Radioembolization for hepatocellular carcinoma: updated strategies and evolving clinical applications
Abstract
Primary liver cancer, particularly hepatocellular carcinoma (HCC), ranks among the top causes of cancer deaths worldwide. Traditionally, liver cancer has been associated with a dismal prognosis, which has led to extensive research into potential treatments. Over the past decade, there have been substantial advancements, particularly in the area of locoregional therapies such as radioembolization. This paper provides a summary of the expanding role of radioembolization in HCC treatment, emphasizing recent updates in therapeutic guidelines and ongoing clinical research.
Keywords
INTRODUCTION
Hepatocellular carcinoma (HCC), which accounts for 75% to 85% of primary liver cancer cases, presents a major global health challenge, as this condition is the third leading cause of cancer-related mortality[1]. In 2020, there were approximately 906,000 new HCC diagnoses and 830,000 deaths attributed to HCC worldwide[2]. The similarity between these incidence and mortality rates underscores the poor prognosis associated with this disease. Although substantial progress has been made over the past decade in the management of HCC, the generally poor prognosis associated with this disease highlights the need for continued innovation in treatment modalities.
Radioembolization has emerged as a promising locoregional therapy for HCC, offering an approach different to that provided by traditional intra-arterial therapies. Unlike transarterial bland embolization, conventional transarterial chemoembolization (TACE), and drug-eluting bead TACE (DEB-TACE), which primarily work by inducing ischemia, radioembolization relies on targeted delivery of high-dose radiation particles. It delivers the beta-emitter yttrium-90 (90Y) incorporated into microspheres directly to the tumor via a microcatheter through an arterial feeder. Importantly, radioembolization delivers these microspheres without compromising hepatic arterial blood flow, thus minimizing the risk of postembolization syndrome that is common with other intra-arterial therapies.
In this article, we discuss the evolving role of radioembolization in the management of HCC, examining the latest evidence and exploring the potential of this treatment to improve patient outcomes. We highlight landmark clinical data comparing radioembolization to other treatment options and discuss the place of radioembolization in the evolving landscape of treatment for HCC.
EVOLVING PARADIGM OF RADIOEMBOLIZATION USE
Early randomized controlled trials failed to demonstrate a clear survival benefit with radioembolization over standard treatments such as TACE or sorafenib in patients with early to intermediate-stage HCC[3,4] or intermediate- to advanced-stage HCC[5-8]. This lack of definitive evidence led to uncertainty regarding the precise role of radioembolization in the HCC treatment landscape[9]. Despite these initial setbacks, radioembolization found its niche in several specific clinical scenarios. It became a valuable palliative option for patients ineligible for TACE and gained recognition as a preferred treatment for HCC in patients with portal vein thrombosis because of its lower risk of hepatic parenchymal damage and ischemia[10]. Additionally, radioembolization was increasingly employed in other settings, including as bridging or downstaging therapy before liver transplant or resection and as salvage therapy for patients with advanced HCC refractory to TACE[11,12].
The limitations of the early randomized controlled trials, which may have contributed to their inconclusive results, included simplistic dosimetry that relied on body surface area for dosing calculations without considering tumor-absorbed dose or liver-absorbed dose, as well as broad patient recruitment criteria that resulted in heterogeneous patient populations within study arms[13,14]. Subsequent post-hoc analyses of these trials have shed a more favorable light on radioembolization. For instance, when modern dosimetry was incorporated into the analyses, a strong correlation was seen between higher tumor-absorbed doses and improved overall survival (OS) and disease control in the radioembolization group[15]. Additionally, when appropriate patient selection criteria were applied in the radioembolization arm (e.g., tumor burden ≤ 25% and albumin-bilirubin grade 1), median OS was longer in the radioembolization group (21.9 months) than in the sorafenib group (17 months)[16]. These findings suggest that optimizing patient selection and treatment planning, particularly regarding dosimetry, is crucial for maximizing the efficacy of radioembolization.
Recently, data regarding long-term outcomes of transarterial radioembolization (TARE) utilization for HCC have emerged. A retrospective study involving 88 patients who underwent TARE for HCC indicated that 77.3% of these patients either achieved downstaging or maintained their status within the Milan criteria 6 months after treatment. This sustained effect was observed in 74.4% one year post-treatment, and in 70.0% of patients two years post-treatment, respectively[17]. Similarly, the long-term outcomes of liver resection and liver transplantation after TARE have been found to be feasible. A retrospective study that included 34 patients who were initially ineligible for upfront surgery due to failure to meet oncological or surgical criteria, reported a 10-year OS and disease-free survival of 57% and 43.1%, respectively, after liver resection, and 51.3% and 43.3%, respectively, after liver transplantation[18].
DOSIMETRY: MOVING BEYOND ONE-SIZE-FITS-ALL
Achieving optimal tumor control while minimizing damage to healthy liver tissue is paramount in radioembolization. Delivering a sufficiently high absorbed dose to the tumor increases the likelihood of achieving complete pathological necrosis[19]. However, early radioembolization trials often relied on simplistic dosimetry methods, assuming a homogeneous distribution of spheres[20]. The simplified medical internal radiation dosimetry (MIRD) equation underlying these methods is as follows:
Absorbed dose (Gy) = activity of 90Y (GBq) × 50 × (1 - lung shunt fraction) / organ or mass (kg)[20]
With this method, the treated area is considered as a single compartment, and the liver tissue and the tumor are assumed to receive a homogeneously equal dose [Figure 1]. This type of homogeneous dose distribution is seen with external beam radiation therapy (RT); in contrast, radioembolization involves a heterogeneous dose distribution that is inherently determined by the biodistribution of the injected radiolabeled microspheres[20]. In light of these differences, the field of radioembolization has shifted toward personalized dosimetry approaches based on refined MIRD schema, including partition models[15,21].
Figure 1. Dosimetry approaches (shown here as used for radiation lobectomy). (A) Single-compartment model, in which tumor and nontumoral parenchyma receive equal doses; (B) Multicompartment model, in which tumor and nontumoral parenchyma receive different doses.
The landmark DOSISPHERE-01 trial provided compelling evidence supporting the use of personalized dosimetry. This randomized controlled trial demonstrated that patients with unresectable HCC who underwent radioembolization using personalized dosimetry experienced significantly higher objective response rates (ORR) (71% vs. 36%; P = 0.007) and downstaging to allow for surgical treatments (36% vs. 4%; P = 0.029), as well as longer OS (26.6 months vs. 10.7 months; P = 0.006), compared to patients who underwent radioembolization using standard dosimetry[22]. The TARGET study also confirmed the effectiveness of personalized dosimetry for radioembolization. In this study, a higher tumor-absorbed dose was associated with a longer OS [hazard ratio (HR): 0.83 per 100 Gy increased, 95% confidence interval (CI): (0.71-0.95); P = 0.009][23].
Determining the optimal radiation dose for radioembolization is complex and requires consideration of multiple factors, including microsphere type and radiological properties of the tumor and the liver parenchyma. Another important factor is the type of embolization device used. The radioembolization devices currently approved by the US FDA include a resin-based device (SIR-Spheres; SIRTeX Medical Limited, Inc; North Sydney, Australia) and a glass-based device (TheraSPhere; BTG International Ltd; London, UK). These devices differ in their physical properties, including the size of the particles and number of particles per vial, specific gravity of the particles, and amount of radiation carried per particle (68 Bq/sphere for resin; 2500 Bq/sphere for glass). These differences, in turn, affect particle distribution within the tumor and the amount of radiation dose delivered. Another important factor to consider is the radiosensitivity of the tumor and surrounding liver tissue. Balancing the tumoricidal dose with the maximum tolerated dose for healthy liver tissue is crucial for achieving optimal tumor response with minimal effect on the surrounding healthy liver parenchyma.
DELIVERY METHODS FOR RADIOEMBOLIZATION
When radioembolization is offered with curative intent, radiation segmentectomy, radiation lobectomy, or modified radiation lobectomy is performed. Radiation segmentectomy is typically used for smaller tumors confined to 1 or 2 segments of the liver where a superselective high dose of radiation can be delivered. In patients with multiple unilobar HCC tumors who are not candidates for curative surgical resection because of inadequate future liver remnant (FLR), radiation lobectomy or modified radiation lobectomy can be offered. In these cases, higher-dose radioembolization of the lobe is performed with the intention of causing atrophy of that lobe and hypertrophy of the FLR in preparation for surgical resection.
When radioembolization is offered with palliative intent, lobar treatments are performed. Typically, these patients have multifocal unilobar or bilobar HCC with or without macrovascular invasions. In these instances, lobar treatment can be performed, with radiation to the liver parenchyma kept below the maximum tolerated dose. In cases of bilobar multifocal HCC, sequential lobar radioembolization can be performed. The methods of delivery, along with recommended dose calculations and dosimetry, are summarized in Table 1.
Methods of delivery, dose calculation, and dosimetry for each type of microsphere
Microsphere | Curative intent | Palliative intent | ||||
Radiation segmentectomy | Radiation lobectomy | Modified radiation lobectomy | Multifocal unilobar disease | Multifocal bilobar disease | HCC with macrovascular invasion | |
Dosimetry model | Single-compartment MIRD model | Multicompartment model | Single-compartment MIRD model | Multicompartment model | Multicompartment model | Multicompartment model |
Resin[24] | > 150 Gy to the angiosome[24] | > 70 Gy of NTAD | N/A | 40 Gy to NTAD | 30-40 Gy to NTAD | N/A |
Glass[25] | ≥ 400 Gy to the angiosome[25] | ≥ 205 Gy of TAD, ≤ 120 Gy of NTAD | 400 Gy to treating segment, 100 Gy to treating lobe | ≥ 205 Gy of TAD (> 250 Gy if possible), ≤ 120 Gy of NTAD | ≥ 205 Gy of TAD (> 250 Gy if possible), 40-70 Gy to NTAD | ≥ 205 Gy of TAD (> 250 Gy if possible), ≤ 120 Gy to NTAD |
Precise delivery of therapeutic radiation while sparing healthy liver tissue relies heavily on accurate pretreatment planning, which must include a thorough evaluation of the location and number of tumors, as well as identification of their feeding arteries. This is typically accomplished with cone-beam computed tomography (CT) performed during the mapping procedure before treatment. This method is considered the gold standard for perfused angiosome volume determination, aiding in precise vessel targeting and ensuring complete tumor coverage[24,25].
Despite meticulous dosimetry, adverse reactions involving the utilization of TARE have been reported. A retrospective cohort study examining 85 patients with HCC who underwent TARE revealed that 44.7% experienced liver decompensation. These patients exhibited significantly shorter OS compared to those without liver decompensation (16 months vs. 31 months, P = 0.001). Additionally, 18.8% of them developed radioembolization-induced liver disease (REILD)[26].
UPDATED TREATMENT LANDSCAPE AND THE ROLE OF RADIOEMBOLIZATION
The Barcelona clinic liver cancer (BCLC) staging system remains the cornerstone of HCC management. However, recent iterations acknowledge the limitations of rigid staging criteria and advocate for a more individualized approach. Concepts such as treatment stage migration and untreatable progression recognize that patients may benefit from therapies outside their initial BCLC stage based on tumor response, treatment availability, and individual patient factors[27,28].
This paradigm shift has opened the door for broader use of radioembolization, particularly in earlier HCC stages, for which it was previously considered a secondary option. The landmark LEGACY study has been instrumental in this evolution[29].
Radioembolization in various BCLC stages
Very early and early stage HCC (BCLC 0 and A)
These stages are characterized by a solitary HCC tumor ≤ 2 cm (BCLC-0), a solitary HCC tumor of any size, or multiple HCC tumors (up to 3) with each measuring < 3 cm in maximum diameter (BCLC-A), without vascular invasion or extrahepatic spread and with preserved liver function and good performance status (Eastern Cooperative Oncology Group grade 0). For patients in these stages, liver transplant or surgical resection is considered; for patients with contraindications to surgery, percutaneous thermal ablation is offered[30,31].
Although liver transplant remains the treatment of choice for these patients, donor shortage and potential waitlist dropout necessitate the use of bridging or alternative therapies[9]. In these cases, radiation segmentectomy can be offered as an alternative[29,32,33]. Radiation segmentectomy involves superselective delivery of 90Y to ≤ 2 Couinaud segments with curative intent [Figures 2 and 3].
Figure 2. Radiation segmentectomy. The single-compartment model is recommended, with treatment superselectively delivered to subsegments of the liver (angiosomes).
Figure 3. (A) Arterial phase CT scan demonstrates a 2.5-cm heterogeneous enhancing lesion at segment VII (arrow); (B) Selective catheterization into the right hepatic artery branch shows a hypervascular lesion (arrow); (C) Cone-beam CT scan confirms the feeder and coverage area; (D) 99mTc-MAA SPECT/CT image shows the accumulation of MAA in the right hepatic lobe, with the most prominent focus at the tumor location in segment VII (arrow); (E) Post-treatment PET/CT scan shows 90Y activity in the posterior right hepatic lobe, with the most intense area accumulating in segment VII, consistent with the location of the tumor (arrow); (F) Follow-up CT scan performed 6 months after treatment shows shrinkage of the treated lesion, now measuring 2 cm, without evidence of viable tumor. CT: Computed tomography; MAA: macroaggregated albumin; SPECT: single-photon emission CT; PET: positron emission tomography; 90Y: yttrium-90.
Multiple studies have evaluated the efficacy of radiation segmentectomy in patients with HCC. For example, the LEGACY trial included patients with solitary HCC tumors < 8 cm. In this trial, glass microspheres were used to deliver a median absorbed dose of 410 Gy. The best ORR was 88.3% during a 29.9-month follow-up, and the 3-year OS rate was 86.6%, with 62.2% of patients exhibiting a duration of response ≥ 6 months[29]. The RASER study included 29 patients with solitary HCC tumors ≤ 3 cm who were not candidates for thermal ablation. Radiation segmentectomy was performed with a target dose of > 205 Gy based on the MIRD model. Complete tumor response was seen in 83% of study patients. Patients who demonstrated a complete response received a median absorbed dose of 584 Gy (range: 181-3,340 Gy)[32].
A recent multi-institutional retrospective study involving 123 patients with solitary HCC tumors ≤ 8 cm demonstrated comparable overall progression in patients treated with radiation segmentectomy and those treated with surgical resection [HR: 1.16, 95%CI: (0.51-2.63); P = 0.71]. Furthermore, the study demonstrated a lower incidence of major adverse events in the radiation segmentectomy arm[34]. In another retrospective study of 68 patients with solitary HCC tumors ≤ 4 cm who underwent either radiation segmentectomy or microwave ablation, the patient groups demonstrated comparable ORR (90.9% vs. 82.6%; P = 0.548) and mean OS (59 months vs. 44.3 months; P = 0.203). Notably, radiation segmentectomy provided longer target progression-free survival (PFS) (57.8 months vs. 38.6 months; P = 0.005)[35]. Radiation segmentectomy has also been shown to be effective as a bridging or downstaging therapy before transplant. A recent retrospective study included patients with UNOS T3 HCC, who underwent locoregional treatment for downstaging before transplantation, demonstrated that TARE resulted in greater pathologic necrosis compared to TACE (n =36 vs. n = 14, P = 0.01). Furthermore, the study found that recurrence was more common in patients who did not achieve complete pathological necrosis (16% vs. 3%, P = 0.11)[36].
Intermediate-stage HCC (BCLC-B)
This stage of HCC represents a heterogeneous group of patients, and treatment decisions, therefore, require careful consideration of tumor burden, liver function, and individual patient factors. The 2022 BCLC update stratifies this stage into three subgroups based on tumor burden and liver function[27]. The group characterized by preserved portal flow and defined tumor burden in patients ineligible for liver transplant is the focus of discussion here. Traditionally, TACE has been the mainstay treatment for this group, encompassing both conventional TACE and DEB-TACE. However, recent studies suggest a potential paradigm shift toward radioembolization[37,38].
In a single-center randomized controlled trial including patients with BCLC-A or BCLC-B HCC, the median OS was comparable for those treated with radioembolization (18.6 months) and those treated with TACE (17.7 months; P = 0.990). However, patients treated with radioembolization demonstrated a significantly longer time to progression (TTP) (> 26 months) than those treated with TACE (6.8 months;
These findings collectively suggest that radioembolization is a safe and effective treatment option for patients with BCLC-B HCC, even in those who were previously treated with TACE. The superior tumor control and comparable survival outcomes, particularly as shown in recent studies, warrant consideration of radioembolization as a primary treatment modality for this patient population.
Advanced-stage HCC (BCLC-C)
This stage is characterized by the presence of vascular invasion or extrahepatic spread in patients with preserved liver function. These patients are typically offered systemic therapy; however, the landscape of systemic treatment has shifted from the use of tyrosine kinase inhibitors such as sorafenib to the use of immunotherapy-based regimens such as atezolizumab plus bevacizumab[27,44,45]. The IMbrave 150 trial demonstrated better median OS with a combination of atezolizumab and bevacizumab than with sorafenib (19.2 months vs. 13.4 months, HR: 0.66, P < 0.001). Similarly, the HIMALAYA study demonstrated better median OS in patients treated with durvalumab and tremelimumab than in those treated with sorafenib (16.4 months vs. 13.8 months, HR: 0.78, P = 0.003)[44,46]. A recent meta-analysis including 2,356 patients diagnosed with HCC and PVT also found higher odds for mortality at 6 months of sorafenib compared to TARE and RT [OR: 2.2, 95%CI: (1.11,4.39)][47]. The possibility of combining these immunotherapy-based regimens with radioembolization is being evaluated in multiple ongoing trials and is discussed in more detail in the subsequent sections of this paper.
Radioembolization in unresectable HCC
Radiation lobectomy and modified radiation lobectomy
These methods aim to expand surgical eligibility to patients with Child-Pugh class A cirrhosis, those with multifocal unilobar HCC, and those who are not currently candidates for resection because of inadequate FLR volume. Radiation lobectomy involves the delivery of a high dose of 90Y to the lobe of the liver with multifocal HCC, with the goal of causing atrophy of the treated lobe and hypertrophy of the FLR. Radiation lobectomy, therefore, offers the dual benefits of hypertrophy of the FLR and containment of the multifocal HCC in the treated lobe. In modified radiation lobectomy, radiation segmentectomy and radiation lobectomy are integrated, leveraging the strengths of both approaches [Figure 4]. This technique can lead to complete tumor necrosis while also contributing to increased FLR volume, ultimately facilitating curative resection in suitable candidates[48].
Figure 4. Modified radiation lobectomy. The single-compartment model is applied to the tumoral segment, followed by lobar infusion.
In one study of radiation lobectomy, a time-dependent increase in FLR volume was observed, with significant hypertrophy evident as early as 1 month after radioembolization. The ideal level of hypertrophy was achieved in most patients at 6 to 9 months, highlighting the importance of careful planning and timing in the context of surgical resection[49]. In the prospective REVoluTion study, which included patients who underwent unilobar radioembolization, hepatic scintigraphy demonstrated a significant increase in metabolic activity in the untreated lobe, suggesting that radioembolization can effectively stimulate compensatory hypertrophy[50]. A systematic review confirmed the ability of radiation lobectomy with radioembolization to induce liver regeneration. The review reported a median kinetic growth rate of 0.7% per week in the contralateral liver lobe after the procedure, highlighting the consistent and predictable nature of this regenerative response[51]. The review also highlighted the clinical benefits of this approach, including an 84% local tumor control rate and a 30% surgical eligibility rate after the procedure. Although these findings were encouraging, another study using matched-pair analysis demonstrated that portal vein embolization induced more rapid and pronounced FLR hypertrophy than radioembolization. This underscores the need for well-designed randomized controlled trials to directly compare these approaches and determine the optimal strategy for liver augmentation in the setting of HCC[52].
Radioembolization for HCC with macrovascular invasion
With careful patient selection, radioembolization can potentially be used to downstage disease in some patients with advanced HCC and macrovascular invasion. In a propensity score-matched analysis including 65 patients with HCC and with intrahepatic portal venous tumor thrombosis, those treated with radioembolization demonstrated longer survival times than those treated with sorafenib (20.3 months vs. 9.1 months; P = 0.001). In addition, disease was successfully downstaged to allow for surgical resection or liver transplant in 24.4% of patients in the radioembolization group[53]. Similarly, in another study, 29.4% of patients with portal vein tumor thrombosis were able to undergo living donor liver transplant with significantly improved survival outcomes compared to patients who did not undergo transplant (5-year survival: 60% vs. 0%; P = 0.03)[54]. The extent of tumor vein invasion and baseline hepatic functions have been shown to be critical prognostic factors in this patient population. One study demonstrated an association between Milan score and OS; patients with a good score demonstrated a median OS of 24.6 months, whereas those with a poor score demonstrated a median OS of 5.9 months [HR: 4.1; 95%CI: (1.2-9.7), P = 0.016][55]. A meta-analysis of multiple retrospective studies evaluating radioembolization for HCC in patients with portal vein thrombosis demonstrated a higher median OS (12.1 months) in patients with Child-Pugh class A disease than in those with Child-Pugh class B (6.1 months). Additionally, patients with branch portal vein tumor thrombus demonstrated an OS of 13.4 months versus an OS of 6.1 months in patients with main portal vein tumor thrombus[56]. These findings suggest that the location of tumor thrombus and hepatic functional reserve are important variables that can affect survival after radioembolization.
Radioembolization plus systemic therapy
Radioembolization plus sorafenib
With the success of the SHARP trial in 2008, sorafenib was recommended as the first-line systemic treatment for patients with BCLC-C disease[57]. Landmark studies including the SARAH, SIRveNib, and SORAMIC trials demonstrated no survival benefit with a combination of radioembolization and sorafenib versus with sorafenib alone[5-7]. However, in patients with adequate liver function, the combination of radioembolization and sorafenib may be beneficial for survival[58]. Currently, there are no data in the literature regarding the combination of other tyrosine kinase inhibitors, such as lenvatinib, with radioembolization.
Radioembolization plus immune checkpoint inhibitors
Early clinical evidence supports the synergistic potential of radioembolization plus immune checkpoint inhibitors (ICIs)[59-62]. The combination of ICIs and radioembolization has shown effectiveness through several mechanisms. Radioembolization induces immunogenic tumor cell death, facilitating the immune system’s recognition of tumor cells. It also recruits and diversifies tumor-infiltrating lymphocytes, increasing the presence of immune cells within the tumor microenvironment. Additionally, radioembolization upregulates markers such as PD-1 on T cells and PD-L1 on tumor cells, enhancing the effectiveness of ICIs. Furthermore, radioembolization elevates levels of proinflammatory cytokines, including IL-1, IL-6, and TNF-α, which stimulate an immune response against the tumor. Radioembolization may also induce an abscopal effect, in which the radiation not only affects the treated tumor but also triggers an immune response that attacks distant tumor sites. This effect is enhanced when radioembolization is combined with ICIs[63].
Multiple studies have demonstrated the benefit of combining radioembolization with an anti-PD-L1 agent (atezolizumab-bevacizumab)[59,64,65]. One of these studies, a retrospective analysis including patients with intermediate to advanced HCC, found that treatment with atezolizumab-bevacizumab combined with radioembolization led to 100% tumor control, a 12-month PFS rate of 66.7%, and a 12-month OS rate of 77.1%[64]. In another retrospective study of 35 patients with HCC who were treated with either radioembolization alone or radioembolization plus concurrent ICIs, PFS was longer in the combined treatment group (11.3 months) than in the radioembolization alone group (5.8 months). The median OS was 14 months for patients treated with radioembolization alone, whereas OS was not reached in patients treated with the combination, indicating that more than half of the patients were still alive at the time of analysis, suggesting a potential OS benefit of the combined treatment[59].
Studies have also demonstrated a survival benefit for radioembolization combined with other ICIs, such as anti-PD-1 agents[61,66,67]. A recent prospective multicenter pilot study included 27 patients with HCC characterized by macrovascular invasion or multifocal/diffuse disease who were treated with pembrolizumab in conjunction with radioembolization. Of these patients, 55.6% were free of progression at 6 months, with a median PFS of 9.95 months and a median OS of 27.3 months[67]. Similarly, the NASIR-HCC study, a single-arm, multicenter, phase 2 trial, included 41 patients with HCC beyond the up-to-7 criteria or with HCC characterized by macrovascular invasion. These patients were treated with radioembolization followed by nivolumab, which led to an ORR of 41.5% [95%CI: (26.3%-57.9%)], a median TTP of 8.8 months [95%CI: (7-10.5)], and a median OS of 20.9 months [95%CI: (17.7-24.1)][61]. A consensus statement regarding the safety of combining radioembolization with systemic treatment indicated that adding an anti-PD-1 agent after radioembolization produces no sign of synergistic toxicity, as evidenced by the low number of treatment-related adverse events reported in prospective studies[68].
Overall, the feasibility of combining 90Y radioembolization and immunotherapy has been supported by Level 4 evidence, with no reports of synergistic toxicity[65,67,68]. However, higher-level evidence for effectiveness recommendation is lacking. Evolving treatment paradigms highlight the need for additional trials and well-designed, prospective, multicenter, real-world studies[68]. Several ongoing clinical trials are investigating the outcomes of combined TARE and ICIs for the treatment of HCC - for instance, a randomized phase II study of atezolizumab and bevacizumab with TARE in patients with unresectable HCC (NCT04541173), durvalumab and tremelimumab in combination with either TARE or DEB-TACE for intermediate stage HCC, (IMMUWIN, NCT04522544), durvalumab and tremelimumab after radioembolization for the treatment of unresectable, locally advanced liver cancer (NCT04605731), TheraSphere with durvalumab and tremelimumab for HCC (ROWAN, NCT05063565), safety and efficacy study of radioembolization in combination with durvalumab in locally advanced and unresectable HCC (SOLID, NCT04124991). The summary of drug classes and their respective roles in the current recommended treatment for HCC is in Table 2.
Summarize systemic treatment for HCC
Drug class | Example of drug name | Current recommendation |
TKI | Sorafenib Lenvatinib Regorafenib Cabozantinib | ● First-line systemic treatment when Atezolizumab-Bevacizumab is not feasible[27] ● Potential hepatotoxicity when combined with TARE[68] ● Second-line systemic treatment[27] |
ICI | Anti-PD-1 agents ● Pembrolizumab ● Nivolumab Anti-PD-L1 agents ● Atezolizumab ● Durvalumab CTLA-4 inhibitors ● Ipilimumab | ● No safety concern, but more clinical trials are needed to proof treatment efficacy[68] |
Anti-VEGF antibody | Bevacizumab Ramucirumab | ● Combined with Atezolizumab as first-line systemic treatment[27] ● Second-line systemic treatment[27] |
CONCLUSION
Radioembolization is a valuable addition to the HCC treatment armamentarium. However, continued research efforts are needed to address the comparative effectiveness of radioembolization versus other established therapies at all stages of HCC, optimal patient selection, and the most effective delivery techniques. Further review regarding the effectiveness of combining radioembolization with newer systemic therapies in cases of advanced HCC is also needed. Such analyses should allow us to optimize patient care in terms of potentially curing disease in patients with early-stage HCC and improving OS and quality of life in patients with advanced disease.
DECLARATIONS
Acknowledgments
The authors thank Megan Griffiths, EPS, for editorial assistance and Harleen Kaur for literature review assistance.
Authors’ contributions
Provided expert guidance on the manuscript’s outline and structure, contributing critical insights that shaped the discussion and conclusions of the research: Gadani S
Took the lead in conceiving and designing the work, conducted data acquisition, and was responsible for drafting and revising the manuscript: Chansangrat J
Availability of data and materials
Not applicable.
Financial support and sponsorship
None.
Conflicts of interest
All authors declared that there are no conflicts of interest.
Ethical approval and consent to participate
Not applicable.
Consent for publication
Not applicable.
Copyright
© The Author(s) 2024.
REFERENCES
1. Vogel A, Meyer T, Sapisochin G, Salem R, Saborowski A. Hepatocellular carcinoma. Lancet 2022;400:1345-62.
2. Sung H, Ferlay J, Siegel RL, et al. Global cancer statistics 2020: GLOBOCAN estimates of incidence and mortality worldwide for 36 cancers in 185 countries. CA Cancer J Clin 2021;71:209-49.
3. Salem R, Gordon AC, Mouli S, et al. Y90 radioembolization significantly prolongs time to progression compared with chemoembolization in patients with hepatocellular carcinoma. Gastroenterology 2016;151:1155-63.e2.
4. Kolligs FT, Bilbao JI, Jakobs T, et al. Pilot randomized trial of selective internal radiation therapy vs. chemoembolization in unresectable hepatocellular carcinoma. Liver Int 2015;35:1715-21.
5. Chow PKH, Gandhi M, Tan SB, et al; Asia-Pacific Hepatocellular Carcinoma Trials Group. SIRveNIB: selective internal radiation therapy versus sorafenib in Asia-Pacific patients with hepatocellular carcinoma. J Clin Oncol 2018;36:1913-21.
6. Vilgrain V, Pereira H, Assenat E, et al; SARAH Trial Group. Efficacy and safety of selective internal radiotherapy with yttrium-90 resin microspheres compared with sorafenib in locally advanced and inoperable hepatocellular carcinoma (SARAH): an open-label randomised controlled phase 3 trial. Lancet Oncol 2017;18:1624-36.
7. Ricke J, Klümpen HJ, Amthauer H, et al. Impact of combined selective internal radiation therapy and sorafenib on survival in advanced hepatocellular carcinoma. J Hepatol 2019;71:1164-74.
8. Lobo L, Yakoub D, Picado O, et al. Unresectable hepatocellular carcinoma: radioembolization versus chemoembolization: a systematic review and meta-analysis. Cardiovasc Intervent Radiol 2016;39:1580-8.
10. Jelic S, Sotiropoulos GC; ESMO Guidelines Working Group. Hepatocellular carcinoma: ESMO clinical practice guidelines for diagnosis, treatment and follow-up. Ann Oncol 2010;21 Suppl 5:v59-64.
11. Kim HC. Radioembolization for the treatment of hepatocellular carcinoma. Clin Mol Hepatol 2017;23:109-14.
12. Clavien PA, Lesurtel M, Bossuyt PM, Gores GJ, Langer B, Perrier A; OLT for HCC Consensus Group. Recommendations for liver transplantation for hepatocellular carcinoma: an international consensus conference report. Lancet Oncol 2012;13:e11-22.
13. Sposito C, Mazzaferro V. The SIRveNIB and SARAH trials, radioembolization vs. sorafenib in advanced HCC patients: reasons for a failure, and perspectives for the future. Hepatobiliary Surg Nutr 2018;7:487-9.
15. Hermann AL, Dieudonné A, Ronot M, et al; SARAH Trial Group. Relationship of tumor radiation-absorbed dose to survival and response in hepatocellular carcinoma treated with transarterial radioembolization with 90Y in the SARAH study. Radiology 2020;296:673-84.
16. Palmer DH, Hawkins NS, Vilgrain V, Pereira H, Chatellier G, Ross PJ. Tumor burden and liver function in HCC patient selection for selective internal radiation therapy: SARAH post-hoc study. Future Oncol 2020;16:4315-25.
17. Makary MS, Bozer J, Miller ED, Diaz DA, Rikabi A. Long-term clinical outcomes of yttrium-90 transarterial radioembolization for hepatocellular carcinoma: a 5-year institutional experience. Acad Radiol 2024;31:1828-35.
18. Aliseda D, Martí-Cruchaga P, Zozaya G, et al. Liver resection and transplantation following yttrium-90 radioembolization for primary malignant liver tumors: a 15-year single-center experience. Cancers 2023;15:733.
19. Gabr A, Riaz A, Johnson GE, et al. Correlation of Y90-absorbed radiation dose to pathological necrosis in hepatocellular carcinoma: confirmatory multicenter analysis in 45 explants. Eur J Nucl Med Mol Imaging 2021;48:580-3.
20. Garin E, Rolland Y, Laffont S, Edeline J. Clinical impact of 99mTc-MAA SPECT/CT-based dosimetry in the radioembolization of liver malignancies with 90Y-loaded microspheres. Eur J Nucl Med Mol Imaging 2016;43:559-75.
21. Garin E, Rolland Y, Edeline J, et al. Personalized dosimetry with intensification using 90Y-loaded glass microsphere radioembolization induces prolonged overall survival in hepatocellular carcinoma patients with portal vein thrombosis. J Nucl Med 2015;56:339-46.
22. Garin E, Tselikas L, Guiu B, et al; DOSISPHERE-01 Study Group. Personalised versus standard dosimetry approach of selective internal radiation therapy in patients with locally advanced hepatocellular carcinoma (DOSISPHERE-01): a randomised, multicentre, open-label phase 2 trial. Lancet Gastroenterol Hepatol 2021;6:17-29.
23. Lam M, Garin E, Maccauro M, et al. A global evaluation of advanced dosimetry in transarterial radioembolization of hepatocellular carcinoma with yttrium-90: the TARGET study. Eur J Nucl Med Mol Imaging 2022;49:3340-52.
24. Levillain H, Bagni O, Deroose CM, et al. International recommendations for personalised selective internal radiation therapy of primary and metastatic liver diseases with yttrium-90 resin microspheres. Eur J Nucl Med Mol Imaging 2021;48:1570-84.
25. Salem R, Padia SA, Lam M, et al. Clinical, dosimetric, and reporting considerations for Y-90 glass microspheres in hepatocellular carcinoma: updated 2022 recommendations from an international multidisciplinary working group. Eur J Nucl Med Mol Imaging 2023;50:328-43.
26. van Doorn DJ, Hendriks P, Burgmans MC, et al. Liver decompensation as late complication in HCC patients with long-term response following selective internal radiation therapy. Cancers 2021;13:5427.
27. Reig M, Forner A, Rimola J, et al. BCLC strategy for prognosis prediction and treatment recommendation: The 2022 update. J Hepatol 2022;76:681-93.
28. Vitale A, Trevisani F, Farinati F, Cillo U. Treatment of hepatocellular carcinoma in the precision medicine era: from treatment stage migration to therapeutic hierarchy. Hepatology 2020;72:2206-18.
29. Salem R, Johnson GE, Kim E, et al. Yttrium-90 radioembolization for the treatment of solitary, unresectable HCC: the LEGACY study. Hepatology 2021;74:2342-52.
30. Takayama T, Hasegawa K, Izumi N, et al. Surgery versus radiofrequency ablation for small hepatocellular carcinoma: a randomized controlled trial (SURF Trial). Liver Cancer 2022;11:209-18.
31. Uhlig J, Sellers CM, Stein SM, Kim HS. Radiofrequency ablation versus surgical resection of hepatocellular carcinoma: contemporary treatment trends and outcomes from the United States National Cancer Database. Eur Radiol 2019;29:2679-89.
32. Kim E, Sher A, Abboud G, et al. Radiation segmentectomy for curative intent of unresectable very early to early stage hepatocellular carcinoma (RASER): a single-centre, single-arm study. Lancet Gastroenterol Hepatol 2022;7:843-50.
33. Lewandowski RJ, Gabr A, Abouchaleh N, et al. Radiation segmentectomy: potential curative therapy for early hepatocellular carcinoma. Radiology 2018;287:1050-8.
34. De la Garza-Ramos C, Montazeri SA, Croome KP, et al. Radiation segmentectomy for the treatment of solitary hepatocellular carcinoma: outcomes compared with those of surgical resection. J Vasc Interv Radiol 2022;33:775-85.e2.
35. Arndt L, Villalobos A, Wagstaff W, et al. Evaluation of medium-term efficacy of Y90 radiation segmentectomy vs percutaneous microwave ablation in patients with solitary surgically unresectable < 4 cm hepatocellular carcinoma: a propensity score matched study. Cardiovasc Intervent Radiol 2021;44:401-13.
36. Mosenthal M, Adams W, Cotler S, et al. Locoregional therapies for hepatocellular carcinoma prior to liver transplant: comparative pathologic necrosis, radiologic response, and recurrence. J Vasc Interv Radiol 2024;35:506-14.
37. Dhondt E, Lambert B, Hermie L, et al. 90Y radioembolization versus drug-eluting bead chemoembolization for unresectable hepatocellular carcinoma: results from the TRACE phase II randomized controlled trial. Radiology 2022;303:699-710.
38. Chung SW, Cho H, Shin H, et al. Transarterial chemoembolization as an alternative to radioembolization is associated with earlier tumor recurrence than in radioembolization-eligible patients. Front Oncol 2023;13:1081479.
39. Brown AM, Kassab I, Massani M, et al. TACE versus TARE for patients with hepatocellular carcinoma: overall and individual patient level meta analysis. Cancer Med 2023;12:2590-9.
40. Chow R, Simone CB 2nd, Jairam MP, Swaminath A, Boldt G, Lock M. Radiofrequency ablation vs radiation therapy vs transarterial chemoembolization vs yttrium 90 for local treatment of liver cancer - a systematic review and network meta-analysis of survival data. Acta Oncol 2022;61:484-94.
41. Young S, Sanghvi T, Ragulojan R, et al. Local recurrence following a complete radiologic response in hepatocellular carcinoma patients: comparison of transarterial chemoembolisation and transarterial radioembolisation. Clin Radiol 2024;79:371-7.
42. Wagenpfeil J, Kupczyk PA, Bruners P, et al. Outcome of transarterial radioembolization in patients with hepatocellular carcinoma as a first-line interventional therapy and after a previous transarterial chemoembolization. Front Radiol 2024;4:1346550.
43. Hund HC, Du L, Matsuoka L, et al. Effect of previous transarterial chemoembolization on survival and toxicity after yttrium-90 transarterial radioembolization of hepatocellular carcinoma in the radiation-emitting SIR-spheres in nonresectable liver tumor registry. J Vasc Interv Radiol 2023;34:2147-54.e2.
44. Cheng AL, Qin S, Ikeda M, et al. Updated efficacy and safety data from IMbrave150: atezolizumab plus bevacizumab vs. sorafenib for unresectable hepatocellular carcinoma. J Hepatol 2022;76:862-73.
45. Finn RS, Qin S, Ikeda M, et al; IMbrave150 Investigators. Atezolizumab plus bevacizumab in unresectable hepatocellular carcinoma. N Engl J Med 2020;382:1894-905.
46. Abou-Alfa GK, Lau G, Kudo M, et al. Tremelimumab plus durvalumab in unresectable hepatocellular carcinoma. NEJM Evid 2022;1:EVIDoa2100070.
47. Liu B, Grindrod N, Meyers BM, et al. Treatment modalities to manage hepatocellular carcinoma patients with portal vein thrombosis: a systematic review and meta-analysis. Ann Palliat Med 2023;12:1165-74.
48. Gabr A, Riaz A, Mouli S, et al. Modified radiation lobectomy: an evolving paradigm to convert patients to liver resection candidacy. Semin Intervent Radiol 2019;36:343-8.
49. Vouche M, Lewandowski RJ, Atassi R, et al. Radiation lobectomy: time-dependent analysis of future liver remnant volume in unresectable liver cancer as a bridge to resection. J Hepatol 2013;59:1029-36.
50. Garlipp B, Amthauer H, Kupitz D, et al. The impact of unilateral 90Y-radioembolization on functional changes in the contralateral hepatic lobe: the prospective, open-label radioembolization, volumetry, and liver function measurements (REVoluTion) study. Ann Surg Open 2021;2:e095.
51. Birgin E, Rasbach E, Seyfried S, et al. Contralateral liver hypertrophy and oncological outcome following radioembolization with 90Y-microspheres: a systematic review. Cancers 2020;12:294.
52. Garlipp B, de Baere T, Damm R, et al. Left-liver hypertrophy after therapeutic right-liver radioembolization is substantial but less than after portal vein embolization. Hepatology 2014;59:1864-73.
53. Tabone M, Calvo A, Russolillo N, et al. Downstaging unresectable hepatocellular carcinoma by radioembolization using 90-yttrium resin microspheres: a single center experience. J Gastrointest Oncol 2020;11:84-90.
54. Serenari M, Cappelli A, Cucchetti A, et al. Deceased donor liver transplantation after radioembolization for hepatocellular carcinoma and portal vein tumoral thrombosis: a pilot study. Liver Transpl 2021;27:1758-66.
55. Bargellini I, Scalise P, Boni G, et al. Yttrium-90 radioembolization for hepatocellular carcinoma with portal vein invasion: validation of the milan prognostic score. J Vasc Interv Radiol 2020;31:2028-32.
56. Jia Z, Jiang G, Tian F, Zhu C, Qin X. A systematic review on the safety and effectiveness of yttrium-90 radioembolization for hepatocellular carcinoma with portal vein tumor thrombosis. Saudi J Gastroenterol 2016;22:353-9.
57. Llovet JM, Ricci S, Mazzaferro V, et al; SHARP Investigators Study Group. Sorafenib in advanced hepatocellular carcinoma. N Engl J Med 2008;359:378-90.
58. Ricke J, Schinner R, Seidensticker M, et al. Liver function after combined selective internal radiation therapy or sorafenib monotherapy in advanced hepatocellular carcinoma. J Hepatol 2021;75:1387-96.
59. Mejait A, Roux C, Soret M, et al. Enhanced therapeutic outcomes with atezolizumab-bevacizumab and SIRT combination compared to SIRT alone in unresectable HCC: a promising approach for improved survival. Clin Res Hepatol Gastroenterol 2024;48:102282.
60. Tai D, Loke K, Gogna A, et al. Radioembolisation with Y90-resin microspheres followed by nivolumab for advanced hepatocellular carcinoma (CA 209-678): a single arm, single centre, phase 2 trial. Lancet Gastroenterol Hepatol 2021;6:1025-35.
61. de la Torre-Aláez M, Matilla A, Varela M, et al. Nivolumab after selective internal radiation therapy for the treatment of hepatocellular carcinoma: a phase 2, single-arm study. J Immunother Cancer 2022;10:e005457.
62. Lee YB, Nam JY, Cho EJ, et al. A phase I/IIa trial of yttrium-90 radioembolization in combination with durvalumab for locally advanced unresectable hepatocellular carcinoma. Clin Cancer Res 2023;29:3650-8.
63. Torkian P, Haghshomar M, Farsad K, Wallace S, Golzarian J, Young SJ. Cancer immunology: impact of radioembolization of hepatocellular carcinoma on immune response modulation. AJR Am J Roentgenol 2023;220:863-72.
64. Yu Q, Wang Y, Ungchusri E, et al. Combination of transarterial radioembolization with atezolizumab and bevacizumab for intermediate and advanced staged hepatocellular carcinoma: a preliminary report of safety and feasibility. J Interv Med 2023;6:187-93.
65. Villalobos A, Dabbous HH, Little O, et al. Safety and efficacy of concurrent atezolizumab/bevacizumab or nivolumab combination therapy with yttrium-90 radioembolization of advanced unresectable hepatocellular carcinoma. Curr Oncol 2023;30:10100-10.
66. Zhan C, Ruohoniemi D, Shanbhogue KP, et al. Safety of combined yttrium-90 radioembolization and immune checkpoint inhibitor immunotherapy for hepatocellular carcinoma. J Vasc Interv Radiol 2020;31:25-34.
67. Yu S, Yu M, Keane B, et al. A pilot study of pembrolizumab in combination with Y90 radioembolization in subjects with poor prognosis hepatocellular carcinoma. Oncologist 2024;29:270-e413.
Cite This Article
How to Cite
Download Citation
Export Citation File:
Type of Import
Tips on Downloading Citation
Citation Manager File Format
Type of Import
Direct Import: When the Direct Import option is selected (the default state), a dialogue box will give you the option to Save or Open the downloaded citation data. Choosing Open will either launch your citation manager or give you a choice of applications with which to use the metadata. The Save option saves the file locally for later use.
Indirect Import: When the Indirect Import option is selected, the metadata is displayed and may be copied and pasted as needed.
About This Article
Special Issue
Copyright
Data & Comments
Data
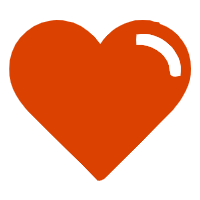
Comments
Comments must be written in English. Spam, offensive content, impersonation, and private information will not be permitted. If any comment is reported and identified as inappropriate content by OAE staff, the comment will be removed without notice. If you have any queries or need any help, please contact us at [email protected].