The relationship between hyperuricemia and hypertension: a short review of current evidence
Abstract
The investigation of the relationship between hyperuricemia and hypertension is a captivating field in hypertension research. The final product of purine metabolism is uric acid (UA), and the elevation of serum UA (SUA) levels directly contributes to the development of hypertension. Numerous studies have substantiated that hyperuricemia is a significant risk factor for hypertension. Furthermore, initial clinical trials have demonstrated that therapeutic interventions aimed at reducing SUA levels lower blood pressure (BP) in individuals with hypertension attributed to hyperuricemia. Recent research has demonstrated that hyperuricemia can facilitate the onset of hypertension via multiple mechanisms, including oxidative stress, inflammation, diminished nitric oxide (NO) synthesis, activation of reactive oxygen species (ROS), insulin resistance, vascular smooth muscle proliferation, and renal impairment. Given the interconnectedness between hyperuricemia and hypertension, it is advantageous to identify potential therapeutic approaches for timely intervention in order to impede the advancement of hypertension in individuals with hyperuricemia. This article reviews the research progress on the pathogenesis of hyperuricemia-induced hypertension.
Keywords
INTRODUCTION
Both hyperuricemia and hypertension are common metabolic diseases, and the harm to people’s quality of life can not be ignored. The definition of hyperuricemia typically involves a SUA level exceeding 7.0 mg/dL in men or 6.0 mg/dL in women[1]. The final product of purine metabolism is SUA, which is primarily excreted by the kidneys[2]. The elevation of UA levels is attributed to either an increase in its production or a decrease in its excretion[3]. Hypertension is a chronic disease characterized by elevated arterial BP. The definition of hypertension is an arterial blood pressure BP ≥ 140/90 mmHg[4]. The hypothesis of the association between UA and hypertension was initially proposed by Mahomed et al. in the 1870s[5]. The past few years have witnessed an increasing number of studies confirming hyperuricemia as a prominent risk factor in the development of hypertension[6,7]. A meta-analysis of prospective cohort studies that assessed the relationship between hyperuricemia and hypertension, including 18 studies and 55,607 participants with normal BP at the time of presentation, demonstrated that hyperuricemia was independently associated with an elevated risk of developing hypertension, irrespective of conventional risk factors for hypertension, particularly among the younger population and females, for a 1 mg/dL increase in UA level, the RR of combined hypertension events after adjusting for potential confounders was 1.13 (95%CI: 1.06-1.20)[8]. Another large-scale Japanese cross-sectional study showed that hyperuricemia was significantly associated with hypertension. After adjusting for age, BMI, blood lipids, diabetes, alcohol consumption, smoking, and eGFR (mL/min/1.73m2), SUA ≥ 5.3 mg/dL in men and ≥ 4.3 mg/dL in women are still positively correlated with the prevalence of hypertension[9]. It is worth noting that this relationship is particularly evident in children and adolescents. A recent study showed that the average SUA level of hypertensive children was higher than that of non-hypertensive children, and the effect of hyperuricemia on the systolic BP of hypertensive children was more significant[10]. The addition of UA-lowering therapy has been demonstrated to effectively reduce BP in hypertensive patients with hyperuricemia[11]. For example, allopurinol significantly reduces systolic and diastolic BP in children and adolescents with newly diagnosed essential hypertension[12].
Hypertension caused by hyperuricemia can be divided into two stages[13]: In the first stage, hyperuricemia inhibits NO production and activates the RAS, leading to excessive vasoconstriction, leading to hypertension. This process is reversible and is a UA-dependent response by which BP can be lowered. In the second stage, when hyperuricemia persists, the vascular wall is thickened, and BP increases due to the proliferation of vascular smooth muscle cells due to structural changes in the blood vessels. This phase was not associated with UA, and BP was no longer restored to its original level by lowering UA as in the first phase. Overall, UA increases BP through oxidative stress, inflammation, reduced NO production, RAS activation, insulin resistance, vascular smooth muscle proliferation, and kidney injury [Figure 1]. In this review, we will discuss the primary mechanisms of hyperuricemia leading to the development of hypertension.
RESEARCH PROGRESS ON THE MECHANISM OF HYPERURICEMIA-INDUCED HYPERTENSION
Oxidative stress
Oxidative stress is a state of imbalance between oxidation and antioxidation in the body. Reactive oxygen species (ROS), derived from oxygen molecules (O2), is an unstable and strong oxidant that can oxidize intracellular proteins, lipids, and DNA, leading to cell damage. UA has two contradictory properties: antioxidant and pro-oxidant. UA outside the cell is an antioxidant, while UA inside the cell is a pro-oxidant[14]. It has been shown that physiological concentrations of UA can inhibit ROS production in endothelial cells. In contrast, high concentrations of UA increase ROS production, mainly through the action of the mitochondrial respiratory chain[15,16]. There are three main mechanisms of increased oxidative stress caused by hyperuricemia[17]: (1) Increased xanthine oxidase activity during UA metabolism and production of ROS; (2) Increased expression and activity of NADPH oxidase also produced ROS; (3) Mitochondrial ROS (mtROS) is produced by mitochondrial damage.
UA produced by intracellular xanthine oxidase induces oxidative stress bursts mediated by mitogen-activated protein kinases (MAPK, such as p38MAPK) and NADPH oxidase, thus becoming the cellular mechanism driving the hypertensive response[18]. Animal model studies have demonstrated a positive correlation between elevated SUA levels and increased BP, while the administration of febuxostat, a xanthine oxidase inhibitor, effectively ameliorates both systemic and glomerular hypertension[19]. In hyperuricemia, NADPH oxidase catalyzes the generation of superoxide anions (such as ROS) through the transmembrane transfer of one electron to molecular oxygen, which induces endothelial dysfunction and subsequently increases BP[20]. UA causes the increase of mtROS and mitochondrial damage, which leads to mitochondrial dysfunction, further induces senescence and apoptosis of vascular endothelial cells, leading to the occurrence of atherosclerosis, and then leads to the occurrence and development of hypertension[17]. Kimura et al. found that UA-induced mitochondrial damage through the AMP-activated protein kinase pathway[21].
In summary, on the one hand, ROS generated during UA generation binds to vasodilator substance NO. It inhibits the function of NO, which in turn causes vasoconstriction and promotes the increase of BP[22]. On the other hand, oxidative stress leads to atherosclerosis by inducing inflammatory responses of inflammatory cells (such as macrophages), platelet aggregation, and LDL oxidation, which in turn causes BP elevation[17].
Inflammatory response
Inflammation is also involved in the association between hyperuricemia and hypertension. Ruggiero et al. found that UA was significantly positively correlated with a variety of inflammatory markers, such as C-reactive protein (CRP) and interleukin-6 (IL-6), indicating that UA may contribute to promoting the inflammatory state[23]. UA promotes inflammation mainly through three inflammatory signaling pathways in cells: (1) Activation of the mitogen-activated protein kinase (MAPK) pathway: Studies have shown that UA exerts proinflammatory effects through the activation of p38 MAPK and p44/42 MAPK, which is time - and concentration-dependent[24]. UA also activates extracellular signal-regulated kinase (ERK), which is involved in the secretion of inflammatory factors, adhesion factors, chemokines, and cell proliferation and migration[25]; (2) Inhibition of adenosine monophosphate-activated protein kinase (AMPK) pathway: AMPK and NLRP3 inflammasome are associated with the disturbance of metabolic and inflammatory pathways. Studies have shown that UA can inhibit the AMPK pathway and regulate the activation of NLRP3 inflammasome, thereby causing inflammation[26]; (3) Activation of phosphatidylinositol-3-kinase (PI3K)-Akt pathway: UA phosphorylates Akt and activates mTOR, which inhibits autophagy and promotes inflammation and angiogenesis through hypoxia-inducible factor (HIF)-1α[27,28].
In endothelial cells, UA stimulates the synthesis of proinflammatory factors such as CRP, IL-6, interleukin-8 (IL-8), angiotensin-II (AngII), tumor necrosis factor-α, intercellular adhesion molecule-1 (ICAM-1), vascular cell adhesion molecule-1 (VCAM-1), and monocyte chemoattractant protein-1 (MCP-1)[29]. In vascular smooth muscle cells, UA stimulates cell proliferation and migration by producing CRP, cyclooxygenase-2, MCP-1, local thromboxane, and AngII and upregulates platelet-derived growth factor-A[30]. Increased expression and release of MCP-1, IL-8, VCAM-1, and ICAM-1 can trigger monocyte migration and attachment to the vascular wall, cause an inflammatory reaction, damage vascular endothelial cells, and lead to vascular endothelial dysfunction[29].
In addition, when the SUA level exceeds 7.0 mg/dL, UA that cannot be dissolved in blood vessels will precipitate to form sodium urate crystals, which can activate multinucleated leukocytes, monocytes, and lymphocytes and produce a variety of inflammatory substances[22]. Notably, the interaction of macrophage through phagocytoses sodium urate crystals with pathogen recognition receptors, Toll-like receptors (TLR) 2/4 and cd14 may trigger the MyD88/TRIF pathway, leading to nuclear factor-κb (NF-κB) activation and NLRP3 inflammasome protein complex formation, which results in the activation of caspase-1 and the processing and secretion of IL-1β[31]. In addition, some studies have found that soluble UA can also promote the expression of NALP3 inflammasome and IL-1β in proximal renal tubular endothelial cells, thereby promoting immune inflammatory response[32].
Inflammatory mediators promote the development of hypertension by increasing vascular permeability and releasing cytokines and ROS. In addition, the release of cytokines leads to the reduction of the luminal diameter of resistance vessels, the increase of vascular resistance and stiffness, the production of angiotensinogen and AngII, and the retention of water and sodium, thereby increasing BP[33].
Inhibition of NO production
NO is a highly reactive and gas-diffusing free radical with potent vasodilator, anti-inflammatory, and antioxidant properties and plays a crucial role in regulating vascular tone, angiogenesis, inflammatory cell adhesion, and platelet aggregation[34]. In addition, NO is an unstable molecule that is easily oxidized by ROS and thus loses its physiological activity[17].
Hyperuricemia mainly causes a decrease in NO production through three mechanisms: increased oxidative stress, decreased L-arginine supply, and endothelial nitric oxide synthase (eNOS) inhibition, leading to endothelial dysfunction and, thus, increased BP[35].
L-arginine is one of the raw materials for NO production. L-arginine can also be cleaved by arginase in the urea cycle (also known as the ornithine cycle) to form urea and L-ornithine[36]. However, hyperuricemia increases arginase activity in human umbilical vein endothelial cells and pulmonary artery endothelial cells, leading to a decrease in the supply of L-arginine. Consequently, NO production is reduced[37,38]. In addition, NO synthesis in endothelial cells is catalyzed by eNOS in endothelial cells[39]. Park et al. found that high UA levels impair eNOS activity by inhibiting the interaction between eNOS and CaM, which in turn leads to reduced NO production[40].
Hyperuricemia can directly act on the renal macula densus to reduce the expression of NO synthase or trigger endoplasmic reticulum stress response, mediated by the protein kinase C/endothelial NO synthase pathway, to inhibit eNOS activity, thereby inhibiting NO production and leading to vascular smooth muscle contraction[41]. The reduction of NO production can promote the expression of angiotensin, angiotensin-converting enzyme, AngII and AngII receptor[42]. In summary, hyperuricemia leads to excessive vasoconstriction and BP elevation through both the reduction of NO production and the activation of the RAS system.
Activation of the RAS
AngII is a pivotal effector of the RAS, primarily exerting its actions on specific receptors. These actions encompass vasoconstriction, regulation of acid-base homeostasis, modulation of immune and inflammatory pathways, and stimulation of chemokine release from macrophages[43]. Studies have shown that UA activates RAS, especially the angiotensin-converting enzyme (ACE)-AngII-AngII type 1 receptor pathway, in which AngII constrains blood vessels and increases BP[44]. Related animal experiments have shown that the use of RAS inhibitors (enalapril and losartan) can prevent hypertension and arteriolar lesions caused by hyperuricemia in rats[45]. In addition, studies have shown that UA stimulates vascular smooth muscle cell proliferation by activating RAS organization in vascular smooth muscle cells, and this stimulation is mediated by UA increasing PDGF A and C chain expression, local thromboxane production, and cyclooxygenase-2 stimulation to induce specific MAPK pathways[46]. Notably, UA not only activate the RAS pathway involved in oxidative stress and inflammation but also elicits more complex inflammatory and oxidative responses when combined with AngII[47].
Insulin resistance
Yu et al. showed that elevated UA was closely related to insulin resistance (IR)[48]. In addition, Choi et al. found that SUA levels were linearly and positively correlated with serum C-peptide, insulin levels, and IR[49]. Similarly, Niu et al. recently confirmed that serum UA plays a mediating role in the occurrence of IR in children and adolescents and that UA is an independent risk factor for IR[50]. Notably, UA inhibits insulin-induced eNOS activation and NO production by impairing PI3K/Akt and insulin signaling pathways, leading to the development of IR, which induces endothelial dysfunction and ultimately leads to the development and progression of hypertension[51,52]. Interestingly, peripheral IR may play a more important role than hepatic IR in the development of hyperuricemia-induced hypertension; hyperuricemia and IR can lead to the development of hypertension through three common mechanisms[53]: (1) RAS activation; (2) UA and insulin directly stimulate renal sodium reabsorption and cause water and sodium retention; (3) Endothelial dysfunction and regulation of vascular tone by NO. In addition, an animal experiment showed that UA induced hepatocyte fat accumulation, IR, and insulin signaling disorders through the NLRP3 inflammasome activation pathway, thereby mediating the occurrence and development of non-alcoholic fatty liver disease (NAFLD) and hypertension[54]. Other reports have demonstrated that reducing UA levels with allopurinol can improve IR and systemic inflammation, and also illustrate the relationship between UA and IR[55].
Kidney injury
The underlying mechanism linking UA and hypertension has been explored in animal experiments, and hyperuricemic rats have been shown to develop hypertension due to preglomerular arterial lesions[45,56]. It is worth noting that hypertension caused by short-term hyperuricemia has no apparent renal oxidative stress or mitochondrial dysfunction. However, long-term hyperuricemia can cause renal oxidative stress to promote mitochondrial dysfunction and decrease ATP content, leading to systemic hypertension[57].
In addition, Sánchez-Lozada et al. found that hyperuricemia can damage the autoregulatory response of glomerular afferent arterioles, resulting in luminal occlusion of preglomerular vessels and vessel wall thickening, leading to severe renal hypoperfusion[58]. Then, the signal of ischemia is a strong stimulator that induces renal tubulointerstitial inflammation and fibrosis as well as elevated BP[58]. Other studies have shown that hyperuricemia leads to renal tubular injury and renal fibrosis by activating endothelin-1 and fibroblast proliferation[59], which in turn reduces renal blood flow, leads to endothelial cell dysfunction, further stimulates the proliferation of systemic vascular smooth muscle cells, and finally, nephropathy and vascular changes lead to the occurrence of hypertension[60].
Other mechanisms
The action of urate anion transporter1 and glucose transporter 9 enables UA to permeate vascular smooth muscle cells, activating p38 MAPK, p44/42 MAPK, and PDGFRβ pathways, which subsequentially promote the growth and migration of vascular smooth muscle cells. This leads to vascular remodeling and hypertension[24]. Gut microbiota is implicated in hyperuricemia and hypertension. It has been documented that intestinal flora can enhance UA excretion through three potential mechanisms: inhibition of xanthine oxidase activity, uricase production, and increased purine metabolism[61]. Robles-Vera et al. demonstrated that gut microbiota can mitigate endotoxemia, augment regulatory T cells, and inhibit the onset and progression of endothelial dysfunction and hypertension[62]. Consequently, gut microbiota contributes to the reduction of UA and BP. Gut microbiota-associated therapeutic pathways might emerge as a novel direction for the treatment of hyperuricemia and hypertension.
ASSOCIATION OF HYPERURICEMIA, HYPERTENSION, AND NAFLD
Hyperuricemia is an independent risk factor for hypertension[6,7] and is also associated with NAFLD[63]. A recent meta-analysis showed that elevated SUA levels were positively associated with NAFLD[64]. In addition, NAFLD is also an independent risk factor for hypertension[65]. Epidemiological evidence shows that about 49.5% of patients with hypertension have NAFLD, and the incidence of hypertension in patients with NAFLD is significantly higher than that in the general population[66]. Recently, it has been found that NAFLD is significantly associated with the increase of systolic BP over time[67]. Similar to the mechanism by which UA causes hypertension, UA also participates in the occurrence and development of NAFLD through oxidative stress, inflammation, and insulin resistance[68], while NAFLD can also induce oxidative stress, inflammation, RAS activation, and IR to promote the development of hypertension[66]. At present, the specific mechanism among the three is not fully understood, and further research is needed to confirm.
EFFECT OF UA-LOWERING DRUGS ON BP
Treatment to reduce UA can reduce BP in patients, which also suggests a direct pathophysiological role of UA in the pathogenesis of hypertension. In a randomized controlled clinical trial, 44 patients aged 12 to 19 years with newly diagnosed essential hypertension and SUA levels greater than 55 mg/L (327 μmol/L) were enrolled. After 8 weeks of treatment, the combination of allopurinol and enalapril was found to be more effective in lowering BP than enalapril alone[69]. Beattie et al. studied hypertensive patients over 65 years of age and 6,678 controls treated with allopurinol[70]. Compared with the control group, systolic BP decreased by 2.1 mmHg and diastolic BP by 1.7 mmHg. Allopurinol use was independently associated with reductions in systolic and diastolic BP after adjustment for multiple factors (P < 0.001), suggesting that allopurinol use in older adults may result in a small reduction in BP[70]. A recent systematic review and meta-analysis of randomized controlled trials supported the positive effect of UA-lowering therapy on BP control, especially systolic BP[71]. However, the effect of UA-lowering drugs on BP is not clear, and there is not enough evidence to recommend the effectiveness of UA-lowering therapy in the treatment of hypertension. In the future, a large number of prospective randomized controlled trials are needed for further verification.
STUDY LIMITATIONS, SUMMARY AND PROSPECT
Hyperuricemia plays an inseparable role in the development of hypertension, especially essential hypertension. Hyperuricemia can promote the development of hypertension through various mechanisms such as oxidative stress, inflammatory response, reduction of NO production, RAS activation, IR, vascular smooth muscle proliferation, and kidney injury, and promote target organ damage. However, the complete mechanism of hyperuricemia-induced hypertension needs to be clarified and needs further research[72]. Studying its mechanism is helpful in selecting potential treatment and intervention strategies, intervening in hypertension early and delaying its progression, and reducing the pathological role of serum UA. However, due to the limitations of clinical studies, the existing evidence on the effect of UA-lowering therapy on BP control is still controversial. Future research should focus on identifying the cardiovascular threshold of normal serum UA, the process of UA fluctuation, and reliable UA detection markers so as to more accurately identify the sequence relationship between hypertension and hyperuricemia and then clarify the actual clinical occurrence of hypertension caused by hyperuricemia, so that clinicians can better intervene.
DECLARATIONS
Author’s contribution
Designed the study: Wang Z
Collected and analyzed the data: Zhanghuang C, Wang Z
Drafted the initial manuscript: Zhanghuang C, Wang Z
Revised the article critically: Zhanghuang C, Yan B
Reviewed and edited the article: Zhanghuang C, Yan B, Yao G
Availability of data and materials
Not applicable.
Financial support and sponsorship
The study was supported by: Scientific Research Foundation of Education Department of Yunnan Province (No. 2020J0228, 2023J0295); Kunming Medical University Joint Project of Department of Science and Technology of Yunnan Province (No. 202301AY070001-108); Kunming City Health Science and Technology Talent “1000” training Project [No. 2020-SW (Reserve)-112]; Kunming Health Personnel Training Project Technology Center Construction Project [No. 2020-SW (Tech)-15] and Open Research Fund of Clinical Research Center for Children’s Health and Diseases of Yunnan Province (2022-ETYY-YJ-03). The funding bodies played no role in the study’s design and collection, analysis and interpretation of data, and writing the manuscript.
Conflicts of interest
All authors declared that there are no conflicts of interest.
Ethical approval and consent to participate
Not applicable.
Consent for publication
Not applicable.
Copyright
© The Author(s) 2024.
REFERENCES
1. Zhu Y, Pandya BJ, Choi HK. Prevalence of gout and hyperuricemia in the US general population: the National Health and Nutrition Examination Survey 2007-2008. Arthritis Rheum 2011;63:3136-41.
2. Gaubert M, Bardin T, Cohen-Solal A, et al. Hyperuricemia and hypertension, coronary artery disease, kidney disease: from concept to practice. Int J Mol Sci 2020;21:4066.
3. Ali N, Mahmood S, Islam F, et al. Relationship between serum uric acid and hypertension: a cross-sectional study in Bangladeshi adults. Sci Rep 2019;9:9061.
6. Cao Z, Cheng Y, Li S, et al. Mediation of the effect of serum uric acid on the risk of developing hypertension: a population-based cohort study. J Transl Med 2019;17:202.
7. Feig DI. The role of uric acid in the pathogenesis of hypertension in the young. J Clin Hypertens 2012;14:346-52.
8. Grayson PC, Kim SY, LaValley M, Choi HK. Hyperuricemia and incident hypertension: a systematic review and meta-analysis. Arthritis Care Res 2011;63:102-10.
9. Yokokawa H, Fukuda H, Suzuki A, et al. Association between serum uric acid levels/hyperuricemia and hypertension among 85,286 Japanese workers. J Clin Hypertens (Greenwich) 2016;18:53-9.
10. Fayemi OB, Ajetunmobi WA, Bolaji OB, et al. Relationship between serum uric acid and hypertension among secondary school adolescents in Ekiti State, Nigeria. West Afr J Med 2023;40:997-1002.
11. Feig DI, Nakagawa T, Karumanchi SA, et al. Hypothesis: uric acid, nephron number, and the pathogenesis of essential hypertension. Kidney Int 2004;66:281-7.
12. Feig DI, Soletsky B, Johnson RJ. Effect of allopurinol on blood pressure of adolescents with newly diagnosed essential hypertension: a randomized trial. JAMA 2008;300:924-32.
13. Ubhadiya TJ, Dubey N, Sojitra MH, et al. Exploring the effects of elevated serum uric acid levels on hypertension: a scoping review of hyperuricemia. Cureus 2023;15:e43361.
14. Sautin YY, Johnson RJ. Uric acid: the oxidant-antioxidant paradox. Nucleosides Nucleotides Nucleic Acids 2008;27:608-19.
15. Huang Z, Hong Q, Zhang X, et al. Aldose reductase mediates endothelial cell dysfunction induced by high uric acid concentrations. Cell Commun Signal 2017;15:3.
16. Sánchez-Lozada LG, Lanaspa MA, Cristóbal-García M, et al. Uric acid-induced endothelial dysfunction is associated with mitochondrial alterations and decreased intracellular ATP concentrations. Nephron Exp Nephrol 2012;121:e71-8.
17. Kimura Y, Tsukui D, Kono H. Uric acid in inflammation and the pathogenesis of atherosclerosis. Int J Mol Sci 2021;22:12394.
18. Sanchez-Lozada LG, Rodriguez-Iturbe B, Kelley EE, et al. Uric acid and hypertension: an update with recommendations. Am J Hypertens 2020;33:583-94.
19. Sánchez-Lozada LG, Tapia E, Soto V, et al. Treatment with the xanthine oxidase inhibitor febuxostat lowers uric acid and alleviates systemic and glomerular hypertension in experimental hyperuricaemia. Nephrol Dial Transplant 2008;23:1179-85.
20. Vermot A, Petit-Härtlein I, Smith SME, Fieschi F. NADPH oxidases (NOX): an overview from discovery, molecular mechanisms to physiology and pathology. Antioxidants 2021;10:890.
21. Kimura Y, Yanagida T, Onda A, Tsukui D, Hosoyamada M, Kono H. Soluble uric acid promotes atherosclerosis via AMPK (AMP-activated protein kinase)-mediated inflammation. Arterioscler Thromb Vasc Biol 2020;40:570-82.
23. Ruggiero C, Cherubini A, Ble A, et al. Uric acid and inflammatory markers. Eur Heart J 2006;27:1174-81.
24. Kırça M, Oğuz N, Çetin A, Uzuner F, Yeşilkaya A. Uric acid stimulates proliferative pathways in vascular smooth muscle cells through the activation of p38 MAPK, p44/42 MAPK and PDGFRβ. J Recept Signal Transduct Res 2017;37:167-73.
25. Li Z, Shen Y, Chen Y, Zhang G, Cheng J, Wang W. High uric acid inhibits cardiomyocyte viability through the ERK/P38 pathway via oxidative stress. Cell Physiol Biochem 2018;45:1156-64.
26. Cordero MD, Williams MR, Ryffel B. AMP-activated protein kinase regulation of the nlrp3 inflammasome during aging. Trends Endocrinol Metab 2018;29:8-17.
27. Crişan TO, Cleophas MCP, Novakovic B, et al. Uric acid priming in human monocytes is driven by the AKT-PRAS40 autophagy pathway. Proc Natl Acad Sci U S A 2017;114:5485-90.
28. Zhao Y, Qian Y, Sun Z, et al. Role of PI3K in the progression and regression of atherosclerosis. Front Pharmacol 2021;12:632378.
29. Liang WY, Zhu XY, Zhang JW, Feng XR, Wang YC, Liu ML. Uric acid promotes chemokine and adhesion molecule production in vascular endothelium via nuclear factor-kappa B signaling. Nutr Metab Cardiovasc Dis 2015;25:187-94.
30. Feig DI. Serum uric acid and the risk of hypertension and chronic kidney disease. Curr Opin Rheumatol 2014;26:176-85.
31. Dhanasekar C, Kalaiselvan S, Rasool M. Morin, a bioflavonoid suppresses monosodium urate crystal-induced inflammatory immune response in RAW 264.7 macrophages through the inhibition of inflammatory mediators, intracellular ROS levels and NF-κB activation. PLoS One 2015;10:e0145093.
32. Xiao J, Zhang XL, Fu C, et al. Soluble uric acid increases NALP3 inflammasome and interleukin-1β expression in human primary renal proximal tubule epithelial cells through the toll-like receptor 4-mediated pathway. Int J Mol Med 2015;35:1347-54.
33. Crouch SH, Botha-Le Roux S, Delles C, Graham LA, Schutte AE. Inflammation and hypertension development: a longitudinal analysis of the African-PREDICT study. Int J Cardiol Hypertens 2020;7:100067.
34. Hong FF, Liang XY, Liu W, et al. Roles of eNOS in atherosclerosis treatment. Inflamm Res 2019;68:429-41.
35. Wei X, Zhang M, Huang S, et al. Hyperuricemia: a key contributor to endothelial dysfunction in cardiovascular diseases. FASEB J 2023;37:e23012.
36. Caldwell RW, Rodriguez PC, Toque HA, Narayanan SP, Caldwell RB. Arginase: a multifaceted enzyme important in health and disease. Physiol Rev 2018;98:641-65.
37. Papežíková I, Pekarová M, Kolářová H, et al. Uric acid modulates vascular endothelial function through the down regulation of nitric oxide production. Free Radic Res 2013;47:82-8.
38. Watanabe T, Ishikawa M, Abe K, et al. Increased lung uric acid deteriorates pulmonary arterial hypertension. J Am Heart Assoc 2021;10:e022712.
39. Kolluru GK, Siamwala JH, Chatterjee S. eNOS phosphorylation in health and disease. Biochimie 2010;92:1186-98.
40. Park JH, Jin YM, Hwang S, Cho DH, Kang DH, Jo I. Uric acid attenuates nitric oxide production by decreasing the interaction between endothelial nitric oxide synthase and calmodulin in human umbilical vein endothelial cells: a mechanism for uric acid-induced cardiovascular disease development. Nitric Oxide 2013;32:36-42.
41. Li P, Zhang L, Zhang M, Zhou C, Lin N. Uric acid enhances PKC-dependent eNOS phosphorylation and mediates cellular ER stress: A mechanism for uric acid-induced endothelial dysfunction. Int J Mol Med 2016;37:989-97.
42. Gersch C, Palii SP, Kim KM, Angerhofer A, Johnson RJ, Henderson GN. Inactivation of nitric oxide by uric acid. Nucleosides Nucleotides Nucleic Acids 2008;27:967-78.
43. Ames MK, Atkins CE, Pitt B. The renin-angiotensin-aldosterone system and its suppression. J Vet Intern Med 2019;33:363-82.
44. Yu MA, Sánchez-Lozada LG, Johnson RJ, Kang DH. Oxidative stress with an activation of the renin-angiotensin system in human vascular endothelial cells as a novel mechanism of uric acid-induced endothelial dysfunction. J Hypertens 2010;28:1234-42.
45. Mazzali M, Kanellis J, Han L, et al. Hyperuricemia induces a primary renal arteriolopathy in rats by a blood pressure-independent mechanism. Am J Physiol Renal Physiol 2002;282:F991-7.
46. Corry DB, Eslami P, Yamamoto K, Nyby MD, Makino H, Tuck ML. Uric acid stimulates vascular smooth muscle cell proliferation and oxidative stress via the vascular renin-angiotensin system. J Hypertens 2008;26:269-75.
47. Li K, Li K, Yao Q, et al. The potential relationship of coronary artery disease and hyperuricemia: a cardiometabolic risk factor. Heliyon 2023;9:e16097.
48. Yu P, Huang L, Wang Z, Meng X, Yu X. The association of serum uric acid with beta-cell function and insulin resistance in nondiabetic individuals: a cross-sectional study. Diabetes Metab Syndr Obes 2021;14:2673-82.
49. Choi HK, Ford ES. Haemoglobin A1c, fasting glucose, serum C-peptide and insulin resistance in relation to serum uric acid levels--the Third National Health and Nutrition Examination Survey. Rheumatology 2008;47:713-7.
50. Niu Y, Tang Q, Zhao X, et al. Obesity-induced insulin resistance is mediated by high uric acid in obese children and adolescents. Front Endocrinol 2021;12:773820.
51. Choi YJ, Yoon Y, Lee KY, et al. Uric acid induces endothelial dysfunction by vascular insulin resistance associated with the impairment of nitric oxide synthesis. FASEB J 2014;28:3197-204.
52. Tassone EJ, Cimellaro A, Perticone M, et al. Uric acid impairs insulin signaling by promoting enpp1 binding to insulin receptor in human umbilical vein endothelial cells. Front Endocrinol 2018;9:98.
53. Han T, Lan L, Qu R, et al. Temporal relationship between hyperuricemia and insulin resistance and its impact on future risk of hypertension. Hypertension 2017;70:703-11.
54. Wan X, Xu C, Lin Y, et al. Uric acid regulates hepatic steatosis and insulin resistance through the NLRP3 inflammasome-dependent mechanism. J Hepatol 2016;64:925-32.
55. Takir M, Kostek O, Ozkok A, et al. Lowering uric acid with allopurinol improves insulin resistance and systemic inflammation in asymptomatic hyperuricemia. J Investig Med 2015;63:924-9.
56. Mazzali M, Hughes J, Kim YG, et al. Elevated uric acid increases blood pressure in the rat by a novel crystal-independent mechanism. Hypertension 2001;38:1101-6.
57. Cristóbal-García M, García-Arroyo FE, Tapia E, et al. Renal oxidative stress induced by long-term hyperuricemia alters mitochondrial function and maintains systemic hypertension. Oxid Med Cell Longev 2015;2015:535686.
58. Sánchez-Lozada LG, Tapia E, Santamaría J, et al. Mild hyperuricemia induces vasoconstriction and maintains glomerular hypertension in normal and remnant kidney rats. Kidney Int 2005;67:237-47.
59. Romi MM, Arfian N, Tranggono U, Setyaningsih WAW, Sari DCR. Uric acid causes kidney injury through inducing fibroblast expansion, Endothelin-1 expression, and inflammation. BMC Nephrol 2017;18:326.
60. Ma H, Wang X, Guo X, Li X, Qi L, Li Y. Distinct uric acid trajectories are associated with different risks of incident hypertension in middle-aged adults. Mayo Clin Proc 2019;94:611-9.
61. Zhao H, Lu Z, Lu Y. The potential of probiotics in the amelioration of hyperuricemia. Food Funct 2022;13:2394-414.
62. Robles-Vera I, Toral M, de la Visitación N, et al. Probiotics prevent dysbiosis and the rise in blood pressure in genetic hypertension: role of short-chain fatty acids. Mol Nutr Food Res 2020;64:e1900616.
63. Li Y, Xu C, Yu C, Xu L, Miao M. Association of serum uric acid level with non-alcoholic fatty liver disease: a cross-sectional study. J Hepatol 2009;50:1029-34.
64. Sun Q, Zhang T, Manji L, et al. Association between serum uric acid and non-alcoholic fatty liver disease: an updated systematic review and meta-analysis. Clin Epidemiol 2023;15:683-93.
65. Ryoo JH, Suh YJ, Shin HC, Cho YK, Choi JM, Park SK. Clinical association between non-alcoholic fatty liver disease and the development of hypertension. J Gastroenterol Hepatol 2014;29:1926-31.
66. Zhao YC, Zhao GJ, Chen Z, She ZG, Cai J, Li H. Nonalcoholic fatty liver disease: an emerging driver of hypertension. Hypertension 2020;75:275-84.
67. Mori K, Tanaka M, Hosaka I, et al. Metabolic dysfunction-associated fatty liver disease is associated with an increase in systolic blood pressure over time: linear mixed-effects model analyses. Hypertens Res 2023;46:1110-21.
68. Abbasi S, Haleem N, Jadoon S, Farooq A. Association Of non-alcoholic fatty liver disease with serum uric acid. J Ayub Med Coll Abbottabad 2019;31:64-6.
69. Assadi F. Allopurinol enhances the blood pressure lowering effect of enalapril in children with hyperuricemic essential hypertension. J Nephrol 2014;27:51-6.
70. Beattie CJ, Fulton RL, Higgins P, et al. Allopurinol initiation and change in blood pressure in older adults with hypertension. Hypertension 2014;64:1102-7.
71. Gill D, Cameron AC, Burgess S, et al. VA Million Veteran Program. Urate, blood pressure, and cardiovascular disease: evidence from mendelian randomization and meta-analysis of clinical trials. Hypertension 2021;77:383-92.
Cite This Article
Export citation file: BibTeX | RIS
OAE Style
Wang Z, Yao G, Yan B, Zhanghuang C. The relationship between hyperuricemia and hypertension: a short review of current evidence. Metab Target Organ Damage 2024;4:3. http://dx.doi.org/10.20517/mtod.2023.41
AMA Style
Wang Z, Yao G, Yan B, Zhanghuang C. The relationship between hyperuricemia and hypertension: a short review of current evidence. Metabolism and Target Organ Damage. 2024; 4(1): 3. http://dx.doi.org/10.20517/mtod.2023.41
Chicago/Turabian Style
Wang, Zhiwei, Guiping Yao, Bing Yan, Chenghao Zhanghuang. 2024. "The relationship between hyperuricemia and hypertension: a short review of current evidence" Metabolism and Target Organ Damage. 4, no.1: 3. http://dx.doi.org/10.20517/mtod.2023.41
ACS Style
Wang, Z.; Yao G.; Yan B.; Zhanghuang C. The relationship between hyperuricemia and hypertension: a short review of current evidence. Metab Target Organ Damage. 2024, 4, 3. http://dx.doi.org/10.20517/mtod.2023.41
About This Article
Copyright
Data & Comments
Data
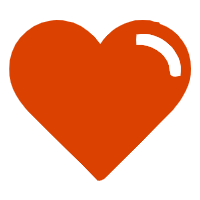

Comments
Comments must be written in English. Spam, offensive content, impersonation, and private information will not be permitted. If any comment is reported and identified as inappropriate content by OAE staff, the comment will be removed without notice. If you have any queries or need any help, please contact us at support@oaepublish.com.