The bidirectional relationship between fatty liver disease and COVID-19
Abstract
COVID-19 and nonalcoholic fatty liver disease (NAFLD) have emerged as global pandemics affecting millions of people worldwide over the past three years. NAFLD is particularly prevalent in individuals with metabolic comorbidities, such as diabetes and obesity, which have been strongly linked to a severe course of Sars-CoV-2 infection. Recently, due to the close association between metabolic abnormalities and NAFLD, the disease has been redefined as metabolic dysfunction-associated fatty liver disease (MAFLD). This review offers an overview of the biological and cellular mechanisms by which COVID-19 can cause liver damage, with a specific focus on the influence of fatty liver in these mechanisms. Additionally, it explores how fatty liver can exacerbate a COVID-19 infection and, conversely, if the presence of COVID-19 may accelerate the development and progression of fatty liver. Finally, the review examines the existing evidence suggesting that NAFLD or MAFLD independently contributes to a heightened severity of COVID-19, while also considering other factors such as age and metabolic comorbidities that may play a role in the disease’s progression.
Keywords
INTRODUCTION
The COVID-19 pandemic, caused by the SARS-CoV-2 virus, has impacted a staggering number of individuals, surpassing 620 million people, and has resulted in more than 6.5 million deaths[1]. While COVID-19 is primarily characterized by respiratory symptoms, it has become evident that multiple organs, including the digestive system and liver, can be involved[2]. Additionally, individuals with pre-existing chronic conditions have been found to experience a more severe trajectory of COVID-19, resulting in hospitalization, the need for intensive care treatments, and increased mortality rates[3]. Notably, individuals with metabolic disorders such as obesity, diabetes, and/or metabolic syndrome have shown a higher susceptibility to severe manifestations of COVID-19 based on clinical observations and studies[4]. Liver steatosis, commonly found in patients with obesity, metabolic syndrome, or diabetes, has been recognized as an independent predictor of COVID-19 severity in some research studies[5].
nonalcoholic fatty liver disease (NAFLD) is a prevalent chronic liver condition, affecting approximately 25.2% of the worldwide population[6]. The occurrence of NAFLD differs among various regions, with the Middle East (32%), South America (31%), Asia (27%), the United States (24%), and Europe (23%) reporting the highest prevalence rates, while Africa has the lowest estimated prevalence at 14%. Interestingly, NAFLD has also been identified among adolescents, with prevalence ranging from 3% to 18%[7]. NAFLD is characterized by the accumulation of fat droplets in the liver, affecting at least 5% of hepatocytes, in patients who do not consume excessive amounts of alcohol (defined as daily alcohol intake of ≥ 30 g for men
In recent years, a new term, “metabolic dysfunction-associated fatty liver disease (MAFLD)”, has been proposed to underline the metabolic mechanisms leading to hepatic fat accumulation. This terminology change was endorsed by international expert consensus[9]. The diagnosis of MAFLD is based on evidence of liver fat accumulation through histology, imaging, or blood biomarkers, along with the presence of either overweight/obesity, type 2 diabetes mellitus, or two or more metabolic risk factors in lean/normal weight individuals. It should be noted that patients meeting the criteria for MAFLD diagnosis may also have other factors contributing to fatty liver, such as viral infections, alcohol misuse, autoimmune hepatitis, or drug-induced liver injury (DILI)[10]. The ongoing debate regarding nomenclature has yet to be definitively resolved[11], so studies investigating the influence of liver steatosis on COVID-19 severity encompass patients categorized under both MAFLD and NAFLD definitions. Given the lack of consensus among researchers, this review includes studies that consider both MAFLD and NAFLD in their analysis.
The objective of this narrative review is to present a comprehensive overview of the close relationship between liver steatosis and COVID-19. We primarily focus this review on two aspects: the biological interplay between COVID-19 and liver cells, and the clinical implications of liver steatosis in COVID-19, particularly in terms of prognosis and the risk of complications.
METHODS
The biological interplay section delves into the intricate mechanisms by which COVID-19 affects liver cells. It explores the interactions between the virus and liver tissues, shedding light on the pathophysiological processes that lead to liver damage. The purpose of this section is to enhance comprehension of the fundamental biological mechanisms involved in COVID-19-mediated liver injury.
Moving on to the clinical implications, the review examines the influence of liver steatosis on COVID-19 disease. It explores the correlation between liver steatosis and the extent of COVID-19 severity, prognosis, and likelihood of developing complications. By synthesizing available evidence, the review aims to elucidate the clinical significance of liver steatosis in COVID-19 disease.
Overall, this narrative review seeks to consolidate the existing knowledge on the interplay between liver steatosis and COVID-19. By addressing both the biological and clinical aspects, it aims to provide valuable insights into the implications of liver steatosis in COVID-19 disease, contributing to a better understanding of this complex interaction.
We focused on examining the interplay between SARS-CoV-2 and liver steatosis (NAFLD/MAFLD). To address this, we conducted a thorough literature review to address two key questions: (1) is there a biological mechanism that can explain the association between the two? and (2) does this association increase the risk of progressive clinical outcomes in patients?
To begin, we provide an overview of the biological and cellular mechanisms that have been recognized as triggered by SARS-CoV-2 infection, particularly in the liver. Moreover, we investigate the potential influence or impact of fatty liver disease on these mechanisms. By examining the existing literature, we aim to shed light on the potential interplay between SARS-CoV-2 and liver steatosis at a cellular level.
Furthermore, we investigate whether NAFLD or MAFLD may serve as significant prognostic factors in COVID-19, specifically in terms of the risk of severe disease and death. To accomplish this, we conducted a literature search using the PubMed Database with specificsearch terms, including “NAFLD”, “MAFLD”, “fatty liver”, “liver steatosis”, “COVID-19”, and “SARS-CoV-2”. We included studies published in English between November 2019 and November 2022, encompassing basic science studies, clinical studies, meta-analyses, and reviews. For our narrative review, we included relevant papers that provided compelling data and theories either supporting or challenging the hypothesis that fatty liver disease acts as a risk factor for a more severe course of COVID-19.
Overall, our review aims to provide a comprehensive analysis of the association between liver steatosis and SARS-CoV-2. It specifically emphasizes the fundamental biological mechanisms involved and the potential implications for clinical outcomes in COVID-19 patients.
SARS-CoV-2 BIOLOGICAL AND CELLULAR MECHANISMS OF DAMAGE
SARS-CoV-2 virus and liver
To gain a better understanding of the association between SARS-CoV-2 and potential liver damage, it is crucial to examine the characteristics of this pathogenic microorganism. SARS-CoV-2, belonging to the Coronaviridae family and Coronavirinae subfamily, shows a strong phylogenetic correlation with Betacoronaviruses like SARS-CoV and MERS-CoV. Similar to other coronaviruses, SARS-CoV-2 possesses a positive single-stranded RNA [(+)ssRNA] genome of approximately 30kb, enclosed in a viral envelope[12,13].
The entry of the virus into host cells relies on the Spike (S) protein, which is a transmembrane protein consisting of two distinct subunits: S1, housing the receptor binding domains (RBD), and S2, responsible for membrane fusion[14,15]. When the S protein binds to its target receptor, the angiotensin-converting enzyme 2 (ACE2), host cell proteases such as transmembrane serine protease 2 (TMPRSS2), furin, and cathepsin L cleave the S2 subunit, facilitating viral entry into the cell[16,17] [Figure 1].
Figure 1. Mechanisms of liver damage by SARS-CoV-2 virus. SARS-CoV-2 can directly cause hepatic cytotoxicity by infecting hepatocytes. The consequent endoplasmic reticulum stress and mitochondrial stress contribute to steatosis. The hypoxic conditions arising from COVID-19 determine the activation of hypoxia-inducible factors (HIFs), exacerbating NAFLD. COVID-19 infection and PAMPs derived from the intestine cause hyperactivation of the immune system, leading to the cytokine storm syndrome, which worsens the pre-existent inflammatory state of NAFLD patients. SARS-CoV-2 can activate mTOR both directly and indirectly through the release of IL-6 during the cytokine storm syndrome. NAFLD: nonalcoholic fatty liver disease.
Once inside the cell cytoplasm, the viral genome, which is uncoated, is initially translated into polyproteins (pp1a and pp1ab). These polyproteins generate 16 non-structural proteins (nsp1-16) that assemble to form viral replicase-transcriptase complexes (RTCs)[15,18]. The virus then replicates its own genome, likely within double-membrane vesicles (DMVs). Subsequently, new virions can be assembled and released from host cells to propagate the infection[13].
ACE2 is well-known as the primary receptor for viral entry and plays a critical role in SARS-CoV-2 pathogenesis[19]. ACE2 is a dipeptidyl carboxypeptidase that plays a decisive role in the renin-angiotensin system, cleaving angiotensin I (Ang I) into the inactive nonapeptide form (Ang 1-9) and Ang II into the vasodilator heptapeptide Ang 1-7. Although ACE2 is primarily expressed in lung type II pneumocytes, which are the main targets of infection, this receptor is also widely distributed in several tissues such as the heart, kidneys, intestines, pancreas, muscular and nervous systems, and the liver[13,20]. Single Cell Portal data indicates that ACE2, TMPRSS2, and Furin are expressed in both cholangiocytes and hepatocytes, suggesting the possibility of viral entry and infection in liver cells[21]. Indeed, studies have demonstrated the capability of SARS-CoV-2 to infect different cell types, including Huh7 human hepatocarcinoma cells and liver organoids derived from human stem cells, indicating the potential for direct infection of liver cells and consequent hepatic injury[21-23]. Additionally, the expression of the papain-like protease domain of the
Furthermore, clinical evidence indicates elevated levels of alanine and aspartate serum transaminases (ALT and AST), gamma-glutamyl transferase (GGT), lactate dehydrogenase, and hypoalbuminemia in individuals with COVID-19, providing support for the occurrence of hepatocyte and cholangiocyte injury[25,26]. Individuals with pre-existing liver conditions, such as NAFLD, seem to have a higher vulnerability to
SARS-CoV-2 can directly cause hepatic cytotoxicity by infecting hepatocytes. The consequent endoplasmic reticulum stress and mitochondrial stress contribute to steatosis. The hypoxic conditions arising from COVID-19 determine the activation of hypoxia-inducible factors (HIFs), exacerbating NAFLD. COVID-19 infection and PAMPs derived from the intestine cause hyperactivation of the immune system, leading to the cytokine storm syndrome, which worsens the pre-existent inflammatory state of NAFLD patients. SARS-CoV-2 can activate mTOR both directly and indirectly through the release of IL-6 during the cytokine storm syndrome.
Sars-CoV-2 infection and altered Insulin homeostasis
Insulin resistance (IR) plays a significant role in the pathogenesis and progression of NAFLD/MAFLD. However, the literature exploring the association between COVID-19 and IR is limited, and the hypothetical effects of SARS-CoV-2 on insulin secretion remain unknown[31]. Nonetheless, it is well-established that ACE2 receptors, including those present in the pancreas, are widely distributed in various tissues and organs. Consequently, the virus could potentially inflict direct damage to pancreatic beta-cells and disrupt glucose metabolism[32]. The overexpression of pancreatic ACE2 receptors caused by IR creates a predisposing condition for SARS-CoV-2 infection. Furthermore, the hyperactivation of the immune response triggered by COVID-19 can exacerbate the pre-existing inflammatory state in individuals with obesity and diabetes[33]. The chronic activation of the pro-inflammatory NF-kB pathway in diabetic patients plays a significant role in increasing their vulnerability to SARS-CoV-2 infection[34]. Indeed, COVID-19 infection leads to immune system dysregulation and increased expression of inflammatory pathways, particularly NF-kB, which induces the production of cytokines resulting in cytokine storm syndrome (CSS)[35]. NF-kB also influences the polarization of macrophages into the M1 phenotype and has an impact on the expression of genes associated with the production of IL1β, IL6, TNFα, COX2, and IL12[36]. Furthermore, hyperinsulinemia may induce a pro-inflammatory profile in dendritic cells (DCs), stimulate advanced glycosylation end products (AGEs), and promote their maturation, although the underlying mechanisms of these processes are not well understood[37]. Elevated glucose levels in diabetic patients inhibit neutrophil functions such as chemotaxis, phagocytosis, and intracellular killing, thereby increasing their vulnerability to viral infections[38]. Concurrently, IR hampers anti-inflammatory pathways by reducing phosphoinositide 3-kinase activity and impairing the effects of nitric oxide (NO)[39]. Kulcsar et al. demonstrated in a diabetic mouse model infected with MERS-CoV that there was a low expression of CD4+ T cells, macrophages, IL6, IL12b, TNFα, and Arg1, but high levels of IL17a, indicating immune response dysregulation[40]. Similarly, the complex interplay between COVID-19 and diabetes may lead to deregulation of the immune response, resulting in more severe outcomes.
BIDIRECTIONAL LINK BETWEEN NAFLD/NASH AND SARS-CoV-2 INFECTION
Clinical evidence
Several risk factors linked with more severe COVID-19 disease were identified a few months after the outbreak began. Severe COVID-19 disease is defined based on specific criteria, including hypoxemia, mean low oxygen saturation (< 93% at rest) or arterial partial pressure of oxygen/fraction of inspired oxygen (P/F ratio) ≤ 300 mmHg, respiratory distress or respiratory failure requiring mechanical ventilation, shock, or the need for intensive care unit treatment due to organ failure. The mortality rate for severe COVID-19 is significantly high, ranging from 20% to above 30%[41].
Numerous studies and meta-analyses have provided evidence linking severe COVID-19 disease with certain risk factors. These include older age[42], male sex[43,44], and various comorbidities such as obesity[43-45], type 2 diabetes[46,47], cardiovascular disease[46,48], and hypertension[49]. The presence of obesity and diabetes, for instance, has been demonstrated to triple and sextuple the likelihood of severe illness, respectively[45,46]. Given the prevalence of these comorbidities in the general population, particularly in Western countries, their impact on COVID-19 disease is even more significant. In the heavily affected New York urban area during the first wave of COVID-19, the prevalence of obesity and diabetes reached 41.7% and 33.8%, respectively[50].
Although NAFLD is commonly observed in individuals with metabolic syndrome, particularly those who are obese or have diabetes[51], its independent role as a risk factor for severe COVID-19 disease remains a topic of debate. Interestingly, a meta-analysis of autopsy data from 116 COVID-19 patients revealed a higher prevalence of steatosis and fibrosis compared to the general population. Hepatic steatosis and fibrosis were found in 55% and 36% of patients, respectively[52]. In a retrospective study by Wang et al., in the overall cohort, there was no significant association found between NAFLD and the severity of COVID-19[53]. However, a subgroup analysis based on body mass index (BMI) revealed that among patients with a normal BMI (18.5-24 kg/m²), those with NAFLD were more prone to experiencing severe symptoms compared to those without NAFLD (adjusted hazard ratio [HR] = 3.26, 95% confidence interval [CI]: 1.17-9.04,
The debate extends beyond whether NAFLD/MAFLD could serve as a risk factor for SARS-CoV-2 infection incidence or severity, as it also questions whether COVID-19 infection could trigger the onset or exacerbation of metabolic chronic liver disease. These questions remain unanswered definitively. However, in the following paragraph, we will provide a comprehensive summary of the available evidence that may contribute to resolving these issues.
Clinical observations have shown that COVID-19 infection can lead to elevated levels of liver enzymes, including ALT, AST, and GGT[54]. A cohort study involving 202 participants revealed that COVID-19-related liver damage can range from mild to moderate, with a higher frequency of hepatocellular pattern compared to cholestatic pattern[55]. Cai et al. reported an association between elevated serum liver enzyme levels and the progression to severe pneumonia[56]. The occurrence of abnormal liver function tests and liver injury during acute COVID-19 infection is more common in patients with NAFLD.
In a retrospective study by Huang et al., which included 280 COVID-19 patients, 86 of whom were diagnosed with NAFLD using the Hepatic Steatosis Index (HSI), NAFLD patients exhibited higher ALT levels both on admission and during hospitalization compared to control patients (40.7% vs. 10.8%;
Numerous studies have consistently shown that patients with pre-existing chronic liver diseases, regardless of the underlying cause, experience greater severity and higher mortality rates from COVID-19[59]. In a recent meta-analysis by Nagarajan et al., the risk of severe COVID-19 disease and death was found to be twice as high among patients with chronic liver diseases compared to those without such conditions. Further subgroup analysis highlighted liver cirrhosis, MAFLD, and NAFLD as the main types of chronic liver diseases associated with severe COVID-19 disease (pooled odds ratio [OR]: 3.06, 3.20, 5.60, respectively)[60]. The connection between NAFLD/MAFLD and the cytokine storm associated with COVID-19 further supports this strong association observed in various clinical studies.
In a cohort study conducted by Gao et al., involving non-diabetic patients, 65 cases with MAFLD and 65 controls without MAFLD were included. They found that the presence of MAFLD, diagnosed by CT scan, was associated with a 4-fold increased risk of severe COVID-19 requiring intensive care[61]. In a study by Younossi et al., the outcomes of COVID-19 were examined in a cohort of 553 hospitalized patients with a documented history of NAFLD determined through imaging or liver biopsy. Upon admission, NAFLD patients displayed more pronounced respiratory symptoms, higher body temperature, and elevated levels of AST and ALT in comparison to non-NAFLD patients. The NAFLD group also had a higher proportion of patients requiring mechanical ventilation, intensive care, and longer hospital stays. However, no significant difference was observed in the mortality rate between the two groups[62]. Interestingly, FIB-4 index, along with obesity, hypoxemia, and older age, was identified as an independent predictor of mortality in this cohort.
Targher et al. evaluated 310 COVID-19 patients, including 94 with MAFLD, and found that among MAFLD patients, the severity of COVID-19 disease was higher in those with intermediate (1.3-2.67) or high (> 2.67) FIB-4 index values, independent of sex, obesity, and diabetes (adjusted OR: 2.59 and 4.04, respectively)[63]. Similarly, Yao et al. conducted a study that included 86 young patients (median age of 43.5 years) with COVID-19 and NAFLD. The diagnosis of NAFLD involved the application of the Hepatic Steatosis Index (HSI), whereas the severity of liver fibrosis was assessed using the NAFLD fibrosis score (NFS) (44.2% of NAFLD patients had advanced fibrosis). The results of the univariate analysis revealed that obesity
The interpretation of the findings from these cohort studies has been controversial, as many authors have raised concerns about the heterogeneity of ethnicity, the prevalence and diagnostic criteria of NAFLD, the variations in the standard of care for COVID-19 infection across different countries, and the differences between the diagnostic criteria of NAFLD and MAFLD[66]. Furthermore, it is still debated whether the severe course of COVID-19 disease is directly linked to NAFLD/MAFLD or indirectly associated with the various comorbidities commonly found in patients with NAFLD and MAFLD.
Several meta-analyses have been conducted in the past two years to address these concerns [Table 1].
Characteristics of Metanalysis that explored the association between NAFLD/MAFLD and severe COVID-19 or Mortality
Paper | Countries | Gender (male)^ | Age* | Cohort size COVID-19 + | NAFLD prevalence | MAFLD prevalence | NAFLD diagnosis | MAFLD diagnosis | Risk factor for | |
Severe COVID-19 | Mortality | |||||||||
Tao et al.[67] | China, USA, Israel, Qatar, and the UK | 28% - 84% | 44 - 63 | 2141 | n/a | 36% 95%CI:23-49% | n/a | consensus diagnostic criteria of MAFLD | MAFLD pOR = 1.8 (95%CI: 1.5 - 2.1) | MAFLD pOR = 0.97 (95%CI: 0.7 - 1.3) |
Pan et al.[68] | China | 25% - 74% | 42 - 47 | 1293 | n/a | 31% 95%CI:28-35% | n/a | consensus diagnostic criteria of MAFLD | MAFLD pOR = 2.9 (95%CI: 1.8 - 4.6) | n/a |
Singh et al.[69] | China, Europe, and USA | n/a | 40.9 - 70.3 | 19149 | 1851 (9,6%) | n/a | Imaging-based (US or CT) or HSI or consensus definition of NAFLD or ICD | n/a | NAFLD pOR = 2.6 (95%CI: 2.2 - 3.0) | NAFLD pOR = 1.01 (95%CI: 0.6 - 1.6) |
Sachdeva et al.[70] | USA, China, and Israel | 28%-74% | n/a | 8142 | 833 (10,2%) | n/a | Imaging-based (US or CT) or consensus definition of NAFLD | n/a | NAFLD pOR = 2.3 (95%CI: 1.9 - 2.9) | n/a |
Hegyi et al.[71] | USA, China, and Israel | 27%-64% | 42 - 63 | NAFLD comparison 7635 MAFLD comparison 948 | 592 (7,7%) | 329 (34,7%) | different methods across studies (HIS > 36 or ICD codes) | consensus diagnostic criteria of MAFLD | MAFLD pOR = 2.6 (95%CI: 1.7 - 3.9) NAFLD pOR = 5.2 (95%CI: 1.9 - 14.3) | n/a |
Wang et al.[72] | China, Europe, and USA | n/a | n/a | 22056 | n/a | n/a | n/a | n/a | NAFLD pOR = 1.7 (95%CI: 1.2 - 2.5) | n/a |
Hegyi et al. conducted a study that included nine studies from USA, China, and Israel, with a homogeneous definition of MAFLD[71]. However, the diagnosis of NAFLD varied across the studies, using different methods such as his > 36 or ICD codes. The results showed that both MAFLD and NAFLD were associated with an increased risk of severe COVID-19 disease, with OR of 2.61 and 5.22, respectively.
In a meta-analysis conducted by Sachdeva et al., eight studies involving 8,142 COVID-positive patients were included. Among these patients, 833 were diagnosed with NAFLD using computed tomography (CT) or abdominal ultrasound (US) and according to an internal expert consensus statement. The analysis revealed that NAFLD was linked to a higher risk of severe COVID-19, including hospitalization and ICU admission. This association remained significant even after adjusting for obesity as a confounding factor. The pooled odds ratio (OR) for severe COVID-19 in individuals with NAFLD, while accounting for obesity, was 2.36 (95%CI: 1.90-2.92, P < 0.01)[70] .
In another meta-analysis by Singh et al., 14 studies with a total of 19,149 COVID-19 patients were included, including 1,851 NAFLD patients[69]. The diagnosis of NAFLD was confirmed through imaging using ultrasound (US) or computed tomography (CT), HSI, consensus definition of NAFLD, or the International Classification of Diseases (ICD) code. The findings from the included studies supported the association between NAFLD and severe COVID-19, as indicated by the reported ORs. After analyzing data from eight included studies, it was found that patients with NAFLD had a significantly higher adjusted OR for severe COVID-19 compared to those without NAFLD (OR: 2.60, 95%CI: 2.24-3.02, P < 0.01). Meta-regression analysis revealed that the risk of severe COVID-19 in NAFLD patients was not influenced by age (P = 0.65) or BMI (P = 0.29). Regarding ICU admission, the pooled OR based on data from two studies showed that patients with NAFLD had a higher likelihood of being admitted to the ICU compared to those without NAFLD (OR: 1.66, 95%CI: 1.26-2.20, P < 0.01) for patients with NAFLD compared to those without NAFLD, based on data from two studies, was 1.66 (95%CI: 1.26-2.20, P < 0.001). However, no significant difference in mortality was observed between patients with NAFLD and those without NAFLD.
In a recent meta-analysis, which included 18 studies with 22,056 patients, the presence of NAFLD was found to be significantly linked to a more severe form of COVID-19, as indicated by a pooled OR of 1.76[72]. Notably, this association retained its significance even after adjusting for various factors such as sex, age, smoking habits, diabetes, obesity, and hypertension. Interestingly, subgroup analysis based on age demonstrated that the association between NAFLD and severe COVID-19 was consistent among younger patients (< 60 years) with a pooled OR of 2.08 (95%CI: 1.33-3.27), but not among older patients (> 60 years) with a pooled OR of 1.37 (95%CI: 0.97-1.93)[72]. These results suggest that NAFLD may have a prognostic impact on COVID-19 disease, particularly in young patients. However, in older patients, other comorbidities might outweigh NAFLD as prognostic factors.
Despite the studies mentioned above suggesting NAFLD/MAFLD as a risk factor for severe COVID-19, conflicting data exist in the literature, leading to the ongoing debate within the hepatology field[73].
During the first wave of COVID-19, Forlano et al. conducted a study involving a cohort of 193 patients from the UK and did not find any association between NAFLD and severe outcomes of COVID-19 disease[73]. Interestingly, in this cohort, NAFLD patients exhibited higher levels of C-reactive protein than non-NAFLD patients, potentially indicating an augmented COVID-19-related inflammatory response in individuals with NAFLD.
Mushtaq et al. examined a cohort of 589 patients, among whom 320 (54.3%) were found to have NAFLD based on HSI. However, the presence of NAFLD did not emerge as an independent predictor of increased mortality, disease severity upon presentation, or disease progression. Instead, older age (> 50 years) stood out as the sole independent predictor of mortality, while age (> 50 years) and overweight (BMI > 25 kg/m2) were the only two significant and independent predictors of disease progression (including mechanical ventilation, intensive care, and the development of ARDS). Notably, the presence of NAFLD emerged as an independent predictor solely for the occurrence of mild-to-moderate liver injury[74].
Furthermore, a recent Mendelian randomization analysis was conducted to further investigate a possible causal relationship between NAFLD and severe COVID-19 disease. The analysis revealed that NAFLD, ALT, grade of steatosis, and fibrosis stage were not associated with severe COVID-19. However, BMI, waist circumference, and hip circumference showed significant and independent associations with severe COVID-19 disease[75].
Possible mechanistic nexus between NAFLD/MAFLD-related hepatic damage and SARS-CoV-2 infection
Therefore, it is crucial to further investigate the mechanisms of COVID-19-mediated hepatic damage. SARS-CoV-2 can directly cause cytotoxicity in the liver by infecting hepatocytes that express the ACE2 receptor. In fact, liver abnormalities such as endoplasmic reticulum dilatation, mitochondrial stress, and apoptosis have been observed in COVID-19 patients[76]. This mitochondrial stress leads to impairment in beta-oxidation, resulting in lipid accumulation and the development of microvesicular steatosis.
Considering that steatosis is a hallmark of NAFLD/MAFLD, SARS-CoV-2 infection may exacerbate the metabolic profile of patients with these conditions[13].
Notably, it is well-established that endoplasmic reticulum (ER) stress can contribute to hepatic steatosis through various mechanisms, such as the induction of de novo lipogenesis, disruption of very low-density lipoprotein (VLDL) secretion, and impairment of insulin signaling[77]. Coronavirus proteins, including the S protein, have been shown to induce ER stress. This occurs when viral proteins accumulate within the ER, leading to the disruption of its normal folding capacity and triggering the unfolded protein response (UPR). The UPR activates key stress factors, such as transcription factor 6 (ATF6), inositol-requiring enzyme 1 (IRE1) and PKR-like ER kinase (PERK)[78]. Moreover, SARS-CoV-infected cells increase the expression of ER stress-associated genes (GRP94 and GRP78)[79].
The mammalian target of rapamycin (mTOR) pathway is another potential mechanism linking COVID-19 with NAFLD/MAFLD. Previous in vitro studies on Huh7 cells infected with MERS-CoV showed direct activation of mTOR[80]. Additionally, interleukin-6 (IL-6), a major inflammatory factor released during the cytokine storm syndrome caused by SARS-CoV-2 infection, can indirectly activate the mTOR pathway[81]. Considering that insulin also triggers the mTOR pathway in the liver, hyperactivation of mTOR activity in patients with metabolic diseases could lead to worse outcomes in COVID-19[13].
Hypoxia is another mechanism that may connect COVID-19 with NAFLD/MAFLD. SARS-CoV-2 primarily affects the respiratory system, impairing gas exchange and leading to acute respiratory distress syndrome (ARDS) and systemic hypoxemia in severe cases[82]. Hypoxia triggers the activation of transcription factors known as hypoxia-inducible factors (HIFs) at the cellular level[83]. Current evidence suggests that HIF signaling can result in altered metabolic conditions. Specifically, studies have shown that upregulation of HIF-2α suppresses peroxisome proliferator-activated receptor alpha (PPARα) in the liver, exacerbating NAFLD[84]. Conversely, HIF-1α inhibits the expression of TMPRSS2 and ACE2, indicating that hypoxic conditions may impair viral infection[82].
Liver steatosis, particularly NASH, is characterized by hepatic inflammation and innate immune activation, which play an essential role in initiating and perpetuating the inflammatory state within the liver[85,86]. Similarly, COVID-19 infection induces a significant release of cytokines that can impact various organs, including the liver[25]. Following infection, pathogenic T cells become activated, leading to the production of inflammatory mediators such as IL-6 and granulocyte-macrophage colony-stimulating factor (GM-CSF), which further activate inflammatory monocytes. This cascade results in the generation of a large quantity of pro-inflammatory factors, contributing to cytokine storm syndrome (CSS)[87]. Notably, IL-6 plays a pivotal role in the initiation and progression of CSS in COVID-19 patients, and elevated levels of IL-6 have been observed in individuals with metabolic conditions such as diabetes, obesity, and NAFLD[86,88].
Furthermore, resident macrophages in the liver, known as Kupffer cells, can modulate the inflammatory response. These macrophages can be classified into two sub-phenotypes: M1, which promotes pro-inflammatory responses, and M2, which exhibits anti-inflammatory functions. In patients with MAFLD, there is a predominance of M1 polarization and a suppression of M2 polarization, which may contribute to the progression of COVID-19 infection[89]. Individuals with pre-existing liver diseases are more susceptible to experiencing gastrointestinal symptoms following SARS-CoV-2 infection[89]. Given that the small intestine expresses a high level of ACE2 receptors, the virus can infect the gut, potentially leading to the release of pathogen-associated molecular patterns (PAMPs) into the portal vein of the liver. Consequently, the hyperactivation of the immune response triggered by PAMPs can contribute to the development of CSS[87]. Finally, gut dysbiosis, characterized by alterations in the microbiome and the translocation of microorganisms into the portal circulation in NAFLD/NASH patients, may represent an additional risk factor for the outcome and progression of COVID-19[87].
DISCUSSION
In recent years, clinical practice has revealed that patients with metabolic comorbidities, including obesity, diabetes, and metabolic syndrome, face a higher risk of severe SARS-CoV-2 infection. Fatty liver disease has been proposed as one of the key factors contributing to this association. It is now well-established that the ACE2 receptor, which facilitates viral entry into cells, is widely expressed in various organs and tissues, including the liver[13]. Notably, cases of NAFLD/MAFLD often exhibit overexpression of the ACE2 receptor, potentially enabling direct infection of hepatic cells by SARS-CoV-2 and causing liver damage. Furthermore, the presence of comorbidities can increase the susceptibility of NAFLD/MAFLD patients to SARS-CoV-2 infection. Increased insulin resistance leads to the upregulation of pancreatic ACE2 receptors and impairs neutrophil functions, thereby enhancing vulnerability to viral infection[38].
Although a clear causal relationship between NAFLD/MAFLD and the development of COVID-19 has yet to be established, clinical observations suggest that patients with pre-existing metabolic conditions are more prone to SARS-CoV-2 infection. Moreover, COVID-19 may exacerbate patients' underlying metabolic disorders, potentially promoting the progression of NAFLD to NASH in the long term, although the underlying mechanism remains unclear[90]. Consistently, SARS-CoV-2 has been found to induce mitochondrial and endoplasmic reticulum stress, leading to lipid accumulation and steatosis, thereby exacerbating the metabolic profile of NAFLD patients[13]. In vitro experiments have demonstrated that SARS-CoV-2 can directly and indirectly activate the mTOR pathway, which in turn promotes de novo lipogenesis[80]. These findings indicate that COVID-19 may act as a risk factor for exacerbation and progression of NAFLD/MAFLD.
Fatty liver disease may also contribute to the systemic inflammation triggered by SARS-CoV-2 infection. In NAFLD patients, an excess of free fatty acids enters the liver and activates Kupffer cells, resulting in a shift from anti-inflammatory M2 polarization to pro-inflammatory M1 polarization and increased production of pro-inflammatory cytokines[89]. Concurrently, COVID-19 triggers a substantial release of pro-inflammatory factors, with IL-6 playing a crucial role[87]. Hence, it is reasonable to speculate that systemic inflammation significantly contributes to the intensified hepatic inflammatory condition observed in NAFLD/MAFLD patients experiencing severe COVID-19 outcomes.
From a clinical standpoint, the studies and meta-analyses discussed in this review present contradictory findings regarding the relationship between NAFLD/MAFLD and the onset of severe COVID-19. In fact, the relationship between NAFLD/MAFLD and severe COVID-19 disease remains a topic of debate in the literature due to several potential biases, conflicting results and a lack of prospective cohort studies. One potential bias arises from the varying definitions of "fatty liver disease" used in the reviewed studies. Some studies refer to MAFLD, others to NAFLD, and many consider both conditions, leading to inconsistencies. Additionally, the diagnosis and assessment of disease severity rely on various methods, such as non-invasive scores (HIS, FIB4, NFS), imaging-based diagnosis (ultrasound or CT), or liver biopsy, further contributing to potential biases among included studies. These biases likely contribute to the significant heterogeneity observed regarding the prevalence of NAFLD and MAFLD in different studies. For instance, the prevalence of NAFLD ranged from 5.5% to 38%, while MAFLD prevalence ranged from 28% to 50% among the studies included in the meta-analysis by Hegyi et al.[71]. In fact, one of the main distinctions between patients with MAFLD and NAFLD is the presence of an additional underlying cause for chronic liver disease, such as alcohol consumption or chronic viral infections. This difference in etiology can readily explain the varying prevalence of these two conditions observed in studies, and it may also contribute to the contrasting prognostic impact of NAFLD and MAFLD in COVID-19-related illness. Moreover, it has been demonstrated that alcohol consumption has significantly increased during the pandemic, potentially further influencing the disparities in susceptibility to severe COVID-19 between NAFLD and MAFLD[91].
CONCLUSION
In conclusion, the relationship between NAFLD/MAFLD and COVID-19 has garnered significant attention in recent research. Accumulating evidence suggests that individuals with NAFLD/MAFLD are at an increased risk of developing severe forms of COVID-19, leading to worse clinical outcomes. The underlying mechanisms linking these two conditions involve multiple pathways, including the dysregulation of the renin-angiotensin-aldosterone system, increased expression of ACE2 receptors in the liver, enhanced systemic inflammation, and polarization shift of hepatic macrophages from anti-inflammatory M2 to pro-inflammatory M1 phenotype. Moreover, the interaction between SARS-CoV-2 and the liver can induce endoplasmic reticulum stress, mitochondrial dysfunction, and the activation of pro-inflammatory pathways, further contributing to liver injury and inflammation.
It is important to acknowledge the limitations and potential biases in the existing studies investigating the relationship between NAFLD/MAFLD and COVID-19. Metabolic dysregulation, a hallmark of NAFLD/MAFLD, also plays a significant role in the susceptibility to and severity of COVID-19. The presence of obesity, insulin resistance, and type 2 diabetes, which are often linked to NAFLD/MAFLD, are recognized risk factors for severe COVID-19. Therefore, it is crucial to give thorough consideration and appropriately account for these factors in the analyses. Furthermore, variations in the definitions and diagnostic criteria of fatty liver disease, as well as the diverse methods used to assess disease severity, introduce heterogeneity in the reported associations.
Despite these limitations, the available evidence from meta-analyses consistently supports the notion that NAFLD/MAFLD is an independent risk factor for the development of severe COVID-19. The pooled OR reported in these meta-analyses indicate a significantly higher risk of adverse outcomes, including the need for intensive care and/or mechanical ventilation, and extended hospital stays, among individuals with NAFLD/MAFLD. However, it is important to note that no significant association between fatty liver disease and COVID-19 mortality has been documented to date.
Considering the growing body of research, it is imperative to conduct further studies to elucidate the underlying mechanisms.
DECLARATIONS
Author’s contributionConception or design: Liguori A, Calvez V, Miele L
Extracted data: Liguori A, Calvez V, Sciarra A, D’Ambrosio F
Acquisition, analysis, or interpretation of data: Liguori A, Calvez V, Sciarra A, D’Ambrosio F
Created the figure of the manuscript with https://app.biorender.com/biorender-templates: D’Ambrosio F
Drafting the work or revising: Liguori A, Calvez V, Sciarra A, D’Ambrosio F, Marrone G, Biolato M, Gasbarrini A, Grieco A, Alisi A, Miele L
Final approval of the manuscript: Liguori A, Calvez V, D’Ambrosio F, Sciarra A, Marrone G, Biolato M, Gasbarrini A, Grieco A, Alisi A, Miele L
Availability of data and materialsNot applicable.
Financial support and sponsorshipNone.
Conflicts of interestAll authors declared that there are no conflicts of interest.
Ethical approval and consent to participateNot applicable.
Consent for publicationNot applicable.
Copyright© The Author 2023.
REFERENCES
1. Wiersinga WJ, Rhodes A, Cheng AC, Peacock SJ, Prescott HC. Pathophysiology, transmission, diagnosis, and treatment of coronavirus disease 2019 (COVID-19): a review. JAMA 2020;324:782-93.
2. Díaz LA, García-Salum T, Fuentes-López E, Ferrés M, Medina RA, Riquelme A. Symptom profiles and risk factors for hospitalization in patients with SARS-CoV-2 and COVID-19: a large cohort from South America. Gastroenterology 2020;159:1148-50.
3. Ejaz H, Alsrhani A, Zafar A, et al. COVID-19 and comorbidities: deleterious impact on infected patients. J Infect Public Health 2020;13:1833-9.
4. Zhou Y, Chi J, Lv W, Wang Y. Obesity and diabetes as high-risk factors for severe coronavirus disease 2019 (Covid-19). Diabetes Metab Res Rev 2021;37:e3377.
5. Steenblock C, Schwarz PEH, Ludwig B, et al. COVID-19 and metabolic disease: mechanisms and clinical management. Lancet Diabetes Endocrinol 2021;9:786-98.
6. Younossi ZM, Golabi P, de Avila L, et al. The global epidemiology of NAFLD and NASH in patients with type 2 diabetes: a systematic review and meta-analysis. J Hepatol 2019;71:793-801.
7. Mundi MS, Velapati S, Patel J, Kellogg TA, Abu Dayyeh BK, Hurt RT. Evolution of NAFLD and its management. Nutr Clin Pract 2020;35:72-84.
8. European Association for the Study of the Liver (EASL), European Association for the Study of Diabetes (EASD), European Association for the Study of Obesity (EASO). EASL-EASD-EASO clinical practice guidelines for the management of nonalcoholic fatty liver disease. J Hepatol 2016;64:1388-402.
9. Eslam M, Newsome PN, Sarin SK, et al. A new definition for metabolic dysfunction-associated fatty liver disease: an international expert consensus statement. J Hepatol 2020;73:202-9.
10. Eslam M, Sanyal AJ, George J; International Consensus Panel. MAFLD: a consensus-driven proposed nomenclature for metabolic associated fatty liver disease. Gastroenterology 2020;158:1999-2014.e1.
11. Younossi ZM, Rinella ME, Sanyal AJ, et al. From NAFLD to MAFLD: implications of a premature change in terminology. Hepatology 2021;73:1194-8.
12. Mohamadian M, Chiti H, Shoghli A, Biglari S, Parsamanesh N, Esmaeilzadeh A. COVID-19: virology, biology and novel laboratory diagnosis. J Gene Med 2021;23:e3303.
13. Nardo AD, Schneeweiss-Gleixner M, Bakail M, Dixon ED, Lax SF, Trauner M. Pathophysiological mechanisms of liver injury in COVID-19. Liver Int 2021;41:20-32.
14. Khailany RA, Safdar M, Ozaslan M. Genomic characterization of a novel SARS-CoV-2. Gene Rep 2020;19:100682.
15. Fehr AR, Perlman S. Coronaviruses: an overview of their replication and pathogenesis. Methods Mol Biol 2015;1282:1-23.
16. V'kovski P, Kratzel A, Steiner S, Stalder H, Thiel V. Coronavirus biology and replication: implications for SARS-CoV-2. Nat Rev Microbiol 2021;19:155-70.
17. Voto C, Berkner P, Brenner C. Overview of the pathogenesis and treatment of SARS-CoV-2 for clinicians: a comprehensive literature review. Cureus 2020;12:e10357.
18. Tan YJ, Lim SG, Hong W. Understanding the accessory viral proteins unique to the severe acute respiratory syndrome (SARS) coronavirus. Antiviral Res 2006;72:78-88.
19. Datta PK, Liu F, Fischer T, Rappaport J, Qin X. SARS-CoV-2 pandemic and research gaps: Understanding SARS-CoV-2 interaction with the ACE2 receptor and implications for therapy. Theranostics 2020;10:7448-64.
20. Tikellis C, Thomas MC. Angiotensin-converting enzyme 2 (ACE2) is a key modulator of the renin angiotensin system in health and disease. Int J Pept Res Ther 2012;2012:1-8.
21. Pirola CJ, Sookoian S. SARS-CoV-2 virus and liver expression of host receptors: Putative mechanisms of liver involvement in COVID-19. Liver Int 2020;40:2038-40.
22. Yang L, Han Y, Nilsson-Payant BE, et al. A human pluripotent stem cell-based platform to study sars-cov-2 tropism and model virus infection in human cells and organoids. Cell Stem Cell 2020;27:125-136.e7.
23. Ou X, Liu Y, Lei X, et al. Characterization of spike glycoprotein of SARS-CoV-2 on virus entry and its immune cross-reactivity with SARS-CoV. Nat Commun 2020;11:1620.
24. Crudele A, Smeriglio A, Ingegneri M, et al. Hydroxytyrosol recovers SARS-CoV-2-PLpro-Dependent impairment of interferon related genes in polarized human airway, intestinal and liver epithelial cells. Antioxidants 2022;11:1466.
25. Li J, Fan JG. Characteristics and mechanism of liver injury in 2019 coronavirus disease. J Clin Transl Hepatol 2020;8:13-7.
26. Zhang C, Shi L, Wang FS. Liver injury in COVID-19: management and challenges. Lancet Gastroenterol 2020;5:428-30.
27. Vujčić I. Outcomes of COVID-19 among patients with liver disease. World J Gastroenterol 2023;29:815-24.
28. Huang Q, Xie Q, Shi CC, et al. Expression of angiotensin-converting enzyme 2 in CCL4-induced rat liver fibrosis. Int J Mol Med 2009:23.
29. Fondevila MF, Mercado-Gómez M, Rodríguez A, et al. Obese patients with NASH have increased hepatic expression of SARS-CoV-2 critical entry points. J Hepatol 2021;74:469-71.
30. Singh MK, Mobeen A, Chandra A, Joshi S, Ramachandran S. A meta-analysis of comorbidities in COVID-19: which diseases increase the susceptibility of SARS-CoV-2 infection? Comput Biol Med 2021;130:104219.
31. Sharifi Y, Payab M, Mohammadi-Vajari E, et al. Association between cardiometabolic risk factors and COVID-19 susceptibility, severity and mortality: a review. J Diabetes Metab Disord 2021;20:1743-65.
32. Rubino F, Amiel SA, Zimmet P, et al. New-onset diabetes in Covid-19. N Engl J Med 2020;383:789-90.
33. Govender N, Khaliq OP, Moodley J, Naicker T. Insulin resistance in COVID-19 and diabetes. Prim Care Diabetes 2021;15:629-34.
34. Mozafari N, Azadi S, Mehdi-Alamdarlou S, Ashrafi H, Azadi A. Inflammation: a bridge between diabetes and COVID-19, and possible management with sitagliptin. Med Hypotheses 2020;143:110111.
35. Li X, Geng M, Peng Y, Meng L, Lu S. Molecular immune pathogenesis and diagnosis of COVID-19. J Pharm Anal 2020;10:102-8.
36. Dorrington MG, Fraser IDC. NF-κB signaling in macrophages: dynamics, crosstalk, and signal integration. Front Immunol 2019;10:705.
37. Ge J, Jia Q, Liang C, et al. Advanced glycosylation end products might promote atherosclerosis through inducing the immune maturation of dendritic cells. Arterioscler Thromb Vasc Biol 2005;25:2157-63.
38. Alba-Loureiro TC, Munhoz CD, Martins JO, et al. Neutrophil function and metabolism in individuals with diabetes mellitus. Braz J Med Biol Res 2007;40:1037-44.
39. Korakas E, Ikonomidis I, Kousathana F, et al. Obesity and COVID-19: immune and metabolic derangement as a possible link to adverse clinical outcomes. Am J Physiol Endocrinol Metab 2020;319:E105-9.
40. Kulcsar KA, Coleman CM, Beck SE, Frieman MB. Comorbid diabetes results in immune dysregulation and enhanced disease severity following MERS-CoV infection. JCI Insight 2019;4:131774.
41. Tian W, Jiang W, Yao J, et al. Predictors of mortality in hospitalized COVID-19 patients: a systematic review and meta-analysis. J Med Virol 2020;92:1875-83.
42. Zhang JJ, Cao YY, Tan G, et al. Clinical, radiological, and laboratory characteristics and risk factors for severity and mortality of 289 hospitalized COVID-19 patients. Allergy 2021;76:533-50.
43. Rapp JL, Lieberman-Cribbin W, Tuminello S, Taioli E. Male sex, severe obesity, older age, and chronic kidney disease are associated with COVID-19 severity and mortality in New York city. Chest 2021;159:112-5.
44. Ebinger JE, Achamallah N, Ji H, et al. Pre-existing traits associated with Covid-19 illness severity. PLoS One 2020;15:e0236240.
45. Gao F, Zheng KI, Wang XB, et al. Obesity is a risk factor for greater COVID-19 severity. Diabetes Care 2020;43:e72-4.
46. Clift AK, Coupland CAC, Keogh RH, et al. Living risk prediction algorithm (QCOVID) for risk of hospital admission and mortality from coronavirus 19 in adults: national derivation and validation cohort study. BMJ 2020;371:m3731.
47. Dennis JM, Mateen BA, Sonabend R, et al. Type 2 diabetes and COVID-19-related mortality in the critical care setting: a national cohort study in England, March-July 2020. Diabetes Care 2021;44:50-7.
48. Williamson EJ, Walker AJ, Bhaskaran K, et al. Factors associated with COVID-19-related death using OpenSAFELY. Nature 2020;584:430-6.
49. Li X, Xu S, Yu M, et al. Risk factors for severity and mortality in adult COVID-19 inpatients in Wuhan. J Allergy Clin Immunol 2020;146:110-8.
50. Richardson S, Hirsch JS, Narasimhan M, et al. the Northwell COVID-19 Research Consortium. Presenting characteristics, comorbidities, and outcomes among 5700 patients hospitalized with COVID-19 in the New York City Area. JAMA 2020;323:2052-9.
51. Henry L, Paik J, Younossi ZM. Review article: the epidemiologic burden of nonalcoholic fatty liver disease across the world. Aliment Pharmacol Ther 2022;56:942-56.
52. Díaz LA, Idalsoaga F, Cannistra M, et al. High prevalence of hepatic steatosis and vascular thrombosis in COVID-19: a systematic review and meta-analysis of autopsy data. World J Gastroenterol 2020;26:7693-706.
53. Wang G, Wu S, Wu C, et al. Association between nonalcoholic fatty liver disease with the susceptibility and outcome of COVID-19: a retrospective study. J Cell Mol Med 2021;25:11212-20.
54. Chen N, Zhou M, Dong X, et al. Epidemiological and clinical characteristics of 99 cases of 2019 novel coronavirus pneumonia in Wuhan, China: a descriptive study. Lancet 2020;395:507-13.
55. Ji D, Qin E, Xu J, et al. Non-alcoholic fatty liver diseases in patients with COVID-19: a retrospective study. J Hepatol 2020;73:451-3.
57. Huang R, Zhu L, Wang J, et al. Clinical features of patients with COVID-19 with nonalcoholic fatty liver disease. Hepatol Commun 2020;4:1758-68.
58. Portincasa P, Krawczyk M, Smyk W, Lammert F, Di Ciaula A. COVID-19 and nonalcoholic fatty liver disease: two intersecting pandemics. Eur J Clin Invest 2020;50:e13338.
59. Martinez MA, Franco S. Impact of COVID-19 in liver disease progression. Hepatol Commun 2021;5:1138-50.
60. Nagarajan R, Krishnamoorthy Y, Rajaa S, Hariharan VS. COVID-19 severity and mortality among chronic liver disease patients: a systematic review and meta-analysis. Prev Chronic Dis 2022;19:E53.
61. Gao F, Zheng KI, Wang XB, et al. Metabolic associated fatty liver disease increases coronavirus disease 2019 disease severity in nondiabetic patients. J Gastroenterol Hepatol 2021;36:204-7.
62. Younossi ZM, Stepanova M, Lam B, et al. Independent predictors of mortality among patients with NAFLD hospitalized with COVID-19 infection. Hepatol Commun 2022;6:3062-72.
63. Targher G, Mantovani A, Byrne CD, et al. Risk of severe illness from COVID-19 in patients with metabolic dysfunction-associated fatty liver disease and increased fibrosis scores. Gut 2020;69:1545-7.
64. Yao R, Zhu L, Wang J, et al. Risk of severe illness of COVID-19 patients with NAFLD and increased NAFLD fibrosis scores. J Clin Lab Anal 2021;35:e23880.
65. Vázquez-Medina MU, Cerda-Reyes E, Galeana-Pavón A, et al. Interaction of metabolic dysfunction-associated fatty liver disease and nonalcoholic fatty liver disease with advanced fibrosis in the death and intubation of patients hospitalized with coronavirus disease 2019. Hepatol Commun 2022;6:2000-10.
66. Marjot T, Eberhardt CS, Boettler T, et al. Impact of COVID-19 on the liver and on the care of patients with chronic liver disease, hepatobiliary cancer, and liver transplantation: an updated EASL position paper. J Hepatol 2022;77:1161-97.
67. Tao Z, Li Y, Cheng B, Zhou T, Gao Y. Risk of severe COVID-19 increased by metabolic dysfunction-associated fatty liver disease: a meta-analysis. J Clin Gastroenterol 2021;55:830-5.
68. Pan L, Huang P, Xie X, Xu J, Guo D, Jiang Y. Metabolic associated fatty liver disease increases the severity of COVID-19: a meta-analysis. Dig Liver Dis 2021;53:153-7.
69. Singh A, Hussain S, Antony B. nonalcoholic fatty liver disease and clinical outcomes in patients with COVID-19: a comprehensive systematic review and meta-analysis. Diabetes Metab Syndr 2021;15:813-22.
70. Sachdeva S, Khandait H, Kopel J, Aloysius MM, Desai R, Goyal H. NAFLD and COVID-19: a pooled analysis. SN Compr Clin Med 2020;2:2726-9.
71. Hegyi PJ, Váncsa S, Ocskay K, et al. Metabolic associated fatty liver disease is associated with an increased risk of severe COVID-19: a systematic review with meta-analysis. Front Med 2021;8:626425.
72. Wang Y, Wang Y, Duan G, Yang H. NAFLD was independently associated with severe COVID-19 among younger patients rather than older patients: a meta-analysis. J Hepatol 2023;78:e136-9.
73. Forlano R, Mullish BH, Mukherjee SK, et al. In-hospital mortality is associated with inflammatory response in NAFLD patients admitted for COVID-19. PLoS One 2020;15:e0240400.
74. Mushtaq K, Khan MU, Iqbal F, et al. NAFLD is a predictor of liver injury in COVID-19 hospitalized patients but not of mortality, disease severity on the presentation or progression - the debate continues. J Hepatol 2021;74:482-4.
75. Li J, Tian A, Zhu H, et al. Mendelian randomization analysis reveals no causal relationship between nonalcoholic fatty liver disease and severe COVID-19. Clin Gastroenterol H 2022;20:1553-1560.e78.
76. Wang Y, Liu S, Liu H, et al. SARS-CoV-2 infection of the liver directly contributes to hepatic impairment in patients with COVID-19. J Hepatol 2020;73:807-16.
77. Lebeaupin C, Vallée D, Hazari Y, Hetz C, Chevet E, Bailly-Maitre B. Endoplasmic reticulum stress signalling and the pathogenesis of nonalcoholic fatty liver disease. J Hepatol 2018;69:927-47.
78. Chan CP, Siu KL, Chin KT, Yuen KY, Zheng B, Jin DY. Modulation of the unfolded protein response by the severe acute respiratory syndrome coronavirus spike protein. J Virol 2006;80:9279-87.
79. Banerjee A, Czinn SJ, Reiter RJ, Blanchard TG. Crosstalk between endoplasmic reticulum stress and anti-viral activities: a novel therapeutic target for COVID-19. Life Sci 2020;255:117842.
80. Kindrachuk J, Ork B, Hart BJ, et al. Antiviral potential of ERK/MAPK and PI3K/AKT/mTOR signaling modulation for Middle East respiratory syndrome coronavirus infection as identified by temporal kinome analysis. Antimicrob Agents Ch 2015;59:1088-99.
81. He M, Shi X, Yang M, Yang T, Li T, Chen J. Mesenchymal stem cells-derived IL-6 activates AMPK/mTOR signaling to inhibit the proliferation of reactive astrocytes induced by hypoxic-ischemic brain damage. Exp Neurol 2019;311:15-32.
82. Serebrovska ZO, Chong EY, Serebrovska TV, Tumanovska LV, Xi L. Hypoxia, HIF-1α, and COVID-19: from pathogenic factors to potential therapeutic targets. Acta Pharmacol Sin 2020;41:1539-46.
83. Gonzalez FJ, Xie C, Jiang C. The role of hypoxia-inducible factors in metabolic diseases. Nat Rev Endocrinol 2018;15:21-32.
84. Chen J, Chen J, Fu H, et al. Hypoxia exacerbates nonalcoholic fatty liver disease via the HIF-2α/PPARα pathway. Am J Physiol-Endoc M 2019;317:E710-22.
85. Arrese M, Cabrera D, Kalergis AM, Feldstein AE. Innate immunity and inflammation in NAFLD/NASH. Dig Dis Sci 2016;61:1294-303.
86. Schmidt-Arras D, Rose-John S. IL-6 pathway in the liver: from physiopathology to therapy. J Hepatol 2016;64:1403-15.
87. Miele L, Napodano C, Cesario A, et al. COVID-19, adaptative immune response and metabolic-associated liver disease. Liver Int 2021;41:2560-77.
88. Fricker ZP, Pedley A, Massaro JM, et al. Liver fat is associated with markers of inflammation and oxidative stress in analysis of data from the framingham heart study. Clin Gastroenterol Hepatol 2019;17:1157-1164.e4.
89. Sharma P, Kumar A, Anikhindi S, et al. Effect of COVID-19 on pre-existing liver disease: what hepatologist should know? J Clin Exp Hepatol 2021;11:484-93.
90. Prins GH, Olinga P. Potential implications of COVID-19 in nonalcoholic fatty liver disease. Liver Int 2020;40:2568.
Cite This Article
Export citation file: BibTeX | RIS
OAE Style
Liguori A, Calvez V, D’Ambrosio F, Sciarra A, Marrone G, Biolato M, Grieco A, Gasbarrini A, Alisi A, Miele L. The bidirectional relationship between fatty liver disease and COVID-19. Metab Target Organ Damage 2023;3:11. http://dx.doi.org/10.20517/mtod.2022.36
AMA Style
Liguori A, Calvez V, D’Ambrosio F, Sciarra A, Marrone G, Biolato M, Grieco A, Gasbarrini A, Alisi A, Miele L. The bidirectional relationship between fatty liver disease and COVID-19. Metabolism and Target Organ Damage. 2023; 3(3): 11. http://dx.doi.org/10.20517/mtod.2022.36
Chicago/Turabian Style
Liguori, Antonio, Valentin Calvez, Francesca D’Ambrosio, Angela Sciarra, Giuseppe Marrone, Marco Biolato, Antonio Grieco, Antonio Gasbarrini, Anna Alisi, Luca Miele. 2023. "The bidirectional relationship between fatty liver disease and COVID-19" Metabolism and Target Organ Damage. 3, no.3: 11. http://dx.doi.org/10.20517/mtod.2022.36
ACS Style
Liguori, A.; Calvez V.; D’Ambrosio F.; Sciarra A.; Marrone G.; Biolato M.; Grieco A.; Gasbarrini A.; Alisi A.; Miele L. The bidirectional relationship between fatty liver disease and COVID-19. Metab Target Organ Damage. 2023, 3, 11. http://dx.doi.org/10.20517/mtod.2022.36
About This Article
Copyright
Data & Comments
Data
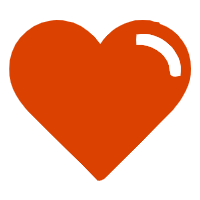

Comments
Comments must be written in English. Spam, offensive content, impersonation, and private information will not be permitted. If any comment is reported and identified as inappropriate content by OAE staff, the comment will be removed without notice. If you have any queries or need any help, please contact us at support@oaepublish.com.