High-silica KFI zeolite: highly efficient synthesis and catalysis in methanol amination reaction
Abstract
The hydrogen form of low-silica KFI zeolite, with a Si/Al ratio of 3.2 (referred to as H-KFI-3.2), has been shown to possess significant selectivity for monomethylamine (MMA) and dimethylamine (DMA) in methanol amination reactions. However, its industrial viability for MMA and DMA synthesis has been hindered by its relatively low methanol (MeOH) conversion and yield of MMA plus DMA. In this study, we synthesize high-silica KFI zeolite with an elevated framework Si/Al ratio of 5.4 (designated as KFI-5.4) using a novel K+ and 18-crown-6 complex [referred to as (K+)CCH, with a ratio of 18-crown-6 to K+ of 2.85] as an organic structure-directing agent. Control experiments have demonstrated that the presence of both K+ and OH- ions is essential for the formation of KFI-5.4 zeolite. The hydrogen form of KFI-5.4 (H-KFI-5.4) exhibits significantly enhanced MeOH conversion (95.2%) and yield (75.1%) of MMA plus DMA compared to the low-silica H-KFI-3.2 under similar reaction conditions. This represents the highest level of catalytic performance among reported small-pore zeolites to date. Furthermore, the yield and selectivity of MMA plus DMA can be further improved by modifying KFI-5.4 with an appropriate loading of Na+, which suppresses the formation of the by-product dimethyl ether. This study introduces a new high-silica KFI zeolite catalyst with exceptional MeOH conversion and yield for the production of MMA plus DMA.
Keywords
INTRODUCTION
Monomethylamine (MMA, 3.7 Å × 3.9 Å × 4.4 Å) and dimethylamine (DMA, 3.9 Å × 4.7 Å × 6.0 Å) are versatile chemicals used in producing surfactants, dyestuffs, solvents, pharmaceuticals, insecticides, and more[1-4]. Presently, the predominant method for synthesizing MMA and DMA involves the reaction of methanol (MeOH) and ammonia (NH3) using amorphous silica-alumina catalysts. However, this process yields a significant amount of thermodynamically favorable yet less desirable trimethylamine (TMA, 3.9 Å × 5.4 Å × 6.1 Å) and the by-product dimethyl ether (DME)[5]. Thus, there is a compelling need to develop shape-selective catalysts that can achieve high yields of MMA and DMA while minimizing the formation of TMA and DME[6].
Zeolite materials have emerged as promising candidates for this purpose owing to their molecular sieving capability and adjustable acid properties. For instance, large-pore MOR zeolite with a 12-membered-ring window of 6.5 Å × 7.0 Å was commercially utilized in methylamines (MAs) production during the 1980s[7]. However, a pore-size-reduction post-treatment was necessary to enhance the yield of MMA plus DMA. Subsequently, small-pore zeolites, such as KFI[8], H-rho (RHO)[9], H-PST-29 (PWN)[9], DNL-6 (RHO)[10], and SAPO-34 (CHA)[11], were discovered to exhibit superior shape selectivity for MMA and DMA due to their smaller pore sizes (3.0 Å ~ 4.5 Å), which restricted the exit of TMA. Among these small-pore zeolites, KFI zeolite stands out as one of the most promising catalysts for MMA and DMA synthesis.
KFI zeolite, with a pore size of 3.9 Å × 3.9 Å, is structured from an alternating sequence of α-cage and
In addition to direct synthesis, KFI zeolite can also be synthesized via the interzeolite transformation of zeolite Y with the assistance of K+, Na+, and Cs+, resulting in a Si/Al ratio of 3.0~4.8[19,20]. Recently, it was reported that a high Si/Al ratio of 5.0 [determined by nuclear magnetic resonance (NMR) analysis] was achieved for the KFI framework only in the presence of K+, albeit requiring a prolonged crystallization time of ten days at 150 °C or 15 days at 140 °C[21]. However, synthesizing KFI zeolite with a framework Si/Al ratio higher than 5.0 remains a challenge. According to the charge balance principle, introducing a structure-directing agent with lower charge density might increase the Si/Al ratio of the resulting zeolites[22].
Our previous study revealed that H-KFI zeolite with a Si/Al ratio of 3.2 (referred to as H-KFI-3.2) exhibited a remarkable selectivity of 94.4% for MMA plus DMA in MA products under reaction conditions of 350 °C, a weight hourly space velocity of MeOH (WHSVMeOH) = 0.813 h-1, and NH3/MeOH = 2.0[8]. However, the corresponding yield (54.7%) was low due to the relatively low MeOH conversion of 63.4%. Furthermore, it was suggested that increasing the Si/Al ratio of the zeolite could effectively enhance the yield of MMA plus DMA by improving MeOH conversion[9,23]. For instance, raising the Si/Al ratio of hydrogen form RHO zeolite (H-rho) and PWN zeolite (H-PST-29) led to increased MeOH conversion and, subsequently, higher yields of MMA plus DMA[9]. Additionally, it was demonstrated that a higher Si/Al ratio for (H)Al-RUB-41 (framework type RRO) zeolite, possessing stronger acid sites, resulted in higher MeOH conversion and yield of MMA plus DMA[23]. Therefore, increasing the Si/Al ratio of KFI zeolite is highly desirable for its further applications in MMA and DMA synthesis.
Unfortunately, increasing the Si/Al ratio of zeolite tends to decrease the selectivity of MMA plus DMA. For instance, elevating the Si/Al ratio of HMOR zeolite (MOR) from 5 to 7.5 decreased MMA plus DMA selectivity from 72.2% to 50.4%[24]. It is noteworthy that Na+ loading of mordenite (MOR) can enhance the selectivity of MMA plus DMA due to several factors, including suitable shape selectivity and acid strength, disruption of the H-bond network of methanol agglomerates and open dimers, and weaker adsorption of MMA[25]. This observation aligns with experimental results obtained by Zhang et al., where Na+ loading of HMOR increased MMA plus DMA selectivity from 61.3% to 69.9%[26]. Thus, exploring the impact of Na+ loading on the catalytic performance of zeolites is essential for developing high-performance catalysts in MMA and DMA synthesis.
In this study, we present the synthesis of KFI zeolite (KFI-5.4) with the highest framework Si/Al ratio recorded to date at 5.4 (determined via NMR analysis). This achievement was realized by employing a novel OSDA comprising an 18-crown-6 and K+ complex, with an 18-crown-6/K+ ratio of 2.85. The resulting
EXPERIMENTAL
Materials and chemicals
Potassium hydroxide (Aladdin, 99.99%) and 18-crown-6 (Energy Chemical, 99%) were employed in the preparation of (K+)CCH. Sodium aluminate (Sinopharm Chemical Reagent, Al2O3 ≥ 41.0%) and Ludox
Materials synthesis
Preparation of OSDA (K+)CCH
Firstly, 5.3 g of 18-crown-6 was completely dissolved in 3.5 g of deionized (DI) water to yield a colorless
Synthesis of KFI-5.4 zeolite
KFI-5.4 zeolite was synthesized from an initial mixture with an optimal chemical composition of 1.4 Na2O : 1.0 Al2O3 : 22.0 SiO2 : 632 H2O : 4.32 (K+)CCH : 4.4 wt.% KFI-3.8 zeolite seed (based on SiO2). In a typical synthesis of KFI-5.4 zeolite, 0.056 g of sodium aluminate and 1.2 g of prepared (K+)CCH were added to 3.0 g of DI water. The solution was then transferred into a 25 mL Teflon beaker and stirred for 1 h. Subsequently, 0.87 g of colloidal silica was added to the solution, which was then aged at ambient temperature for 24 h under continuous stirring. After adding the KFI-3.8 seed (0.015 g), the mixture was transferred into a stainless-steel autoclave and crystallized at 150 °C for five days, 140 °C for eight days, or 120 °C for 15 days. Once the crystallization was complete, the zeolite product was filtered, washed with DI water, dried at 80 °C overnight, and calcined at 550 °C for 6 h.
NH4/KFI-5.4 was obtained by subjecting the calcined zeolite sample to three cycles of ion exchange with a
Characterization
The phases of solid products were analyzed using a Rigaku D/Max 2250 X-ray diffractometer (XRD) equipped with Cu Kα radiation, scanning within a range of 2θ = 4°~40°. Morphological observations of the obtained samples were conducted using a JEOL JSM-7800F field emission scanning electron microscope (SEM). The acid property was assessed through NH3 temperature-programmed desorption (NH3-TPD) on a Micromeritics AutoChem II 2920. The local atomic environments were investigated using 13C, 27Al, and 29Si solid-state magic angle spinning NMR (MAS NMR) and 13C liquid NMR measurements performed on a Bruker Avance NEO system with a magnetic field intensity of 14.09 T. Bulk elemental contents were determined by inductively coupled plasma-optical emission spectrometry (ICP-OES) using a Thermo Scientific iCAP 7000 Series. Thermogravimetric analysis (TG) was carried out on a NETZSCH STA449F3 from 40 to 800 °C in air at a heating rate of 20 °C·min-1.
The interactions between positive ions and 18-crown-6 were investigated via liquid-phase ultraviolet-visible (UV-Vis) absorption using a UV-Vis spectrophotometer (UV-2450) with a 2 nm slit width and a wavelength range from 200 to 700 nm. The presence of 18-crown-6 related species in (K+)CCH was analyzed by mass spectrometry (MS) using an LCMS-9030 (SHIMADZU, Japan) equipped with an electrospray ionization source, operating in positive ionization mode. The interface voltage was set at
Catalytic tests for methylamine synthesis
Typically, 0.3 g of catalyst [H-KFI-5.4, Na(3%)-KFI-5.4, Na(22%)-KFI-5.4, or Na(60%)-KFI-5.4] with 40~60 mesh size was loaded into a fixed-bed reactor. The catalyst was pretreated in flowing nitrogen at 380 °C for 1 h and then cooled to the desired reaction temperature of 300 or 350 °C. Subsequently, pure NH3 gas was introduced into the reactor followed by MeOH carried into the reactor by N2 (38.0 mL·min-1), which had passed through a saturator containing liquid MeOH. The weight hourly space velocity of MeOH (WHSVMeOH) was maintained at 0.813 h-1, and the molar ratio of NH3 to MeOH was kept at 2/1. The partial pressures of MeOH, NH3, and N2 were 6.7, 13.4, and 81.2 kPa, respectively.
For comparison, the activity of the H-KFI-5.4 catalyst was also measured at a reaction temperature of
RESULTS AND DISCUSSION
Textural property of KFI-5.4 zeolite
The experimental XRD pattern of KFI-5.4 zeolite (synthesis condition: 150 °C, 5 days) is depicted in Figure 1A, demonstrating a match to the simulated pattern. Figure 1B presents the SEM image of KFI crystals, revealing irregularly shaped aggregates composed of intergrown micrometer cubic-like crystals, consistent with prior reports on KFI crystals[27]. These findings affirm the successful synthesis of pure and highly crystalline KFI zeolite.
Figure 1. (A) Simulated XRD pattern (bottom) of KFI and experimental XRD pattern (top); (B) SEM image; and (C) TG curve of KFI-5.4 zeolite (synthesis condition: 150 °C, 5 days); (D) 13C liquid NMR spectrum (bottom) of (K+)CCH and 13C solid-state MAS NMR spectrum (top) of KFI-5.4 zeolite; (E) 27Al and (F) 29Si solid-state MAS NMR spectra of KFI-5.4 zeolite. XRD: X-ray diffractometer; SEM: scanning electron microscope; TG: thermogravimetric analysis; NMR: nuclear magnetic resonance; MAS: magic angle spinning.
The TG curve of KFI-5.4 zeolite is displayed in Figure 1C. The weight loss of 8.66 wt.% observed at
Furthermore, the 13C MAS NMR spectrum of KFI-5.4 zeolite [Figure 1D] reveals a signal at 69.2 ppm, attributed to the oxygen-substituted methylene carbon[30,31], consistent with the liquid 13C NMR spectrum of (K+)CCH. This confirms that the as-prepared K+ and 18-crown-6 complex is occluded in the KFI-5.4 zeolite as an OSDA.
The 27Al MAS NMR spectrum [Figure 1E] exhibits a resonance peak at 59 ppm assigned to tetrahedral aluminum, indicating the absence of extra-framework aluminum species in KFI-5.4 zeolite[32,33]. Additionally, the 29Si MAS NMR spectrum [Figure 1F] displays three major signals at around -110, -105, and -99 ppm, assigned to Si(0Al), Si(1Al), and Si(2Al), respectively[19,34]. Based on the deconvolution results of Si(nAl) species, the Si/Al ratio of KFI-5.4 zeolite is calculated to be 5.4, closely matching the value of 5.8 determined via ICP-OES analyses. These comprehensive analyses confirm the successful synthesis of zeolite KFI with a higher Si/Al ratio than previously reported.
Influence of the synthetic parameters on the KFI-5.4 zeolite crystallization
Crystallization temperature
The crystallization temperature is widely recognized as a critical factor influencing both the thermodynamics and kinetics of zeolite crystallization[35-37]. Hydrothermal treatment at different crystallization temperatures (100, 120, 140, and 160 °C) yielded varying products from the initial mixtures optimized for KFI-5.4 zeolite [Figure 2A]. At 100 °C, an amorphous product was obtained, while a mixture of KFI and ERI was acquired at 160 °C. Thus, the suitable crystallization temperature range for KFI-5.4 zeolite lies between 120~150 °C, albeit with differing crystallization periods. Pure and highly crystalline
Figure 2. (A) Influence of crystallization temperature and time on the formation of KFI-5.4 zeolite. The phases of KFI-5.4 zeolite marked with circles were obtained in this work, while the phases of the KFI-5.0 zeolites (framework Si/Al = 5.0) marked with stars were reported by the reference[21]. “Am” represents an amorphous material; (B) Crystallization curve of KFI-5.4 zeolite at 150 °C.
Chemical compositions of the initial mixture
The influence of the SiO2/Al2O3, H2O/Al2O3, and OH-/Al2O3 ratios of the initial mixture was further explored at a crystallization temperature of 150 °C. The summarized results are presented in Figure 3, with corresponding X-ray diffraction (XRD) patterns of the products shown in Supplementary Figures 3-5. Notably, the presence of KFI-3.8 zeolite seed was found to be crucial for the crystallization of KFI-5.4
Figure 3. Influence of (A) SiO2/Al2O3 and H2O/Al2O3, and (B) SiO2/Al2O3 and OH-/Al2O3 ratios in the initial mixtures on the products crystallized. The initial mixtures compositions are as follows: 1.4 Na2O : 1.0 Al2O3 : 19.6~23.4 SiO2 : 211~1,054 H2O : 2.88~5.76 OH-, where OH- was introduced by OSDA (K+)CCH. OSDA: Organic structure-directing agent.
Maintaining the H2O/Al2O3 ratio at 632 and the OH-/Al2O3 ratio at 4.32 (represented by horizontal lines in Figure 3A and B), pure KFI zeolite was obtained when the SiO2/Al2O3 ratio of the initial mixture was 20.6 or 19.6. However, the Si/Al ratio of the resultant KFI zeolite decreased to 5.1 and 4.5, respectively. Conversely, when the SiO2/Al2O3 ratio increased to 23.4, an undesirable amorphous phase formed. The optimal product, pure KFI zeolite with the highest framework Si/Al ratio of 5.4, was attained at a SiO2/Al2O3 ratio of 22.0.
Under these optimal conditions, the influence of the H2O/Al2O3 ratio of the initial mixture, ranging from 211 to 1,054, was investigated while maintaining the SiO2/Al2O3 ratio at 22.0 and the OH-/Al2O3 ratio at 4.32. As depicted by the vertical line in Figure 3A and the XRD patterns in Supplementary Figure 4, the crystallinities of the KFI zeolite products significantly decreased when the H2O/Al2O3 ratio fell below 421 or exceeded 841, forming an amorphous phase. Pure KFI-5.4 zeolite with high crystallinity was obtained at an H2O/Al2O3 ratio of 632.
Furthermore, the influence of the OH-/Al2O3 ratio of the initial mixture, varying from 2.88 to 5.76, on the crystallization of KFI-5.4 was explored while maintaining the SiO2/Al2O3 ratio at 22.0 and the H2O/Al2O3 ratio at 632, with OH- introduced by (K+)CCH. As illustrated by the vertical line in Figure 3B, an amorphous product was obtained at an OH-/Al2O3 ratio of 2.88. At an OH-/Al2O3 ratio of 3.60, KFI zeolite, along with an amorphous phase, was obtained, while a co-crystallized product of KFI and ERI zeolite formed at OH-/Al2O3 ratios of 5.04 or 5.76. Highly crystalline KFI-5.4 zeolite was formed at an OH-/Al2O3 ratio of 4.32.
New OSDA (K+)CCH
In this study, the addition of KOH to the 18-crown-6 aqueous solution led to the observation of stratification phenomenon and color change, possibly due to the interaction between K+ and crown ether. This interaction was investigated through liquid-phase UV-Vis adsorption. The UV-Vis adsorption spectra of (K+)CCH diluted with various fractions of DI water [Figure 4 and Supplementary Figure 7] revealed a distinctive band at 352 nm, attributed to the charge transfer between K+ and crown ether[41]. Notably, this band was absent in the UV-Vis spectra of pure 18-crown-6 or KOH aqueous solutions [Supplementary Figures 8 and 9], indicating the formation of a strong interaction between K+ and 18-crown-6 in the (K+)CCH complex. Furthermore, the discrepancy in XRD patterns between pure 18-crown-6 and (K+)CCH powders obtained from vacuum freeze-drying further corroborated the existence of a strong interaction between K+ and 18-crown-6 [Supplementary Figure 10].
Figure 4. UV-Vis adsorption spectra of (K+)CCH diluted with different fractions of DI water, and the inserted photographs for the
To gain further insights into the structure of (K+)CCH, we conducted MS analysis on the 18-crown-6 related species present in (K+)CCH. A peak at m/z 795.2272 was observed in the mass spectrum of (K+)CCH
To elucidate the role of (K+)CCH in directing the formation of KFI-5.4 zeolite, we replaced the as-prepared (K+)CCH with equivalent chemicals of H2O, KOH, and 18-crown-6 under identical crystallization conditions. Only KFI zeolite with low crystallinity was obtained [Supplementary Figure 12], suggesting that the stable structure of (K+)CCH plays a critical role in the formation of KFI-5.4 zeolite.
Control experiments were conducted to investigate the roles of K+ and OH- in the formation of KFI-5.4 zeolite, and the results are summarized in Figure 5. Four types of hydroxides, namely LiOH, NaOH, CsOH, and NH3·H2O (NH4OH), were added to a colorless 18-crown-6 aqueous solution (5.7 mol·L-1), with the mole of each hydroxide equal to that of KOH, respectively. After stirring in the water bath for the same duration as that of KOH, different mixtures were obtained. Photographs of these mixtures are provided in the inset of Figure 6 (i.e., 18-crown-6 aqueous solution mixed with NaOH, CsOH, or NH3·H2O) and Supplementary Figure 13A (18-crown-6 aqueous solution mixed with LiOH). Notably, LiOH could not be completely dissolved in the 18-crown-6 aqueous solution. Upon addition of NH3·H2O to the 18-crown-6 aqueous solution, a colorless mixture was formed. However, when NaOH or CsOH was added to the 18-crown-6 aqueous solution, a stratified mixture with a yellow supernatant was obtained, similar to the mixture of KOH and 18-crown-6 aqueous solution. These observations suggest that Na+ and Cs+ can interact with
Figure 5. Control experiments investigating the role of K+ and OH- in the formation of KFI-5.4 zeolite.
Figure 6. UV-Vis adsorption spectra of (Na+)CCH, (Cs+)CCH, NH3·H2O and 18-crown-6 (aq) mixture, and 18-crown-6 aqueous solution (5.7 mol·L-1), and the inserted photographs for the mixtures of 18-crown-6 (5.7 mol·L-1) aqueous solutions with NaOH, CsOH, and
The interactions between the positive ions and 18-crown-6 were further examined through liquid-phase UV-Vis adsorption. In the UV-Vis adsorption spectra [Figure 6 and Supplementary Figure 14] of the yellow supernatant of the Na+ with 18-crown-6 mixture [designated as (Na+)CCH], a band at 315 nm is observed, indicative of ligand-to-metal charge transfer[43,44], suggesting that Na+ is bound to 18-crown-6. Similarly, a band at 317 nm is observed in the UV-Vis adsorption spectra [Figure 6 and Supplementary Figure 15] of the yellow supernatant of the Cs+ with 18-crown-6 mixture [designated as (Cs+)CCH], indicating that Cs+ is also bound to 18-crown-6[45]. In contrast, the mixture of NH3·H2O and 18-crown-6 exhibits a band similar to that of the 18-crown-6 aqueous solution (228 vs. 225 nm) in the UV-Vis adsorption spectra [Figure 6 and Supplementary Figure 16], suggesting no interaction between NH4+ and 18-crown-6 in the NH3·H2O and
Subsequently, the yellow supernatant of (Na+)CCH or (Cs+)CCH, instead of (K+)CCH, was added to the initial mixture for the crystallization of KFI-5.4. After heating at 150 °C for 5 days, no KFI zeolite was obtained [Figure 5], indicating that only the 18-crown-6 complex of K+ serves as the structure-directing agent for KFI-5.4 zeolite, even though other metal ions can also form complexes with 18-crown-6.
Furthermore, the role of OH- was investigated using alternative potassium sources instead of KOH, and the results are presented in Figure 5. In this experiment, KNO3 or KCl, equimolar to KOH, was added to the
Catalytic performance
The catalytic performances of H-KFI-5.4 and other reported small-pore zeolites are summarized in Table 1. Under the conditions of 0.813 h-1 WHSVMeOH and NH3/MeOH ratio of 2, it is observed that the MMA plus DMA selectivity increases to 78.9% from 76.9%, and the MeOH conversion significantly rises to 95.2% from 49.9% with the reaction temperature raised from 300 to 350 °C, thereby greatly improving the yield for MMA plus DMA from 38.4% to 75.1%. Although H-KFI-3.2 exhibits a higher selectivity of 86.2% for MMA plus DMA, the lower MeOH conversion of 63.4% results in a lower MMA plus DMA yield of 54.7%[8]. Previous studies have indicated that increasing the Si/Al ratio of zeolite could enhance the strength of the Brønsted acid sites, thus improving the MeOH conversion and MMA plus DMA yield[23]. Based on this observation, it is reasonable to conclude that the MeOH conversion and MMA plus DMA yield of H-KFI-5.4 are higher than those of H-KFI-3.2. Furthermore, H-KFI-5.4 exhibits a superior MMA plus DMA yield compared to SAPO-34[11]. Although the MMA plus DMA yield of DNL-6 at 300 °C (63.6%) is much better than that at 260 °C (38.9%), it remains lower than that of H-KFI-5.4 at 350 °C[10]. Under similar conditions, i.e., 400 °C, 4.3 h-1 WHSVMeOH, and 1.0 NH3/MeOH, the H-KFI-5.4, H-rho, and H-PST-29 exhibit comparable MMA plus DMA yields Supplementary Figure 18[9]. These results indicate that H-KFI-5.4 possesses the top level of catalytic performance among the small-pore zeolites reported up to date, demonstrating that KFI-5.4 is a promising solid catalyst for the shape-selective synthesis of MAs.
Comparison of the catalytic performances in the shape-selective synthesis of MAs over as-synthesized high-silica KFI-5.4 zeolite in this work and previously reported small-pore zeolites
Catalyst | Si/Al | T (°C)a | MeOH conversion (%) | Selectivity (%) | (MMA + DMA) yield (%)c | Ref. | |||
MMA | DMA | TMA | (MMA + DMA)b | ||||||
H-KFI-5.4 | 5.4 | 300 | 49.9 | 35.9 | 41.0 | 10.2 | 76.9 | 38.4 | This work |
350 | 95.2 | 24.1 | 54.8 | 17.1 | 78.9 | 75.1 | |||
400 | 49.6 | 36.1 | 33.6 | 15.7 | 69.7 | 34.6 | |||
Na(3%)-KFI-5.4 | 5.4 | 350 | 97.0 | 22.7 | 54.4 | 19.7 | 77.1 | 74.8 | |
Na(22%)-KFI-5.4 | 5.4 | 350 | 95.0 | 26.0 | 56.4 | 16.6 | 82.4 | 78.3 | |
Na(60%)-KFI-5.4 | 5.4 | 350 | 72.1 | 35.6 | 51.6 | 11.4 | 87.2 | 62.9 | |
H-KFI-3.2 | 3.2 | 350 | 63.4 | 33.7 | 52.5 | 5.1 | 86.2 | 54.7 | [8] |
SAPO-34d | - | 350 | 51.1 | 34.2 | 48.9 | 10.4 | 83.1 | 42.5 | [11] |
380 | 82.0 | 26.8 | 54.7 | 14.5 | 81.5 | 66.8 | |||
DNL-6e | - | 260 | 49.7 | 42.1 | 36.2 | 4.6 | 78.3 | 38.9 | [10] |
300 | 88.3 | 26.1 | 45.9 | 12.5 | 72.0 | 63.6 | |||
H-rho | 3.9 | 400 | ~35.0 | - | - | - | - | ~31.0 | [9] |
H-PST-29 | 4.5 | 400 | ~48.0 | - | - | - | - | ~39.0 |
We further prepared Na(3%)-KFI-5.4, Na(22%)-KFI-5.4, and Na(60%)-KFI-5.4 catalysts and investigated the influence of Na+ loading on MeOH conversion and product formation. As shown in Figure 7A-C and Supplementary Figure 19 and summarized in Table 1, the extremely low Na+ loading (i.e., Na/Al ratio × 100% = 3%) has no significant influence on the MeOH conversion and the selectivity/yield of MMA plus DMA. When the Na+ loading increases to 22%, MeOH conversion remains almost unchanged, while the selectivity of MMA and DMA significantly rises, thereby enhancing the yield of MMA plus DMA. However, further increasing the Na+ loading to 60% results in an increase in MMA plus DMA selectivity but a significant reduction in MeOH conversion, ultimately reducing the yield of MMA plus DMA.
Figure 7. (A) MeOH conversion; (B) MMA plus DMA selectivity; and (C) MMA plus DMA yield over H-KFI-5.4, Na(3%)-KFI-5.4, Na(22%)-KFI-5.4, and Na(60%)-KFI-5.4 catalysts; (D) DME selectivity of H-KFI-5.4 and Na(3%)-KFI-5.4, and the increase in MA selectivity of Na(22%)-KFI-5.4 or Na(60%)-KFI-5.4 over H-KFI-5.4 in MA synthesis at 350 °C, 0.813 h-1 WHSVMeOH, and 2.0 NH3/MeOH. MMA: Monomethylamine; DMA: dimethylamine; DME: dimethyl ether; MA: methylamine; WHSV: weight hourly space velocity.
It is worth noting that DME is not formed for Na(22%)-KFI-5.4 and Na(60%)-KFI-5.4 catalysts, and the DME selectivity reduction is closely aligned with the increase in MA selectivity for Na(22%)-KFI-5.4 or Na(60%)-KFI-5.4 [Figure 7D]. This observation is consistent with previously reported findings indicating that Na+ can suppress the DME formation[25,46]. Therefore, it is inferred that the presence of Na+ alters the reaction pathway, thereby inhibiting the DME formation while promoting the generation of MAs. This phenomenon contributes to improving the yield and/or selectivity of the catalyst, explaining the similarity between the decrease in DME and the enhancement of MA selectivity in reactions catalyzed by Na(22%)-KFI-5.4 and Na(60%)-KFI-5.4. However, an excessive amount of Na+ in Na(60%)-KFI-5.4 will occupy a significant portion of the acid sites and restrict the accessibility of the KFI framework, resulting in a substantial reduction in MeOH conversion and, consequently, decreasing the yield of MMA plus DMA.
CONCLUSIONS
The low-silica H-KFI-3.2 exhibited superior MMA plus DMA selectivity but lower MeOH conversion (63.4%), resulting in a reduced MMA plus DMA yield (54.7%) in the shape-selective synthesis of MAs, thus limiting its potential application in MA synthesis. To address this limitation, KFI zeolite with a high framework Si/Al ratio of 5.4 was rapidly synthesized using a newly developed OSDA of 18-crown-6/K+ complex [(K+)CCH]. The stable structure of the (K+)CCH complex proved crucial for forming KFI-5.4 zeolite. The high-silica KFI zeolite obtained in this study exhibited top-level catalytic performance, with high MeOH conversion (95.2%) and MMA plus DMA yield (75.1%) among small-pore zeolites reported to date. This highlights KFI-5.4 as a promising solid catalyst for the shape-selective synthesis of MAs. Moreover, we discovered that a Na+ loading of 22% further enhanced the yield and selectivity of MMA plus DMA. This research presents a high-performance catalyst for MMA and DMA synthesis and offers an effective zeolite modification method to improve the yield and selectivity of MMA plus DMA in MA synthesis.
DECLARATIONS
Authors’ contributions
Conceptualization, investigation, methodology, data curation, formal analysis, writing - original draft: Wu R
Conceptualization, methodology, formal analysis, writing - review and editing: Han J
Data curation: Li J
Data curation, writing - review and editing: Li L
Investigation, formal analysis: Liu S
Conceptualization, funding acquisition, writing - review and editing: Tian P
Conceptualization, funding acquisition, resources, supervision, project administration, writing - review and editing: Yan W
Availability of data and materials
Experimental details on synthesis, characterization, and catalysis are available in the Supplementary Materials.
Financial support and sponsorship
This work was supported by the National Key Research and Development Program of China (2021YFA1500401, 2021YFA1501202, and 2022YFB3504000), the National Natural Science Foundation of China (22288101, 21991091), the 111 Project (B17020), and the Innovation Platform for Academicians of Hainan Province, China.
Conflicts of interest
All authors declared that there are no conflicts of interest.
Ethical approval and consent to participate
Not applicable.
Consent for publication
Not applicable.
Copyright
© The Author(s) 2024.
Supplementary Materials
REFERENCES
1. Kumar R, Karmilowicz MJ, Burke D, et al. Biocatalytic reductive amination from discovery to commercial manufacturing applied to abrocitinib JAK1 inhibitor. Nat Catal 2021;4:775-82.
2. Liu J, Guo C, Zhang Z, et al. Synthesis of dimethylformamide from CO2, H2 and dimethylamine over Cu/ZnO. Chem Commun 2010;46:5770-2.
3. Lammens TM, Franssen MCR, Scott EL, Sanders JPM. Synthesis of biobased N-methylpyrrolidone by one-pot cyclization and methylation of γ-aminobutyric acid. Green Chem 2010;12:1430-6.
4. Corbin DR, Schwarz S, Sonnichsen GC. Methylamines synthesis: a review. Catal Today 1997;37:71-102.
5. Bosch M, Röttger R, Eberhardt J, et al. Shaped body comprising an aluminosilicate and aluminium oxide and process for the continuous preparation of methylamines. Available from: https://ppubs.uspto.gov/dirsearch-public/print/downloadPdf/7754921. [Last accessed on 22 Apr 2024]
6. Jeon H, Shin C, Jung HJ, Hong SB. Catalytic evaluation of small-pore molecular sieves with different framework topologies for the synthesis of methylamines. Appl Catal A Gen 2006;305:70-8.
7. Ashina Y, Fujita T, Fukatsu M, Niwa K, Yagi J. Manufacture of dimethylamine using zeolite catalyst. Stud Surf Sci Catal 1986;28:779-86.
8. Wu R, Han J, Wang Y, et al. Exclusive SAPO-seeded synthesis of ZK-5 zeolite for selective synthesis of methylamines. Inorg Chem Front 2022;9:5766-73.
9. Lee H, Lee K, Shin J, Hong SB. A comparative study of methylamines synthesis over zeolites H-rho and H-PST-29. Microporous Mesoporous Mater 2020;300:110150.
10. Wu P, Yang M, Zhang W, et al. Silicoaluminophosphate molecular sieve DNL-6: synthesis with a novel template, N,N′-dimethylethylenediamine, and its catalytic application. Chin J Catal 2018;39:1511-9.
11. Qiao Y, Wu P, Xiang X, et al. SAPO-34 synthesized with n -butylamine as a template and its catalytic application in the methanol amination reaction. Chin J Catal 2017;38:574-82.
12. van Well WJM, Jänchen J, de Haan JW, van Santen RA. Adsorption of linear alkanes in the α-cages and γ-cages of H-ZK-5 and K-ZK-5. J Phys Chem B 1999;103:1841-53.
13. Barrer RM. Synthesis of a zeolitic mineral with chabazite-like sorptive properties. J Chem Soc 1948:127-32.
14. United States Patent Office. 1966. Synthetic zeolite and method for preparing the same. George T. Kerr, Trenton, N.J., assignor to Socony Mobil Oil Company, Inc., a corporation of New York. No Drawing. Filed Jan. 4, 1965, Ser. No. 423,346. 14 Claims. (Ci.260 - 242). Available from: https://ppubs.uspto.gov/dirsearch-public/print/downloadPdf/3247195. [Last accessed on 22 Apr 2024].
15. Chatelain T, Patarin J, Farré R, Pétigny O, Schulz P. Synthesis and characterization of 18-crown-6 ether-containing KFI-type zeolite. Zeolites 1996;17:328-33.
16. Remy T, Peter SA, Van Tendeloo L, et al. Adsorption and separation of CO2 on KFI zeolites: effect of cation type and Si/Al ratio on equilibrium and kinetic properties. Langmuir 2013;29:4998-5012.
17. Remy T, Gobechiya E, Danaci D, et al. Biogas upgrading through kinetic separation of carbon dioxide and methane over Rb- and Cs-ZK-5 zeolites. RSC Adv 2014;4:62511-24.
18. Ji Y, Birmingham J, Deimund MA, Brand SK, Davis ME. Steam-dealuminated, OSDA-free RHO and KFI-type zeolites as catalysts for the methanol-to-olefins reaction. Microporous Mesoporous Mater 2016;232:126-37.
19. Kim J, Cho SJ, Kim DH. Facile synthesis of KFI-type zeolite and its application to selective catalytic reduction of NOx with NH3. ACS Catal 2017;7:6070-81.
20. Lozinska MM, Bruce EL, Mattock J, et al. Understanding the anion-templated, OSDA-free, interzeolite conversion synthesis of high silica zeolite ZK-5. Chemistry 2022;28:e202201689.
21. Han S, Tang X, Wang L, et al. Potassium-directed sustainable synthesis of new high silica small-pore zeolite with KFI structure (ZJM-7) as an efficient catalyst for NH3-SCR reaction. Appl Catal B Environ 2021;281:119480.
22. Li J, Gao M, Yan W, Yu J. Regulation of the Si/Al ratios and Al distributions of zeolites and their impact on properties. Chem Sci 2023;14:1935-59.
23. Tijsebaert B, Yilmaz B, Müller U, et al. Shape-selective synthesis of methylamines over the RRO zeolite Al-RUB-41. J Catal 2011;278:246-52.
24. Veefkind VA, Gründling C, Lercher JA. Steric aspects in methylamine and dimethylether synthesis over acidic mordenites. J Mol Catal A Chem 1998;134:111-9.
25. Maeda N, Meemken F, Hungerbühler K, Baiker A. Selectivity-controlling factors in catalytic methanol amination studied by isotopically modulated excitation IR spectroscopy. ACS Catal 2013;3:219-23.
26. Zhang YZ, Xu ZL, Wang J, Ke YY. Studies on the nature of catalysts for the selective synthesis of methylamine. Stud Surf Sci Catal 1994;84:1927-33.
27. Kamimura Y, Endo A. Seed-assisted, organic structure-directing agent-free synthesis of KFI-type zeolite with enhanced micropore volume and CO2 adsorption capacity. Adsorption 2019;25:1099-113.
28. Yang Y, Meng X, Zhu L, et al. Rapid synthesis of Si-rich SSZ-13 zeolite under fluoride-free conditions. Inorg Chem 2022;61:21115-22.
29. Mi Z, Li J, Lu T, et al. Reducing the dosage of the organic structure-directing agent in the crystallization of pure silica zeolite MFI (silicalite-1) for volatile organic compounds (VOCs) adsorption. Inorg Chem Front 2021;8:3354-62.
30. Roslund MU, Tähtinen P, Niemitz M, Sjöholm R. Complete assignments of the 1H and 13C chemical shifts and JH,H coupling constants in NMR spectra of D-glucopyranose and all D-glucopyranosyl-D-glucopyranosides. Carbohydr Res 2008;343:101-12.
31. Glinel K, Sauvage JP, Oulyadi H, Huguet J. Determination of substituents distribution in carboxymethylpullulans by NMR spectroscopy. Carbohydr Res 2000;328:343-54.
32. Liang J, Fu W, Liu C, et al. Synthesis of FER zeolite using 4-(aminomethyl)pyridine as structure-directing agent. Chem Res Chin Univ 2022;38:243-9.
33. Ye Z, Kong L, Zhao Y, et al. Alkalinity-controlled zeolite nucleation and growth: ultrafast synthesis of total-morphology zeolite L mesocrystals and adsorption evaluation. Chem Synth 2022;2:20.
34. Zhang Y, Dai W, Wu G, Guan N, Li L. Catalytic hydration of aromatic alkynes to ketones over H-MFI zeolites. Chem Res Chin Univ 2022;38:173-80.
35. Bian C, Zhang C, Pan S, et al. Generalized high-temperature synthesis of zeolite catalysts with unpredictably high space-time yields (STYs). J Mater Chem A 2017;5:2613-8.
36. Mi Z, Lu T, Zhang J, Xu R, Yan W. Synthesis of pure silica zeolites. Chem Res Chin Univ 2022;38:9-17.
37. Tai W, Dai W, Wu G, Li L. A simple strategy for synthesis of b-axis-oriented MFI zeolite macro-nanosheets. Chem Synth 2023;3:38.
38. Kamimura Y, Tanahashi S, Itabashi K, et al. Crystallization behavior of zeolite Beta in OSDA-free, seed-assisted synthesis. J Phys Chem C 2011;115:744-50.
39. Zhang H, Wang B, Yan W. The structure-directing role of heterologous seeds in the synthesis of zeolite. Green Energy Environ 2024;9:792-801.
40. Li J, Rong H, Chen C, et al. Synthesis optimization of SSZ-13 zeolite membranes by dual templates for N2/NO2 Separation. Chem Res Chin Univ 2022;38:250-6.
41. Yam VWW, Li CK, Chan CL. Proof of potassium ions by luminescence signaling based on weak gold-gold interactions in dinuclear gold(I) complexes. Angew Chem Int Ed Engl 1998;37:2857-9.
42. Moliner M, Rey F, Corma A. Towards the rational design of efficient organic structure-directing agents for zeolite synthesis. Angew Chem Int Ed Engl 2013;52:13880-9.
43. Ding Y, Wang P, Tian YK, Tian YJ, Wang F. Formation of stimuli-responsive supramolecular polymeric assemblies via orthogonal metal-ligand and host-guest interactions. Chem Commun 2013;49:5951-3.
44. Georgieva I, Danchova N, Gutzov S, Trendafilova N. DFT modeling, UV-Vis and IR spectroscopic study of acetylacetone-modified zirconia sol-gel materials. J Mol Model 2012;18:2409-22.
45. Ke Q, Sun T, Cheng H, et al. Targeted synthesis of ultrastable high-silica RHO zeolite through alkali metal-crown ether interaction. Chem Asian J 2017;12:1043-7.
Cite This Article
Export citation file: BibTeX | EndNote | RIS
OAE Style
Wu R, Han J, Li J, Li L, Liu S, Tian P, Yan W. High-silica KFI zeolite: highly efficient synthesis and catalysis in methanol amination reaction. Chem Synth 2024;4:25. http://dx.doi.org/10.20517/cs.2023.58
AMA Style
Wu R, Han J, Li J, Li L, Liu S, Tian P, Yan W. High-silica KFI zeolite: highly efficient synthesis and catalysis in methanol amination reaction. Chemical Synthesis. 2024; 4(2): 25. http://dx.doi.org/10.20517/cs.2023.58
Chicago/Turabian Style
Rui Wu, Jinfeng Han, Jing Li, Lin Li, Shuang Liu, Peng Tian, Wenfu Yan. 2024. "High-silica KFI zeolite: highly efficient synthesis and catalysis in methanol amination reaction" Chemical Synthesis. 4, no.2: 25. http://dx.doi.org/10.20517/cs.2023.58
ACS Style
Wu, R.; Han J.; Li J.; Li L.; Liu S.; Tian P.; Yan W. High-silica KFI zeolite: highly efficient synthesis and catalysis in methanol amination reaction. Chem. Synth. 2024, 4, 25. http://dx.doi.org/10.20517/cs.2023.58
About This Article
Special Issue
Copyright
Data & Comments
Data
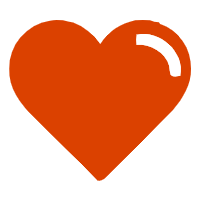
Comments
Comments must be written in English. Spam, offensive content, impersonation, and private information will not be permitted. If any comment is reported and identified as inappropriate content by OAE staff, the comment will be removed without notice. If you have any queries or need any help, please contact us at support@oaepublish.com.