally imprinted gene in both humans and mice. The expression of H19 gradually decreases from fetal tissue to adult, which indicates its importance in embryo development.[55] Initial reports suggested that H19 could work as a tumor suppressor in different cancer cases;[56] however, other studies suggested that H19 expression is high in tumor tissues.[57,58] Despite high expression in tumor samples, overexpression of H19 in T24 bladder carcinoma cell line did not provide proliferative advantage. This implies that H19 may regulate metastasis rather than formation of primary tumor. Indeed, overexpression of H19 up-regulates genes [e.g. uPAR, tumor necrosis factor-α, interleukin-6 (IL-6), and Ezrin] that are required for angiogenesis and metastasis in T24 cells.[59] Furthermore, H19 is highly expressed in most cases of bladder carcinoma which subsequently metastasize compared to those that do not metastasize. Similarly, H19 level is substantially higher in invasive bladder carcinoma cell lines than non-invasive cell lines. Mechanistically, H19 recruits EZH2 in the promoter region of Nkd1 (an antagonist gene of Wnt/β-catenin) and suppresses its transcription by hyper-methylation. This makes Wnt/β-catenin constitutively active while E-cadherin is suppressed, leading to metastasis of bladder cancer.[60] In addition to alterations in the gene expression pattern, H19 also enhances the interaction of the tumor cell with extracellular matrix. MDA-MB-231 cells growing in three-dimensional culture exhibit high level of H19, which helps in enhanced scattering of the cells, suggesting a role of H19 in breast cancer metastasis.[61] In addition to bladder and breast cancer, H19 may also contribute to metastasis of colorectal cancer. For instance, H19 is highly expressed in methotrexate resistant HT-29 cells which reveal mesenchymal morphology. Overexpression of H19 increases the EMT markers vimentin, ZEB-1, and ZEB-2 and also promotes cell migration.[62]
Nuclear factor-κB interacting lncRNA
Nuclear factor-κB (NF-κB) interacting lncRNA (NKILA) is a 2.5 kb transcript mostly found in the cytoplasm and it negatively regulates the NF-κB signaling.[63] NF-κB is a transcription factor which mediates inflammatory signaling pathways and is often constitutively active in various cancer cells.[64] NF-κB is in an active (phosphorylated) or inactive state (dephosphorylated) in the cell. In the inactive state, the dimer NF-κB (p65 and p50) is bound with an inhibitory subunit IκB. This complex keeps the dimer in the cytoplasm by masking the nuclear localization signal. Several external stimuli activate IKKβ which phosphorylates IκBα (a subunit of IκB) and leads to proteasomal degradation of IκBα. Now, the free NF-κB dimer translocates to the nucleus where it binds to NF-κB response element and activates transcription of different genes.[65] Thus, NKILA adds a new layer of regulation for NF-κB activity, by interacting with IκBα and masking its phosphorylation site from IKKβ. This prevents phosphorylation of IκBα and translocation of NF-κB dimer from the cytoplasm to the nucleus.
The highly metastatic breast cancer cell lines express a very low level of NKILA while less aggressive breast cancer cell lines exhibit a high level of NKILA. Furthermore, overexpression of NKILA in MDA-MB-231 cells reduces their metastasis in the lung, liver, and lymph nodes. In contrast, knockdown of NKILA in MCF-7 cells increases their metastasis to those distant sites. Clinically, loss of NKILA is associated with advanced breast cancer and distant metastases; low expression of NKILA is associated with the patient survival.[63] Therefore, NKILA can predict the outcome of breast cancer and may serve as a prognostic marker.
LncRNA-ATB
This lncRNA is activated by cytokine TGF-β (lncRNA-ATB) that is well-known for its role in tumor metastasis. TGF-β modulates different signaling pathways involved in EMT, migration, invasion, and metastasis.[66-68] A long time treatment of cells with TGF-β induces EMT (decreased E-cadherin and increased N-cadherin, vimentin, slug, twist1, ZEB-1 and ZEB-2). Similar treatment of hepatocellular carcinoma (HCC) cells with TGF-β activates the lncRNA-ATB in a time- and dose-dependent manner. Clinically, lncRNA-ATB level is high in HCC tumors as compared to adjacent normal tissue. Similarly, a high level of lncRNA-ATB is positively correlated with microvascular invasion and portal vein tumor thrombosis. Consistent with these observations, injection of HCC tumor cells overexpressing lncRNA-ATB into orthotropic mice promotes metastasis to different organs.[69] One of the possible mechanisms is through enhancement of EMT by interfering the action of miR-200 which can inhibit EMT by suppressing ZEB-1 and ZEB-2.[70] This 2.5 kb long lncRNA carries 6 binding sites for miR-200. Therefore, lncRNA-ATB traps miR-200 and prevents degradation of ZEB-1 and ZEB-2 by miR-200. The high level of ZEB-1 and ZEB-2 ultimately promotes EMT and invasiveness of different cells in vitro and in vivo. In addition, lncRNA-ATB enhances colonization of migrating cells by enhancing the function of IL-11-STAT3 signaling pathway. In this case, lncRNA-ATB binds to IL-11 mRNA and stabilizes it. The increased stability of IL-11 facilitates its secretion. As a ligand, IL-11 promotes phosphorylation of STAT3. This autocrine mitogenic signal helps in robust cell survival and effective colonization in distant organs.[69]
LncRNA-low expression in tumor
Low expression in tumor (LET) was originally identified in HCC cells.[71] Along with HCC, a reduced level of LET is also found in lung squamous carcinoma and colorectal cancer as compared to adjacent normal tissue. Overexpression of lncRNA-LET suppresses metastasis of HCC and colon cancer cells in vivo.[72] LncRNA-LET could limit HCC metastasis in both hypoxic and normoxic condition by different mechanisms. In hypoxic condition, lncRNA-LET interferes with the function of hypoxia-inducible factor-1α (HIF-1α), a transcription factor that regulates a number of genes under tumor hypoxia, and promotes angiogenesis and metastasis.[73] The high expression of lncRNA-LET suppresses HIF-1α through inhibiting NF90 which is required for accumulation of HIF-1α mRNA. However, hypoxia keeps the level of lncRNA-LET low by deacetylating its promotor. As a result, HIF-1α is increased promoting metastasis. In normoxic condition, lncRNA-LET inhibits expression of CDC42 (which is required for trans-endothelial migration) of circulating tumor cells. The low level of lncRNA-LET in HCC keeps CDC42 high and this results in profound metastasis of HCC.[72]
Colon cancer-associated transcript 1
Colon cancer-associated transcript 1 (CCAT1) was found up-regulated in colon cancer tissue, circulating blood cells of colon cancer patient and metastasis cases, indicating its role in colon cancer progression.[74] Besides, high expression of CCAT1 is also associated with primary tumor tissue, lymph node metastasis, and metastatic cases of gastric carcinoma.[75] The elevated level of CCAT1 reduces the survival of HCC patients. In both gastric cancer and HCC cell lines, overexpression of CCAT1 enhances the proliferation and migration of cells driven by c-Myc, an oncogenic transcription factor required for cell survival. On one hand, c-Myc binds to promoter of the CCAT1 and up-regulates its level in cancer cells.[75] On the other hand, CCAT1 prevents degradation of c-Myc by interaction with let-7, a known miRNA that can target c-Myc through its 3’-UTR.[76]
Deregulation of lncRNA expression in cancer
It is well-known that most of lncRNAs are transcribed through RNA polymerase II, just like protein-coding genes; they are also spliced products via canonical genomic splice site motifs, frequently ended with a poly A tail. Importantly, lncRNAs are often regulated by well-established transcription factors and are expressed in a tissue-specific manner.[77] For example, we have shown that wild-type p53 can transcriptionally induce linc-RoR and loc285194, but mutant p53 cannot.[17,24] On the other hand, c-Myc, as an oncogene, can regulate a group of lncRNAs.[78] In cancer, c-Myc is often amplified or up-regulated, which may explain why some lncRNAs are often deregulated.
Conclusion and perspective
A tremendous progress has been made in our understanding of the genes and events involved in metastasis in recent years. Moreover, emerging evidence indicates that lncRNAs have also joined this complex regulatory network and may serve as very important regulators at different stages of metastasis (e.g. EMT, invasion, migration, and colonization) often through their expression levels [Figure 2]. However, overall, lncRNA research in this field is still at the infancy stage. Given the complex interactions of lncRNAs with DNA, RNA, and protein, a systematic approach may be needed to better understand the molecular mechanism of lncRNA-mediated metastasis. With the development of advanced technology such as CRISPR/Cas9, it is now feasible to perform knockout or knockin experiments and these research tools will no doubt speed up new discovery. In this system, nuclease Cas9 assisted by a sequence-specific guild RNA (gRNA) which is functionally similar to RNAi, cuts targeted DNA sequence.[79] Once the double strand break is made, the cell employs one of two major DNA repair mechanisms, non-homologous end joining (NHEJ), and homologous recombination (HR). Unlike HR, the NHEJ mechanism often leads to deletions or insertions, and thus it is an error-prone repair, a feature important for knockout. The HR mechanism would allow for introducing mutations or correcting a mutant sequence by knockin. Increasing evidence indicates that this technology has a potential to transform the field of cancer genetics such as the development of next-generation models of human cancer.[80]
Given the nuclear localization nature for a number of lncRNAs, genetic manipulations at the DNA level provides a better alternative to RNAi approach which mainly works through RISC complex in the cytoplasm. Our recent study indicates that a dual gRNA/Cas9 system combined with donor vector for HR can greatly improve the efficiency of obtaining complete lncRNA knockouts in various cancer cell lines.[81] As this field advances, we anticipate that more lncRNAs will be identified to be important players in cancer metastasis. More importantly, further characterization of this regulatory system will reveal many of detailed mechanisms. As a result, these studies will help develop novel strategies for cancer treatment. Furthermore, lncRNAs may serve as biomarkers for diagnosis/prognosis as supported by profiling studies of clinical specimens. Finally, given their important role in metastasis, lncRNAs may also prove to be valuable targets for cancer therapy. In particular, ribonucleoprotein complexes through lncRNAs are critical to lncRNA-mediated metastasis, drugs that block or enhance such interactions may have a bright future.
Financial support and sponsorship
This work was supported by NIH grant R01 CA154989 (YM).
Conflicts of interest
There are no conflicts of interest.
REFERENCES
1. Lander ES, Linton LM, Birren B, Nusbaum C, Zody MC, Baldwin J, Devon K, Dewar K, Doyle M, FitzHugh W, Funke R, Gage D, Harris K, Heaford A, Howland J, Kann L, Lehoczky J, LeVine R, McEwan P, McKernan K, Meldrim J, Mesirov JP, Miranda C, Morris W, Naylor J, Raymond C, Rosetti M, Santos R, Sheridan A, Sougnez C, Stange-Thomann N, Stojanovic N, Subramanian A, Wyman D, Rogers J, Sulston J, Ainscough R, Beck S, Bentley D, Burton J, Clee C, Carter N, Coulson A, Deadman R, Deloukas P, Dunham A, Dunham I, Durbin R, French L, Grafham D, Gregory S, Hubbard T, Humphray S, Hunt A, Jones M, Lloyd C, McMurray A, Matthews L, Mercer S, Milne S, Mullikin JC, Mungall A, Plumb R, Ross M, Shownkeen R, Sims S, Waterston RH, Wilson RK, Hillier LW, McPherson JD, Marra MA, Mardis ER, Fulton LA, Chinwalla AT, Pepin KH, Gish WR, Chissoe SL, Wendl MC, Delehaunty KD, Miner TL, Delehaunty A, Kramer JB, Cook LL, Fulton RS, Johnson DL, Minx PJ, Clifton SW, Hawkins T, Branscomb E, Predki P, Richardson P, Wenning S, Slezak T, Doggett N, Cheng JF, Olsen A, Lucas S, Elkin C, Uberbacher E, Frazier M, Gibbs RA, Muzny DM, Scherer SE, Bouck JB, Sodergren EJ, Worley KC, Rives CM, Gorrell JH, Metzker ML, Naylor SL, Kucherlapati RS, Nelson DL, Weinstock GM, Sakaki Y, Fujiyama A, Hattori M, Yada T, Toyoda A, Itoh T, Kawagoe C, Watanabe H, Totoki Y, Taylor T, Weissenbach J, Heilig R, Saurin W, Artiguenave F, Brottier P, Bruls T, Pelletier E, Robert C, Wincker P, Smith DR, Doucette-Stamm L, Rubenfield M, Weinstock K, Lee HM, Dubois J, Rosenthal A, Platzer M, Nyakatura G, Taudien S, Rump A, Yang H, Yu J, Wang J, Huang G, Gu J, Hood L, Rowen L, Madan A, Qin S, Davis RW, Federspiel NA, Abola AP, Proctor MJ, Myers RM, Schmutz J, Dickson M, Grimwood J, Cox DR, Olson MV, Kaul R, Raymond C, Shimizu N, Kawasaki K, Minoshima S, Evans GA, Athanasiou M, Schultz R, Roe BA, Chen F, Pan H, Ramser J, Lehrach H, Reinhardt R, McCombie WR, de la Bastide M, Dedhia N, Blocker H, Hornischer K, Nordsiek G, Agarwala R, Aravind L, Bailey JA, Bateman A, Batzoglou S, Birney E, Bork P, Brown DG, Burge CB, Cerutti L, Chen HC, Church D, Clamp M, Copley RR, Doerks T, Eddy SR, Eichler EE, Furey TS, Galagan J, Gilbert JG, Harmon C, Hayashizaki Y, Haussler D, Hermjakob H, Hokamp K, Jang W, Johnson LS, Jones TA, Kasif S, Kaspryzk A, Kennedy S, Kent WJ, Kitts P, Koonin EV, Korf I, Kulp D, Lancet D, Lowe TM, McLysaght A, Mikkelsen T, Moran JV, Mulder N, Pollara VJ, Ponting CP, Schuler G, Schultz J, Slater G, Smit AF, Stupka E, Szustakowski J, Thierry-Mieg D, Thierry-Mieg J, Wagner L, Wallis J, Wheeler R, Williams A, Wolf YI, Wolfe KH, Yang SP, Yeh RF, Collins F, Guyer MS, Peterson J, Felsenfeld A, Wetterstrand KA, Patrinos A, Morgan MJ, de Jong P, Catanese JJ, Osoegawa K, Shizuya H, Choi S, Chen YJ; International Human Genome Sequencing Consortium. Initial sequencing and analysis of the human genome. Nature 2001;409:860-921.
2. Bertone P, Stolc V, Royce TE, Rozowsky JS, Urban AE, Zhu X, Rinn JL, Tongprasit W, Samanta M, Weissman S, Gerstein M, Snyder M. Global identification of human transcribed sequences with genome tiling arrays. Science 2004;306:2242-6.
3. Mattick JS. Challenging the dogma: the hidden layer of non-protein-coding RNAs in complex organisms. Bioessays 2003;25:930-9.
4. Ponting CP, Oliver PL, Reik W. Evolution and functions of long noncoding RNAs. Cell 2009;136:629-41.
6. Carninci P, Kasukawa T, Katayama S, Gough J, Frith MC, Maeda N, Oyama R, Ravasi T, Lenhard B, Wells C, Kodzius R, Shimokawa K, Bajic VB, Brenner SE, Batalov S, Forrest AR, Zavolan M, Davis MJ, Wilming LG, Aidinis V, Allen JE, Ambesi-Impiombato A, Apweiler R, Aturaliya RN, Bailey TL, Bansal M, Baxter L, Beisel KW, Bersano T, Bono H, Chalk AM, Chiu KP, Choudhary V, Christoffels A, Clutterbuck DR, Crowe ML, Dalla E, Dalrymple BP, de Bono B, Della Gatta G, di Bernardo D, Down T, Engstrom P, Fagiolini M, Faulkner G, Fletcher CF, Fukushima T, Furuno M, Futaki S, Gariboldi M, Georgii-Hemming P, Gingeras TR, Gojobori T, Green RE, Gustincich S, Harbers M, Hayashi Y, Hensch TK, Hirokawa N, Hill D, Huminiecki L, Iacono M, Ikeo K, Iwama A, Ishikawa T, Jakt M, Kanapin A, Katoh M, Kawasawa Y, Kelso J, Kitamura H, Kitano H, Kollias G, Krishnan SP, Kruger A, Kummerfeld SK, Kurochkin IV, Lareau LF, Lazarevic D, Lipovich L, Liu J, Liuni S, McWilliam S, Madan Babu M, Madera M, Marchionni L, Matsuda H, Matsuzawa S, Miki H, Mignone F, Miyake S, Morris K, Mottagui-Tabar S, Mulder N, Nakano N, Nakauchi H, Ng P, Nilsson R, Nishiguchi S, Nishikawa S, Nori F, Ohara O, Okazaki Y, Orlando V, Pang KC, Pavan WJ, Pavesi G, Pesole G, Petrovsky N, Piazza S, Reed J, Reid JF, Ring BZ, Ringwald M, Rost B, Ruan Y, Salzberg SL, Sandelin A, Schneider C, Schönbach C, Sekiguchi K, Semple CA, Seno S, Sessa L, Sheng Y, Shibata Y, Shimada H, Shimada K, Silva D, Sinclair B, Sperling S, Stupka E, Sugiura K, Sultana R, Takenaka Y, Taki K, Tammoja K, Tan SL, Tang S, Taylor MS, Tegner J, Teichmann SA, Ueda HR, van Nimwegen E, Verardo R, Wei CL, Yagi K, Yamanishi H, Zabarovsky E, Zhu S, Zimmer A, Hide W, Bult C, Grimmond SM, Teasdale RD, Liu ET, Brusic V, Quackenbush J, Wahlestedt C, Mattick JS, Hume DA, Kai C, Sasaki D, Tomaru Y, Fukuda S, Kanamori-Katayama M, Suzuki M, Aoki J, Arakawa T, Iida J, Imamura K, Itoh M, Kato T, Kawaji H, Kawagashira N, Kawashima T, Kojima M, Kondo S, Konno H, Nakano K, Ninomiya N, Nishio T, Okada M, Plessy C, Shibata K, Shiraki T, Suzuki S, Tagami M, Waki K, Watahiki A, Okamura-Oho Y, Suzuki H, Kawai J, Hayashizaki Y; FANTOM Consortium, RIKEN Genome Exploration Research Group and Genome Science Group (Genome Network Project Core Group). The transcriptional landscape of the mammalian genome. Science 2005;309:1559-63.
7. Harrow J, Frankish A, Gonzalez JM, Tapanari E, Diekhans M, Kokocinski F, Aken BL, Barrell D, Zadissa A, Searle S, Barnes I, Bignell A, Boychenko V, Hunt T, Kay M, Mukherjee G, Rajan J, Despacio-Reyes G, Saunders G, Steward C, Harte R, Lin M, Howald C, Tanzer A, Derrien T, Chrast J, Walters N, Balasubramanian S, Pei B, Tress M, Rodriguez JM, Ezkurdia I, van Baren J, Brent M, Haussler D, Kellis M, Valencia A, Reymond A, Gerstein M, Guigo R, Hubbard TJ. GENCODE: the reference human genome annotation for the ENCODE project. Genome Res 2012;22:1760-74.
8. Sparmann A, van Lohuizen M. Polycomb silencers control cell fate, development and cancer. Nat Rev Cancer 2006;6:846-56.
9. Pasmant E, Laurendeau I, Heron D, Vidaud M, Vidaud D, Bieche I. Characterization of a germ-line deletion, including the entire INK4/ARF locus, in a melanoma-neural system tumor family: identification of ANRIL, an antisense noncoding RNA whose expression coclusters with ARF. Cancer Res 2007;67:3963-9.
10. Yap KL, Li S, Munoz-Cabello AM, Raguz S, Zeng L, Mujtaba S, Gil J, Walsh MJ, Zhou MM. Molecular interplay of the noncoding RNA ANRIL and methylated histone H3 lysine 27 by polycomb CBX7 in transcriptional silencing of INK4a. Mol Cell 2010;38:662-74.
11. Plath K, Fang J, Mlynarczyk-Evans SK, Cao R, Worringer KA, Wang H, de la Cruz CC, Otte AP, Panning B, Zhang Y. Role of histone H3 lysine 27 methylation in X inactivation. Science 2003;300:131-5.
12. Kim K, Choi J, Heo K, Kim H, Levens D, Kohno K, Johnson EM, Brock HW, An W. Isolation and characterization of a novel H1.2 complex that acts as a repressor of p53-mediated transcription. J Biol Chem 2008;283:9113-26.
13. Yoon JH, Abdelmohsen K, Srikantan S, Yang X, Martindale JL, De S, Huarte M, Zhan M, Becker KG, Gorospe M. LincRNA-p21 suppresses target mRNA translation. Mol Cell 2012;47:648-55.
14. Derrien T, Johnson R, Bussotti G, Tanzer A, Djebali S, Tilgner H, Guernec G, Martin D, Merkel A, Knowles DG, Lagarde J, Veeravalli L, Ruan X, Ruan Y, Lassmann T, Carninci P, Brown JB, Lipovich L, Gonzalez JM, Thomas M, Davis CA, Shiekhattar R, Gingeras TR, Hubbard TJ, Notredame C, Harrow J, Guigo R. The GENCODE v7 catalog of human long noncoding RNAs: analysis of their gene structure, evolution, and expression. Genome Res 2012;22:1775-89.
15. Rodriguez A, Griffiths-Jones S, Ashurst JL, Bradley A. Identification of mammalian microRNA host genes and transcription units. Genome Res 2004;14:1902-10.
16. Cai X, Cullen BR. The imprinted H19 noncoding RNA is a primary microRNA precursor. RNA 2007;13:313-6.
17. Liu Q, Huang J, Zhou N, Zhang Z, Zhang A, Lu Z, Wu F, Mo YY. LncRNA loc285194 is a p53-regulated tumor suppressor. Nucleic Acids Res 2013;41:4976-87.
18. Zhang Z, Zhu Z, Watabe K, Zhang X, Bai C, Xu M, Wu F, Mo YY. Negative regulation of lncRNA GAS5 by miR-21. Cell Death Differ 2013;20:1558-68.
19. Poliseno L, Salmena L, Zhang J, Carver B, Haveman WJ, Pandolfi PP. A coding-independent function of gene and pseudogene mRNAs regulates tumour biology. Nature 2010;465:1033-8.
20. Schneider C, King RM, Philipson L. Genes specifically expressed at growth arrest of mammalian cells. Cell 1988;54:787-93.
21. Kino T, Hurt DE, Ichijo T, Nader N, Chrousos GP. Noncoding RNA gas5 is a growth arrest- and starvation-associated repressor of the glucocorticoid receptor. Sci Signal 2010;3:ra8.
22. Good MC, Zalatan JG, Lim WA. Scaffold proteins: hubs for controlling the flow of cellular information. Science 2011;332:680-6.
23. Loewer S, Cabili MN, Guttman M, Loh YH, Thomas K, Park IH, Garber M, Curran M, Onder T, Agarwal S, Manos PD, Datta S, Lander ES, Schlaeger TM, Daley GQ, Rinn JL. Large intergenic non-coding RNA-RoR modulates reprogramming of human induced pluripotent stem cells. Nat Genet 2010;42:1113-7.
24. Zhang A, Zhou N, Huang J, Liu Q, Fukuda K, Ma D, Lu Z, Bai C, Watabe K, Mo YY. The human long non-coding RNA-RoR is a p53 repressor in response to DNA damage. Cell Res 2013;23:340-50.
25. Fox AH, Lam YW, Leung AK, Lyon CE, Andersen J, Mann M, Lamond AI. Paraspeckles: a novel nuclear domain. Curr Biol 2002;12:13-25.
26. Emili A, Shales M, McCracken S, Xie W, Tucker PW, Kobayashi R, Blencowe BJ, Ingles CJ. Splicing and transcription-associated proteins PSF and p54nrb/nonO bind to the RNA polymerase II CTD. RNA 2002;8:1102-11.
27. Auboeuf D, Dowhan DH, Li X, Larkin K, Ko L, Berget SM, O'Malley BW. CoAA, a nuclear receptor coactivator protein at the interface of transcriptional coactivation and RNA splicing. Mol Cell Biol 2004;24:442-53.
28. Fox AH, Bond CS, Lamond AI. P54nrb forms a heterodimer with PSP1 that localizes to paraspeckles in an RNA-dependent manner. Mol Biol Cell 2005;16:5304-15.
29. Clemson CM, Hutchinson JN, Sara SA, Ensminger AW, Fox AH, Chess A, Lawrence JB. An architectural role for a nuclear noncoding RNA: NEAT1 RNA is essential for the structure of paraspeckles. Mol Cell 2009;33:717-26.
30. Morin GB. The human telomere terminal transferase enzyme is a ribonucleoprotein that synthesizes TTAGGG repeats. Cell 1989;59:521-9.
31. Greider CW, Blackburn EH. A telomeric sequence in the RNA of tetrahymena telomerase required for telomere repeat synthesis. Nature 1989;337:331-7.
32. Ly H, Blackburn EH, Parslow TG. Comprehensive structure-function analysis of the core domain of human telomerase RNA. Mol Cell Biol 2003;23:6849-56.
33. Vulliamy T, Marrone A, Dokal I, Mason PJ. Association between aplastic anaemia and mutations in telomerase RNA. Lancet 2002;359:2168-70.
35. Oka H, Shiozaki H, Kobayashi K, Inoue M, Tahara H, Kobayashi T, Takatsuka Y, Matsuyoshi N, Hirano S, Takeichi M, Mori T. Expression of E-cadherin cell adhesion molecules in human breast cancer tissues and its relationship to metastasis. Cancer Res 1993;53:1696-701.
36. Schipper JH, Frixen UH, Behrens J, Unger A, Jahnke K, Birchmeier W. E-cadherin expression in squamous cell carcinomas of head and neck: inverse correlation with tumor dedifferentiation and lymph node metastasis. Cancer Res 1991;51:6328-37.
38. Cheng GZ, Chan J, Wang Q, Zhang W, Sun CD, Wang LH. Twist transcriptionally up-regulates AKT2 in breast cancer cells leading to increased migration, invasion, and resistance to paclitaxel. Cancer Res 2007;67:1979-87.
39. Chambers AF, Groom AC, MacDonald IC. Dissemination and growth of cancer cells in metastatic sites. Nat Rev Cancer 2002;2:563-72.
41. Wang H, Fu W, Im JH, Zhou Z, Santoro SA, Iyer V, DiPersio CM, Yu QC, Quaranta V, Al-Mehdi A, Muschel RJ. Tumor cell alpha3beta1 integrin and vascular laminin-5 mediate pulmonary arrest and metastasis. J Cell Biol 2004;164:935-41.
43. Aguirre-Ghiso JA. Models, mechanisms and clinical evidence for cancer dormancy. Nat Rev Cancer 2007;7:834-46.
44. Ji P, Diederichs S, Wang W, Boing S, Metzger R, Schneider PM, Tidow N, Brandt B, Buerger H, Bulk E, Thomas M, Berdel WE, Serve H, Muller-Tidow C. MALAT-1, a novel noncoding RNA, and thymosin beta4 predict metastasis and survival in early-stage non-small cell lung cancer. Oncogene 2003;22:8031-41.
45. Tripathi V, Ellis JD, Shen Z, Song DY, Pan Q, Watt AT, Freier SM, Bennett CF, Sharma A, Bubulya PA, Blencowe BJ, Prasanth SG, Prasanth KV. The nuclear-retained noncoding RNA MALAT1 regulates alternative splicing by modulating SR splicing factor phosphorylation. Mol Cell 2010;39:925-38.
46. Gutschner T, Hammerle M, Eissmann M, Hsu J, Kim Y, Hung G, Revenko A, Arun G, Stentrup M, Gross M, Zornig M, MacLeod AR, Spector DL, Diederichs S. The noncoding RNA MALAT1 is a critical regulator of the metastasis phenotype of lung cancer cells. Cancer Res 2013;73:1180-9.
47. Tano K, Mizuno R, Okada T, Rakwal R, Shibato J, Masuo Y, Ijiri K, Akimitsu N. MALAT-1 enhances cell motility of lung adenocarcinoma cells by influencing the expression of motility-related genes. FEBS Lett 2010;584:4575-80.
48. Jiang Y, Li Y, Fang S, Jiang B, Qin C, Xie P, Zhou G, Li G. The role of MALAT1 correlates with HPV in cervical cancer. Oncol Lett 2014;7:2135-41.
49. Jiao F, Hu H, Han T, Yuan C, Wang L, Jin Z, Guo Z, Wang L. Long noncoding RNA MALAT-1 enhances stem cell-like phenotypes in pancreatic cancer cells. Int J Mol Sci 2015;16:6677-93.
50. Gupta RA, Shah N, Wang KC, Kim J, Horlings HM, Wong DJ, Tsai MC, Hung T, Argani P, Rinn JL, Wang Y, Brzoska P, Kong B, Li R, West RB, van de Vijver MJ, Sukumar S, Chang HY. Long non-coding RNA HOTAIR reprograms chromatin state to promote cancer metastasis. Nature 2010;464:1071-6.
51. Tsai MC, Manor O, Wan Y, Mosammaparast N, Wang JK, Lan F, Shi Y, Segal E, Chang HY. Long noncoding RNA as modular scaffold of histone modification complexes. Science 2010;329:689-93.
52. Wu Y, Zhang L, Zhang L, Wang Y, Li H, Ren X, Wei F, Yu W, Liu T, Wang X, Zhou X, Yu J, Hao X. Long non-coding RNA HOTAIR promotes tumor cell invasion and metastasis by recruiting EZH2 and repressing E-cadherin in oral squamous cell carcinoma. Int J Oncol 2015;46:2586-94.
53. Niinuma T, Suzuki H, Nojima M, Nosho K, Yamamoto H, Takamaru H, Yamamoto E, Maruyama R, Nobuoka T, Miyazaki Y, Nishida T, Bamba T, Kanda T, Ajioka Y, Taguchi T, Okahara S, Takahashi H, Nishida Y, Hosokawa M, Hasegawa T, Tokino T, Hirata K, Imai K, Toyota M, Shinomura Y. Upregulation of miR-196a and HOTAIR drive malignant character in gastrointestinal stromal tumors. Cancer Res 2012;72:1126-36.
54. Pachnis V, Belayew A, Tilghman SM. Locus unlinked to alpha-fetoprotein under the control of the murine raf and rif genes. Proc Natl Acad Sci U S A 1984;81:5523-7.
55. Brannan CI, Dees EC, Ingram RS, Tilghman SM. The product of the H19 gene may function as an RNA. Mol Cell Biol 1990;10:28-36.
56. Hao Y, Crenshaw T, Moulton T, Newcomb E, Tycko B. Tumour-suppressor activity of H19 RNA. Nature 1993;365:764-7.
57. Hibi K, Nakamura H, Hirai A, Fujikake Y, Kasai Y, Akiyama S, Ito K, Takagi H. Loss of H19 imprinting in esophageal cancer. Cancer Res 1996;56:480-2.
58. Ariel I, Miao HQ, Ji XR, Schneider T, Roll D, de Groot N, Hochberg A, Ayesh S. Imprinted H19 oncofetal RNA is a candidate tumour marker for hepatocellular carcinoma. Mol Pathol 1998;51:21-5.
59. Ayesh S, Matouk I, Schneider T, Ohana P, Laster M, Al-Sharef W, De-Groot N, Hochberg A. Possible physiological role of H19 RNA. Mol Carcinog 2002;35:63-74.
60. Luo M, Li Z, Wang W, Zeng Y, Liu Z, Qiu J. Long non-coding RNA H19 increases bladder cancer metastasis by associating with EZH2 and inhibiting E-cadherin expression. Cancer Lett 2013;333:213-21.
61. Adriaenssens E, Lottin S, Berteaux N, Hornez L, Fauquette W, Fafeur V, Peyrat JP, Le Bourhis X, Hondermarck H, Coll J, Dugimont T, Curgy JJ. Cross-talk between mesenchyme and epithelium increases H19 gene expression during scattering and morphogenesis of epithelial cells. Exp Cell Res 2002;275:215-29.
62. Liang WC, Fu WM, Wong CW, Wang Y, Wang WM, Hu GX, Zhang L, Xiao LJ, Wan DC, Zhang JF, Waye MM. The lncRNA H19 promotes epithelial to mesenchymal transition by functioning as miRNA sponges in colorectal cancer. Oncotarget 2015;6:22513-25.
63. Liu B, Sun L, Liu Q, Gong C, Yao Y, Lv X, Lin L, Yao H, Su F, Li D, Zeng M, Song E. A cytoplasmic NF-kappaB interacting long noncoding RNA blocks IkappaB phosphorylation and suppresses breast cancer metastasis. Cancer Cell 2015;27:370-81.
64. Chaturvedi MM, Sung B, Yadav VR, Kannappan R, Aggarwal BB. NF-kappaB addiction and its role in cancer: 'one size does not fit all'. Oncogene 2011;30:1615-30.
65. Ghosh S, Karin M. Missing pieces in the NF-kappaB puzzle. Cell 2002;109 Suppl:S81-96.
66. Thiery JP, Acloque H, Huang RY, Nieto MA. Epithelial-mesenchymal transitions in development and disease. Cell 2009;139:871-90.
67. Akhurst RJ, Hata A. Targeting the TGFbeta signalling pathway in disease. Nat Rev Drug Discov 2012;11:790-811.
68. Pardali K, Moustakas A. Actions of TGF-beta as tumor suppressor and pro-metastatic factor in human cancer. Biochim Biophys Acta 2007;1775:21-62.
69. Yuan JH, Yang F, Wang F, Ma JZ, Guo YJ, Tao QF, Liu F, Pan W, Wang TT, Zhou CC, Wang SB, Wang YZ, Yang Y, Yang N, Zhou WP, Yang GS, Sun SH. A long noncoding RNA activated by TGF-beta promotes the invasion-metastasis cascade in hepatocellular carcinoma. Cancer Cell 2014;25:666-81.
70. Burk U, Schubert J, Wellner U, Schmalhofer O, Vincan E, Spaderna S, Brabletz T. A reciprocal repression between ZEB1 and members of the miR-200 family promotes EMT and invasion in cancer cells. EMBO Rep 2008;9:582-9.
71. Yang F, Zhang L, Huo XS, Yuan JH, Xu D, Yuan SX, Zhu N, Zhou WP, Yang GS, Wang YZ, Shang JL, Gao CF, Zhang FR, Wang F, Sun SH. Long noncoding RNA high expression in hepatocellular carcinoma facilitates tumor growth through enhancer of zeste homolog 2 in humans. Hepatology 2011;54:1679-89.
72. Yang F, Huo XS, Yuan SX, Zhang L, Zhou WP, Wang F, Sun SH. Repression of the long noncoding RNA-LET by histone deacetylase 3 contributes to hypoxia-mediated metastasis. Mol Cell 2013;49:1083-96.
73. Peinado H, Olmeda D, Cano A. Snail, Zeb and bHLH factors in tumour progression: an alliance against the epithelial phenotype? Nat Rev Cancer 2007;7:415-28.
74. Nissan A, Stojadinovic A, Mitrani-Rosenbaum S, Halle D, Grinbaum R, Roistacher M, Bochem A, Dayanc BE, Ritter G, Gomceli I, Bostanci EB, Akoglu M, Chen YT, Old LJ, Gure AO. Colon cancer associated transcript-1: a novel RNA expressed in malignant and pre-malignant human tissues. Int J Cancer 2012;130:1598-606.
75. Yang F, Xue X, Bi J, Zheng L, Zhi K, Gu Y, Fang G. Long noncoding RNA CCAT1, which could be activated by c-Myc, promotes the progression of gastric carcinoma. J Cancer Res Clin Oncol 2013;139:437-45.
76. Deng L, Yang SB, Xu FF, Zhang JH. Long noncoding RNA CCAT1 promotes hepatocellular carcinoma progression by functioning as let-7 sponge. J Exp Clin Cancer Res 2015;34:18.
77. Prensner JR, Chinnaiyan AM. The emergence of lncRNAs in cancer biology. Cancer Discov 2011;1:391-407.
78. Winkle M, van den Berg A, Tayari M, Sietzema J, Terpstra M, Kortman G, de Jong D, Visser L, Diepstra A, Kok K, Kluiver J. Long noncoding RNAs as a novel component of the Myc transcriptional network. FASEB J 2015;29:2338-46.
79. Jinek M, Chylinski K, Fonfara I, Hauer M, Doudna JA, Charpentier E. A programmable dual-RNA-guided DNA endonuclease in adaptive bacterial immunity. Science 2012;337:816-21.
80. Sanchez-Rivera FJ, Jacks T. Applications of the CRISPR-Cas9 system in cancer biology. Nat Rev Cancer 2015;15:387-95.
81. Ho TT, Zhou N, Huang J, Koirala P, Xu M, Fung R, Wu F, Mo YY. Targeting non-coding RNAs with the CRISPR/Cas9 system in human cell lines. Nucleic Acids Res 2015;43:e17.
Cite This Article
Export citation file: BibTeX | EndNote | RIS
OAE Style
Koirala P, Zou DH, Mo YY. Long non-coding RNAs as key regulators of cancer metastasis. J Cancer Metastasis Treat 2016;2:1-10. http://dx.doi.org/10.4103/2394-4722.171829
AMA Style
Koirala P, Zou DH, Mo YY. Long non-coding RNAs as key regulators of cancer metastasis. Journal of Cancer Metastasis and Treatment. 2016; 2: 1-10. http://dx.doi.org/10.4103/2394-4722.171829
Chicago/Turabian Style
Pratirodh Koirala, De-Hong Zou, Yin-Yuan Mo. 2016. "Long non-coding RNAs as key regulators of cancer metastasis" Journal of Cancer Metastasis and Treatment. 2: 1-10. http://dx.doi.org/10.4103/2394-4722.171829
ACS Style
Koirala, P.; Zou D.H.; Mo Y.Y. Long non-coding RNAs as key regulators of cancer metastasis. J. Cancer. Metastasis. Treat. 2016, 2, 1-10. http://dx.doi.org/10.4103/2394-4722.171829
About This Article
Copyright
Author Biographies
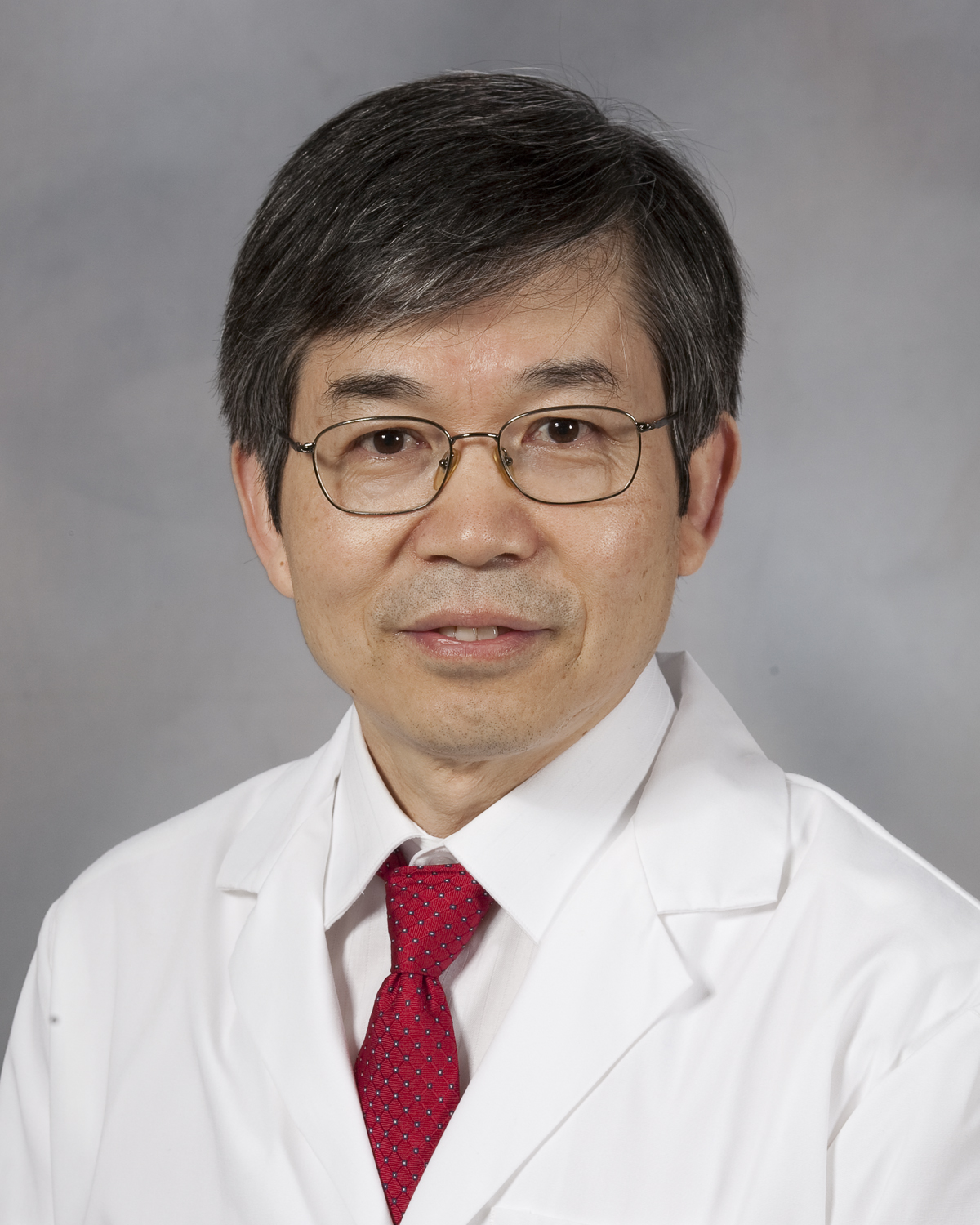
Comments
Comments must be written in English. Spam, offensive content, impersonation, and private information will not be permitted. If any comment is reported and identified as inappropriate content by OAE staff, the comment will be removed without notice. If you have any queries or need any help, please contact us at support@oaepublish.com.