Cancer-associated fibroblast cell surface markers as potential biomarkers or therapeutic targets in lung cancer
Abstract
Cancer-associated fibroblasts (CAFs) are the vital constituent of the tumor microenvironment, and in communication with other cells, they contribute to tumor progression and metastasis. Fibroblasts are the proposed origin of CAFs, which are mediated by pro-inflammatory cytokines and the recruitment of immune cells akin to wound healing. Although various studies have identified different subpopulations of CAFs in lung cancer, the heterogeneity of CAFs, particularly in lung cancer, and their potential as a therapeutic target remain largely unknown. Notwithstanding CAFs were previously thought to have predominantly tumor-promoting features, their pro- or anti-tumorigenic properties may depend on various conditions and cell origins. The absence of distinct markers to identify CAF subpopulations presents obstacles to the successful therapeutic targeting and treatment of CAFs in cancer. Human clinical and animal studies targeting CAFs have shown that targeting CAFs exacerbates the disease progression, suggesting that subpopulations of CAFs may exert opposing functions in cancer progression. Therefore, it is essential to pinpoint specific markers capable of characterizing these subpopulations and revealing their mechanisms of function. The cell-specific surface markers of CAFs will serve as an initial step in investigating precise CAF subpopulations and their role in diagnosing and targeting therapy against cancer-promoting CAF subsets in lung cancer.
Keywords
INTRODUCTION
Global Cancer Statistics 2020 (GLOBOCAN) highlighted lung cancer as the second most commonly diagnosed, trailing only behind breast cancer, and yet the leading cause of death worldwide[1]. The etiology of lung cancer is multifaceted, implicating genetic and non-genetic factors. Genetic mutations play a central role in identifying several key driver mutations. Epigenetic modifications are other contributing factors to lung cancer[2]. Notably, smoking stands out as the main risk factor for all lung cancer[3]. The heterogenicity of the cells in the tumor microenvironment predominantly manifests during disease onset and progression. Therefore, this heterogenicity is the main obstacle in the way of innovative treatment resistance, such as immune therapy and targeted therapy[4].
Different cellular, cytokine, and immune components regulate the tumor microenvironment, and cancer-associated fibroblasts (CAFs) play a seemingly ever‐increasing role in this context.
The tumor microenvironment and its components, such as fibroblasts, were first hinted at in the late 19th century, and after that, the tumor stromal component and its function were explored. Researchers discovered that the stromal cells, mainly fibroblasts, play a role in cancer cell support in the tumor microenvironment and orchestrate the broad range of activity in that environment[5]. At this point, these cells started gaining more research attention and were named CAFs.
Like other cancers, in lung cancer, CAFs constitute the major component of the stroma. In the lung, the normal stroma helps regulate the homeostasis and integrity of epithelial cells. In this regard, any secreted mediator or immune cells could alter the directional crosstalk between the stroma and epithelial cells[6].
The resting fibroblasts within the normal stroma get activated, and they differentiate into specialized cells, a process facilitated by releasing inflammatory mediators and recruiting immune cells akin to wound healing[7]. Through communication with tumor cells, CAFs contribute to many key tumor characteristics. They contribute to extracellular matrix (ECM) degradation via different pathways [e.g., matrix metalloproteinase (MMP) secretion] to facilitate tumor dissemination. At the same time, they orchestrate new ECM production to maintain the tumor structure[8]. Moreover, CAFs interact with immunosuppressive cells such as M2 macrophages, myeloid-derived suppressor cells (MDSCs), and regulatory T (Treg) cells, which further promote tumor progression and metastasis[8] [Figure 1].
Figure 1. Schematic Crosstalk of CAFs and immune cells in the tumor microenvironment of lung cancer. CAFs orchestrate the tumor microenvironment, interacting with various cell types within the tumor microenvironment of lung cancer. An example of this immune component is cancer cells, MSCs, activated fibroblasts, monocytes, macrophages, and Tregs. MSCs could differentiate into CAFs and other cell types, supporting the tumor. Activated fibroblasts are involved in remodeling the ECM and promoting tumor invasion. Monocytes can differentiate into macrophages within the tumor microenvironment. Macrophages can have pro-tumorigenic roles, promoting tumor growth and suppressing the immune response. Tregs contribute to the immunosuppressive environment within the tumor, aiding in tumor evasion from the immune system. CAFs: Cancer-associated fibroblasts; MSCs: mesenchymal stem cells, Tregs: regulatory T cells; ECM: extracellular matrix.
In addition to resting fibroblasts in tumor stroma, various origins have been suggested for CAFs in lung cancer, including tissue fibroblasts, bone marrow progenitor cells, pericytes, and epithelial cells via epithelial-mesenchymal transition (EMT)[9-12].
While the tumor microenvironment can prompt the transformation of resident fibroblasts into CAFs[13], this process is not unidirectional and can be reversed[14].
In addition to the proposed origins of CAFs in lung cancer, a recent study has identified the contribution of tumor-associated macrophages (TAMs) to the formation of CAFs in non-small cell lung cancer (NSCLC). By single-cell RNA sequencing (scRNA-seq), they identified macrophage-to-myofibroblast transition (MMT) as a mechanism by which M2 macrophages give rise to CAFs in NSCLC through a Smad3-centric gene network in experimental models and humans[15]. However, CAFs' origins might be different for different cancers.
CAFs function in tumor progression through inducing drug resistance and angiogenesis[16]. In lung cancer, the transition of fibroblasts into CAFs is mediated by the profibrotic cytokine transforming growth factor beta 1 (TGF-β1), produced from epithelial cells, much like other wound healing processes. A microarray study in lung cancer patients demonstrated that TGF-β1-related genes contributed to cell invasion, angiogenesis, and immune invasion[17] and are highly upregulated compared to normal tissues[18].
Another activated signaling pathway in lung cancer CAFs is the platelet-derived growth factor receptors (PDGF/PDGFR) signaling pathway and its downstream component, mitogen-activated protein kinase (MAPK). PDGFR regulates the proliferation of fibroblasts, modulates angiogenesis, enhances CAF‐mediated ECM remodeling, and promotes tumor invasion in lung adenocarcinoma cells[19]. Lung cancer CAFs produce inflammatory mediators such as interleukin (IL)-6, IL-8, IL-17, IL-22, tumor necrosis factor (TNF)-α, and vascular endothelial growth factor (VEGF) to support progression, invasion, and angiogenesis. The hypoxic environment of lung cancer provokes the expression of hypoxia-inducible factor 1-alpha (HIF-1α) in fibroblasts and facilitates their transformation to CAFs [Figure 2A and B][20,21].
Figure 2. The origin of CAFs and their crosstalk signaling pathways regulating tumor microenvironment. (A) CAFs in lung cancer are potentially derived from tissue-resident fibroblasts, mesenchymal stem cells, epithelial cells, and pericytes; (B) These CAFs modulate the tumor microenvironment by activating a variety of signaling pathways, including TGF-β, Wnt/β-catenin, MAPK, IL6, EGFR, JAK/STAT, and NF-κB, resulting in the orchestration of processes such as proliferation and ECM remodeling, immunosuppression, EMT, drug resistance, and angiogenesis. CAFs: Cancer-associated fibroblasts; TGF-β: transforming growth factor beta; MAPK: mitogen-activated protein kinases; IL: interleukin; EGFR: epidermal growth factor receptor; JAK/STAT: Janus kinases/signal transducers and activators of transcription; NF-κB: nuclear factor kappa-light-chain-enhancer of activated B cells; ECM: extracellular matrix, EMT: epithelial-mesenchymal transition.
As the number of CAFs increases, patients generally have a poorer prognosis and lower survival rates. Although the critical role of CAFs in the tumor microenvironment is now better understood, the heterogeneity of these populations, particularly in lung cancer, is still largely unknown.
There have been clinical cohorts and animal studies targeting CAFs that have exacerbated the disease progression[22-24], implying that distinct subpopulations of CAFs may exert opposing roles in cancer progression.
It is widely held that CAFs are a cellular state rather than a specific cell type; however, the lack of specific cell markers that would allow therapeutic targeting makes it challenging to effectively target and treat CAFs in cancer.
This review aims to offer a detailed and thorough overview of the current comprehension of the potential biomarkers of CAFs presented in Table 1 in NSCLC and explore the potential implications of these findings for treating NSCLC. In addition, the specific markers recently identified through scRNA-seq in NSCLC will be discussed.
List of potential CAF biomarkers in lung cancer
CAF marker | Bio function in tumor microenvironment | Expressing cell | Ref. |
α-SMA | Promoting tumorigenesis, ECM remodeling, proliferation, drug resistance, immune tolerance through T and NK, DC cells exhaustion, Th2 responses, M2 and Treg recruitment | Vascular, muscular cells pericytes, fibroblasts | [25-27] |
FAP | Promoting tumorigenesis, tumor invasion, ECM remodeling, immune tolerance, Th2 responses, M2 and Treg recruitment | Rarely expressed in normal tissues, epithelial tumor cells, M2 macrophages, tumor cells, tumor-promoting mesenchymal stromal cells, fibroblastic stromal cells | [27-30] |
FSP1 | ECM remodeling, tumor development, metastasis through VEGF-A and TNC-C, immune tolerance through M2 polarization recruitment | Fibroblasts, epithelial and endothelial cells | [31] |
PDPN | tumor development, immune tolerance through T cell anergy, M2 polarization, and Treg recruitment | Endothelial cells | [32,33] |
Collagen | ECM remodeling, angiogenesis, metastasis, immune suppression through M2 recruitment | Fibroblasts, tumor cells, endothelial cells | [34-36] |
Vimentin | EMT mediated metastasis, tumorigenesis, and tumor development | Fibroblasts, mesenchymal cells | [37,38] |
ITGA11 | EMT, tumor progression | Mesenchymal cells | [39-41] |
PDGFRα/β | Tumor development, immune evasion via T cell exhaustion and M2 polarization, angiogenesis | Fibroblasts, vascular smooth muscle cells, pericytes | [42,43] |
CD10/GPR77 | Inflammatory signaling, supporting CSC survival, promoting tumorigenesis, chemoresistance | Bone marrow mesenchymal stem cells, pre-B lymphocytes | [44,45] |
TNC | ECM remodeling, EMT-mediated metastasis, angiogenesis and immunomodulation, tumor development | Fibroblasts, tumor cells, endothelial cells | [46-48] |
Periostin | Tumor proliferation, metastasis | Fibroblasts, tumor cells, mesenchymal stem cells | [49,50] |
Caveolin-1 | ECM remodeling and desmoplastic reaction, cancer cell migration, and invasion | Adipocytes, endothelial cells, epithelial cells, pneumocytes, and fibroblasts | [51,52] |
ALPHA-SMOOTH MUSCLE ACTIN POSITIVE CAFS
Numerous efforts have been made to identify a reliable marker for CAFs in NSCLC. Alpha smooth muscle actin (α-SMA), a marker commonly expressed by activated fibroblasts and associated with TGF-β1 stimulation, has been extensively studied and considered a traditional CAF marker[53,54]. However, the expression of α-SMA is not exclusive to fibroblasts and can be influenced by the tumor microenvironment; regarding the identification of cell surface markers, it is worth mentioning that fibroblasts and airway smooth muscle (ASM) cells both express α-SMA, which precludes differentiation of these two cells by cell surface markers. ASM cells are proposed as proliferative and contractile cells expressing high levels of α-SMA in asthmatic patients and contribute to airway remodeling in asthma. The α-SMA positive ASM cells exhibit more proliferative and immunoreactive properties, increasing the notion that these cells are responsible for thickening the airway in asthma[55]. Furthermore, it is shown that in severe asthma, myofibroblasts contribute to airway thickening asthma by migrating and/or differentiating ASM-like cells[55]. Isolated fibroblasts from asthmatic patients after activation with TGF-β1 acquire ASM phenotype in vitro[55].
In lung cancer and α-SMA expressing CAFs, the obtained tumor tissues from 78 patients of NSCLC in different stages (I-III) have indicated that α-SMA is defined only by stromal fibroblasts and not cancer cells in NSCLC. Moreover, the clinicopathological features of NSCLC cancer correlate with α-SMA high expression. Interestingly, a higher expression of α-SMA was observed in squamous cell carcinoma (SCC) and adenocarcinoma samples. On the other hand, TGF-β1/Smad signaling is regarded as one of the CAF activation pathways. Unlike esophageal SCC, no significant correlation was detected between the levels of TGF-β1 in cancer cells and the levels of αSMA in stromal fibroblasts. Patients with high TGF-β1-expressing stromal fibroblasts had poor overall survival, indicating a potentially significant prognostic value of TGF-β1 and α-SMA within stromal fibroblasts[56]. In agreement with these findings, Alcaraz et al. reported that the immunostaining of obtained NSCLC-diagnosed tissues indicated that high expression of α-SMA in CAFs is correlated with a higher risk of recurrence and death in NSCLC patients[57]. However, the diagnostic value of α-SMA as a marker of CAFs is still controversial[58].
FIBROBLAST ACTIVATION PROTEIN 1
Fibroblast activation protein (FAP) is another potential CAF marker in various cancers[59,60]. It is involved in wound healing and is expressed during inflammatory conditions such as the tumor microenvironment but rarely on normal cells. In the tumor stroma, FAP is expressed by mesenchymal stem cells. CAFs play a significant role in angiogenesis and metastasis, and FAP is expressed in about 90% of stromal fibroblasts in different cancers, including lung cancer[61,62]. A study of 122 NSCLC patient samples revealed that tumors enriched with CAFs are more susceptible to lymphatic metastasis and angiogenesis. In addition, CAF-enriched tumors exhibited high expression levels of FAP, which was linked to poorly differentiated tumors and metastasis in SCC. Similar results were also observed in FAP knockout animal models of lung and colon cancers[63]. However, conflicting results have been reported regarding the function of FAP. In vitro studies showed that overexpression of FAP in SCC cell lines correlated with pro-tumorigenic features through the phosphoinositide 3-kinases (PI3K)/protein kinase B (Akt) and sonic hedgehog (Shh) signaling pathways[64]. An analysis of 59 NSCLC patient specimens after tumor resection indicated that high expression of FAP is a negative indicator of poor survival[65].
Conversely, a larger cohort of NSCLC patients in Kilvaer et al.’s study showed favorable results of high expression of FAP in SCC patients but not in ADC[58]. This inconsistency has also been reported in other malignancies[66]. These discrepant results may depend on the infiltration status of CD3+/CD8+ T-cells. In this regard, chimeric antigen receptor (CAR) T-cell therapy targeting tumor-promoting stromal cells shows potential for inhibiting tumor growth and enhancing endogenous CD8+ T-cell antitumor responses. The designed retroviral CAR construct specific for the mouse FAP selectively reduces FAPhi stromal cells and inhibits the growth of various murine tumors, namely lung cancer[67]. Studies have shown that increased survival of NSCLC patients with high FAP expression may be positively related to forkhead box protein 3 (FOXP3) cells, which are involved in the chemotaxis of immunosuppressive cells[68]. This finding was observed in animal models of breast and colon cancers that received an oral DNA vaccine targeting FAP, which resulted in an inhibitory effect on tumor growth and lung metastasis in a CD8+ T-cell-dependent manner[69]. Despite these findings, caution is necessary when considering the clinical implementation of therapeutic strategies aimed at targeting FAP.
FIBROBLAST-SPECIFIC PROTEIN-1
Fibroblast-specific protein-1 (FSP1), also recognized as the metastasis-associated protein S100A4, is a commonly employed marker for CAFs. The upregulation of S100A4 has been linked to unfavorable clinical outcomes in several types of cancer, including lung cancer[70-72]. A meta-analysis by Zhang et al. established the clinicopathological significance of S100A4 overexpression in the advancement and metastasis of NSCLC patients[73].
Activated fibroblasts isolated from primary tumors of NSCLC patients displayed elevated expression levels of α-SMA, FAP, and S100A4 compared to normal fibroblasts[74]. Consistent with these findings, inhibiting S100A4 with small hairpin RNA (shRNA) or Niclosamide impeded nuclear factor kappa-light-chain-enhancer of activated B cells (NF-κB) activity and, subsequently, the expression of MMP9 in various lung cancer cell lines, ultimately curtailing their invasiveness[72]. Additionally, the suppression of S100A4 sensitized A549 cells to radiation therapy[75]. Hou et al. demonstrated that overexpression of S100A4 in lung cancer cells promoted cell proliferation and tumor development via the Wnt/β-catenin pathway by inhibiting starvation-induced autophagy[76].
PODOPLANIN
Podoplanin (PDPN) is a commonly used CAF marker implicated in the invasiveness of lung cancer cells[77].
PDPN expression in poorly differentiated carcinomas of SCC has been observed, indicating its role in tumor invasion and lymph node metastasis[78]. An in vivo experiment was conducted in a lung cancer animal model by co-injecting cancer cells with two types of human fibroblasts (vascular adventitial or lung tissue-derived fibroblasts) transfected with a vector expressing PDPN. The study’s findings showed that the lung tumor formation was increased with overexpression of PDPN and was independent of the number of injected cancer cells. However, these models also demonstrated lymph node metastasis and a high risk of disease recurrence[79].
PDPN is expressed in CAFs, lymphatic endothelium, and inflammatory macrophages in the tumor microenvironment. This expression is crucial in tumor development, ECM remodeling, and immunosuppression in lung cancer[80]. Studies have shown that NSCLC patients (ADC and SCC) with PDPN-positive CAFs have a shorter overall survival[81-84]. Moreover, PDPN-positive fibroblasts recruited to the tumor microenvironment are also a poor prognostic marker for lung cancer patients. A meta-analysis conducted on tumor-infiltrating PDPN+ fibroblasts showed that the overall survival of lung cancer patients decreased significantly with an increase in the recruitment of PDPN-positive fibroblasts[85].
Although PDPN-positive CAFs are an independent marker for recurrence and short survival[23], the presence of CD204-positive TAMs along with PDPN overexpressing CAFs is another marker associated with a high risk of recurrence in lung cancer patients[81].
CD204 is a scavenger receptor highly expressed in macrophages with immunosuppressive and pro-tumorigenic properties. In lung adenocarcinoma patients, PDPN-positive CAFs have been shown to induce resistance to gefitinib, an epidermal growth factor receptor/tyrosine kinase inhibitor (EGFR-TKI) used to treat advanced NSCLC[86]. This resistance may be mediated by the secretion of hepatocyte growth factor (HGF) by PDPN-positive CAFs, which activates the mesenchymal-epithelial transition (MET) signaling pathway and promotes cancer cell survival and growth. Targeting the PDPN/MET signaling axis may be a potential therapeutic strategy to overcome resistance to EGFR-TKIs in lung adenocarcinoma patients.
INTEGRIN α11
The ECM deposition is a critical process in tumor progression and metastasis, and it is accelerated via the action of TGF-β through the transdifferentiation and activation of myofibroblasts[87,88]. These extracellular proteins, such as collagen and fibronectin, are among the differentially expressed genes in CAFs compared with normal fibroblasts.
One of the commonly overexpressed markers in stromal fibroblasts and resident mesenchymal cells in lung fibrosis and NSCLC is integrin α11 (ITGA11)[18,89,90]. ITGA11-expressing fibroblasts in NSCLC contribute to tumor progression and the EMT process, and its high expression in NSCLC patients correlates with poor prognosis. In a study using A549 cells cocultured with ITGA11-positive stromal fibroblasts, the cells had a greater potential for tumorigenicity and growth in a severe combined immunodeficient (SCID) mice model by regulating the expression of insulin-like growth factor 2 (IGF2) in the fibroblasts[89]. In line with these findings, the cell invasion and tumor growth of NSCLC tumors were hampered in α11-/- xenograft NSCLC models[91], followed by diminished stiffness of tumor stroma and downregulation of focal adhesion kinase (FAK) and protein tyrosine kinase 2 (PTK2) activity. ITGA11 is expressed in various types of CAFs in different cancers, and its expression is regulated by TGF-β1 and its downstream signaling pathway Smad in fibroblasts[41,92-94]. Since ITGA11 is expressed only in lung fibroblasts and is involved in fibroblast differentiation and recognition of collagen, it serves as a specific marker for CAFs in NSCLC[91,95-97].
VIMENTIN
The process of EMT is closely associated with the development and progression of lung cancer, with more than half of cases diagnosed with metastasis[98]. During EMT, there is a decrease in E-cadherin expression and an increase in vimentin expression. In solid tumors, it has been observed that vimentin expression is associated with invasion and poor patient survival[99-101]. In a genetically engineered mouse model with co-mutations for KRAS/liver kinase B1 (LKB1), whole-body vimentin knockout resulted in early-stage lung tumor development with less invasive foci. Both the knockout and wild-type models had CAFs positive for vimentin. Inhibiting vimentin expression in surrounding CAFs in these models and 3D culture significantly reduced invasion. This indicates that invasiveness is more closely related to vimentin expression in CAFs than in tumor cells[102]. In support of this notion, CAFs have been shown to enhance lung tumor cells’ migration and invasion ability by regulating metastasis-related genes, including vimentin and E-cadherin[103].
Nevertheless, it is essential to note that although vimentin is commonly expressed on fibroblasts of all types and used as an identifying marker, it is not a specific marker of CAFs.
PDGFRS
The PDGF/PDGFR axis is well studied and is known as an important receptor tyrosine kinase (RTK) pathway in cancer development, metastasis, angiogenesis, and stromal cell functions[104,105].
Generally, NSCLC tumors expressing PDGF and their corresponding receptors have a poorer prognosis[106]. Tejada et al. demonstrated that the PDGFR alpha signaling might contribute to the recruitment of stromal-activated fibroblasts and tumor growth in lung cancer carcinoma models. Moreover, tumor development is reduced by inhibiting the PDGFRα-mediated signaling in CAFs[107]. Consistent with the findings above, MEDI-575, a human-neutralizing monoclonal antibody explicitly targeting PDGFRα, has been shown to reduce tumor growth in NSCLC cancer models through modulation of stromal fibroblasts. These results further support the notion that the interplay between CAFs and tumor cells plays a crucial role in tumor development and progression[108].
Imatinib mesylate is a well-known tyrosine kinase inhibitor prescribed for various cancers, including chronic myelogenous leukemia, acute lymphocytic leukemia, and gastrointestinal stromal tumors[109]. PDGFR α and β are predominantly expressed on stromal fibroblasts in lung cancer, while PDGFs are expressed on cancer cell lines. In vitro and in vivo administration of imatinib inhibits the phosphorylation of PDGFRβ, Akt1/2, and extracellular signal-regulated kinase (ERK)1/2 in stromal cells and the proliferation of fibroblasts[110].
Dasatinib, another FDA-approved PDGFR inhibitor, has also been shown to affect CAFs in lung cancer in vitro at nanomolar concentrations compared to other counterparts, including imatinib, nilotinib, and sorafenib[111].
Therefore, PDGFR inhibitors or newly designed small molecules targeting PDGFRs may be a promising strategy to enhance the efficacy of conventional therapies in lung cancer.
CD200
CD200, also known as OX-2, is a cell surface glycoprotein expressed by a wide variety of cells, including tumor cells, endothelial fibroblasts, and immune cells[112]. CD200R, the responsive receptor to CD200, is primarily expressed in myeloid cells such as macrophages, neutrophils, and mast cells[113]. Furthermore, the crosstalk between cancer cells and immune cells suggests therapeutic targets in cancer therapy. This immunoregulatory axis functions in different conditions, such as lung injury.
As previously noted, CAFs that express PDPN in lung cancer patients have been found to be resistant to EGFR/TKI drugs, such as Gefitinib, and are associated with poor outcomes. Recently, CD200 has been proposed as a marker for CAFs in lung cancer, and it has been shown that high expression of CD200 in CAFs increases their sensitivity to EGFR/TKI inhibitors, such as Gefitinib, and induces cancer cell apoptosis. Furthermore, in patients with resected lung adenocarcinomas who have high expression of CD200, administration of Gefitinib has been associated with more prolonged progression-free survival[114].
Research on CD200R has also shown that its expression in stromal cells is associated with poor prognosis and recruitment of tumor-suppressive immune cells. Although an increased number of the transcription factor FoxP3+ Tregs and programmed cell death protein (PD-1)+ cells were observed in CD200R-expressing stromal cells, the expression of CD200 in NSCLC increased the levels of cytotoxic T lymphocytes (CTL), natural killer (NK) cells, and T helper (Th)1 cytokine, IL-2 and interferon-gamma (IFNγ)[115-117]. Preclinical studies have demonstrated that activation of CD200R signaling inhibits Ras, ERK, P38, and Jun N-terminal kinase (JNK) signaling pathways. Additionally, blocking CD200R abolishes cell proliferation through MAPK and Akt suppression[118]. In line with these results, analysis of dissected tumor samples from NSCLC patients demonstrated that 75% of infiltrated T cells had high levels of CD200R expression on their surface. Furthermore, the expression levels of other immune checkpoints, such as PD-1 and cytotoxic T-lymphocyte–associated antigen 4 (CTLA-4), were elevated in these T cells, indicating immune suppression and T cell exhaustion[119]. These findings support the development of a new treatment strategy targeting CD200/CD200R expressing CAFs in NSCLC.
CD10+ GPR77+
CD10 and G protein-coupled receptor 77 (GPR77) are two markers identified as pro-tumoral markers in CAFs, specifically in NSCLC and breast cancer. In NSCLC, the CD10+ GPR77+ CAF niche is characterized by the persistent secretion of IL-6 and IL-8 cytokines. The maintained activation of NF-κB signaling, as well as the subsequent phosphorylation and acetylation of p65, promote and contribute to the formation of CD10+ GPR77+ CAFs in NSCLC[44]. Furthermore, CD10+ GPR77+ CAFs provide a niche that supports cancer stem cell (CSC) survival, which promotes tumor progression and chemoresistance. Therefore, targeting this specific subset of CAFs could be a practical approach to cancer treatment.
TENASCIN-C
The glycoprotein tenascin-C (TNC) is an ECM-related component highly expressed in tumor stroma and plays a comprehensive role in cancer[120]. Mounting evidence demonstrates that the tumor ECM actively promotes malignancy[121]. TNC is an anti-adhesive molecule that mainly functions through the inhibition of fibronectin[122-124], which promotes cell invasion and EMT in cancer. TNC also has mitogenic activity and promotes the activation of Wnt and MAPK signaling pathways[125]. Angiogenesis and immunomodulation are other characteristics of TNC in cancer promotion and development. The high level of TNC expression is not limited to the stromal cells[126,127]; it is also detectable in the sera of NSCLC patients and shows a correlation with tumor size, the spread of cancer to lymph nodes, and the overall survival of patients[128,129]. TNC also suppresses T cell activity in lung cancer[130,131]. Since TNC is overexpressed in CAFs and rarely detectable in normal tissues, it could be a promising therapeutic target for lung cancer.
CAVEOLIN-1
Caveolin, an essential component of caveolae, is a family of scaffolding proteins consisting of caveolin-1, caveolin-2, and caveolin-3. While caveolin-1 is expressed in various terminally differentiated cells, its role in different malignancies is controversial[132]. It has been proposed that caveolin-1 regulates the tyrosine kinase signal transduction molecules, including the EGFR, H-RAS, and the Src family. Gastric cancer patients who express caveolin-1 have been shown to have poor progression-free survival[133], and its expression has been found to contribute to tumor progression and metastasis in lung adenocarcinoma cell lines, with a positive correlation with the tumor stage[134]. Additionally, caveolin has been shown to play a role in integrin-mediated ECM remodeling in CAFs. In solid predominant adenocarcinoma cases, the expression of caveolin-1 by CAFs was higher[52]. Furthermore, gemcitabine-based chemotherapy resistance has been associated with high expression of caveolin-1 in lung adenocarcinoma[135,136]. However, Bertino et al. demonstrated that high expression of stromal caveolin-1 improved drug response and survival rate in recipients of nab-paclitaxel in a phase II trial of NSCLC patients[137].
PERIOSTIN
Periostin belongs to a class of proteins known as matricellular proteins that are capable of binding to both the ECM and cell surface receptors. Specifically, periostin is recognized for its involvement in developing airways and repairing alveolar epithelial tissue[138]. Periostin induces cell proliferation via integrin binding and is essential in fibrotic diseases such as idiopathic pulmonary fibrosis (IPF)[139]. Periostin is not only expressed in CAFs in NSCLC, but the activated fibroblasts in IPF also express periostin. The inhibition of periostin in IPF resulted in suppression of fibrosis in bleomycin models, and the high expression of this molecule is correlated with poor prognosis of NSCLC patients[140-142].
On the other hand, periostin in A549 cells contributes to their resistance to cisplatin. This resistance occurs through activating signal transducers and activators of transcription 3 (STAT3) and Akt pathways and increasing survivin expression[143]. In addition, IPF patients are at significant risk of developing lung cancer, and these patients illustrate a more aggressive form of the disease compared with non-IPF patients. Periostin in IPF activates the tumorigenesis process of NSCLC through major inflammatory pathways such as TGF-β and Wnt/β-catenin, acting as signals that facilitate the advancement of NSCLC[144,145]. These findings indicate that periostin could be a favorable target in IPF and NSCLC[146]. In this regard, nintedanib as a tyrosine kinase inhibitor has been approved for treating lung adenocarcinoma and IPF. It also shows potential in LC by attenuating the immunosuppressive tumor microenvironment and enhancing the infiltration of cytotoxic CD8+ T cells. This mechanism may augment the responses to immune checkpoint blockade in an animal model of NSCLC[147-149].
Based on the CAF markers in NSCLC introduced above, clinical trials are currently underway to test the therapeutic efficacy of these markers in the context of NSCLC therapy [Table 2].
Clinical trials targeting CAFs in lung cancer
Trial name | Identifier | Description | Status |
A phase I/II study of RO6874281 (a FAP-targeted immunocytokine) in combination with atezolizumab in patients with advanced solid tumors | NCT03386721 | Investigates the safety and efficacy of RO6874281 combined with atezolizumab in advanced solid tumors, including lung cancer, by targeting FAP-expressing CAFs | Active, not recruiting |
A study of crenolanib in patients with advanced or metastatic solid tumors | NCT01243346 | Assesses the safety, tolerability, and preliminary efficacy of crenolanib, a PDGFR inhibitor, in targeting PDGFR-β-expressing CAFs in advanced or metastatic solid tumors, including lung cancer | Active, not recruiting |
Phase I study of simtuzumab in combination with nivolumab in advanced solid tumors | NCT02472977 | Examines the combination of simtuzumab, an anti-LOXL2 antibody, with nivolumab in advanced solid tumors, including lung cancer, to target CAFs | Completed |
Phase I/II study of galunisertib in combination with nivolumab in recurrent NSCLC | NCT02423343 | Explores the combination of galunisertib, a TGF-β receptor inhibitor, with nivolumab in recurrent NSCLC, aiming to modulate CAF activity | Completed |
A study of volociximab (M200) in combination with paclitaxel and carboplatin in subjects with NSCLC | NCT00313701 | Investigates volociximab, an anti-α5β1 integrin antibody, combined with chemotherapy in NSCLC to target integrin-expressing CAFs | Completed |
A study of vismodegib (GDC-0449) in combination with erlotinib in patients with advanced NSCLC | NCT01064622 | Examines the combination of vismodegib, a hedgehog pathway inhibitor, with erlotinib in advanced NSCLC to inhibit hedgehog pathway-activated CAFs | Completed |
A study of FAP-IL2v and anti-PD-1 in patients with advanced solid tumors | NCT03875079 | Evaluates the combination of FAP-IL2v with anti-PD-1 immunotherapy in advanced solid tumors, including lung cancer, to target CAFs and enhance the antitumor immune response | Recruiting |
SCRNA-SEQ REVEALS HETEROGENEOUS SUBPOPULATIONS OF CAFS IN LUNG CANCER
Through scRNA-seq, researchers have identified several subpopulations of CAFs, namely myofibroblast-like CAFs (myCAFs), inflammatory CAFs (iCAFs), and antigen-presenting CAFs (ApCAFs). Previously, CAFs were thought to have predominantly tumor-promoting features, but their pro- or anti-tumorigenic properties may depend on their cellular origin.
As shown in Table 3, three different approaches have been taken to define lung cancer CAF subpopulations. Kim et al. utilized scRNA-seq and pseudotime trajectory analysis to reveal the heterogeneity of lung CAFs. The THY1 marker was used to separate the active CAF subpopulation from surgically resected human lung adenocarcinoma tissues, which was associated with cancer cell invasion, migration, and poor prognosis in lung adenocarcinoma patients. Four major functionally distinct CAF branches (lineages) were identified: immunosuppressive, neoantigen-presenting, myofibroblastic, and proliferative CAFs. The study also identified specific markers for each CAF branch and confirmed the invasiveness-promoting role of ubiquitin-conjugating enzyme E2 T (UBE2T) and karyopherin subunit alpha 2 (KPNA2) in neoantigen-presenting CAFs[150]. Hu et al., to address a better understanding of CAFs in NSCLC, established patient-derived fibroblasts (PDF) from NSCLC biopsies with specific oncogenic alterations such as EGFR mutations or anaplastic large-cell lymphoma kinase (ALK) fusions. The authors identify three major functional subtypes of CAFs expressing HGF and fibroblast growth factor (FGF) that have different impacts on treatments using EGFR and ALK TKIs. A link was also found between a patient's clinical response and the functional classification of CAFs from their tumors, suggesting potential clinical value. The expression of high HGF and FGF7 in certain types of fibroblasts (type I and II) is influenced by TGF-β signaling and downstream transcription factors[151].
CAF subpopulations identified in lung cancer
CAF Subtype | Gene expression pattern | Ref. |
Cluster 1 | • COL10A1 • Epithelial-mesenchymal transition-related genes • Expression of a high range of ECM proteins and TGF-β-associated genes • Upregulation of genes regulated by HOXB2 and FOXO1 (e.g., COL1A1, COL3A1 and COL6A1) | [152] |
Cluster 2 | • COL4A1 • Highest expression of myofibroblast marker ACTA2 • High expression of myogenesis (for example, MEF2C, MYH11 or ITGA7 • NOTCH pathway-related genes • Angiogenesis-related genes • Pericytes subset expressing RGS5 (specific marker for pericytes) • Upregulation of MEF2C (myogenic transcription factor) and ELK3-regulated genes • Downregulation of FOXO1 and MSC (myogenic inhibitor) regulated genes | |
Cluster 5 | • Lower myogenesis and high mTOR signature expression | |
Cluster 6 (non-malignant fibroblasts) | • High levels of elastin • Low levels of collagens type I, III, V and VIII • Lack of collagen type VI expression | |
Cluster 7 | • Lower myogenesis and high mTOR signature expression | |
Subtype I | Function marker • HGFHigh, FGFHigh/Low, p-SMAD2Low Molecular marker • ADAMTS8, MMP3, MMP1, DLL4, … Suggested therapy: MET-plus-FGFR pathway blockade | [151] |
Subtype II | Function marker • HGFLow, FGFHigh, p-SMAD2Low Molecular marker • WNT16, TNFSF4, KRT7, MALL, … Suggested therapy: FGFR pathway blockade | |
Subtype III | Function marker • HGFLow, FGFLow, p-SMAD2High Molecular marker • RPS4Y1, EIF1AY, RPL10P9, HOXB9, … | |
Branch 1 | Immunosuppressive CAFs • IGFBP6, IFITM3, LGALS3 Apoptosis, immune system, cytokine signal | [150] |
Branch 2 | Antigen processing and presentation CAFs • UBE2T, TK1, CXCL12, KPNA2, and HMGB3 Cellular response to cytokine stimulus, antigen processing and presentation, and T cell receptor signaling pathway | |
Branch 4 | Myofibroblastic CAFs • Enriched markers of extracellular organization ECM, cytokine secretion such as CCL2 and TGF-β, cell migration | |
Branch 5 | Proliferative CAFs (the main identified population) • PRC1, AURKA Mitotic cell cycle process, cell division, cellular metabolic process |
The single-cell analysis of human lung tumors in Lambrechts et al.’s study revealed 52 stromal subtypes, including CAFs, compared to non-malignant samples. Five different types of fibroblasts were found, and non-cancerous samples were more abundant in cluster 6. The fibroblast subtypes were more enriched with ECM and distinct collagen components[152]. The component of each cluster is presented in Table 3 and Figure 3.
Figure 3. A schematic presentation of different branches/subtypes of CAFs in lung cancer CAFs. Single-cell RNAseq analysis revealed heterogeneity of CAFs in NSCLC. Kim et al. identified different branches of CAFs[150], including immunosuppressive, ApCAFs, myCAFs, and proliferative CAFs. Hu et al. introduced three subtypes with various HGF and FGF expression levels[151]. Five unique CAFs were found in Lambrechts’s investigation; one was categorized as non-malignant fibroblasts[152]. CAFs: Cancer-associated fibroblasts; NSCLC: non-small cell lung cancer; ApCAFs: antigen-presenting CAFs; myCAFs: myofibroblast-like CAFs; HGF: hepatocyte growth factor; FGF: fibroblast growth factor.
ROLE OF CAFS IN DRUG RESISTANCE
Regarding the domination of CAFs in tumor microenvironments, especially in solid tumors, drug resistance could be the attributed feature of these cells. Therefore, it is crucial to identify the specifically expressed markers on these cells for targeted therapy to overcome drug resistance in NSCLC patients. Drug resistance could be provoked by supporting the survival of cancer cells and CSCs, tumor metabolism, and ECM regulation. In this regard, CD10+ GPR77+ CAFs, by supporting the formation of stem cell niches in lung cancer patients, help maintain the stemness of tumors and chemotherapeutic resistance in these patients. Su et al.’s study indicated that neutralizing monoclonal antibodies against GPR77 could reverse these adverse features in NSCLC patients[44].
Zhao et al. demonstrated that oxidative stress induces TGF-β signaling in CAFs and boosts the synthesis of glycolytic byproducts, including L-lactate, pyruvate, and ketone bodies. These metabolites promote remodeling of the tumor microenvironment and cancer cell stemness and increase treatment resistance[153].
Additionally, it has been shown that CAF-derived midkine as a heparin-binding growth factor is elevated in patients with ovarian, lung, and oral squamous cell carcinoma (OSCC) tissue. Secretion of the midkine from CAFs promotes cancer progression and cisplatin resistance via the elevated expression of lncRNA ANRIL[154].
However, as we discussed before, not all the CAFs have the tumor suppressor feature and targeting them may exacerbate the patient’s condition. The major drug resistance mechanisms modulated by CAF in various cancers and affected pathways are presented in Table 4.
CAF modulates cancer progression and drug resistance in different cancers
CAF-mediated cancer progression | Secreted cytokines and chemokines from CAFs | Modulated pathways and signaling in cancer cells | Functions | Resistance to | Cancer types | Ref. |
Cancer cell survival | IL-6 CCL5 midkine SDF-1 IGF-1 HGF IL-8 CXCL12 CCL1 | STAT3/PI3K/Akt signaling NF-κB pathway JAK2/STAT3 pathway TGF- signaling CXCR4/Wnt/-catenin signaling TGF-β/NF-κB pathway | Proliferation Chemoresistance EMT Cancer cell viability Cell survival | Cisplatin TKI Paclitaxel 5-fluorouracil Paclitaxel Gemcitabine | Ovarian cancer OSCC Lung cancer Breast cancer Pancreatic cancer Colorectal cancer Gastric cancer | [155-163] |
CSC modulation | IL-17A TGF-β2 IL-6 IL-8 CD10 and GPR77 | Wnt/β-catenin HGF/Met signaling Hh signaling | Self-renewal | Tamoxifen 5-fluorouracil Gefitinib Cetuximab | Breast cancer Colon cancer Melanoma Lung cancer | [44,164-166] |
Cancer metabolism | HGF TIGAR | PI3K/Akt GPER/cAMP/PKA/CREB NF-κB NF-κB signaling | Cell survival Tumor progression | TKI Mitoxantrone Paclitaxel Topotecan Tamoxifen | NSCLC | [167-173] |
ECM modulation | MMPs Caveolin-1 PDPN | Integrinβ1/PI3K/Akt pathway PI3K/Akt pathways | Tumor progression Tumor invasion | Doxorubicin Tamoxifen TKI | NSCLC Breast cancer PDAC | [86,91,174-182] |
CONCLUSION
Lung cancer is a prevalent and lethal form of cancer, with a five-year survival rate of approximately 19%. CAFs have attracted attention in targeted therapy for cancer due to their involvement in cancer development, progression, and metastasis[183]. The tumor microenvironment contains various subpopulations of CAFs with either tumor-promoting or tumor-suppressing features. CAFs have been shown to contribute to cancer progression through numerous signaling pathways[184,185].
CAFs’ biological behavior, encompassing their interaction with cancer cells, the immune system, and metastasis and invasion, has been extensively studied. CAFs play a pivotal role in advancing tumorigenesis through mechanisms such as cytokine secretion, ECM modification, and EMT reprogramming. Notably, the rigidity of the ECM acts as a protective barrier shielding tumor cells from chemotherapy[186]. This ECM stiffening is notably augmented by collagen and the expression of integrins α11β1 by CAFs in NSCLC. Consequently, there has been a growing interest in using tumor organoids derived from patient’s tumor tissue as “cancer surrogates” to mimic tumor characteristics[187,188]. Nevertheless, it is imperative to acknowledge that these approaches have certain limitations, particularly in their ability to replicate the full spectrum of functions and attributes associated with CAFs, notably the absence of immune cells.
There are also unresolved questions about the relationship between CAFs at metastatic and primary sites. To illustrate, the analysis of RNA sequencing data from fibroblasts obtained from patients supports this observation. Results demonstrate that fibroblasts derived from different liver metastases of the same patient express some common markers like FAP but vary in the expression of other markers[151,189]. Nonetheless, it is worth noting that FAP may be considered a dependable marker for CAFs in the context of lung cancer. This is due to its expression in 90% of epithelial cells, and the inhibition has been shown to reduce tumor growth and increase anticancer medication uptake in tumor tissue[63,189].
These findings indicate that fibroblast variations are not solely determined by their location and genetic factors, emphasizing the significance of understanding these variations to comprehend fibroblasts’ functional heterogeneity. Age and tumor location are also influential variables for CAF marker expression[190,191].
Although S100A4 and α-smooth muscle actin are conventionally recognized as markers for lung cancer CAFs, their expression levels exhibit considerable variation among CAFs sourced from different biopsies. Interestingly, collagen type 1 α2 and αSMA expression correlated strongly with patients’ age, while PDGFRA and S100A4 expression correlated with the biopsy site[192-195].
Considering the similarities in CAFs across different cancer types, it is important to note that these markers’ expression levels and functional roles can vary depending on the type and stage of cancer[196]. Nevertheless, some of these markers were investigated as therapeutic targets in lung cancer in different clinical trials.
For instance, Dasatinib, which binds to PDGFR, has demonstrated a promising effect on CAFs in lung cancer[197].
Additionally, FAP inhibitors including a FAP-targeted immunocytokine in combination with Atezolizumab (an Anti-PD-L1 agent) (NCT03386721), the combination of a FAP-targeted interleukin-2 variant (FAP-IL2v) with anti-PD-1 immunotherapy (NCT03875079), and PDGFR inhibitors (NCT01243346) targeting PDGFR-β are currently undergoing clinical evaluation.
Nonetheless, identifying biomarkers for CAFs remains the initial and most important endeavor in this context. Numerous studies employing comparable methodologies such as RNAseq have identified various subtypes of CAFs with distinct markers and are expected to provide greater insight into these concerns and the diverse CAF populations. Identifying these biomarkers as potential therapeutic targets offers hope for treating lung cancer and other lung diseases, such as IPF.
In conclusion, due to the molecular diversity inherent to CAFs, it is imperative to conduct further investigations to comprehensively unravel the molecular mechanisms and clinical significance of CAFs in NSCLC and related respiratory conditions to develop targeted therapeutic approaches.
DECLARATIONS
Authors’ contributions
Conceptualizations, manuscript writing, and figure and table preparation: Tokhanbigli S
Revision of the manuscript: Haghi M
Review and editing: Dua K
Supervision, review, and editing: Oliver BGG
Availability of data and materials
Not applicable.
Financial support and sponsorship
None.
Conflicts of interest
All authors declared that there are no conflicts of interest.
Ethical approval and consent to participate
Not applicable.
Consent for publication
Not applicable.
Copyright
© The Author(s) 2024.
REFERENCES
1. Sung H, Ferlay J, Siegel RL, et al. Global Cancer Statistics 2020: GLOBOCAN estimates of incidence and mortality worldwide for 36 cancers in 185 countries. CA Cancer J Clin 2021;71:209-49.
2. Ferone G, Lee MC, Sage J, Berns A. Cells of origin of lung cancers: lessons from mouse studies. Genes Dev 2020;34:1017-32.
3. Fidler-Benaoudia MM, Torre LA, Bray F, Ferlay J, Jemal A. Lung cancer incidence in young women vs. young men: a systematic analysis in 40 countries. Int J Cancer 2020;147:811-9.
4. Miller KD, Nogueira L, Mariotto AB, et al. Cancer treatment and survivorship statistics, 2019. CA Cancer J Clin 2019;69:363-85.
5. Hanahan D, Coussens LM. Accessories to the crime: functions of cells recruited to the tumor microenvironment. Cancer Cell 2012;21:309-22.
6. Mahale J, Smagurauskaite G, Brown K, Thomas A, Howells LM. The role of stromal fibroblasts in lung carcinogenesis: a target for chemoprevention? Int J Cancer 2016;138:30-44.
7. Foster DS, Jones RE, Ransom RC, Longaker MT, Norton JA. The evolving relationship of wound healing and tumor stroma. JCI Insight 2018;3:99911.
8. Belhabib I, Zaghdoudi S, Lac C, Bousquet C, Jean C. Extracellular matrices and cancer-associated fibroblasts: targets for cancer diagnosis and therapy? Cancers 2021;13:3466.
9. Chan TS, Shaked Y, Tsai KK. Targeting the interplay between cancer fibroblasts, mesenchymal stem cells, and cancer stem cells in desmoplastic cancers. Front Oncol 2019;9:688.
10. Miyazaki Y, Oda T, Mori N, Kida YS. Adipose-derived mesenchymal stem cells differentiate into pancreatic cancer-associated fibroblasts in vitro. FEBS Open Bio 2020;10:2268-81.
11. Shi Y, Du L, Lin L, Wang Y. Tumour-associated mesenchymal stem/stromal cells: emerging therapeutic targets. Nat Rev Drug Discov 2017;16:35-52.
13. Kojima Y, Acar A, Eaton EN, et al. Autocrine TGF-beta and stromal cell-derived factor-1 (SDF-1) signaling drives the evolution of tumor-promoting mammary stromal myofibroblasts. Proc Natl Acad Sci U S A 2010;107:20009-14.
14. Ren Y, Zhou X, Liu X, et al. Reprogramming carcinoma associated fibroblasts by AC1MMYR2 impedes tumor metastasis and improves chemotherapy efficacy. Cancer Lett 2016;374:96-106.
15. Tang PCT, Chung JYF, Xue VWW, et al. Smad3 promotes cancer-associated fibroblasts generation via macrophage-myofibroblast transition. Adv Sci 2022;9:e2101235.
16. Glabman RA, Choyke PL, Sato N. Cancer-associated fibroblasts: tumorigenicity and targeting for cancer therapy. Cancers 2022;14:3906.
17. Saito A, Horie M, Nagase T. TGF-β signaling in lung health and disease. Int J Mol Sci 2018;19:2460.
18. Navab R, Strumpf D, Bandarchi B, et al. Prognostic gene-expression signature of carcinoma-associated fibroblasts in non-small cell lung cancer. Proc Natl Acad Sci U S A 2011;108:7160-5.
19. Neri S, Miyashita T, Hashimoto H, et al. Fibroblast-led cancer cell invasion is activated by epithelial-mesenchymal transition through platelet-derived growth factor BB secretion of lung adenocarcinoma. Cancer Lett 2017;395:20-30.
20. Liu Y, Song X, Wang X, et al. Effect of chronic intermittent hypoxia on biological behavior and hypoxia-associated gene expression in lung cancer cells. J Cell Biochem 2010;111:554-63.
21. Zhang Y, Bian Y, Wang Y, et al. HIF-1α is necessary for activation and tumour-promotion effect of cancer-associated fibroblasts in lung cancer. J Cell Mol Med 2021;25:5457-69.
22. Özdemir BC, Pentcheva-Hoang T, Carstens JL, et al. Depletion of carcinoma-associated fibroblasts and fibrosis induces immunosuppression and accelerates pancreas cancer with reduced survival. Cancer Cell 2014;25:719-34.
23. Rhim AD, Oberstein PE, Thomas DH, et al. Stromal elements act to restrain, rather than support, pancreatic ductal adenocarcinoma. Cancer Cell 2014;25:735-47.
24. Amakye D, Jagani Z, Dorsch M. Unraveling the therapeutic potential of the hedgehog pathway in cancer. Nat Med 2013;19:1410-22.
25. Li Z, Zhou J, Zhang J, Li S, Wang H, Du J. Cancer-associated fibroblasts promote PD-L1 expression in mice cancer cells via secreting CXCL5. Int J Cancer 2019;145:1946-57.
26. Turley SJ, Cremasco V, Astarita JL. Immunological hallmarks of stromal cells in the tumour microenvironment. Nat Rev Immunol 2015;15:669-82.
27. Ziani L, Chouaib S, Thiery J. Alteration of the antitumor immune response by cancer-associated fibroblasts. Front Immunol 2018;9:414.
28. Arnold JN, Magiera L, Kraman M, Fearon DT. Tumoral immune suppression by macrophages expressing fibroblast activation protein-α and heme oxygenase-1. Cancer Immunol Res 2014;2:121-6.
29. Yang X, Lin Y, Shi Y, et al. FAP promotes immunosuppression by cancer-associated fibroblasts in the tumor microenvironment via STAT3-CCL2 signaling. Cancer Res 2016;76:4124-35.
30. Kobayashi H, Enomoto A, Woods SL, Burt AD, Takahashi M, Worthley DL. Cancer-associated fibroblasts in gastrointestinal cancer. Nat Rev Gastroenterol Hepatol 2019;16:282-95.
31. Zhang J, Chen L, Liu X, Kammertoens T, Blankenstein T, Qin Z. Fibroblast-specific protein 1/S100A4-positive cells prevent carcinoma through collagen production and encapsulation of carcinogens. Cancer Res 2013;73:2770-81.
32. Astarita JL, Acton SE, Turley SJ. Podoplanin: emerging functions in development, the immune system, and cancer. Front Immunol 2012;3:283.
33. Krishnan H, Rayes J, Miyashita T, et al. Podoplanin: an emerging cancer biomarker and therapeutic target. Cancer Sci 2018;109:1292-9.
34. Vázquez-Villa F, García-Ocaña M, Galván JA, et al. COL11A1/(pro)collagen 11A1 expression is a remarkable biomarker of human invasive carcinoma-associated stromal cells and carcinoma progression. Tumour Biol 2015;36:2213-22.
35. Jia D, Liu Z, Deng N, et al. A COL11A1-correlated pan-cancer gene signature of activated fibroblasts for the prioritization of therapeutic targets. Cancer Lett 2016;382:203-14.
36. Nishishita R, Morohashi S, Seino H, et al. Expression of cancer-associated fibroblast markers in advanced colorectal cancer. Oncol Lett 2018;15:6195-202.
37. Yu Y, Xiao CH, Tan LD, Wang QS, Li XQ, Feng YM. Cancer-associated fibroblasts induce epithelial-mesenchymal transition of breast cancer cells through paracrine TGF-β signalling. Br J Cancer 2014;110:724-32.
38. Nurmik M, Ullmann P, Rodriguez F, Haan S, Letellier E. In search of definitions: cancer-associated fibroblasts and their markers. Int J Cancer 2020;146:895-905.
39. Martínez-Nieto GA, Teppo HR, Petrelius N, et al. Upregulated integrin α11 in the stroma of cutaneous squamous cell carcinoma promotes skin carcinogenesis. Front Oncol 2022;12:981009.
40. Zeltz C, Navab R, Heljasvaara R, et al. Integrin α11β1 in tumor fibrosis: more than just another cancer-associated fibroblast biomarker? J Cell Commun Signal 2022;16:649-60.
41. Zeltz C, Alam J, Liu H, et al. α11β1 Integrin is induced in a subset of cancer-associated fibroblasts in desmoplastic tumor stroma and mediates in vitro cell migration. Cancers 2019;11:765.
42. Hu G, Huang L, Zhong K, et al. PDGFR-β+ fibroblasts deteriorate survival in human solid tumors: a meta-analysis. Aging 2021;13:13693-707.
43. Yoon H, Tang CM, Banerjee S, et al. Cancer-associated fibroblast secretion of PDGFC promotes gastrointestinal stromal tumor growth and metastasis. Oncogene 2021;40:1957-73.
44. Su S, Chen J, Yao H, et al. CD10+GPR77+ cancer-associated fibroblasts promote cancer formation and chemoresistance by sustaining cancer stemness. Cell 2018;172:841-56.e16.
45. Tong Y, Zhao Z, Zhang J, Wang W, Zhu Y. High expressions of CD10, FAP and GPR77 in CAFs are associated with chemoresistance and worse prognosis in gastric cancer. Front Oncol 2022;12:984817.
46. Katoh D, Kozuka Y, Noro A, Ogawa T, Imanaka-Yoshida K, Yoshida T. Tenascin-C induces phenotypic changes in fibroblasts to myofibroblasts with high contractility through the integrin αvβ1/transforming growth factor β/SMAD signaling axis in human breast cancer. Am J Pathol 2020;190:2123-35.
47. Ni WD, Yang ZT, Cui CA, Cui Y, Fang LY, Xuan YH. Tenascin-C is a potential cancer-associated fibroblasts marker and predicts poor prognosis in prostate cancer. Biochem Biophys Res Commun 2017;486:607-12.
48. Hashimoto M, Uesugi N, Osakabe M, et al. Expression patterns of microenvironmental factors and tenascin-C at the invasive front of stage II and III colorectal cancer: novel tumor prognostic markers. Front Oncol 2021;11:690816.
49. Le PN, Keysar SB, Miller B, et al. Wnt signaling dynamics in head and neck squamous cell cancer tumor-stroma interactions. Mol Carcinog 2019;58:398-410.
50. Qin X, Yan M, Zhang J, et al. TGFβ3-mediated induction of periostin facilitates head and neck cancer growth and is associated with metastasis. Sci Rep 2016;6:20587.
51. Chen D, Che G. Value of caveolin-1 in cancer progression and prognosis: emphasis on cancer-associated fibroblasts, human cancer cells and mechanism of caveolin-1 expression (review). Oncol Lett 2014;8:1409-21.
52. Shimizu K, Kirita K, Aokage K, et al. Clinicopathological significance of caveolin-1 expression by cancer-associated fibroblasts in lung adenocarcinoma. J Cancer Res Clin Oncol 2017;143:321-8.
53. Shi X, Young CD, Zhou H, Wang X. Transforming growth factor-β signaling in fibrotic diseases and cancer-associated fibroblasts. Biomolecules 2020;10:1666.
54. Erdogan B, Webb DJ. Cancer-associated fibroblasts modulate growth factor signaling and extracellular matrix remodeling to regulate tumor metastasis. Biochem Soc Trans 2017;45:229-36.
55. Hassan M, Jo T, Risse PA, et al. Airway smooth muscle remodeling is a dynamic process in severe long-standing asthma. J Allergy Clin Immunol 2010;125:1037-45.e3.
56. Chen Y, Zou L, Zhang Y, et al. Transforming growth factor-β1 and α-smooth muscle actin in stromal fibroblasts are associated with a poor prognosis in patients with clinical stage I-IIIA nonsmall cell lung cancer after curative resection. Tumour Biol 2014;35:6707-13.
57. Alcaraz J, Carrasco JL, Millares L, et al. Stromal markers of activated tumor associated fibroblasts predict poor survival and are associated with necrosis in non-small cell lung cancer. Lung Cancer 2019;135:151-60.
58. Kilvaer TK, Khanehkenari MR, Hellevik T, et al. Cancer associated fibroblasts in stage I-IIIA NSCLC: prognostic impact and their correlations with tumor molecular markers. PLoS One 2015;10:e0134965.
59. Kieffer Y, Hocine HR, Gentric G, et al. Single-cell analysis reveals fibroblast clusters linked to immunotherapy resistance in cancer. Cancer Discov 2020;10:1330-51.
60. Feig C, Jones JO, Kraman M, et al. Targeting CXCL12 from FAP-expressing carcinoma-associated fibroblasts synergizes with anti-PD-L1 immunotherapy in pancreatic cancer. Proc Natl Acad Sci U S A 2013;110:20212-7.
61. Scanlan MJ, Raj BK, Calvo B, et al. Molecular cloning of fibroblast activation protein alpha, a member of the serine protease family selectively expressed in stromal fibroblasts of epithelial cancers. Proc Natl Acad Sci U S A 1994;91:5657-61.
62. Shi X, Luo J, Weigel KJ, et al. Cancer-associated fibroblasts facilitate squamous cell carcinoma lung metastasis in mice by providing TGFβ-mediated cancer stem cell niche. Front Cell Dev Biol 2021;9:668164.
63. Santos AM, Jung J, Aziz N, Kissil JL, Puré E. Targeting fibroblast activation protein inhibits tumor stromagenesis and growth in mice. J Clin Invest 2009;119:3613-25.
64. Jia J, Martin TA, Ye L, et al. Fibroblast activation protein-α promotes the growth and migration of lung cancer cells via the PI3K and sonic hedgehog pathways. Int J Mol Med 2018;41:275-83.
65. Liao Y, Ni Y, He R, Liu W, Du J. Clinical implications of fibroblast activation protein-α in non-small cell lung cancer after curative resection: a new predictor for prognosis. J Cancer Res Clin Oncol 2013;139:1523-8.
66. Coto-Llerena M, Ercan C, Kancherla V, et al. High expression of FAP in colorectal cancer is associated with angiogenesis and immunoregulation processes. Front Oncol 2020;10:979.
67. Wang LC, Lo A, Scholler J, et al. Targeting fibroblast activation protein in tumor stroma with chimeric antigen receptor T cells can inhibit tumor growth and augment host immunity without severe toxicity. Cancer Immunol Res 2014;2:154-66.
68. Kilvaer TK, Rakaee M, Hellevik T, et al. Tissue analyses reveal a potential immune-adjuvant function of FAP-1 positive fibroblasts in non-small cell lung cancer. PLoS One 2018;13:e0192157.
69. Loeffler M, Krüger JA, Niethammer AG, Reisfeld RA. Targeting tumor-associated fibroblasts improves cancer chemotherapy by increasing intratumoral drug uptake. J Clin Invest 2006;116:1955-62.
70. Shen W, Tan X, Hao F. S100A4 expression is associated with poor prognosis in patients with resectable gastrointestinal stromal tumor. Libyan J Med 2019;14:1659669.
71. Destek S, Gul VO. S100A4 may be a good prognostic marker and a therapeutic target for colon cancer. J Oncol 2018;2018:1828791.
72. Stewart RL, Carpenter BL, West DS, et al. S100A4 drives non-small cell lung cancer invasion, associates with poor prognosis, and is effectively targeted by the FDA-approved anti-helminthic agent niclosamide. Oncotarget 2016;7:34630-42.
73. Zhang J, Gu Y, Liu X, Rao X, Huang G, Ouyang Y. Clinicopathological and prognostic value of S100A4 expression in non-small cell lung cancer: a meta-analysis. Biosci Rep 2020;40:BSR20201710.
74. Sun X, Chen Z. Cancer-associated fibroblast-derived CCL5 contributes to cisplatin resistance in A549 NSCLC cells partially through upregulation of lncRNA HOTAIR expression. Oncol Lett 2021;22:696.
75. Qi R, Qiao T, Zhuang X. Small interfering RNA targeting S100A4 sensitizes non-small-cell lung cancer cells (A549) to radiation treatment. Onco Targets Ther 2016;9:3753-62.
76. Hou S, Tian T, Qi D, et al. S100A4 promotes lung tumor development through β-catenin pathway-mediated autophagy inhibition. Cell Death Dis 2018;9:277.
77. Neri S, Ishii G, Hashimoto H, et al. Podoplanin-expressing cancer-associated fibroblasts lead and enhance the local invasion of cancer cells in lung adenocarcinoma. Int J Cancer 2015;137:784-96.
78. Hesse K, Satzger I, Schacht V, et al. Characterisation of prognosis and invasion of cutaneous squamous cell carcinoma by podoplanin and E-cadherin expression. Dermatology 2016;232:558-65.
79. Hoshino A, Ishii G, Ito T, et al. Podoplanin-positive fibroblasts enhance lung adenocarcinoma tumor formation: podoplanin in fibroblast functions for tumor progression. Cancer Res 2011;71:4769-79.
80. Suzuki J, Aokage K, Neri S, et al. Relationship between podoplanin-expressing cancer-associated fibroblasts and the immune microenvironment of early lung squamous cell carcinoma. Lung Cancer 2021;153:1-10.
81. Ito M, Ishii G, Nagai K, Maeda R, Nakano Y, Ochiai A. Prognostic impact of cancer-associated stromal cells in patients with stage I lung adenocarcinoma. Chest 2012;142:151-8.
82. Kawase A, Ishii G, Nagai K, et al. Podoplanin expression by cancer associated fibroblasts predicts poor prognosis of lung adenocarcinoma. Int J Cancer 2008;123:1053-9.
83. Koriyama H, Ishii G, Yoh K, et al. Presence of podoplanin-positive cancer-associated fibroblasts in surgically resected primary lung adenocarcinoma predicts a shorter progression-free survival period in patients with recurrences who received platinum-based chemotherapy. J Cancer Res Clin Oncol 2015;141:1163-70.
84. Ono S, Ishii G, Nagai K, et al. Podoplanin-positive cancer-associated fibroblasts could have prognostic value independent of cancer cell phenotype in stage I lung squamous cell carcinoma: usefulness of combining analysis of both cancer cell phenotype and cancer-associated fibroblast phenotype. Chest 2013;143:963-70.
85. Hu G, Wang S, Xu F, et al. Tumor-infiltrating podoplanin+ fibroblasts predict worse outcome in solid tumors. Cell Physiol Biochem 2018;51:1041-50.
86. Yoshida T, Ishii G, Goto K, et al. Podoplanin-positive cancer-associated fibroblasts in the tumor microenvironment induce primary resistance to EGFR-TKIs in lung adenocarcinoma with EGFR mutation. Clin Cancer Res 2015;21:642-51.
87. Sugiura H, Ichikawa T, Liu X, et al. N-acetyl-L-cysteine inhibits TGF-β1-induced profibrotic responses in fibroblasts. Pulm Pharmacol Ther 2009;22:487-91.
88. Biernacka A, Dobaczewski M, Frangogiannis NG. TGF-β signaling in fibrosis. Growth Factors 2011;29:196-202.
89. Zhu CQ, Popova SN, Brown ERS, et al. Integrin α 11 regulates IGF2 expression in fibroblasts to enhance tumorigenicity of human non-small-cell lung cancer cells. Proc Natl Acad Sci U S A 2007;104:11754-9.
90. Wang KK, Liu N, Radulovich N, et al. Novel candidate tumor marker genes for lung adenocarcinoma. Oncogene 2002;21:7598-604.
91. Navab R, Strumpf D, To C, et al. Integrin α11β1 regulates cancer stromal stiffness and promotes tumorigenicity and metastasis in non-small cell lung cancer. Oncogene 2016;35:1899-908.
92. Zhang Q, Hou X, Evans BJ, VanBlaricom JL, Weroha SJ, Cliby WA. LY2157299 monohydrate, a TGF-βR1 inhibitor, suppresses tumor growth and ascites development in ovarian cancer. Cancers 2018;10:260.
93. Carracedo S, Lu N, Popova SN, Jonsson R, Eckes B, Gullberg D. The fibroblast integrin α11β1 is induced in a mechanosensitive manner involving activin A and regulates myofibroblast differentiation. J Biol Chem 2010;285:10434-45.
94. Honda E, Yoshida K, Munakata H. Transforming growth factor-beta upregulates the expression of integrin and related proteins in MRC-5 human myofibroblasts. Tohoku J Exp Med 2010;220:319-27.
95. Iwai M, Tulafu M, Togo S, et al. Cancer-associated fibroblast migration in non-small cell lung cancers is modulated by increased integrin α11 expression. Mol Oncol 2021;15:1507-27.
96. Lu N, Carracedo S, Ranta J, Heuchel R, Soininen R, Gullberg D. The human alpha11 integrin promoter drives fibroblast-restricted expression in vivo and is regulated by TGF-beta1 in a Smad- and Sp1-dependent manner. Matrix Biol 2010;29:166-76.
97. Talior-Volodarsky I, Connelly KA, Arora PD, Gullberg D, McCulloch CA. α11 integrin stimulates myofibroblast differentiation in diabetic cardiomyopathy. Cardiovasc Res 2012;96:265-75.
99. Dauphin M, Barbe C, Lemaire S, et al. Vimentin expression predicts the occurrence of metastases in non small cell lung carcinomas. Lung Cancer 2013;81:117-22.
100. Burch TC, Watson MT, Nyalwidhe JO. Variable metastatic potentials correlate with differential plectin and vimentin expression in syngeneic androgen independent prostate cancer cells. PLoS One 2013;8:e65005.
101. Chen Z, Fang Z, Ma J. Regulatory mechanisms and clinical significance of vimentin in breast cancer. Biomed Pharmacother 2021;133:111068.
102. Richardson AM, Havel LS, Koyen AE, et al. Vimentin is required for lung adenocarcinoma metastasis via heterotypic tumor cell-cancer-associated fibroblast interactions during collective invasion. Clin Cancer Res 2018;24:420-32.
103. Wang L, Cao L, Wang H, et al. Cancer-associated fibroblasts enhance metastatic potential of lung cancer cells through IL-6/STAT3 signaling pathway. Oncotarget 2017;8:76116-28.
104. Zou X, Tang XY, Qu ZY, et al. Targeting the PDGF/PDGFR signaling pathway for cancer therapy: a review. Int J Biol Macromol 2022;202:539-57.
105. Masoumi J, Jafarzadeh A, Khorramdelazad H, Abbasloui M, Abdolalizadeh J, Jamali N. Role of Apelin/APJ axis in cancer development and progression. Adv Med Sci 2020;65:202-13.
106. Donnem T, Al-Saad S, Al-Shibli K, Andersen S, Busund LT, Bremnes RM. Prognostic impact of platelet-derived growth factors in non-small cell lung cancer tumor and stromal cells. J Thorac Oncol 2008;3:963-70.
107. Tejada ML, Yu L, Dong J, et al. Tumor-driven paracrine platelet-derived growth factor receptor alpha signaling is a key determinant of stromal cell recruitment in a model of human lung carcinoma. Clin Cancer Res 2006;12:2676-88.
108. Laing N, McDermott B, Wen S, et al. Inhibition of platelet-derived growth factor receptor α by MEDI-575 reduces tumor growth and stromal fibroblast content in a model of non-small cell lung cancer. Mol Pharmacol 2013;83:1247-56.
109. Jones RL, Judson IR. The development and application of imatinib. Expert Opin Drug Saf 2005;4:183-91.
110. Kinoshita K, Nakagawa K, Hamada JI, et al. Imatinib mesylate inhibits the proliferation-stimulating effect of human lung cancer-associated stromal fibroblasts on lung cancer cells. Int J Oncol 2010;37:869-77.
111. Haubeiss S, Schmid JO, Mürdter TE, et al. Dasatinib reverses cancer-associated fibroblasts (CAFs) from primary lung carcinomas to a phenotype comparable to that of normal fibroblasts. Mol Cancer 2010;9:168.
112. Liu JQ, Hu A, Zhu J, Yu J, Talebian F, Bai XF. CD200-CD200R pathway in the regulation of tumor immune microenvironment and immunotherapy. Adv Exp Med Biol 2020;1223:155-65.
113. Kotwica-Mojzych K, Jodłowska-Jędrych B, Mojzych M. CD200:CD200R interactions and their importance in immunoregulation. Int J Mol Sci 2021;22:1602.
114. Ishibashi M, Neri S, Hashimoto H, et al. CD200-positive cancer associated fibroblasts augment the sensitivity of epidermal growth factor receptor mutation-positive lung adenocarcinomas to EGFR tyrosine kinase inhibitors. Sci Rep 2017;7:46662.
115. Zhao S, Jiang T, Zhang L, et al. Clinicopathological and prognostic significance of regulatory T cells in patients with non-small cell lung cancer: a systematic review with meta-analysis. Oncotarget 2016;7:36065-73.
116. Maeda Y, Nishikawa H, Sugiyama D, et al. Detection of self-reactive CD8+ T cells with an anergic phenotype in healthy individuals. Science 2014;346:1536-40.
117. Pollack SM, He Q, Yearley JH, et al. T-cell infiltration and clonality correlate with programmed cell death protein 1 and programmed death-ligand 1 expression in patients with soft tissue sarcomas. Cancer 2017;123:3291-304.
118. Zhang S, Cherwinski H, Sedgwick JD, Phillips JH. Molecular mechanisms of CD200 inhibition of mast cell activation. J Immunol 2004;173:6786-93.
119. Su Y, Yamazaki S, Morisue R, et al. Tumor-infiltrating T cells concurrently overexpress CD200R with immune checkpoints PD-1, CTLA-4, and TIM-3 in non-small-cell lung cancer. Pathobiology 2021;88:218-27.
120. Tucker RP, Degen M. Revisiting the tenascins: exploitable as cancer targets? Front Oncol 2022;12:908247.
121. Bonnans C, Chou J, Werb Z. Remodelling the extracellular matrix in development and disease. Nat Rev Mol Cell Biol 2014;15:786-801.
123. Okada T, Suzuki H. The role of tenascin-C in tissue injury and repair after stroke. Front Immunol 2020;11:607587.
124. Radwanska A, Grall D, Schaub S, et al. Counterbalancing anti-adhesive effects of tenascin-C through fibronectin expression in endothelial cells. Sci Rep 2017;7:12762.
125. Geleta B, Tout FS, Lim SC, et al. Targeting Wnt/tenascin C-mediated cross talk between pancreatic cancer cells and stellate cells via activation of the metastasis suppressor NDRG1. J Biol Chem 2022;298:101608.
126. Gocheva V, Naba A, Bhutkar A, et al. Quantitative proteomics identify tenascin-C as a promoter of lung cancer progression and contributor to a signature prognostic of patient survival. Proc Natl Acad Sci U S A 2017;114:E5625-34.
127. Tang YA, Chen CH, Sun HS, et al. Global Oct4 target gene analysis reveals novel downstream PTEN and TNC genes required for drug-resistance and metastasis in lung cancer. Nucleic Acids Res 2015;43:1593-608.
128. Gebauer F, Gelis S, Zander H, et al. Tenascin-C serum levels and its prognostic power in non-small cell lung cancer. Oncotarget 2016;7:20945-52.
129. Ishiwata T, Takahashi K, Shimanuki Y, et al. Serum tenascin-C as a potential predictive marker of angiogenesis in non-small cell lung cancer. Anticancer Res 2005;25:489-95.
130. Huang JY, Cheng YJ, Lin YP, et al. Extracellular matrix of glioblastoma inhibits polarization and transmigration of T cells: the role of tenascin-C in immune suppression. J Immunol 2010;185:1450-9.
131. Onion D, Isherwood M, Shridhar N, et al. Multicomponent analysis of the tumour microenvironment reveals low CD8 T cell number, low stromal caveolin-1 and high tenascin-C and their combination as significant prognostic markers in non-small cell lung cancer. Oncotarget 2018;9:1760-71.
132. Raudenska M, Gumulec J, Balvan J, Masarik M. Caveolin-1 in oncogenic metabolic symbiosis. Int J Cancer 2020;147:1793-807.
133. Zhao X, He Y, Gao J, et al. Caveolin-1 expression level in cancer associated fibroblasts predicts outcome in gastric cancer. PLoS One 2013;8:e59102.
134. Luan TY, Zhu TN, Cui YJ, et al. Expression of caveolin-1 is correlated with lung adenocarcinoma proliferation, migration, and invasion. Med Oncol 2015;32:207.
135. Ho CC, Kuo SH, Huang PH, Huang HY, Yang CH, Yang PC. Caveolin-1 expression is significantly associated with drug resistance and poor prognosis in advanced non-small cell lung cancer patients treated with gemcitabine-based chemotherapy. Lung Cancer 2008;59:105-10.
136. Zhan P, Shen XK, Qian Q, et al. Expression of caveolin-1 is correlated with disease stage and survival in lung adenocarcinomas. Oncol Rep 2012;27:1072-8.
137. Bertino EM, Williams TM, Nana-Sinkam SP, et al. Stromal caveolin-1 is associated with response and survival in a phase II trial of nab-paclitaxel with carboplatin for advanced NSCLC patients. Clin Lung Cancer 2015;16:466-74.
138. González-González L, Alonso J. Periostin: a matricellular protein with multiple functions in cancer development and progression. Front Oncol 2018;8:225.
139. O’Dwyer DN, Moore BB. The role of periostin in lung fibrosis and airway remodeling. Cell Mol Life Sci 2017;74:4305-14.
140. Naik PK, Bozyk PD, Bentley JK, et al; COMET Investigators. Periostin promotes fibrosis and predicts progression in patients with idiopathic pulmonary fibrosis. Am J Physiol Lung Cell Mol Physiol 2012;303:L1046-56.
141. Ratajczak-Wielgomas K, Kmiecik A, Grzegrzołka J, et al. Prognostic significance of stromal periostin expression in non-small cell lung cancer. Int J Mol Sci 2020;21:7025.
142. Yoshihara T, Nanri Y, Nunomura S, et al. Periostin plays a critical role in the cell cycle in lung fibroblasts. Respir Res 2020;21:38.
143. Ouyang G, Liu M, Ruan K, Song G, Mao Y, Bao S. Upregulated expression of periostin by hypoxia in non-small-cell lung cancer cells promotes cell survival via the Akt/PKB pathway. Cancer Lett 2009;281:213-9.
144. Shochet G, Brook E, Bardenstein-Wald B, Shitrit D. TGF-β pathway activation by idiopathic pulmonary fibrosis (IPF) fibroblast derived soluble factors is mediated by IL-6 trans-signaling. Respir Res 2020;21:56.
145. Chen X, Shi C, Cao H, et al. The hedgehog and Wnt/β-catenin system machinery mediate myofibroblast differentiation of LR-MSCs in pulmonary fibrogenesis. Cell Death Dis 2018;9:639.
146. Yamato H, Kimura K, Fukui E, et al. Periostin secreted by activated fibroblasts in idiopathic pulmonary fibrosis promotes tumorigenesis of non-small cell lung cancer. Sci Rep 2021;11:21114.
147. Reck M, Kaiser R, Mellemgaard A, et al; LUME-Lung 1 Study Group. Docetaxel plus nintedanib versus docetaxel plus placebo in patients with previously treated non-small-cell lung cancer (LUME-Lung 1): a phase 3, double-blind, randomised controlled trial. Lancet Oncol 2014;15:143-55.
148. Richeldi L, du Bois RM, Raghu G, et al; INPULSIS Trial Investigators. Efficacy and safety of nintedanib in idiopathic pulmonary fibrosis. N Engl J Med 2014;370:2071-82.
149. Kato R, Haratani K, Hayashi H, et al. Nintedanib promotes antitumour immunity and shows antitumour activity in combination with PD-1 blockade in mice: potential role of cancer-associated fibroblasts. Br J Cancer 2021;124:914-24.
150. Kim D, Kim JS, Cheon I, et al. Identification and characterization of cancer-associated fibroblast subpopulations in lung adenocarcinoma. Cancers 2022;14:3486.
151. Hu H, Piotrowska Z, Hare PJ, et al. Three subtypes of lung cancer fibroblasts define distinct therapeutic paradigms. Cancer Cell 2021;39:1531-47.e10.
152. Lambrechts D, Wauters E, Boeckx B, et al. Phenotype molding of stromal cells in the lung tumor microenvironment. Nat Med 2018;24:1277-89.
153. Zhao X, He Y, Chen H. Autophagic tumor stroma: mechanisms and roles in tumor growth and progression. Int J Cancer 2013;132:1-8.
154. Zhang D, Ding L, Li Y, et al. Midkine derived from cancer-associated fibroblasts promotes cisplatin-resistance via up-regulation of the expression of lncRNA ANRIL in tumour cells. Sci Rep 2017;7:16231.
155. Leung CS, Yeung TL, Yip KP, et al. Cancer-associated fibroblasts regulate endothelial adhesion protein LPP to promote ovarian cancer chemoresistance. J Clin Invest 2018;128:589-606.
156. Li Z, Chan K, Qi Y, et al. Participation of CCL1 in snail-positive fibroblasts in colorectal cancer contribute to 5-fluorouracil/paclitaxel chemoresistance. Cancer Res Treat 2018;50:894-907.
157. Wei L, Ye H, Li G, et al. Cancer-associated fibroblasts promote progression and gemcitabine resistance via the SDF-1/SATB-1 pathway in pancreatic cancer. Cell Death Dis 2018;9:1065.
158. Wang L, Zhang F, Cui JY, Chen L, Chen YT, Liu BW. CAFs enhance paclitaxel resistance by inducing EMT through the IL‑6/JAK2/STAT3 pathway. Oncol Rep 2018;39:2081-90.
159. Blanco-Gómez A, Hontecillas-Prieto L, Corchado-Cobos R, et al. Stromal SNAI2 is required for ERBB2 breast cancer progression. Cancer Res 2020;80:5216-30.
160. Li J, Guan J, Long X, Wang Y, Xiang X. mir-1-mediated paracrine effect of cancer-associated fibroblasts on lung cancer cell proliferation and chemoresistance. Oncol Rep 2016;35:3523-31.
161. Yi Y, Zeng S, Wang Z, et al. Cancer-associated fibroblasts promote epithelial-mesenchymal transition and EGFR-TKI resistance of non-small cell lung cancers via HGF/IGF-1/ANXA2 signaling. Biochim Biophys Acta Mol Basis Dis 2018;1864:793-803.
162. Zhou B, Sun C, Li N, et al. Cisplatin-induced CCL5 secretion from CAFs promotes cisplatin-resistance in ovarian cancer via regulation of the STAT3 and PI3K/Akt signaling pathways. Int J Oncol 2016;48:2087-97.
163. Quante M, Tu SP, Tomita H, et al. Bone marrow-derived myofibroblasts contribute to the mesenchymal stem cell niche and promote tumor growth. Cancer Cell 2011;19:257-72.
164. Lotti F, Jarrar AM, Pai RK, et al. Chemotherapy activates cancer-associated fibroblasts to maintain colorectal cancer-initiating cells by IL-17A. J Exp Med 2013;210:2851-72.
165. Butti R, Gunasekaran VP, Kumar TVS, Banerjee P, Kundu GC. Breast cancer stem cells: Biology and therapeutic implications. Int J Biochem Cell Biol 2019;107:38-52.
166. Vermeulen L, De Sousa E Melo F, van der Heijden M, et al. Wnt activity defines colon cancer stem cells and is regulated by the microenvironment. Nat Cell Biol 2010;12:468-76.
167. Bu L, Baba H, Yasuda T, Uchihara T, Ishimoto T. Functional diversity of cancer-associated fibroblasts in modulating drug resistance. Cancer Sci 2020;111:3468-77.
168. Yu T, Yang G, Hou Y, et al. Cytoplasmic GPER translocation in cancer-associated fibroblasts mediates cAMP/PKA/CREB/glycolytic axis to confer tumor cells with multidrug resistance. Oncogene 2017;36:2131-45.
169. Apicella M, Giannoni E, Fiore S, et al. Increased lactate secretion by cancer cells sustains non-cell-autonomous adaptive resistance to MET and EGFR targeted therapies. Cell Metab 2018;28:848-65.e6.
170. Vukovic V, Tannock IF. Influence of low pH on cytotoxicity of paclitaxel, mitoxantrone and topotecan. Br J Cancer 1997;75:1167-72.
171. Bartrons R, Simon-Molas H, Rodríguez-García A, et al. Fructose 2,6-bisphosphate in cancer cell metabolism. Front Oncol 2018;8:331.
173. Hu Y, Liu J, Huang H. Recent agents targeting HIF-1α for cancer therapy. J Cell Biochem 2013;114:498-509.
174. Glentis A, Oertle P, Mariani P, et al. Cancer-associated fibroblasts induce metalloprotease-independent cancer cell invasion of the basement membrane. Nat Commun 2017;8:924.
175. Soysal SD, Tzankov A, Muenst SE. Role of the tumor microenvironment in breast cancer. Pathobiology 2015;82:142-52.
176. Gonzalez-Avila G, Sommer B, Mendoza-Posada DA, Ramos C, Garcia-Hernandez AA, Falfan-Valencia R. Matrix metalloproteinases participation in the metastatic process and their diagnostic and therapeutic applications in cancer. Crit Rev Oncol Hematol 2019;137:57-83.
177. Hao J, Zeltz C, Pintilie M, et al. Characterization of distinct populations of carcinoma-associated fibroblasts from non-small cell lung carcinoma reveals a role for ST8SIA2 in cancer cell invasion. Neoplasia 2019;21:482-93.
178. Joyce MH, Lu C, James ER, et al. Phenotypic basis for matrix stiffness-dependent chemoresistance of breast cancer cells to doxorubicin. Front Oncol 2018;8:337.
179. Li X, Li Q, Yu X, Li H, Huang G. Reverse of microtubule-directed chemotherapeutic drugs resistance induced by cancer-associated fibroblasts in breast cancer. Onco Targets Ther 2019;12:7963-73.
180. Folgueira MAAK, Maistro S, Katayama MLH, et al. Markers of breast cancer stromal fibroblasts in the primary tumour site associated with lymph node metastasis: a systematic review including our case series. Biosci Rep 2013;33:e00085.
181. Ishii G, Ochiai A, Neri S. Phenotypic and functional heterogeneity of cancer-associated fibroblast within the tumor microenvironment. Adv Drug Deliv Rev 2016;99:186-96.
182. Pontiggia O, Sampayo R, Raffo D, et al. The tumor microenvironment modulates tamoxifen resistance in breast cancer: a role for soluble stromal factors and fibronectin through β1 integrin. Breast Cancer Res Treat 2012;133:459-71.
183. Howlader N, Forjaz G, Mooradian MJ, et al. The effect of advances in lung-cancer treatment on population mortality. N Engl J Med 2020;383:640-9.
184. Bughda R, Dimou P, D’Souza RR, Klampatsa A. Fibroblast activation protein (FAP)-targeted CAR-T cells: launching an attack on tumor stroma. Immunotargets Ther 2021;10:313-23.
185. Shintani Y, Fujiwara A, Kimura T, et al. IL-6 secreted from cancer-associated fibroblasts mediates chemoresistance in NSCLC by increasing epithelial-mesenchymal transition signaling. J Thorac Oncol 2016;11:1482-92.
187. Qu J, Kalyani FS, Liu L, Cheng T, Chen L. Tumor organoids: synergistic applications, current challenges, and future prospects in cancer therapy. Cancer Commun 2021;41:1331-53.
188. Nakamura H, Sugano M, Miyashita T, et al. Organoid culture containing cancer cells and stromal cells reveals that podoplanin-positive cancer-associated fibroblasts enhance proliferation of lung cancer cells. Lung Cancer 2019;134:100-7.
189. Dominguez CX, Müller S, Keerthivasan S, et al. Single-cell RNA sequencing reveals stromal evolution into LRRC15+ myofibroblasts as a determinant of patient response to cancer immunotherapy. Cancer Discov 2020;10:232-53.
190. Mahmoudi S, Mancini E, Xu L, et al. Heterogeneity in old fibroblasts is linked to variability in reprogramming and wound healing. Nature 2019;574:553-8.
191. LeBleu VS, Kalluri R. A peek into cancer-associated fibroblasts: origins, functions and translational impact. Dis Model Mech 2018;11:dmm029447.
193. Laughney AM, Hu J, Campbell NR, et al. Regenerative lineages and immune-mediated pruning in lung cancer metastasis. Nat Med 2020;26:259-69.
194. Maynard A, McCoach CE, Rotow JK, et al. Therapy-induced evolution of human lung cancer revealed by single-cell RNA sequencing. Cell 2020;182:1232-51.e22.
195. Lawson WE, Polosukhin VV, Zoia O, et al. Characterization of fibroblast-specific protein 1 in pulmonary fibrosis. Am J Respir Crit Care Med 2005;171:899-907.
196. Lyu P, Gu X, Wang F, et al. Advances in targeting cancer-associated fibroblasts through single-cell spatial transcriptomic sequencing. Biomark Res 2024;12:73.
Cite This Article
How to Cite
Download Citation
Export Citation File:
Type of Import
Tips on Downloading Citation
Citation Manager File Format
Type of Import
Direct Import: When the Direct Import option is selected (the default state), a dialogue box will give you the option to Save or Open the downloaded citation data. Choosing Open will either launch your citation manager or give you a choice of applications with which to use the metadata. The Save option saves the file locally for later use.
Indirect Import: When the Indirect Import option is selected, the metadata is displayed and may be copied and pasted as needed.
About This Article
Copyright
Data & Comments
Data
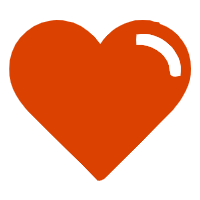
Comments
Comments must be written in English. Spam, offensive content, impersonation, and private information will not be permitted. If any comment is reported and identified as inappropriate content by OAE staff, the comment will be removed without notice. If you have any queries or need any help, please contact us at [email protected].