Wastewater monitoring of a community COVID-19 outbreak in a Spanish municipality
Abstract
Severe acute respiratory syndrome coronavirus 2 (SARS-CoV-2) wastewater surveillance has become an increasingly important tool since the initial moments of the coronavirus disease (COVID-19) pandemic, mainly conducted at a large population scale. However, the large-sized sewersheds may not provide suitable information for monitoring localized outbreaks. After the declaration of a community COVID-19 outbreak in two neighborhoods of Castellón (Spain), SARS-CoV-2 RNA levels were monitored in wastewater samples. Moreover, genomic sequencing was performed. Thirty-three samples were collected in 2020, distributed over three points of the sewage network, two of which were close to the areas where the cases were declared. The third point was located at the inlet of the municipal wastewater treatment plant (WWTP). The samples were analyzed by RT-qPCR, using specific N1 and N2 target regions. The sum of confirmed cases, with the date of symptoms onset within the 3 weeks before each sampling day, was calculated. SARS-CoV-2 RNA was detected on most days in the two sampling points at neighborhood level, and the detection became negative when the number of cases with symptoms onset during the last 21 days in the study areas decreased to 0 or 1 case. The genomic sequencing performed for RNA from wastewater and clinical samples showed the same variant. The detection of SARS-CoV-2 and the subsequent non-detection provided the epidemiologists in charge of controlling the outbreak with useful information to confirm its closure, complementing the clinical and epidemiological data. Our findings illustrate the value of wastewater surveillance for localized outbreaks, especially in situations of low incidence of COVID-19 at the broader community level.
Keywords
INTRODUCTION
SARS-CoV-2, identified in humans and the causative agent of coronavirus disease COVID-19, has been responsible for more than 670 million cases and 6.8 million deaths as of 3 March 2023[1,2]. Although on 5 May 2023, WHO stated that “COVID-19 is now an established and ongoing health issue which no longer constitutes a public health emergency of international concern”[3], surveillance for COVID-19 remains critical to monitoring the evolution of the virus and changes in epidemiological patterns, the burden of disease on health care capacity, and the impact of vaccination and other public health interventions[4].
There is evidence of SARS-CoV-2 replication in the gastrointestinal tract, which facilitates its excretion through feces of infected individuals including asymptomatic cases. This aspect has been reported by numerous clinical studies, describing the frequency, magnitude and duration of excretion through this route[5]. Subsequently, SARS-CoV-2 enters the sewage system in municipalities via human excretions, mainly through feces. Although less frequently, its excretion through urine has been evidenced[6]. In addition, disposal of respiratory secretions (e.g., hand washing, disposable tissues, nasal rinses) may contribute to the load of the SARS-CoV-2 in sewage networks, as its RNA has been detected in washbasin and shower siphons[7].
From the initial moments of the pandemic, different studies around the globe have reported the presence of SARS-CoV-2 genetic material in wastewater samples collected from WWTP, at neighborhood scale, in samples taken from a University Campus, schools, nursery homes, and sanitary centers[8-14]. Surveillance of the virus in wastewater has been considered a tool for monitoring the pandemic evolution and even an early warning monitoring system[15,16], given its ability to cover undeclared cases, including pre-symptomatic and asymptomatic, which can more easily escape sanitary control. In this sense, different studies have reported a correlation between the genetic load of the virus in wastewater and different health outcomes related to COVID-19[17,18]. However, SARS-CoV-2 gene concentrations presented a poorer correlation with
Several countries have performed national wastewater surveillance programs[16,20,21]. Moreover, the detection of SARS-CoV-2 in wastewater has been included in the HERA incubator initiative from the European Commission to accelerate the search for variants using sequencing information retrieved from sewage analysis of SARS-CoV-2 titters[22]. In this context, genomic sequencing of wastewater samples is useful to identify mutations and viral lineages/variants of interest in circulating population.
However, despite the recognized value of wastewater analysis, there are uncertainties in the estimation of COVID-19 prevalence through WBE and more information is needed to increase the knowledge about this key issue. Aspects related to virus shedding, in-sewer transportation, sampling and storage, analysis of SARS-CoV-2 and back-estimation have a great impact on the data obtained and on the accuracy of the prevalence estimation[23]. In the particular case of fecal shedding, it is worth noticing that prolonged fecal shedding of viral RNA has been observed in patients, e.g., from 1 week to up to 5 weeks even after the respiratory swabs identified negative[6].
However, according to WHO[24], SARS-CoV-2 wastewater surveillance can be a useful tool to observe trends and changes in viral circulation at a population level, including its presence or absence, rather than to provide a true quantitative estimation of the incidence and prevalence of COVID-19 cases in the community. Thus, it can help to inform decisions on interventions and could allow for more targeted public health monitoring in a specific community.
At the end of Jun 2020, shortly after restrictions on interregional mobility in Spain were lifted and
Methods
Clinical and survey information
The COVID-19 outbreak presented in this study was notified on 27 Jun 2020, a time of very low incidence of cases in the town (two COVID-19 positive cases, PCR-based, in the previous 14 days) [Figure 1]. It was a community outbreak, with 37 identified cases, all members of a group formed by relatives and friends. According to an internal source of information, the first cases reached the town from a municipality located more than 200 km away. The information about the cases enabled the identification of two neighborhoods where most outbreak cases were concentrated, both considered to be socially vulnerable areas.
Figure 1. Evolution of daily confirmed cases of COVID-19 in municipalities continuously connected to the Castelló de la Plana WWTP (January/October 2020). In the red box, the cases in the area associated with the study outbreak are included.
The data on the total daily confirmed cases in the study area were provided by the General Sub-directorate of Epidemiology, Health Surveillance and Environmental Health of the regional government of the Valencian Community. Information on the cases affected by the outbreak was obtained from the epidemiological surveys carried out by the Castelló Public Health Centre, derived from the active search for cases, following the Recommendations of the Spanish Society of Epidemiology (SEE) on the ethical aspects of epidemiological research[27]. These surveys have socio-demographic information on each patient (age, gender, and address), clinical aspects (date of symptoms onset, date of diagnosis, asymptomatic, and hospital admission) and information on trips made and contact with cases, among others. Confirmed cases of COVID-19 were defined as symptomatic patients with positive PCR or with negative PCR but positive immunoglobulin M (IgM) determined by high-throughput serology. Confirmed cases were also those asymptomatic with positive PCR and/or positive IgM[28].
Meteorological data (daily temperature and precipitation) were obtained from the State Meteorological Agency.
Study area sampling points
The study was carried out in two neighborhoods located in the city of Castelló (174,264 inhabitants)[25], a municipality located on the east coast of Spain, where the COVID-19 outbreak was declared. One neighborhood was located in the southwest (point A) and the other in the north of the town (point B) [Figure 2], separated by a distance of 3 km. We selected one manhole sewer access sampling point as representative as possible in each neighborhood [Table 1], prioritizing proximity to the most affected homes. In fact, the sewer access points were carefully chosen through discussion with the wastewater management company on the structure of the sewer network to ensure that the effluent sampled was mainly from the neighborhood buildings of interest to the study.
Figure 2. Location of the sampling points A (39°58'49.20"N, 0° 4'17.02"O) and B (39°59'55.18"N, 0° 2'29.11"O) in the corresponding affected neighborhoods and the Wastewater Treatment Plant (WWTP) (point C) (39°59'10.37"N, 0° 0'18.12"O). Castelló de la Plana, Spain. Source: Google Earth.
Characteristics of the sewer network in relation to the selected sampling points in the neighborhoods affected by the COVID-19 outbreak studied
Sampling point A | Sampling point B | |
Distance from hotspotsa to the sampling points (m) | 20 | 118 |
Distance from sampling points to the WWTP (m) | 9,224 | 4,124 |
No. of intermediate pumps up to the WWTP | 1 | 2 |
Residence time from sampling points to WWTP (min) | 256 | 115 |
Average speed (m/s) | 0.6 | 0.6 |
In addition, the inlet of the WWTP of Castelló city was added as a third sampling point (point C). During the study period, this WWTP also provided continuous service to the neighboring municipality of Borriol (5,397 inhabitants)[25].
Sample collection
Sampling was designed considering the evidence derived from previous studies on WBE[8]. Wastewater samples were collected twice a week (Tuesday and Friday) between 7 Jul and 7 Aug 2020. In the sampling points located in the two studied neighbourhoods (A and B), 10 samples (4 h-composite) were collected in total in this study, from 8:00-12:00 am, with a total volume of 2 liters. These samples consisted of 6 subsamples spaced approximately 45 min taken along this time period. Moreover, 13 influent wastewater composite samples were also collected in the WWTP (point C) on the same days, in a time proportional sampling mode every 15 min, seven of them for 4 h (8:00-12:00 am) and another six during 24 h (24 h-composite samples) (8:00 am day X -8:00 am day X + 1). Samples were refrigerated and transported to the laboratory, where they were processed upon arrival (samples were always processed a few hours after arrival).
Analytical procedure
Water samples were subjected to a concentration process using a protocol based on adsorption and precipitation by aluminium[29]. To determine the efficiency of the entire process and validate the results, the samples were spiked with 105 PCR units of Mengovirus (MgV) vs. MC0 (CECT100000) as a process control according to ISO 10705-3: 2003, ISO15216-1: 2017. RNA extraction was performed using the Nucleospin RNA virus kit (Macherey-Nagel) following the recommended protocol, along with an initial pre-treatment step with Plant RNA Isolation Aid (Ambion, USA)[29]. Reverse transcription polymerase chain reaction (RT-qPCR) analyses were performed using the Prime Script TM One Step RT-PCR kit, validated by the US Centers for Disease Control and Prevention (2019-nCoV RUO kit), in a StepOne Plus Applied Biosystem instrument, following the manufacturer´s instructions. Specific primers and probes for nucleocapsid N1 and N2 target regions[30] and envelope (E) target genes were used to determine the presence of SARS-CoV-2 (Charité Berlin procedures)[31]. Briefly, a first step of retrotranscription at 45 °C for 10 s, followed by 40 cycles of PCR amplification and fluorescence recording (consisting of a denaturing step at 95 °C for 5 s, and annealing/elongation at 55 °C for 34 s), was set up[32]. A calibration curve was performed using the 2019-nCoV_N and 2019-nCoV_E positive controls (IDT). Cycle threshold (Ct) values were used to calculate genomic copies per liter (gc/L) in the original sample. For Mengovirus, the detection was carried out using primers and probes described by ISO 15216-1:2017. Standard curves for MgV quantifications were performed using extracted MgV genomic RNA in triplicate and with serial 10-fold dilutions. For each sampling day on which virus was detected, the average of gc/L was calculated from the two replicates analyzed at each point for each of the selected targets. Ct values were used to calculate the viral load, which was reported as gc/L, in the original sample. Ct values lower than 40 were considered positive for SARS-CoV-2, as proposed previously[33].
Genomic sequencing
The genomic sequencing was performed for RNA samples from wastewater and clinical samples were confirmed as SARS-CoV-2-positive by RT-PCR. Clinical samples corresponded to the remaining RNA extracts from naso- and oropharyngeal clinical specimens employed for diagnosis. RNA was retro-transcribed into cDNA and SARS-CoV-2 complete genome amplification was performed in two multiplex PCR, in accordance with an openly available protocol developed by the ARTIC network[34] using the V3 multiplex primers scheme[35]. Two resulting amplicon pools were combined and used for sequencing library preparation. Genomic libraries were constructed with the Nextera DNA Flex Sample Preparation kit (Illumina Inc., San Diego, CA) according to the manufacturer’s protocol with 5 cycles for indexing PCR. Whole genome sequencing was carried out in the MiSeq platform (2 × 150 cycles paired-end run; Illumina).
The sequences obtained were analyzed with a pipeline based on iVar[36], which is open-source and can be accessed at (https://gitlab.com/fisabio-ngs/sars-cov2-mapping).
Phylogenetic analysis
Additional complete SARS-CoV-2 sequences were retrieved from the GISAID database to be used as references for the analysis of outbreak-related sequences. The corresponding multiple alignment was used to derive a maximum likelihood tree with IQTREE2[37] using GTR + G as an evolutionary model, collapsing branches of zero length, and performing 1000 ultrafast bootstrap replicates[38]. The resulting tree was visualized with iTOL (https://itol.embl.de)[39].
Epidemiological study
The evolution of the cases in each location was studied. The sum of confirmed cases, with the date of symptoms onset within the 3 weeks before each sampling day, was calculated. For asymptomatic cases, the diagnosis date was considered as the onset date.
The frequency distribution of cases and the viral concentration based on each target region detected by location and dates were described. The SPSS vs. 22 software package was used for descriptive analysis.
RESULTS
The 37 cases associated with the outbreak (20 women and 17 men) were nasopharyngeal PCR positive, except one symptomatic patient with positive IgM and negative IgG. Ten cases (27%) were asymptomatic and 16 (43%) were under 15 years old. None of the cases died or was hospitalized. Of the total cases associated with the outbreak, 23 were located in the two studied neighborhoods. The characteristics of the cases in each neighborhood are described in Table 2. At sampling point A, 9 cases resided in the same building blocks, and at point B, 14 cases resided in the same dwelling.
Characteristics of the COVID-19 cases declared in the areas related to each sampling point
Sampling point A | Sampling point B | |
Associated cases | 9 | 14 |
Women/Men | 6/3 | 9/5 |
Age (median and range) | 15 (3-55) | 16.5 (4-70) |
Asymptomatic | 2 | 4 |
Evidence of diarrhoea | 1 | 0 |
Hospital admissions | 0 | 0 |
At that moment, there was a protocol in place for COVID-19 outbreak management for quarantining new COVID-19 positives and their close contact. It was mandatory that positive individuals had to quarantine for 10 days in their homes. During home isolation, it was recommended to use a face mask when sharing common spaces with other family members, and to keep a frequent routine of hand hygiene. In addition, close contacts (family or leisure partners) were also quarantined during the incubation period, and a test was carried out after 7 days if they remained asymptomatic. In the case they presented COVID-like symptoms before the 7 days, they were immediately tested by PCR (there was no antigen test available at that time). Finally, COVID-19-positive individuals were discharged when they were asymptomatic for 48 h and tested negative, which in some cases could last between 7 to 10 days.
The presence of SARS-CoV-2 in wastewater was determined and quantified by RT-qPCR. At sampling point A, SARS-CoV-2 RNA was detected for N1 and N2 regions, while at sampling point B, only N1 region was amplified. N1 was the target region for which the highest viral concentration was obtained, while target gen E was not detected in any of the samples. In all samples, the mean recovery rate of MgV control was 17.7% ± 6.39%, an acceptable value according to previous work[40].
SARS-CoV-2 RNA concentrations obtained were higher at point A, with 645,654 gc/L for N1 on 14 Jul. At sampling point B, the maximum viral concentration measured was 67,608 gc/L, recorded on the first day of the sampling collection. In general, a progressive decrease in SARS-CoV-2 RNA material was observed over time in these samples, with the exception of the samples collected on 10 Jul. SARS-CoV-2 RT-qPCR detection for all target regions remained negative when the number of cases with symptoms onset during the last 21 days decreased to 0 cases at sampling point B and to 1 case at point A.
In relation to the samples collected at the inlet of the WWTP (point C), SARS-CoV-2 RNA was not detected in the 4-hour composite samples during the study period, except for the one on 10 Jul, where a concentration close to 22,000 gc/L (N1) was found. The 24-h composite sample collected at the WWTP on the last day when SARS-CoV-2 RNA was detected at sampling points A and B (17 Jul) was also positive for N1. Later, there was a continuous detection in the 24-h composite samples of the WWTP in the last three sampling dates, when the accumulated number of cases in the city of Castelló de la Plana, no longer linked to the studied outbreak, increased considerably (60 COVID-19 positive by oropharyngeal PCRs in the last 14 days).
Quantification of genome copies for N1 and N2 in wastewater samples is shown in Table 3. The same table includes the cases with symptoms onset in each point during the 7, 14 and 21 days prior to each sampling to consider sustained excretion. Supplementary Figure 1 from Supporting Information offers an illustrative image based on these data.
Description of RNA SARS-CoV-2 detected levels (genomic copies (gc)/L) for N1 and N2 target regions in sampling points (A, B and WWTP: 4 h/24 h sampling), and the number of declared cases in each point with onset of symptoms during the 7, 14 and 21 days prior to sampling dates (from 7/7/20 to 7/8/20)
Date (dd/mm/yy) | Sampling point A (n = 9) | Sampling point B (n = 14) | WWTP (influent) | ||||||||||||||
N1 gc/L | N2 gc/L | n07 | n014 | n021 | N1 gc/L | N2 gc/L | n07 | n014 | n021 | N1 (4 h) gc/L | N1 (24 h) gc/L | N2 (24 h) gc/l | n07 | n014 | n021 | N1 (24 h) gc/L | |
7/7/20 | 446,683 | 162,181 | 1 | 8 | 8 | 67,608 | ND | 0 | 12 | 14 | ND | - | - | 8 | 31 | 34 | - |
10/7/20 | ND | ND | 0 | 8 | 8 | ND | ND | 0 | 10 | 14 | 22,387 | - | - | 5 | 26 | 35 | - |
14/7/20 | 645,654 | 147,910 | 0 | 6 | 8 | 35,481 | ND | 0 | 0a | 7 | ND | - | - | 1 | 32 | 32 | - |
17/7/20 | ND | 17,378 | 0 | 1 | 8 | 30,199 | ND | 0 | 0 | 5 | ND | 34,674 | ND | 0 | 5 | 26 | 34,674 |
21/7/20 | ND | ND | 1b | 1b | 2b | ND | ND | 0 | 0 | 0 | ND | ND | ND | 6 | 7 | 15 | ND |
24/7/20 | ND | ND | 1 | 1 | 1 | ND | ND | 0 | 0 | 0 | - | ND | ND | 11 | 11 | 16 | ND |
28/7/20 | ND | ND | 0 | 1 | 1 | ND | ND | 0 | 0 | 0 | ND | - | - | 18 | 22 | 23 | - |
31/7/20 | ND | ND | 0 | 1 | 1 | ND | ND | 0 | 0 | 0 | - | 19,055 | ND | 21 | 29 | 29 | 19,055 |
4/8/20 | ND | ND | 0 | 0 | 1 | ND | ND | 0 | 0 | 0 | ND | ND | 23,442 | 22,387 | 29 | 43 | 47 |
7/8/20 | ND | ND | 0 | 0 | 1 | ND | ND | 0 | 0 | 0 | - | - | 23,740 | 22,613 | 40 | 60 | 68 |
Six wastewater samples and 29 biological samples from human cases associated with the outbreak were available for genomic sequencing. Respectively, only 3 and 21 yielded results with enough quality to be included in the ensuing phylogenetic analysis. Consensus sequences of the two sampling sites identified from wastewater samples showed majority variant characterized by two mutations in the spike protein. Those mutations were D614G and A222V, which are markers of the 20E/EU1 variant. Sequencing from human nasopharyngeal swabs of cases belonging to the outbreak also identified 20E/EU1 as the viral variant causing the outbreak. The genetic distance between wastewater samples and human cases overlapped with the genetic distance observed exclusively among human cases [Table 4]. 20E/EU1 emerged in Northeast Spain and epidemiologically linked data is compatible with the introduction in the area from the first cases detected of the outbreak who traveled from Northeast Spain to Castellón on June 20[41]. For their phylogenetic analyses, we included 79 additional sequences derived from Spanish samples taken in Jun and Jul 2020 and belonging to the 20E (EU1) clade. The resulting phylogenetic tree is shown in Supplementary Figure 2, and it reflects the early expansion of this clade in our country. The few mutations found among the outbreak sequences were enough to split them into several groups. The two largest groups also included wastewater samples. Altogether, our data showed that wastewater samples reflected the variant associated with the outbreak, thus allowing high-resolution monitoring of community outbreaks from wastewater.
Nucleotide differences (SNPs) between complete genome sequences of clinical (COV*) and wastewater (CASTE*) samples associated with the outbreak
COV006443 | |||||||||||||||||||||||
COV006444 | 0 | ||||||||||||||||||||||
COV006445 | 0 | 0 | |||||||||||||||||||||
COV006446 | 1 | 1 | 1 | ||||||||||||||||||||
COV006447 | 2 | 2 | 2 | 1 | |||||||||||||||||||
COV006448 | 2 | 2 | 2 | 1 | 0 | ||||||||||||||||||
COV006449 | 3 | 3 | 3 | 2 | 1 | 1 | |||||||||||||||||
COV006450 | 2 | 2 | 2 | 1 | 0 | 0 | 1 | ||||||||||||||||
COV006451 | 2 | 2 | 2 | 1 | 0 | 0 | 1 | 0 | |||||||||||||||
COV006452 | 1 | 1 | 1 | 0 | 1 | 1 | 2 | 1 | 1 | ||||||||||||||
COV006455 | 1 | 1 | 1 | 0 | 1 | 1 | 2 | 1 | 1 | 0 | |||||||||||||
COV006457 | 0 | 0 | 0 | 1 | 2 | 2 | 3 | 2 | 2 | 1 | 1 | ||||||||||||
COV006459 | 2 | 2 | 2 | 1 | 0 | 0 | 1 | 0 | 0 | 1 | 1 | 2 | |||||||||||
COV006461 | 2 | 2 | 2 | 1 | 0 | 0 | 1 | 0 | 0 | 1 | 1 | 2 | 0 | ||||||||||
COV006463 | 3 | 3 | 3 | 2 | 1 | 0 | 2 | 1 | 1 | 2 | 2 | 3 | 1 | 1 | |||||||||
COV006464 | 2 | 2 | 2 | 1 | 0 | 0 | 1 | 0 | 0 | 1 | 1 | 2 | 0 | 0 | 1 | ||||||||
COV006466 | 1 | 1 | 1 | 0 | 1 | 1 | 2 | 1 | 1 | 0 | 0 | 1 | 1 | 1 | 2 | 1 | |||||||
COV006467 | 1 | 1 | 1 | 0 | 1 | 1 | 2 | 1 | 1 | 0 | 0 | 1 | 1 | 1 | 2 | 1 | 0 | ||||||
COV006468 | 3 | 3 | 3 | 2 | 1 | 0 | 2 | 1 | 1 | 2 | 2 | 3 | 1 | 1 | 0 | 1 | 2 | 2 | |||||
COV006469 | 3 | 3 | 3 | 2 | 1 | 1 | 2 | 1 | 1 | 2 | 2 | 3 | 1 | 1 | 2 | 1 | 2 | 2 | 2 | ||||
COV006471 | 2 | 2 | 2 | 1 | 0 | 0 | 1 | 0 | 0 | 1 | 1 | 2 | 0 | 0 | 1 | 0 | 1 | 1 | 1 | 1 | |||
CASTE02 | 3 | 3 | 3 | 2 | 2 | 2 | 3 | 2 | 2 | 2 | 2 | 3 | 2 | 2 | 3 | 2 | 2 | 2 | 3 | 3 | 2 | ||
CASTE03 | 3 | 3 | 3 | 2 | 2 | 2 | 3 | 2 | 2 | 2 | 2 | 3 | 2 | 2 | 2 | 2 | 2 | 2 | 2 | 3 | 2 | 2 | |
CASTE05 | 1 | 1 | 1 | 0 | 1 | 1 | 2 | 1 | 1 | 0 | 0 | 1 | 1 | 1 | 2 | 1 | 0 | 0 | 2 | 2 | 1 | 2 | 1 |
DISCUSSION
In the present study, SARS-CoV-2 RNA was detected and quantified (gc/L) in wastewater samples collected in the vicinity of two clusters of cases affected by the same outbreak. Samples were positive up to three weeks after the date of symptoms onset of those people affected. Although previous studies have studied wastewater from communities[42,43], from specific places such as college campuses[44-46], aircraft[47], and from quarantined COVID-19 patients[7], in the present work, we have performed continuous surveillance of SARS-CoV-2 RNA levels in wastewater during a community outbreak in the affected neighborhoods. Unfortunately, limited data have been published in this respect so far[48-52].
Sampling frequency was designed considering the evidence derived from previous studies[8]. According to our results, when samples are taken in the sewage network sufficiently close to the neighbourhood buildings with a COVID-19 outbreak, sampling twice a week would be sufficient to monitor SARS-CoV-2 genetic material throughout the duration of the active outbreak. The Centers for Disease Control and Prevention (CDC) recommends a minimum of three samples per week as an ideal frequency for wastewater testing. However, the CDC also emphasizes that the frequency of wastewater sampling should be tailored to the intended utilization of the data for public health purposes, as well as to reflect the prevalence of COVID-19 in the community. In fact, when wastewater surveillance aims to monitor for the presence of SARS-CoV-2 in wastewater, sampling once per week may be adequate[53].
The 4-h composite wastewater samples were collected early in the morning to cover the times with the highest probability of excretion through feces and of achieving a higher concentration[54,55]. These samples allowed the detection of SARS-CoV-2 RNA in the vicinity of those affected, despite the risk of loss of relevant pulses since 24-h sampling was not feasible for technical reasons. Nevertheless, other sampling approaches, such as grab samples or a few hours-composite samples, have also demonstrated significant epidemiological utility when the sampling timeframe is appropriately selected (typically during the morning peak)[48].
However, RNA was not detected in the 4-h samples collected in parallel from the WWTP of Castelló de la Plana, located at 9 and 4 km from sampling points A and B, respectively. The decay of SARS-CoV-2 RNA might be attributed to the circulation time through the sewer system[56] (about 4 and 2 h, respectively), the dilution of the virus residues in the water[50], and the possible effects that RNAses and conditions such as temperature, pH, organic matter or suspended solids[6] may have an impact on viral genetic material detection . The information derived from the 4-h samples collected at the WWTP raises questions about the minimum number of cases or the distance (residence time) required to enable viral RNA detection in the studied WWTP. In this sense, geolocation of cases could help to better understand the correlation between epidemiological indicators and virus detection levels in the studied WWTP. During the study period, there was no significant rainfall in the area (data not shown) that could have affected flow and, consequently, the results.
Although the number of COVID-19 cases in the vicinity of location A was lower, higher RNA concentrations were obtained at this point, and both N1 and N2 target regions were detected versus a single target N1 region at point B. This can be partly explained because sampling point A was only 20 meters away from the residence blocks of those affected, a distance 6 times shorter than from point B. In other words, the distance between the isolated building and the sampling point (residence time) might be a parameter affecting viral RNA detection of the sample, in that dilution and mixing of wastewater from multiple sources at the WWTP mask the underlying COVID-19 outbreak[50]. From the epidemiological standpoint, despite the limited sample size, both groups presented similar socio-demographic and clinical characteristics. However, it is important to highlight that the outbreak began at point B. Consequently, viral fecal shedding commenced earlier in this locale, possibly leading to an earlier cessation of viral shedding in COVID-19-infected individuals compared to those at point A, with later cases contributing to RNA detection. In fact, as mentioned in Methods, the primary cases reached point B from an outbreak declared in a municipality located 200 km away. Then, transmission happened from point B to point A. Therefore, our hypothesis is that despite point A had a small number of infected individuals, they were shedding SARS-CoV-2 into wastewater closer to the beginning of the sampling. However, it is necessary to highlight that according to the CDC, variability in the load of SARS-CoV-2 in wastewater samples is greater in the case of smaller populations[53]. This is because the SARS-CoV-2 RNA accumulated in wastewater has less opportunity to spread out, which is characterized by parameters such as inter- and intra-individual differences in the frequency of bowel movements[57], among others.
Additionally, other environmental factors may influence RNA detection. In the present study, it is striking that the SARS-CoV-2 RNA was not detected on 10 Jul, while high viral concentrations were found on the sampling days before and after, especially at sampling point A. A possible explanation of the high and unexpected differences in the genetic loads over consecutive days might be the exhaustive cleaning and disinfection measures that were undertaken in the area in the previous days (including inside the buildings), which included the application of hypochlorite and other biocides. These practices affect the stability of the genetic material[55,58], as well as the microorganisms present in the wastewater system, with the consequent impact on the degradation of SARS-CoV-2 nucleocapsid, which might promote the release of genetic material.
This study provides evidence on the relationship between the detection of SARS-CoV-2 genetic material in wastewater and the number of people affected in the vicinity of their homes at different times after the onset of symptoms. The fact that the sampling began 10 days after the outbreak was declared, when practically all the cases had started symptoms for more than 7 days, did not prevent the detection of SARS-CoV-2 RNA. The lack of SARS-CoV-2 genetic material detection in the neighbourhoods only occurred when there were hardly any cases, with started symptoms in the vicinity of the sampling points, during the 3 weeks prior to sampling. Despite the limitations due to the small size of the studied population, the results obtained would be consistent with those derived from clinical studies on SARS-CoV-2 feces excretion. Different studies have indicated prolonged fecal shedding of viral RNA for up to 5 weeks[6,59]. The results of this study showed long periods of RNA SARS-CoV-2 excretion in the cases. Specifically, Zhang et al. reported a mean period of SARS-CoV-2 feces excretion of 22 days, which is similar to what was obtained in this work[60]. Therefore, the SARS-CoV-2 RNA detection in wastewater should not necessarily imply the occurrence of active cases. In fact, it has been described that the contribution of residual viral shedding is happening from both active cases and recovered cases[61]. Therefore, the temporal pattern of excretion through feces from active cases and recovered cases might be considered in the study of the relationship between the levels of RNA SARS-CoV-2 in wastewater and its correlation with different epidemiological indicators.
Among all 4-h composite samples from the WWTP, only one was found positive for N1 (sample from 10 Jul 2020), while the detection of N1 and N2 occurred in several 24-h composite samples analyzed. It is worth noting that positive results at the end of the study period in the samples collected from the WWTP (point C) corresponded to an increase in the number of reported cases accumulated in its coverage area [Table 3], coinciding with the relaxation of restrictive measures and the beginning of the second wave, but were not related with the case study herein reported.
Wastewater samples can be used to monitor viral variants (mutations and lineages)[41]. However, until now, very little is known about the resolution to identify variants in ongoing community outbreaks. In this study, we recovered the genome sequence of the dominating viral variant during the outbreak, 20E/EU1. Variant 20E/EU1 was first identified on 20 Jun 2020 in superspreading events in northeast Spain associated with seasonal fruit workers[18,41]. Epidemiological data link the first cases of the Castelló de la Plana outbreak to travel from those high-risk areas. Our analysis shows how the imported variant propagated in the community and how this is reflected in the SARS-CoV-2 wastewater composition in the proximity of the outbreak.
Although this study reports useful data that help to understand the role of urban wastewater in monitoring a community outbreak, there have been obvious limitations that might be overcome in future studies considering the experience gained in the current pandemic. Thus, it would have been convenient to begin collecting samples earlier in the outbreak to better assess the correspondence, both in the ascending and descending phases of the outbreak curve. The fact that 4-h and 24-h WWTP composite samples could not always be available made it difficult to verify which of these samples could have been more useful and would correlate better with data obtained at points A and B located in the proximity of the cases. The lack of flow data in sampling points A and B made it unfeasible to calculate viral loads (gc/day), which is more appropriate to get conclusions in WBE studies. Therefore, it was necessary to deal with data expressed as concentrations (gc/L). In relation to wastewater genomic sequencing, it must be noticed that it comprises a mixture of various variants that are in circulation at the given time and possess a sufficient presence to be detectable. This situation can complicate the identification of the outbreak variant. In addition, consensus sequences from wastewater many times do not cover the whole genome, complicating the analysis. However, in our analysis, these limitations were less relevant. One of the samples was nearly complete and therefore the results were robust. As the wastewater samples came from local locations, the diversity of SARS-CoV-2 was low. Furthermore, this was among the first outbreaks after the major lockdown in Spain that removed almost all diversity of SARS-CoV-2[62], and the origin of the variant closely matched the origin of the outbreak and therefore there was little room for diversification. This convergence of factors enabled the precise identification of the outbreak variant through wastewater genomic analysis.
Among the potential uses of SARS-CoV-2 wastewater surveillance, the WHO highlights the generation of knowledge about COVID-19, the detection and monitoring of the virus in localities where clinical surveillance is limited. It is especially useful in high-risk environments where the response can be implemented quickly, such as large or crowded workplaces, nursing homes, or prisons[15]. Moreover, at neighborhood scale, WBE also contributes to identifying covert local outbreaks[13]. The present work illustrates the potential of WBE applied to the monitoring of SARS-CoV-2 as a complementary tool for epidemiologists to assess neighbourhood effectiveness of the containment and mitigation protocols, as somehow indicated by Pico-Tomás et al. For instance, targeted wastewater surveillance at a neighbourhood scale can help to verify the closure of a localized outbreak (cessation of viral RNA detection) without the need to perform clinical surveillance[49]. In addition, sewage monitoring might be useful to direct tailored community-level interventions, for instance, by defining the quarantine period of both symptomatic or even close contacts only considering detection of SARS-CoV-2 RNA.
CONCLUSION
Our findings highlight the usefulness of neighbourhood-scale wastewater surveillance for localized outbreaks, especially in situations of low incidence of COVID-19 at the broader community level. Wastewater surveillance at a small scale can be used as a complementary tool for the management of certain community outbreaks, where the cases can be delimited to a perimeter whose wastewater is collected or channelled by specific collectors. In this case study, WBE results provided an additional and complementary tool to epidemiologists in charge of controlling the outbreak, allowing them to evaluate the implemented measures. In addition, WBE data reported in this paper facilitated the conclusion of the outbreak surveillance with greater guarantees by verifying the maintained absence of viral circulation in the wastewater of the initially most affected areas. It is worth noticing the importance of collaboration and the coordinated work of professionals from different fields and disciplines to carry out this study, which was essential to integrate the necessary aspects for its development, implementation and draw conclusions.
DECLARATIONS
Acknowledgments
The authors acknowledge the General Directorate of Water, the General Sub-Directorate of Epidemiology, Health Surveillance and Environmental Health and the Department of Agriculture, Rural Development, Climate Emergency and Ecological Transition of the regional government of the Valencian Community. The water management company of Castelló de la Plana, Sociedad de Fomento Agrícola Castellonense, S.A. (FACSA) and the City Council. Maribel Peñalver, COVID-19 nurse at Castelló Public Health Centre. The health care workers from San Agustín and Pintor Sorolla Health Centres. Sequencing at SeqCOVID-Consortium was supported by Centro Superior de Investigaciones Científicas (CSIC) and Instituto de Salud Carlos III (ISCIII).
Authors’ contributions
Conceptualization and study design: Barberá-Riera M, Hernández F, Bellido-Blasco J, Bijlsma L
Sampling methodology definition: Bijlsma L, Celma A, Hernández F, Santateresa E, Zamorano-López N
Viral methodology design: Barneo-Muñoz M, de Llanos R
Wastewater RT-qPCR analysis: Barneo-Muñoz M, de Llanos R, Goterris-Cerisuelo R
Clinical cases diagnosis and family contacts study: Bretón-Ramos R, Aguilar-Perdiguer E
Clinical RT-PCR análisis: Gomila B
Epidemiological work: Barberá-Riera M, Bellido-Blasco J, Rebagliato M
Genomic sequencing: Comas I, González-Candelas F, Torres-Puente M
Original draft preparation: Barberá-Riera M, Rebagliato M
Writing, reviewing and editing: Barneo-Muñoz M, Bellido-Blasco J, Bijlsma L, Celma A, Comas I, de Llanos R, Hernández F, Martínez-García F, Santateresa E, Zamorano-López N
Availability of data and materials
Data are available throughout the manuscript in Tables 1-4 and Figures 1 and 2. Additionally, the evolution of SARS-CoV-2 levels and confirmed associated cases (sample points A and B) is shown in Supplementary Figure 1 and the maximum likelihood tree derived from complete genome sequences of SARS-CoV-2 associated with the outbreak of clinical and wastewater samples is shown in Supplementary Figure 2. Supplementary Figures 1 and 2 are available in the Supporting Information.
Financial support and sponsorship
Rosa de Llanos was funded through a Beatriz Galindo Fellowship of the Ministry of Education and Professional Training, Spanish Government (BGP18/00062).
Conflicts of interest
All authors declared that there are no conflicts of interest.
Ethical approval and consent to participate
Clinical data were collected as part of the outbreak investigation. The data were collected by a study team member who worked for the Public Health Centre of Castellón as a Public Health Officer and authorized the collection of anonymized clinical data as part of the outbreak investigation. All clinical data were collected using a standardized anonymized structured case report form with no patient identifiers recorded. General Sub-Directorate of Epidemiology, Health Surveillance and Environmental Health of the regional government of the Valencian Community allowed the use of epidemiological data.
Consent to Participate
Not applicable.
Consent for publication
Not applicable.
Copyright
© The Author(s) 2023.
Supplementary Materials
REFERENCES
1. John hopkins university coronavirus resource centre. https://coronavirus.jhu.edu/ [Last accessed on 21 Aug 2023].
2. Dong E, Du H, Gardner L. An interactive web-based dashboard to track COVID-19 in real time. Lancet Infect Dis 2020;20:533-4.
3. World Health Organization. Statement on the fifteenth meeting of the IHR (2005) emergency committee on the COVID-19 pandemic. Available from: https://www.who.int/news/item/05-05-2023-statement-on-the-fifteenth-meeting-of-the-international-health-regulations-(2005)-emergency-committee-regarding-the-coronavirus-disease-(covid-19)-pandemic [Last accessed on 21 Aug 2023].
4. World Health Organization. Public health surveillance for COVID-19: interim Guidance. Available from: https://www.who.int/publications-detail-redirect/WHO-2019-nCoV-SurveillanceGuidance-2022.2 [Last accessed on 21 Aug 2023].
5. Zhang Y, Cen M, Hu M, et al. Prevalence and persistent shedding of fecal SARS-CoV-2 RNA in patients with COVID-19 infection: a systematic review and meta-analysis. Clin Transl Gastroenterol 2021;12:e00343.
6. Saawarn B, Hait S. Occurrence, fate and removal of SARS-CoV-2 in wastewater: current knowledge and future perspectives. J Environ Chem Eng 2021;9:104870.
7. Döhla M, Schulte B, Wilbring G, et al. SARS-CoV-2 in environmental samples of quarantined households. Viruses 2022;14:1075.
8. de Llanos R, Cejudo-Marín R, Barneo M, et al. Monitoring the evolution of SARS-CoV-2 on a Spanish university campus through wastewater analysis: a pilot project for the reopening strategy. Sci Total Environ 2022;845:157370.
9. Bivins A, North D, Ahmad A, et al. Wastewater-based epidemiology: global collaborative to maximize contributions in the fight against COVID-19. Environ Sci Technol 2020;54:7754-7.
10. Rimoldi SG, Stefani F, Gigantiello A, et al. Presence and infectivity of SARS-CoV-2 virus in wastewaters and rivers. Sci Total Environ 2020;744:140911.
11. Guerrero-Latorre L, Ballesteros I, Villacrés-Granda I, Granda MG, Freire-Paspuel B, Ríos-Touma B. SARS-CoV-2 in river water: implications in low sanitation countries. Sci Total Environ 2020;743:140832.
12. Mohan SV, Hemalatha M, Kopperi H, Ranjith I, Kumar AK. SARS-CoV-2 in environmental perspective: occurrence, persistence, surveillance, inactivation and challenges. Chem Eng J 2021;405:126893.
13. Oh C, Zhou A, O'Brien K, et al. Application of neighborhood-scale wastewater-based epidemiology in low COVID-19 incidence situations. Sci Total Environ 2022;852:158448.
14. Shah S, Gwee SXW, Ng JQX, Lau N, Koh J, Pang J. Wastewater surveillance to infer COVID-19 transmission: a systematic review. Sci Total Environ 2022;804:150060.
15. World Health Organization. Status of Environmental surveillance for SARS-CoV-2 virus. scientific brief. Available from: https://www.who.int/news-room/commentaries/detail/status-of-environmental-surveillance-for-SARS-CoV-2-virus [Last accessed on 21 Aug 2023].
16. Prado T, Rey-Benito G, Miagostovich MP, et al. Wastewater-based epidemiology for preventing outbreaks and epidemics in Latin America - lessons from the past and a look to the future. Sci Total Environ 2023;865:161210.
17. Medema G, Been F, Heijnen L, Petterson S. Implementation of environmental surveillance for SARS-CoV-2 virus to support public health decisions: opportunities and challenges. Curr Opin Environ Sci Health 2020;17:49-71.
18. Bonanno Ferraro G, Veneri C, Mancini P, et al. A state-of-the-art scoping review on SARS-CoV-2 in sewage focusing on the potential of wastewater surveillance for the monitoring of the COVID-19 pandemic. Food Environ Virol 2022;14:315-54.
19. National Institute for Public Health and Environment (RIVM). Sewage research coronovirus. Available from: https://www.rivm.nl/coronavirus-covid-19/onderzoek/rioolwater [Last accessed on 21 Aug 2023].
20. UK centre for Ecology & Hydrology UK National Wastewater Epidemiology Surveillance Programme. Available from: https://nwesp.ceh.ac.uk/ [Last accessed on 21 Aug 2023].
21. Spanish Ministry for the Ecological Transition and the Demographic Challenge VATar COVID. Early warning COVID 19 in wastewater. Available from: https://www.miteco.gob.es/es/agua/temas/concesiones-y-autorizaciones/vertidos-de-aguas-residuales/alerta-temprana-covid19/default.aspx [Last accessed on 28 Aug 2023].
22. HERA Incubator: anticipating together the threat of COVID-19 variants. Available from: https://commission.europa.eu/system/files/2021-02/communication-hera-incubator-anticipating-threat-covid-19-variants_en.pdf [Last accessed on 28 Aug 2023].
23. Li X, Zhang S, Shi J, Luby SP, Jiang G. Uncertainties in estimating SARS-CoV-2 prevalence by wastewater-based epidemiology. Chem Eng J 2021;415:129039.
24. World Health Organization. Environmental surveillance for SARS-CoV-2 to complement public health surveillance - interim guidance. Available from: https://www.who.int/publications/i/item/WHO-HEP-ECH-WSH-2022.1 [Last accessed on 21 Aug 2023].
25. Spanish Statistics National Institute. Available from: https://www.ine.es/dyngs/INEbase/es/categoria.htm?c=Estadistica_P&cid=1254734710990 [Last accessed on 28 Aug 2023].
26. Generalitat Valenciana COVID-19 C. Valenciana. Available from: https://coronavirus.san.gva.es/es/estadisticas [Last accessed on 21 Aug 2023].
27. Dal-Ré R, Tormo MJ, Pérez G, Bolúmar F. Ethical review of epidemiologic studies: a need and a proposal. Med Clin 1998;111:587-91.
28. Spanish Ministry of Health COVID-19 early detection, surveillance and control strategy. Available from: https://www.semg.es/images/2020/Coronavirus/20200709_COVID19_Estrategia_vigilancia_y_control_e_indicadores.pdf [Last accessed on 21 Aug 2023].
29. Randazzo W, Truchado P, Cuevas-Ferrando E, Simón P, Allende A, Sánchez G. SARS-CoV-2 RNA in wastewater anticipated COVID-19 occurrence in a low prevalence area. Water Res 2020;181:115942.
30. Centers for Disease Control and Prevention (Research Use Only). Available from: https://www.cdc.gov/coronavirus/2019-ncov/lab/rt-pcr-panel-primer-probes.html [Last accessed on 21 Aug 2023].
31. Corman VM, Landt O, Kaiser M, et al. Detection of 2019 novel coronavirus (2019-nCoV) by real-time RT-PCR. Euro Surveill 2020;25:2000045.
32. CDC 2019-novel coronavirus (2019-nCoV) real-time RT-PCR diagnostic panel: instructions for use; 2023.p. 16-28. Available from: https://www.fda.gov/media/134922/download [Last accessed on 28 Aug 2023].
33. Wang J, Feng H, Zhang S, et al. SARS-CoV-2 RNA detection of hospital isolation wards hygiene monitoring during the coronavirus disease 2019 outbreak in a Chinese hospital. Int J Infect Dis 2020;94:103-6.
34. Quick J. NCoV-2019 sequencing protocol V.1. Available from: https://www.protocols.io/view/ncov-2019-sequencing-protocol-bp2l6n26rgqe/v1 [Last accessed on 28 Aug 2023].
35. Artic-network Artic-Network/Artic-Ncov2019. Available from: https://github.com/artic-network/artic-ncov2019 [Last accessed on 21 Aug 2023].
36. Grubaugh ND, Gangavarapu K, Quick J, et al. An amplicon-based sequencing framework for accurately measuring intrahost virus diversity using PrimalSeq and iVar. Genome Biol 2019;20:8.
37. Minh BQ, Schmidt HA, Chernomor O, et al. IQ-TREE 2: new models and efficient methods for phylogenetic inference in the genomic era. Mol Biol Evol 2020;37:1530-4.
38. Hoang DT, Chernomor O, von Haeseler A, Minh BQ, Vinh LS. UFBoot2: improving the ultrafast bootstrap approximation. Mol Biol Evol 2018;35:518-22.
39. Letunic I, Bork P. Interactive Tree Of Life (iTOL) v5: an online tool for phylogenetic tree display and annotation. Nucleic Acids Res 2021;49:W293-6.
40. Haramoto E, Kitajima M, Hata A, et al. A review on recent progress in the detection methods and prevalence of human enteric viruses in water. Water Res 2018;135:168-86.
41. Hodcroft EB, Zuber M, Nadeau S, et al. SeqCOVID-SPAIN consortium. Spread of a SARS-CoV-2 variant through Europe in the summer of 2020. Nature 2021;595:707-12.
42. Hamouda M, Mustafa F, Maraqa M, Rizvi T, Aly Hassan A. Wastewater surveillance for SARS-CoV-2: lessons learnt from recent studies to define future applications. Sci Total Environ 2021;759:143493.
43. Mahon J, Criado Monleon AJ, Gill LW, O'Sullivan JJ, Meijer WG. Wastewater-based epidemiology (WBE) for SARS-CoV-2 - a review focussing on the significance of the sewer network using a Dublin city catchment case study. Water Sci Technol 2022;86:1402-25.
44. Betancourt WQ, Schmitz BW, Innes GK, et al. COVID-19 containment on a college campus via wastewater-based epidemiology, targeted clinical testing and an intervention. Sci Total Environ 2021;779:146408.
45. Gibas C, Lambirth K, Mittal N, et al. Implementing building-level SARS-CoV-2 wastewater surveillance on a university campus. Sci Total Environ 2021;782:146749.
46. Harris-Lovett S, Nelson KL, Beamer P, et al. Wastewater surveillance for SARS-CoV-2 on college campuses: initial efforts, lessons learned, and research needs. Int J Environ Res Public Health 2021;18:4455.
47. Ahmed W, Bivins A, Simpson SL, et al. Wastewater surveillance demonstrates high predictive value for COVID-19 infection on board repatriation flights to Australia. Environ Int 2022;158:106938.
48. Zdenkova K, Bartackova J, Cermakova E, et al. Monitoring COVID-19 spread in Prague local neighborhoods based on the presence of SARS-CoV-2 RNA in wastewater collected throughout the sewer network. Water Res 2022;216:118343.
49. Pico-Tomàs A, Mejías-Molina C, Zammit I, et al. Surveillance of SARS-CoV-2 in sewage from buildings housing residents with different vulnerability levels. Sci Total Environ 2023;872:162116.
50. Sellers SC, Gosnell E, Bryant D, et al. Building-level wastewater surveillance of SARS-CoV-2 is associated with transmission and variant trends in a university setting. Environ Res 2022;215:114277.
51. Spurbeck RR, Minard-Smith A, Catlin L. Feasibility of neighborhood and building scale wastewater-based genomic epidemiology for pathogen surveillance. Sci Total Environ 2021;789:147829.
52. Barrios RE, Lim C, Kelley MS, Li X. SARS-CoV-2 concentrations in a wastewater collection system indicated potential COVID-19 hotspots at the zip code level. Sci Total Environ 2021;800:149480.
53. Developing a wastewater surveillance sampling strategy. Available from: https://www.cdc.gov/healthywater/surveillance/wastewater-surveillance/developing-a-wastewater-surveillance-sampling-strategy.html [Last accessed on 21 Aug 2023].
54. Heaton KW, Radvan J, Cripps H, Mountford RA, Braddon FE, Hughes AO. Defecation frequency and timing, and stool form in the general population: a prospective study. Gut 1992;33:818-24.
55. Rasero FJ, Moya Ruano LA, Rasero Del Real P, Cuberos Gómez L, Lorusso N. Associations between SARS-CoV-2 RNA concentrations in wastewater and COVID-19 rates in days after sampling in small urban areas of seville: a time series study. Sci Total Environ 2022;806:150573.
56. Hart OE, Halden RU. Computational analysis of SARS-CoV-2/COVID-19 surveillance by wastewater-based epidemiology locally and globally: feasibility, economy, opportunities and challenges. Sci Total Environ 2020;730:138875.
57. Bassotti G, Bellini M, Pucciani F, et al. Italian Constipation Study Group. An extended assessment of bowel habits in a general population. World J Gastroenterol 2004;10:713-6.
58. Brahim Belhaouari D, Wurtz N, Grimaldier C, et al. Microscopic observation of SARS-like particles in RT-qPCR SARS-CoV-2 positive sewage samples. Pathogens 2021;10:516.
59. Kaplan EH, Zulli A, Sanchez M, Peccia J. Scaling SARS-CoV-2 wastewater concentrations to population estimates of infection. Sci Rep 2022;12:3487.
60. Zhang N, Gong Y, Meng F, et al. Comparative study on virus shedding patterns in nasopharyngeal and fecal specimens of COVID-19 patients. Sci China Life Sci 2021;64:486-8.
61. Wong JCC, Tan J, Lim YX, et al. Non-intrusive wastewater surveillance for monitoring of a residential building for COVID-19 cases. Sci Total Environ 2021;786:147419.
Cite This Article
Export citation file: BibTeX | RIS
OAE Style
Barberá-Riera M, de Llanos R, Barneo-Muñoz M, Bijlsma L, Celma A, Comas I, Gomila B, González-Candelas F, Goterris-Cerisuelo R, Martínez-García F, Santateresa E, Torres-Puente M, Zamorano-López N, Bretón-Ramos R, Aguilar-Perdiguer E, Rebagliato M, Bellido-Blasco J, Hernández F. Wastewater monitoring of a community COVID-19 outbreak in a Spanish municipality. J Environ Expo Assess 2023;2:16. http://dx.doi.org/10.20517/jeea.2023.05
AMA Style
Barberá-Riera M, de Llanos R, Barneo-Muñoz M, Bijlsma L, Celma A, Comas I, Gomila B, González-Candelas F, Goterris-Cerisuelo R, Martínez-García F, Santateresa E, Torres-Puente M, Zamorano-López N, Bretón-Ramos R, Aguilar-Perdiguer E, Rebagliato M, Bellido-Blasco J, Hernández F. Wastewater monitoring of a community COVID-19 outbreak in a Spanish municipality. Journal of Environmental Exposure Assessment. 2023; 2(4): 16. http://dx.doi.org/10.20517/jeea.2023.05
Chicago/Turabian Style
Barberá-Riera, María, Rosa de Llanos, Manuela Barneo-Muñoz, Lubertus Bijlsma, Alberto Celma, Iñaki Comas, Bárbara Gomila, Fernando González-Candelas, Rafael Goterris-Cerisuelo, Fernando Martínez-García, Ernesto Santateresa, Manuela Torres-Puente, Núria Zamorano-López, Rafael Bretón-Ramos, Eduardo Aguilar-Perdiguer, Marisa Rebagliato, Juan Bellido-Blasco, Félix Hernández. 2023. "Wastewater monitoring of a community COVID-19 outbreak in a Spanish municipality" Journal of Environmental Exposure Assessment. 2, no.4: 16. http://dx.doi.org/10.20517/jeea.2023.05
ACS Style
Barberá-Riera, M.; de Llanos R.; Barneo-Muñoz M.; Bijlsma L.; Celma A.; Comas I.; Gomila B.; González-Candelas F.; Goterris-Cerisuelo R.; Martínez-García F.; Santateresa E.; Torres-Puente M.; Zamorano-López N.; Bretón-Ramos R.; Aguilar-Perdiguer E.; Rebagliato M.; Bellido-Blasco J.; Hernández F. Wastewater monitoring of a community COVID-19 outbreak in a Spanish municipality. J. Environ. Expo. Assess. 2023, 2, 16. http://dx.doi.org/10.20517/jeea.2023.05
About This Article
Special Issue
Copyright
Data & Comments
Data
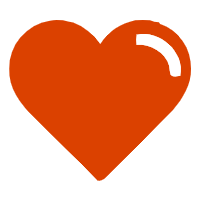

Comments
Comments must be written in English. Spam, offensive content, impersonation, and private information will not be permitted. If any comment is reported and identified as inappropriate content by OAE staff, the comment will be removed without notice. If you have any queries or need any help, please contact us at support@oaepublish.com.