The role of smart technologies in wastewater-based epidemiology
Abstract
This article explores the role of smart technologies in advancing Wastewater-based epidemiology (WBE) for enhanced disease surveillance. Disease surveillance is crucial for monitoring and controlling infectious diseases, and WBE provides a complementary approach by analyzing wastewater to identify and track pathogens. During the COVID-19 pandemic, WBE has been successfully used to detect and monitor SARS-CoV-2 in various types of wastewater, providing early warning of outbreaks and identifying emerging hotspots. However, WBE faces challenges such as the need for specialized equipment and sensitive methodologies. To overcome these limitations, biosensors have been developed, offering high sensitivity, specificity, and rapid results. Electrochemical biosensors are particularly promising for WBE due to their real-time connectivity, low-cost design, and wireless data collection, despite their limitations. Integration of smart sensors into the Internet of Things (IoT) enables seamless data integration and real-time monitoring. Furthermore, the widespread use of smartphones presents an opportunity to revolutionize smart diagnostics by leveraging their features for data analysis and communication.
Keywords
INTRODUCTION
Disease surveillance is the ongoing, systematic collection, analysis, interpretation, and dissemination of health data. It is a key component of public health efforts to monitor and control infectious diseases[1]. The main goals of disease surveillance are to identify and track the spread of disease, determine the impact of disease on communities, and provide the information needed to design and implement effective prevention and control measures[1-4]. Surveillance can be conducted at various levels, including local, national, and international. It involves the collection of data from a variety of sources, such as medical records, laboratory reports, and reports from healthcare providers and public health agencies. The data are analyzed to identify trends, patterns, and risk factors for diseases, and to inform the development of public health policies and programs[2,5]. There are different types of disease surveillance, including passive surveillance, which relies on the reporting of cases by healthcare providers and laboratories, and active surveillance, which involves actively seeking out and identifying cases through systematic efforts[6,7]. Effective disease surveillance requires strong collaboration and coordination among public health agencies, healthcare providers, and other partners, as well as the use of technology to facilitate the collection, analysis, and dissemination of data. It is an essential tool for protecting public health and preventing the spread of infectious diseases[1,8].
DISEASE SURVEILLANCE THROUGH WASTEWATER
WBE is a public health field that uses the analysis of wastewater to identify and monitor infectious agents such as viruses and bacteria, and to study their occurrence and spread in a given population[7]. Prior to their use with infectious agents, WBE has been used as an innovative tool to monitor human exposure to xenobiotics and chemicals. By analyzing wastewater samples, which contain a wide range of excreted compounds, WBE can provide valuable insights into the collective chemical exposure of a population. It allows for the detection and quantification of various substances, including pharmaceuticals, illicit drugs, and environmental pollutants. WBE offers a non-intrusive and cost-effective approach to monitor trends, identify hotspots of exposure, and inform public health interventions aimed at mitigating potential risks associated with exposure to these substances[9]. The field of WBE has predominantly been developed, standardized, and orchestrated for its application in identifying and quantifying drug use within communities[10-12]. During the recent COVID-19 pandemic, numerous efforts were made to tackle the spread of SARS-CoV-2 in the population, including the implementation of WBE surveillance systems in many countries[2,13,14]. SARS-CoV-2 RNA has been detected and monitored in various types of wastewater, including municipal and hospital wastewater[3,15-18], as well as polluted surface water not connected to a sewage system[2,19,20]. Monitoring of SARS-CoV-2 in wastewater has proven to be an effective complementary tool to track the spread of the virus in communities[2]. It has been used to identify emerging hotspots, estimate the number of infected people and provide early warning of potential outbreaks[21,22]. However, it also has limitations, such as the need for specialized equipment and expertise, and the need for extremely sensitive methodologies[3,18,23].
The ultimate goal of WBE is to provide real-time, on-site data to detect infectious agents in time to provide early warning of infectious disease outbreaks. Current detection and quantification methods either have low sensitivity, such as ELISA assays, or are time-consuming and expensive, such as (RT)-qPCR[24]. To overcome these limitations, biosensors have recently been developed that have the potential to be used for wastewater monitoring with remote access to the data in real time[11,23,25]. Despite undeniable improvements in recent decades, the burden of infectious diseases and antimicrobial resistance remains high. Emerging and re-emerging infectious agents have persisted throughout the twenty-first century, and new trends in globalization and climate change point to a new era of infectious diseases[26]. Real-time monitoring of infectious agents in wastewater and receiving water needs to be further explored.
THE USE OF BIOSENSORS TO QUANTIFY PATHOGENS IN WASTEWATER
Biosensing techniques use smart affinity materials and nanomaterials that can be used to track pathogens and biomarkers in wastewater through biochemical reactions mediated by a biological receptor element[27]. They are increasingly being used for disease detection, as they offer several advantages over traditional methods[11]. One of the main advantages of biosensors for disease detection is their sensitivity and specificity. Biosensors can detect very small amounts of a substance, and they are highly selective, meaning they can distinguish between different substances with high accuracy. This allows them to detect the presence of specific disease-related biomarkers in a sample with a high degree of precision[28]. Another advantage of biosensors is their speed. Many biosensors can provide results in real time, making them useful for rapid diagnostic testing. This can be particularly useful in the early stages of an outbreak when quick action is needed to prevent the spread of a disease. Despite the promising advantages, these technologies are still in their infancy[23,29].
There are a variety of biosensors available for disease detection, including those that use enzymes, piezoelectric elements, DNA, and other biological molecules to detect the presence of specific substances[30]. Some of the biosensing types include enzyme-based sensors, which are made with proteins that catalyze chemical reactions, to detect the presence of specific substances. The enzymes are usually immobilized on a surface, such as a microelectrode or a nanoparticle, and the reaction they catalyze is used to generate a signal that can be measured[31]. Antibody-based sensors use antibodies that recognize and bind to specific substances[32]. Another technique is DNA-based sensors, which use DNA or RNA molecules. The DNA or RNA molecules are designed to specifically bind to the target substance. Electrochemical sensors use an electrode to detect the presence of specific substances. The electrode is typically coated with a material that is sensitive to the presence of the target substance, and the reaction of the target substance with the electrode is used to generate a signal that can be measured. Optical sensors use light to detect the presence of specific substances. The light may be generated by a laser or other light source, and the interaction of the light with the target substance can be measured[25,33].
Among biosensing techniques for infectious agent detection, electrochemical biosensors are the most feasible options. The advantages of electrochemical biosensors include the fact that no sample preparation is required, they can be used in a variety of matrices, enable rapid detection, boast low-cost sensor design, support multiplexable capabilities and facilitate wireless data collection[34]. Electrochemical biosensors also enable not only detection but also estimation of the amount of target analyte. Investigation and identification of highly sensitive biorecognition elements, including antibodies, aptamers and imprinted polymers, are needed. Aptamers have several advantages and are particularly promising for novel viruses for which no antibodies are known[25]. Given the typically low concentrations of viruses such as SARS-CoV-2 in wastewater[3], particularly during periods of limited community infection rates, the precision of electrochemical biosensors for detection poses a challenge. Notably, the necessity for higher viral loads for effective detection suggests that real-time monitoring might not yield further insights beyond data collected from active hospitalizations. Importantly, the critical window for SARS-CoV-2 detection precedes outbreaks, when wastewater viral levels are minimal. Given the current lack of a robust approach for substantial sample concentration, ongoing research and development are imperative to establish a method for significant sample concentration before quantification, especially for complex matrices such as wastewater with a high abundance of inhibitory substances[3,34,35].
TOWARDS SMART, DATA-DRIVEN DISEASE SURVEILLANCE
The development of smart sensors for WBE has become increasingly important in recent years. To effectively monitor and track the spread of infectious agents, these sensors must be designed with high sensitivity and specificity to avoid false-negative and false-positive results. In addition, the ability of these sensors to connect to other smart systems and end users/decision makers via the Internet is critical for seamless data integration and real-time monitoring[33]. The use of digital technologies such as machine learning, deep learning, big data analytics, artificial neural networks and artificial intelligence is critical to the success of a smart WBE. The concept of the IoT plays a key role in connecting and exchanging data between smart devices and systems, making them smarter, more reportable, controllable, and manageable. By leveraging the power of IoT, smart WBE sensors can be seamlessly integrated into Industry 4.0, Analytics 4.0 and Healthcare 4.0, paving the way for real-time connectivity, data sharing, analytics, and optimization. This integration leads to the generation of smart data, which in turn serves as a valuable resource for intelligent decision-making in disease monitoring and control[36].
The widespread adoption of smartphone technology has the potential to revolutionize the field of smart diagnostics. Smartphones are an incredibly versatile tool with a wide range of features that make them an ideal platform for healthcare applications[37,38]. They can be used as miniature computers for data analysis, imaging and communication, all essential components of smart diagnostics. In addition, the availability of high-speed wireless connectivity and data-sharing modes enables real-time communication between the smartphone and other smart devices, making it an indispensable tool for smart diagnostics[39]. It is worth noting that the high penetration of smartphones around the world - more than 90% of the world’s population using smartphones-makes them an accessible and cost-effective technology for smart diagnostics. This provides an opportunity to develop novel approaches that harness the power of smartphone technology to facilitate smart diagnostics, especially in resource-limited settings[40,41].
Biosensors to be used in WBE must meet additional criteria beyond real-time connectivity, sensitivity, and specificity. Ten criteria for diagnostic devices/tests based on the World Health Organization ASSURED criteria have been proposed, known as REASSURED (Real-time connectivity, Ease of specimen collection, Affordable, Sensitive, Specific, User-Friendly, Rapid and Robust, Environmentally friendly, Deliverable to end-users)[42]. Microfluidic and nanofluidic technologies have recently emerged as miniaturized platforms with capabilities of molecular-scale sensitivity on low-cost and rapidly fabricated devicese[43-45]. Despite their potential, these platforms have not yet been adopted in clinical diagnostics and disease surveillance[46], but they represent a promising future in disease surveillance.
DECLARATIONS
Authors’ contributionsThe author contributed solely to the article.
Availability of data and materialsNot applicable.
Financial support and sponsorshipThis work had support from the Regional Government of Castilla y León and the FEDER program (projects CLU 2017-09, UIC315, and VA266P20).
Conflicts of interestAll authors declared that there are no conflicts of interest.
Ethical approval and consent to participateNot applicable.
Consent for publicationNot applicable.
Copyright© The Author(s) 2023.
REFERENCES
1. Aborode AT, Hasan MM, Jain S, et al. Impact of poor disease surveillance system on COVID-19 response in africa: time to rethink and rebuilt. Clin Epidemiol Glob Health 2021;12:100841.
2. Gonçalves J, Torres-Franco A, Rodriguéz E, et al. Centralized and decentralized wastewater-based epidemiology to infer COVID-19 transmission-a brief review. One Health 2022;15:100405.
3. Gonçalves J, Koritnik T, Mioč V, et al. Detection of SARS-CoV-2 RNA in hospital wastewater from a low COVID-19 disease prevalence area. Sci Total Environ 2021;755:143226.
4. Wolfe MK. Invited perspective: the promise of wastewater monitoring for infectious disease surveillance. Environ Health Perspect 2022;130:51302.
5. Paquet C, Coulombier D, Kaiser R, Ciotti M. Epidemic intelligence: a new framework for strengthening disease surveillance in Europe. Eurosurveillance 2006;11:5-6.
6. Scallan E. Activities, achievements, and lessons learned during the first 10 years of the foodborne diseases active surveillance network: 1996-2005. Clin Infect Dis 2007;44:718-25.
7. Ladner JT, Grubaugh ND, Pybus OG, Andersen KG. Precision epidemiology for infectious disease control. Nat Med 2019;25:206-11.
8. Chen C, Kostakis C, Gerber JP, Tscharke BJ, Irvine RJ, White JM. Towards finding a population biomarker for wastewater epidemiology studies. Sci Total Environ 2014;487:621-8.
9. Boogaerts T, Ahmed F, Choi PM, et al. Current and future perspectives for wastewater-based epidemiology as a monitoring tool for pharmaceutical use. Sci Total Environ 2021;789:148047.
10. González-Mariño I, Baz-Lomba JA, Alygizakis NA, et al. Spatio-temporal assessment of illicit drug use at large scale: evidence from 7 years of international wastewater monitoring. Addiction 2020;115:109-20.
11. Hernández F, Castiglioni S, Covaci A, et al. Mass spectrometric strategies for the investigation of biomarkers of illicit drug use in wastewater. Mass Spectrom Rev 2018;37:258-80.
12. Zuccato E, Chiabrando C, Castiglioni S, Bagnati R, Fanelli R. Estimating community drug abuse by wastewater analysis. Environ Health Perspect 2008;116:1027-32.
13. Adelodun B, Ajibade FO, Ibrahim RG, Bakare HO, Choi KS. Snowballing transmission of COVID-19 (SARS-CoV-2) through wastewater: any sustainable preventive measures to curtail the scourge in low-income countries? Sci Total Environ 2020;742:140680.
15. Acosta N, Bautista MA, Hollman J, et al. A multicenter study investigating SARS-CoV-2 in tertiary-care hospital wastewater. viral burden correlates with increasing hospitalized cases as well as hospital-associated transmissions and outbreaks. Water Res 2021;201:117369.
16. Ahmed W, Angel N, Edson J, et al. First confirmed detection of SARS-CoV-2 in untreated wastewater in Australia: a proof of concept for the wastewater surveillance of COVID-19 in the community. Sci Total Environ 2020;728:138764.
17. Bar-Or I, Weil M, Indenbaum V, et al. Detection of SARS-CoV-2 variants by genomic analysis of wastewater samples in Israel. Sci Total Environ 2021;789:148002.
18. Davó L, Seguí R, Botija P, et al. Early detection of SARS-CoV-2 infection cases or outbreaks at nursing homes by targeted wastewater tracking. Clin Microbiol Infect 2021;27:1061-3.
19. Huraimel K, Alhosani M, Kunhabdulla S, Stietiya MH. SARS-CoV-2 in the environment: modes of transmission, early detection and potential role of pollutions. Sci Total Environ 2020;744:140946.
20. Mota CR, Bressani-Ribeiro T, Araújo JC, et al. Assessing spatial distribution of COVID-19 prevalence in Brazil using decentralised sewage monitoring. Water Res 2021;202:117388.
21. Gracia-Lor E, Rousis NI, Hernández F, Zuccato E, Castiglioni S. Wastewater-based epidemiology as a novel biomonitoring tool to evaluate human exposure to pollutants. Environ Sci Technol 2018;52:10224-6.
22. Polo D, Quintela-Baluja M, Corbishley A, et al. Making waves: wastewater-based epidemiology for COVID-19-approaches and challenges for surveillance and prediction. Water Res 2020;186:116404.
23. Mao K, Zhang H, Pan Y, Yang Z. Biosensors for wastewater-based epidemiology for monitoring public health. Water Res 2021;191:116787.
24. Corpuz MVA, Buonerba A, Vigliotta G, et al. Viruses in wastewater: occurrence, abundance and detection methods. Sci Total Environ 2020;745:140910.
25. Pilevar M, Kim KT, Lee WH. Recent advances in biosensors for detecting viruses in water and wastewater. J Hazard Mater 2021;410:124656.
26. Baker RE, Mahmud AS, Miller IF, et al. Infectious disease in an era of global change. Nat Rev Microbiol 2022;20:193-205.
27. Chen Y, Liu J, Yang Z, Wilkinson JS, Zhou X. Optical biosensors based on refractometric sensing schemes: a review. Biosens Bioelectron 2019;144:111693.
28. Bao J, Hou C, Huo D, et al. Sensitive and selective electrochemical biosensor based on ELP-OPH/BSA/TiO2NFs/AuNPs for determination of organophosphate pesticides with p-Nitrophenyl substituent. J Electrochem Soc 2017;164:G17-22.
29. Mao K, Zhang H, Yang Z. Can a paper-based device trace COVID-19 sources with wastewater-based epidemiology? Environ Sci Technol 2020;54:3733-5.
30. Turner AP. Tech.sight. biochemistry. biosensors--sense and sensitivity. Science 2000;290:1315-7.
31. Biswas P, Karn AK, Balasubramanian P, Kale PG. Biosensor for detection of dissolved chromium in potable water: a review. Biosens Bioelectron 2017;94:589-604.
32. Mahmoudi T, Pourhassan-Moghaddam M, Shirdel B, Baradaran B, Morales-Narváez E, Golmohammadi H. (Nano)tag-antibody conjugates in rapid tests. J Mater Chem B 2021;9:5414-38.
33. Mahmoudi T, Naghdi T, Morales-Narváez E, Golmohammadi H. Toward smart diagnosis of pandemic infectious diseases using wastewater-based epidemiology. Trends Analyt Chem 2022;153:116635.
34. Cesewski E, Johnson BN. Electrochemical biosensors for pathogen detection. Biosens Bioelectron 2020;159:112214.
35. Gonçalves J, Díaz I, Torres-Franco A, et al. Microbial contamination of environmental waters and wastewater: detection methods and treatment technologies. Modern approaches in waste bioremediation: environmental microbiology. Springer International Publishing; 2023. pp. 461-83.
36. Javaid M, Khan IH. Internet of things (IoT) enabled healthcare helps to take the challenges of COVID-19 Pandemic. J Oral Biol Craniofac Res 2021;11:209-14.
37. Madrid RE, Ashur Ramallo F, Barraza DE, Chaile RE. Smartphone-based biosensor devices for healthcare: technologies, trends, and adoption by end-users. Bioengineering 2022;9:101.
38. Manickam P, Mariappan SA, Murugesan SM, et al. Artificial intelligence (AI) and internet of medical things (IoMT) assisted biomedical systems for intelligent healthcare. Biosensors 2022;12:562.
39. Sahu AK, Sharma S, Raja R. Deep learning-based continuous authentication for an iot-enabled healthcare service. Comput Electr Eng 2022;99:107817.
40. Vashist SK, Luong JHT. Smartphone-based point-of-care technologies for mobile healthcare. point-of-care technologies enabling next-generation healthcare monitoring and management. Cham: Springer International Publishing; 2019. pp. 27-79.
41. Xu X, Akay A, Wei H, et al. Advances in smartphone-based point-of-care diagnostics. Proc IEEE 2015;103:236-47.
42. Land KJ, Boeras DI, Chen XS, Ramsay AR, Peeling RW. REASSURED diagnostics to inform disease control strategies, strengthen health systems and improve patient outcomes. Nat Microbiol 2019;4:46-54.
43. Lin YL, Huang YJ, Teerapanich P, Leïchlé T, Chou CF. Multiplexed immunosensing and kinetics monitoring in nanofluidic devices with highly enhanced target capture efficiency. Biomicrofluidics 2016;10:034114.
44. Mortelmans T, Kazazis D, Padeste C, Berger P, Li X, Ekinci Y. A nanofluidic device for rapid and multiplexed SARS-CoV-2 serological antibody detection. Research Square 2021;1:online ahead of print.
45. Ye X, Li Y, Wang L, Fang X, Kong J. All-in-one microfluidic nucleic acid diagnosis system for multiplex detection of sexually transmitted pathogens directly from genitourinary secretions. Talanta 2021;221:121462.
Cite This Article
Export citation file: BibTeX | RIS
OAE Style
Gonçalves J. The role of smart technologies in wastewater-based epidemiology. J Environ Expo Assess 2023;2:18. http://dx.doi.org/10.20517/jeea.2023.16
AMA Style
Gonçalves J. The role of smart technologies in wastewater-based epidemiology. Journal of Environmental Exposure Assessment. 2023; 2(4): 18. http://dx.doi.org/10.20517/jeea.2023.16
Chicago/Turabian Style
Gonçalves, José. 2023. "The role of smart technologies in wastewater-based epidemiology" Journal of Environmental Exposure Assessment. 2, no.4: 18. http://dx.doi.org/10.20517/jeea.2023.16
ACS Style
Gonçalves, J. The role of smart technologies in wastewater-based epidemiology. J. Environ. Expo. Assess. 2023, 2, 18. http://dx.doi.org/10.20517/jeea.2023.16
About This Article
Special Issue
Copyright
Data & Comments
Data
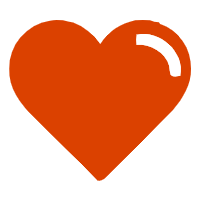

Comments
Comments must be written in English. Spam, offensive content, impersonation, and private information will not be permitted. If any comment is reported and identified as inappropriate content by OAE staff, the comment will be removed without notice. If you have any queries or need any help, please contact us at support@oaepublish.com.