Next-generation sequencing of the athletic gut microbiota: a systematic review
Abstract
Aim: There is growing evidence that physical activity modulates gut microbiota composition through complex interactions between diet and microbial species. On the other hand, next-generation sequencing techniques include shotgun metagenomics and 16S amplicon sequencing. These methodologies allow a comprehensive characterisation of microbial communities of athletes from different disciplines as well as non-professional players and sedentary adults exposed to training. This systematic review summarises recent applications of next-generation sequencing to characterise the athletic gut microbiome.
Methods: A systematic review of microbiome research was performed to determine the association of microbiota composition profiles with sports performance.
Results: Bibliographic analysis revealed the importance of a novel research trend aiming at deciphering the associations between individual microbial species and sports performance. In addition, literature review highlighted the role of butyrate-producing bacteria such as Anaerostipes hadrus, Clostridium bolteae, Faecalibacterium prausnitzii, Roseburia hominis and unidentified species belonging to Clostridiales, Lachnospiraceae and Subdoligranulum species in gut health and sports performance across several disciplines. Interestingly, metabolic activities of Prevotella copri and Veillonella atypica involved in branched amino acid and lactate metabolism may contribute to reducing muscular fatigue. Other microbial metabolic pathways of interest involved in carbohydrate metabolism showed increased proportions in athletes´ metagenomes.
Conclusion: Future research will aim at developing personalised nutrition interventions to modulate key species associated with certain components of exercise.
Keywords
INTRODUCTION
There is growing evidence that physical activity modulates gut microbiota composition[1]. In this sense, human microbiome is sensitive to the physiological changes associated with training, including increased intestinal permeability and altered profiles of inflammatory markers. It has been described that gut microbiota regulates the energetic balance and the inflammatory, redox, and hydration status. Specifically, physical activity may lead to an increase in microbiota diversity[2]. These increased microbial diversity estimators include α-diversity, indicating the number of clades in one sample within an individual[3]. Previous studies suggest that athlete microbiota shows higher proportions of certain members of the Firmicutes phylum and the genus Akkermansia[3,4]. The interactions between diet, exercise, and gut microbiota in athletes have been reported[5]. Common dietary strategies and recommendations for elite athletes are primarily based on high protein and simple carbohydrate intake and low consumption of plant polysaccharides. However, other factors that should be considered in these recommendations include the sports discipline (that may require specific physiological and metabolic demands), sex, nutrient sources with different amino acid contents, and sports periodization (involving pre- and post-training and pre- and post-competition phases). Some of these dietary recommendations may result in reduced microbiota diversity and functionality[5,6]. It has been reported that elite athletes suffer from psychological and gastrointestinal conditions associated with gut microbiome[6]. Administration of specific probiotic strains, prebiotics and synbiotic combinations could promote a healthy microbiota, which may exert a direct influence on the hypothalamus-pituitary-adrenal axis in athletes[2,5,6].
On the other hand, computational tools involving machine learning have been used to predict exercise-induced alterations in the microbiota of athletes[7]. These pattern-recognition algorithms might be especially useful to distinguish between responders and non-responders to specific nutrients or supplements in order to develop personalised nutrition strategies aiming at improving sports performance. Similarly, machine learning could be of great interest to rationally design prebiotic interventions targeting species and strains associated with health benefits[8], and perform systematic literature reviews dealing with microbial carbohydrate metabolism[9]. Systematic reviews in the field of gut-microbiota-brain axis study revealed the associations between physical and emotional stress during exercise in animal models. This fact may induce changes in gut microbiome including microbial clades involved in the degradation of intestinal mucus and immune response[6]. Other systematic reviews suggested that probiotic administration may improve athletes’ general health and performance while controlling inflammation and redox levels[3]. To our knowledge, no systematic review has addressed the association of gut microbes with sports performance with an emphasis on metagenomics. In this regard, shotgun sequencing shows several advantages compared to amplicon sequencing and culture depending techniques, including the taxonomic identification of non-culturable microbes at species and strain level, the recovery of microbial metagenome-assembled genomes and the study of microbial metabolic pathways [Table 1]. Therefore, this systematic review provides an overview of next-generation sequencing of the athletic microbiota and summarises the main genera and species associated with physical performance. Microbial clades associated with physical activity in non-professional players and sedentary adults are discussed.
Advantages and disadvantages of culture depending techniques, amplicon and shotgun sequencing
Shotgun sequencing | Amplicon sequencing | Culture depending techniques | |
Advantages | Identification of non-culturable microorganisms | Identification of non-culturable microorganisms | Possibility to perform phenotypic studies |
Taxonomic identification at species and strain level | Taxonomic identification at genus level | Low cost per sample | |
Recovery of microbial metagenome-assembled genomes | Lower cost per sample | ||
Study of microbial metabolic pathways | Lower concentrations of DNA needed | ||
Disadvantages | High cost per sample | Microbes cannot be identified at species and strain level | Limited to culturable microorganisms |
High concentrations of DNA needed | Microbial metabolic pathways cannot be studied | Less accurate view of microbial biodiversity |
METHODS
To select the articles included in this systematic review, an advanced search of original articles listed in two different databases (Scopus and Web of Science) was first performed. This search contained two conceptual terms involving athletes and metagenomics while excluding review articles and book articles. For this purpose, an advanced search option with boolean operators was used. The format of this initial search was adjusted for each database [Table 2]. It should be noted that word roots and synonyms were introduced in the metagenomics conceptual term in order to retrieve the maximum number of results (26 and 18 for Scopus and Web of Science, respectively).
Advanced queries introduced Scopus and Web of Science databases
Bibliographic search (n = 27) | |
Database | Query (boolean string) |
Scopus | TITLE-ABS-KEY [(athlete AND metagen*) AND NOT (review OR chapter)] |
Web of science | TI = [(athlete AND metagen*) NOT (review OR chapter)] OR AB = [(athlete AND metagen*) NOT (review OR chapter)] OR KP = [(athlete AND metagen*) NOT (review OR chapter)] |
Results from individual searches were merged using revtools v0.4.1 package implemented in R v3.6.2[10]. Duplicated articles were filtered by title using synthesisr v0.3.0 library[11]. Once bibliographic records were deduplicated, studies dealing with fecal samples analysed by shotgun metagenomics or 16S amplicon sequencing were selected. As a result, up to 16 original articles were retrieved. Figure 1 illustrates the Preferred Reporting Items for Systematic reviews and Meta-Analyses (PRISMA) object describing the article selection process.
Figure 1. Preferred Reporting Items for Systematic reviews and Meta-Analyses (PRISMA) flow diagram illustrating the selection of articles describing the association of gut microbes with sports and exercise performance assessed by shotgun metagenomics or 16S amplicon sequencing.
Bibliographical analysis of screened articles was performed using several computational tools. These tools allow for summarising all scientific publications generated in one field to highlight the relationships between common terms and keywords. In this sense, novel research trends can be monitored by computing mathematical models like keyword co-occurrence networks[12]. In this regard, manuscript keywords were extracted and new keywords were generated from article titles and abstracts. These new keywords reflect those terms that are present in at least four article titles/abstracts and were generated using Rapid Automatic Keyword Extraction (RAKE) method implemented in litsearchr v1.0.0 package[12]. In addition, a co-occurrence network of article keywords and new keywords extracted from article titles and abstracts was computed using litsearchr v1.0.0 and ggrapgh v2.0.1 packages[12,13].
RESULTS
Bibliographic analysis
A total of 16 original articles reported the applications of shotgun metagenomics or 16S amplicon sequencing for the analysis of fecal samples of athletes and individuals practicing sports
Articles (n = 16) reporting the applications of next-generation sequencing, including both shotgun metagenomics and 16S amplicon sequencing of fecal samples on athlete microbiome research. Articles describing the association of gut microbiota with the exercise performance of non-athlete individuals were also considered. Some articles (n = 12) reported the associations between specific microbial clades and sports performance
Database | Study | Technique | Participants | Sex | Age range | n | Taxa associated with sports performance |
Sequencing experiments describing microbial taxa associated with sports performance (n = 12) | |||||||
Scopus, WOS | Barton et al.[14] | Shotgun | Professional rugby players (n = 40) and controls (n = 46) | Male | - | 86 | Akkermansia, Erysipelotrichaceae incertae sedis |
Scopus, WOS | Cronin et al.[15] | Shotgun | Healthy sedentary adults (n = 90) with a short-term exercise regime | Male and female | 18-40 | 90 | Prevotella copri |
WOS | Kostic[16] | Shotgun | Marathon runners, ultramarathon runners, Olympic-caliber rowers and sedentary controls | Male and female | - | 50 | Veillonella |
Scopus, WOS | Keohane et al.[17] | Shotgun | Athletes who completed an unsupported transatlantic row (n = 4) | Male | - | 4 | Dorea longicatena, Prevotella copri, Roseburia hominis, unclassified members of Subdoligranulum |
Scopus, WOS | Scheiman et al.[18] | 16S and Shotgun | Marathon athletes (n = 15) and sedentary controls (n = 10) | Male and female | - | 25 | Veillonella atypica |
Scopus, WOS | Petersen et al.[19] | Shotgun | Competitive cyclists (n = 33) | Male and female | 19-49 | 33 | Akkermansia, Bacteroides, Eubacterium, Prevotella, Methanobrevibacter smithii, Ruminococcus |
Scopus, WOS | O’Donovan et al.[20] | Shotgun | International level athletes (n = 37) across 16 different sports | Male and female | - | 37 | Streptococcus suis, Clostridium bolteae, Lactobacillus phage LfeInf, Anaerostipes hadrus in sports with moderate dynamic component. Bifidobacterium animalis, Lactobacillus acidophilus, Prevotella intermedia, Faecalibacterium prausnitzii in sports with high dynamic and low static components. Bacteroides caccae in sports with high dynamic and static components |
Scopus | Fart et al.[21] | Shotgun | Physically active senior orienteers (n = 28) and community-dwelling older adults (n = 70) | Male and female | 67-76 | 98 | Faecalibacterium prausnitzii |
Scopus, WOS | Kulecka et al.[22] | 16S | Marathon runners (n = 11), cross-country skiers | Male and female | 14-72 | 68 | Lachnospiraceae, Prevotella |
Scopus, WOS | Moitinho-Silva et al.[23] | 16S | Healthy sedentary adults exposed to endurance and strength training and controls (n = 42) | Male and female | 20-45 | 42 | Coprococcus, Parasutterella, Ruminococcaceae |
Scopus | Fukuchi et al.[24] | 16S | Endurance athletes (n = 13) | Male and female | 19-21 | 13 | Firmicutes |
Scopus | Han et al.[25] | 16S | Rowing athletes (n = 19) | Female | 12-26 | 19 | Firmicutes, Bacteroidetes, Proteobacteria and Actinobacteria in all athletes. Faecalibacterium and unclassified members of Clostridiales and Lachnospiraceae in adult elite athletes. Bacteroides in young elite athletes |
Sequencing experiments describing athlete microbiota but no microbial taxa associated with sports performance (n= 4) | |||||||
Scopus, WOS | Genç[26] | Shotgun | Professional athletes (n = 5), amateur athletes | Male | 18-24 | 15 | - |
Scopus, WOS | O’Donovan et al.[27] | 16S and Shotgun | Cricket players (n = 21) | Male and female | - | 21 | - |
Scopus | Axelrod et al.[28] | Shotgun | Endurance athletes (n = 7) | - | 18-45 | 7 | - |
Scopus | Özkan et al.[29] | 16S | Professional football players (n = 5), amateur football players (n = 5) and sedentary controls (n = 5) | Male | 18-24 | 15 | - |
Network modelling highlighted the co-occurrence of several terms in these scientific publications: dietary intake, physical activity, and taxonomic and functional profiles of the microbiota. In this regard, previous authors suggested that training modulates microbial diversity and microbial metabolism[4,5]. Moreover, a high degree of compliance with dietary recommendations for athletes greatly affects gut microbiota functionality and sports performance[5,6]. Interestingly, this mathematical model suggests the association of specific taxonomic clades with sports performance indicators such as endurance in professional athletes. It has been reported that athletes show increased microbiome diversity estimators like α-diversity as well as microbial pathways and fecal metabolite production compared to controls[2,3,14].
The importance of each term represented in the co-occurrence network was calculated considering the number of other terms with which it appears. Some relevant terms highlight the role of physical activity on the health status, taxonomic and functional profiles of the gut microbiota. It should be noted that the number of microbiome studies dealing with sports performance of elite athletes based on parameters like endurance has incremented in the last five years. In contrast, studies published before tend to focus on the relationship between gut microbes and general dietary and lifestyle factors in professional athletes.
To assess the potential impact of co-variables in the network analysis, Spearman correlation coefficients between terms were calculated using caret package[30]. Then, terms showing correlation coefficients greater than 0.6 were determined. These terms were “intestinal microbiota”, “metabolic” and “specific”. It should be noted that these co-variables do not have a great impact on keyword relationships established by the co-occurrence network. On the other hand, synonyms and terms describing very similar concepts were excluded from the network to avoid bias.
The association of gut microbes with sports performance
Among the original articles included in this systematic review, nine and five microbiome studies involved shotgun metagenomics and amplicon 16S sequencing, respectively. Moreover, two studies involved both metagenomics and 16S sequencing. These next-generation sequencing experiments involve microbiota analysis of fecal samples of professional athletes and physically active individuals [Table 3]. Specifically, participant recruitment involved elite athletes from several disciplines, including: rugby players[14], marathon runners (professional and non-professional)[16,18,22], endurance athletes[24,28], competitive cyclists[19], rowers[16,17,25], skiers[22], football and cricket players[27,29], and other athletes across 16 different sports[20]. In addition, some studies characterised the microbiota of physically active senior orienteers[21], as well as healthy but sedentary adults exposed to endurance and strength training[23], and short-term exercise regime[15]. It should be noted that most of these studies compared microbiota profiles of athletes and physically-active participants to sedentary controls [Table 3]. Most studies included both male and female participants, while four and one focused on male and female athletes, respectively [Table 3]. It should be noted that these authors did not determine potential taxonomic differences according to the sex of individuals. In general, the age of participants comprising young and elite athletes ranged from 18 to 40.
A total of 12 articles described the association of specific microbial clades with sports and exercise performance [Table 3]. Figure 2 provides a schematic representation of characteristic microbial clades found in the microbiota of athletes across different disciplines as well as sedentary adults exposed to exercise regime. As can be seen, Figure 2 summarizes characteristic taxonomic profiles reported in the selected studies and presented in Table 3. In this sense, Firmicutes phylum including Ruminococcaceae or Faecalibacterium was increased in endurance athletes compared to physically inactive individuals[1,24]. Moreover, Firmicutes/Bacteroidetes ratio is correlated with obesity as well as controlling fungal occupancy in athletes[24,31]. In this regard, bacteria from the phyla Firmicutes and Bacteroidetes represent 90% of the gut microbiota and Firmicutes/Bacteroidetes ratio has been associated with maintaining normal intestinal homeostasis. Increased or decreased Firmicutes/Bacteroidetes ratio may lead to various pathologies. Gut microbiota unbalance usually observed with obesity, including increases in the abundance of specific Firmicutes or Bacteroidetes species[31]. On the other hand, high Akkermansia abundances were found in the microbiota of professional rugby players[14] and competitive cyclists[19]. Low-abundance Akkermansia species such as A. muciniphila comprise mucin-degrading microorganisms that are negatively correlated with obesity and metabolic syndrome[19]. In contrast, Veillonella genus and Veillonella atypica might be slightly increased in marathon runners and rowers, although these increments are not statistically significant[16,18]. It has been suggested that lactate is the primary source of energy for V. atypica. Blood lactate resulting from muscle activity is associated with fatigue, and its concentration and accumulation depend on various factors, including exercise intensity, load and density. Scheiman et al. demonstrated in a murine model that plasma lactate may reach the intestinal lumen, where it may also be metabolized by V. atypica[18]. The relationship between physical activity levels and the prevalence of Veillonella involved in metabolic, protective, structural, and histological functions was also reported by Dorelli et al., and Manor et al.[1,32]. The proposed mechanism of action has been proposed: Veillonella species metabolize lactate into acetate and propionate via the methylmalonyl-CoA pathway. Unlike V. atypica, many other microbes are capable of utilizing lactate through lactate dehydrogenase, but do not possess the full pathway to convert lactate into propionate. In this sense, systemic lactate resulting from muscle activity during exercise may enter the gastrointestinal lumen and is metabolized by Veillonella into propionate in the colon. Gut colonization of Veillonella promotes the Cori cycle by providing an alternative lactate-processing mechanism whereby systemic lactate is converted into SCFAs that re-enter the circulation. SCFAs are absorbed in the sigmoid and rectal region of the colon and enter circulation via the pelvic plexus, bypassing the liver and draining via the vena cava to reach the systemic circulation directly. As a consequence, microbial SCFAs derived from lactate improve athletic performance[18].
Figure 2. Schematic representation of characteristic microbial clades and metabolic activities with a higher representation in the microbiota of athletes across different disciplines as well as sedentary adults exposed to exercise regime. *Microbial taxa showing significantly higher abundances in the microbiota of athletes or sedentary participants exposed to exercise regime compared to sedentary controls.
Similarly, Prevotella copri was increased in rowers who completed an east–west transatlantic rowing race[17], and sedentary adults with a short-term exercise regime involving combined aerobic and resistance training[15]. It has been reported that gene expression of metabolic pathways of P. copri involved in L-lysine is increased after ultra-endurance exercise. This essential amino acid plays a critical role in reducing muscular fatigue and contributes to muscular integrity. Furthermore, microbial derived lysine contributes to the human body protein pool[17].
Other microbial clades that may be characteristic of the microbiota of athletes include unidentified taxa belonging to Erysipelotrichaceae in rugby players [Table 3]. These unclassified microorganisms are positively correlated to phenylacetylglutamine derived from phenylalanine. This compound is increased in athletes and associated with a lean phenotype[14]. However, different rugby player positions require different physiological and physical demands. High abundances of several bacterial genera (Bacteroides, Eubacterium, Prevotella and Ruminococcus) were also found in competitive cyclists compared to sedentary adults [Table 3]. These genera are correlated with branched-chain amino acid and carbohydrate metabolism pathways. It has been suggested that upregulation of branched-chain amino acids biosynthesis leads to a decrease in exercise-induced muscle fatigue and promotes muscle-protein synthesis[19]. Similarly, several clades like Dorea longicatena, Faecalibacterium prausnitzii, Roseburia hominis and unclassified species belonging to Subdoligranulum were increased in rowers throughout a race as well as physically active senior orienteers compared to the general older adult population[17,21]. F. prausnitzii, R. hominis and Subdoligranulum are butyrate producers that contribute to reducing gut inflammation and oxidative stress. It should be noted that butyrate is the main nutrient for colonocytes that exert an anti-inflammatory activity[21]. With regard to Dorea longicatena, this taxon is associated with insulin sensitivity[17,33]. In addition, high abundances of Lachnospiraceae were determined in marathon runners and skiers[22], while Coprococcus, Parasutterella genera and Ruminococcaceae family were increased in physically active individuals[23].
Some studies reported characteristic differences in the microbiota profiles of young and adult elite athletes in order to monitor the potential of elite athletic candidates [Table 3]. In this sense, Han et al. determined the prevalence of Firmicutes, Bacteroidetes, Proteobacteria and Actinobacteria in the fecal microbiota of rowing athletes[25]. However, unidentified species belonging to Clostridiales and Lachnospiraceae, as well as Faecalibacterium genus, showed higher abundances in metagenomes from elite adult participants than young elite athletes [Table 3]. These taxa are likely to produce short-chain fatty acids and may be associated with exercise-induced butyrate concentrations that may contribute to improving sports performance[25]. It should be noted that Lachnospiraceae increments are strongly associated with fiber consumptions, although general dietary recommendations for athletes are characterised by low intake of non-amylaceous polysaccharides[5,6]. In contrast, Bacteroides was enriched in the microbiota of young elite athletes [Table 3]. High abundances of oxidative stress tolerant clades may improve performance in competitive sports. The microbiota of elite athletes may also show increased proportions of microbial metabolic pathways involved in carbohydrate metabolism and multiple sugar transport systems compared to young non-elite athletes[25].
Efforts to establish associations between individual microbial species and different types of physical activities have also been made [Table 3]. In this regard, Streptococcus suis, Clostridium bolteae, Lactobacillus phage LfeInf and Anaerostipes hadrus were associated with moderate dynamic components of exercise. It should be noted that dynamic components of exercise involve aerobic exercise, while static components of exercise are performed by increasing tension in a muscle while keeping its length constant. Therefore, sports disciplines can be classified into nine different categories according to the contributions (high, moderate or low) of both components[34].
It has been reported that S. suis showed high proportions of metabolic pathways involved in the fermentation of sugar alcohols, while C. bolteae and A. hadrus may produce butyrate to promote gut homeostasis and anti-inflammatory effects[20]. Other clades, including Bifidobacterium animalis, Lactobacillus acidophilus, Prevotella intermedia and butyrate-producing species such as Faecalibacterium prausnitzii, were associated with high dynamic and low static components of exercise. On the contrary, Bacteroides caccae was enriched in athletes from disciplines involving high dynamic and static components of exercise [Table 3].
Most articles selected in this systematic review do not involve intervention studies. In this regard, characteristic patterns in the fecal microbiota of athletes were elucidated at taxonomic and functional levels [Figure 2]. In addition, some studies report differences in the metabolomic profiles of participants. In this sense, differences in the composition and functional capacity of the gut microbiome of international-level athletes from several disciplines have been determined[20]. It has been described that fecal microbiota samples of professional rugby players and sedentary controls show even greater separation at the functional metagenomic and metabolomic than at compositional levels[14]. Similarly, microbial diversity, including butyrate-producing species, increased throughout the ultra-endurance events such as transoceanic rowing races. The functional potential of bacterial species involved in specific amino and fatty acid biosynthesis also increased[17]. Additional studies dealing with rowing athletes report that ATP metabolism, multiple sugar transport systems and carbohydrate metabolism are enriched in the microbial community of these athletes[25]. Differences in functional bile acid and histidine metabolism also differentiate power athletes from sedentary controls, while galactose metabolism markers differentiate endurance athletes from controls. Moreover, D-alanine and primary bile acid biosynthesis differentiate power and endurance athletes from controls. Scheiman et al. and Kostic reported that every gene in a major pathway metabolizing lactate to propionate is at higher relative abundance postexercise in marathon runners[18,16]. On the other hand, an increment in the abundance of Prevotella was correlated with a number of amino acid and carbohydrate metabolism pathways in cyclists, including branched-chain amino acid metabolism[19]. In contrast, some studies report characteristic microbiota patterns mainly at taxonomic level, including increased abundances of Faecalibacterium prausnitzii in physically active senior orienteers[21].
Some studies report characteristic microbial-derived metabolic profiles of athletes from different disciplines. In this regard, cis-aconitate, succinic acid and lactate in urine samples and creatinine in faeces were found to be significantly different between groups of sports. These differences were evident despite the absence of significant differences in diet[20]. In addition, microbial-derived SCFAs are enhanced within rugby players and their gut microbiota was predominantly correlated with creatine kinase (CK), total bilirubin and total energy intake[14].
Dietary factors that may confound the association between exercise and the abundance of microbial taxa of interest were considered in several of these studies[16,18]. Other studies report the assessment of macronutrient intake per day to enhance inter-individual comparison[21] as well as quantitative diet evaluation[22]. It has been reported that the versatility of the microbial community of athletes, which might affect their performance, is associated with dietary factors[25]. However, other studies report the lack of in-depth dietary analysis and a matching non-athlete cohort (i.e., control group comprising non-athlete participants with similar demographic characteristics and exposed to the same intervention)[19].
On the contrary, three of the selected articles comprised intervention studies. In this regard, Cronin et al. demonstrated modest changes in gut microbial composition and function in healthy but sedentary adults exposed to short-term exercise regime, with and without concurrent daily whey protein consumption[15]. An association between whey protein intake and the β-diversity of the adult gut virome was found, while no major changes in the functional activity of the gut microbiota were determined, with the exception of urinary levels of trimethylamine N-oxide (TMAO) and phenylacetylglycine (PAG) excretion. Similarly, Moitinho-Silva et al. elucidated taxonomic differences, a significant increase in lymphocytes and a decrease in mean corpuscular haemoglobin concentration in healthy sedentary adults exposed to strength training[23]. No change in dietary patterns towards the end of the intervention period and after it was observed. Finally, Fukuchi et al. investigated the effects of both official competition and a multi-strain lactic acid bacteria-fermented soymilk extract in taxonomy and urine metabolites in endurance athletes[24]. Changes in urinary metabolites included a significant reduction in yeast and fungal markers, neurotransmitters, and mitochondrial metabolites including the tricarboxylic acid (TCA) cycle. Tricarballylic acid was positively correlated with the ratio of Firmicutes, while Parabacteroides distasonis was negatively correlated with several urinary metabolites, including 3-metylglutaconic, vanillylmandelic, quinolinic and kynurenic acids and thymine[24].
Applications of next-generating sequencing technologies to assess the relationships between gut microbiota composition and sports performance have been summarised. Computational methods for text processing and bibliographic analysis revealed recent research trends focusing on the impact of microbial diversity and metabolic pathways on different components of physical activity. These studies highlight the role of butyrate-producing bacteria in promoting gut health and contributing to an optimal composition of the athletic microbiota.
DISCUSSION
Mathematical modelling for text processing opens new possibilities for evidence synthesis in microbiome research. This could be of special interest to monitor research trends in emerging fields like metagenomics applied to sports science. However, this novel approach has several limitations. In this sense, the authors from selected articles may use general and unprecise terms to describe their findings, making keyword co-occurrence analysis difficult. In addition, unprecise terminology in the field of sports science, including “performance”, “exercise”, “athlete”, “elite”, is used in several articles. In this regard, authors should provide detailed participant metadata for accurate interpretation of results. Moreover, specific indicators of physical activity performance, such as endurance, should be properly described in the study design. Other limitations of current research summarised in this review include different dietary patterns among participants from different studies that show an association with gut microbiota regardless of physical activity. Similarly, different geographical origins of individuals and control groups such as non-elite athletes or sedentary adults are potential confounding variables that may be considered to assess bias in these studies.
Diet modulates the composition of the athletic gut microbiota. Long-term diets modulate gut microbiota composition. For example, protein and animal fat lead to an increase in Bacteroides, while simple carbohydrates are associated with Prevotella enrichment[35]. It is difficult to establish standard diet regimes due to the considerable complexity of stress responses in elite athletes. It has been proposed that gut microbiota acts like an endocrine organ by secreting serotonin, dopamine or other neurotransmitters and may control the hypothalamus-pituitary-adrenal axis in athletes resulting in the release of stress and catabolic hormones, inflammatory cytokines and microbial molecules. Therefore, dietary interventions may contribute to an improvement in sports performance[6,36]. However, some dietary recommendations for elite athletes are primarily based on low consumption of plant polysaccharides, which is commonly associated with reduced microbiota diversity and SCFA and neurotransmitter synthesis. In this regard, dietary recommendations for athletes should consider fiber intake to promote microbial species reduced in athlete’s gut[6,35]. Some studies reported a reduced diversity of the athlete’s gut microbiota that could be attributed to their higher protein intake, although there is a need for longer-term studies on athletes from different disciplines to assess the impact of diet on the athletic gut microbiota. It has been reported that protein intake was negatively correlated with diversity in marathon runners and fat intake was negatively correlated with Bifidobacteria in bodybuilders[35,37]. As explained, high-protein diets may decrease microbial diversity in endurance athletes who consume lower amounts of carbohydrates. Similarly, a decrease in SCFA-producing species has been observed in resistance athletes that follow a high-protein, low-carbohydrate, and high-fat diet. However, some dietary recommendations for endurance athletes resulting in the consumption of high amounts of both simple and complex carbohydrates in combination with training may lead to an increased abundance of Prevotella. This genus is associated with fiber-rich diets and participates in several amino acid and carbohydrate metabolism pathways, including branched-chain amino acid metabolism[35]. Interestingly, five bacterial families (Lachnospiraceae, Clostridiaceae, Paraprevotellaceae, Ruminococcaceae, and Veillonellaceae) were associated with physical activity in studies that controlled diet as a possible confounding factor[1].
Future trends
Future research trends may focus on the interactions of diet and gut microbial composition in the context of athletic performance as well as potential applications of pro- pre- and symbiotic supplements specifically developed for athletes. For this purpose, more studies dealing with metabolic activities and other mechanisms of action of specific clades associated with several indicators of sports performance are needed. In this regard, metagenomic sequencing enables the identification of microbial metabolic pathways showing higher abundances in the gut microbiota of athletes from several disciplines than in sedentary controls. This fact may contribute to a better understanding of the role of gut commensals in athlete physiology. In addition, further studies including a multi-omics approach (i.e. the combined analysis of metabolomic and metagenomic data) are needed to better elucidate the associations between physical activity, dietary patterns and gut microbiota. It should be noted that most articles published up to date comprise observational studies and the mechanisms of action of specific microbial clades contributing to sports performance remain unknown. In this regard, genomic sequences recovered from athlete metagenomes could be of great benefit to perform metabolic modelling in order to characterize those activities that may have a physiological impact. The integration of these techniques may lead to personalized nutrition interventions including specific nutrient recommendations aimed at improving performance by promoting certain taxa and enhancing certain metabolites of interest during exercise. Finally, more randomized controlled studies analyzing wider cohorts and controlling for potential confounding factors are needed to identify microbial species that could be used as biomarkers of sports performance.
Conclusions
This systematic review gives a general overview of the applications of next-generation sequencing, including shotgun metagenomics and 16S amplicon sequencing, to study the athletic gut microbiota. Bibliographic analysis using computational models revealed the growing research interest in elucidating the associations between specific microbial clades and sports performance. In this sense, butyrate producers such as A. hadrus, C. bolteae, F. prausnitzii, R. hominis and unidentified species belonging to Clostridiales, Lachnospiraceae and Subdoligranulum may contribute to maintaining gut homeostasis in athletes. On the other hand, species involved in branched amino acid and lactate metabolism, such as P. copri and V. atypica, may reduce muscular fatigue. In addition, athletic gut microbiota shows an increase in microbial metabolic pathways involved in carbohydrate metabolism. Future research will aim at developing personalised nutrition strategies and recommendations to optimise microbiota composition profiles associated with different components of exercise (high, moderate and low static and dynamic components). Future research trends may focus on the interactions of diet and gut microbial composition in the context of athletic performance. For this purpose, multi-omic studies dealing with metabolic activities and other mechanisms of action of specific clades associated with several indicators of sports performance are needed. As a consequence, these microorganisms could be used as biomarkers of sports performance to assess the potential of athletic candidates.
DECLARATIONS
Authors’ contributions
Design: Sabater C, Ruiz L, Margolles A
Literature research: Sabater C
Data analysis: Sabater C
Manuscript writing: Sabater C
Manuscript editing: Sabater C, Iglesias-Gutiérrez E, Ruiz L, Margolles A
Manuscript revision: Sabater C, Iglesias-Gutiérrez E, Ruiz L, Margolles A
Availability of data and materials
PRISMA diagram is provided in Figure 1. Bibliographical analysis was performed using ggrapgh v2.0, litsearchr v1.0.0, revtools v0.4.1, synthesisr v0.3.0 packages implemented in R v3.6.2.
Financial support and sponsorship
Carlos Sabater is a postdoctoral researcher supported by the Juan de la Cierva-Formación Postdoctoral Trainee Program from MICINN, the Spanish Ministry of Science and Innovation (FJC2019-042125-I).
Conflicts of interest
Authors declared that there are no conflicts of interest.
Ethical approval and consent to participate
Not applicable.
Consent for publication
Not applicable.
Copyright
© The Author(s) 2023.
Supplementary Materials
REFERENCES
1. Dorelli B, Gallè F, De Vito C, et al. Can physical activity influence human gut microbiota composition independently of diet? Nutrients 2021;13:1890.
2. Donati Zeppa S, Agostini D, Gervasi M, et al. Mutual interactions among exercise, sport supplements and microbiota. Nutrients 2019;12:17.
3. Mach N, Fuster-Botella D. Endurance exercise and gut microbiota: a review. J Sport Health Sci 2017;6:179-97.
4. Clarke SF, Murphy EF, O’Sullivan O, et al. Exercise and associated dietary extremes impact on gut microbial diversity. Gut 2014;63:1913-20.
5. Hughes RL, Holscher HD. Fueling gut microbes: a review of the interaction between diet, exercise, and the gut microbiota in athletes. Adv Nutr 2021;12:2190-215.
6. Clark A, Mach N. Exercise-induced stress behavior, gut-microbiota-brain axis and diet: a systematic review for athletes. J Int Soc Sports Nutr 2016;13:43.
7. Mancin L, Rollo I, Mota JF, et al. Optimizing microbiota profiles for athletes. Exerc Sport Sci Rev 2021;49:42-9.
8. Sabater C, Calvete-torre I, Villamiel M, Moreno FJ, Margolles A, Ruiz L. Vegetable waste and by-products to feed a healthy gut microbiota: current evidence, machine learning and computational tools to design novel microbiome-targeted foods. Trends Food Sci Technol 2021;118:399-417.
9. Sabater C, Villamiel M, Montilla A. Integral use of pectin-rich by-products in a biorefinery context: a holistic approach. Food Hydrocolloids 2022;128:107564.
10. Westgate MJ. revtools: An R package to support article screening for evidence synthesis. Res Synth Methods 2019;10:606-14.
11. Westgate MJ, Grames E. synthesisr: Import, assemble, and deduplicate bibliographic datasets R package version 0.3.0. Available from: https://CRAN.R-project.org/package=synthesisr [Last accessed on 20 Feb 2023].
12. Grames EM, Stillman AN, Tingley MW, Elphick CS, Freckleton R. An automated approach to identifying search terms for systematic reviews using keyword co-occurrence networks. Methods Ecol Evol 2019;10:1645-54.
13. Pedersen TL. ggraph: an implementation of grammar of graphics for graphs and networks. R package version 2.0.1. Available from: https://cran.r-project.org/web/packages/ggraph [Last accessed on 20 Feb 2023].
14. Barton W, Penney NC, Cronin O, et al. The microbiome of professional athletes differs from that of more sedentary subjects in composition and particularly at the functional metabolic level. Gut 2018;67:625-33.
15. Cronin O, Barton W, Skuse P, et al. A Prospective metagenomic and metabolomic analysis of the impact of exercise and/or whey protein supplementation on the gut microbiome of sedentary adults. mSystems 2018:3.
16. Kostic A. Metagenomic and functional analysis of athletes identify an exercise-promoting microbiome. Diabetes 2018;67:2251-PUB.
17. Keohane DM, Woods T, O’Connor P, et al. Four men in a boat: ultra-endurance exercise alters the gut microbiome. J Sci Med Sport 2019;22:1059-64.
18. Scheiman J, Luber JM, Chavkin TA, et al. Meta-omics analysis of elite athletes identifies a performance-enhancing microbe that functions via lactate metabolism. Nat Med 2019;25:1104-9.
19. Petersen LM, Bautista EJ, Nguyen H, et al. Community characteristics of the gut microbiomes of competitive cyclists. Microbiome 2017;5:98.
20. O’Donovan CM, Madigan SM, Garcia-Perez I, Rankin A, O’Sullivan O, Cotter PD. Distinct microbiome composition and metabolome exists across subgroups of elite Irish athletes. J Sci Med Sport 2020;23:63-8.
21. Fart F, Rajan SK, Wall R, et al. Differences in gut microbiome composition between senior orienteering athletes and community-dwelling older adults. Nutrients 2020;12:2610.
22. Kulecka M, Fraczek B, Mikula M, et al. The composition and richness of the gut microbiota differentiate the top Polish endurance athletes from sedentary controls. Gut Microbes 2020;11:1374-84.
23. Moitinho-Silva L, Wegener M, May S, et al. Short-term physical exercise impacts on the human holobiont obtained by a randomised intervention study. BMC Microbiol 2021;21:162.
24. Fukuchi M, Sugita M, Banjo M, Yonekura K, Sasuga Y. The impact of a competitive event and the efficacy of a lactic acid bacteria-fermented soymilk extract on the gut microbiota and urinary metabolites of endurance athletes: an open-label pilot study. PLoS One 2022;17:e0262906.
25. Han M, Yang K, Yang P, et al. Stratification of athletes’ gut microbiota: the multifaceted hubs associated with dietary factors, physical characteristics and performance. Gut Microbes 2020;12:1-18.
26. Genç A. Does exercise and nutrition style affect intestinal micro-biota diversity? Prog Nutr 2020;22:e2020070.
27. Donovan CM, Connor B, Madigan SM, Cotter PD, O' Sullivan O. Instances of altered gut microbiomes among Irish cricketers over periods of travel in the lead up to the 2016 World Cup: a sequencing analysis. Travel Med Infect Dis 2020;35:101553.
28. Axelrod CL, Brennan CJ, Cresci G, et al. UCC118 supplementation reduces exercise-induced gastrointestinal permeability and remodels the gut microbiome in healthy humans. Physiol Rep 2019;7:e14276.
29. Özkan A, Demirhan B, Genç A, et al. Effect of different intensity exercise on intestinal microbiota. Prog Nutr 2020;22:e2020080.
30. Kuhn M. Caret: classification and Regression Training. R package version 6.0-90. Available from: https://CRAN.R-project.org/package=caret [Last accessed on 20 Feb 2023].
31. Stojanov S, Berlec A, Štrukelj B. The influence of probiotics on the firmicutes/bacteroidetes ratio in the treatment of obesity and inflammatory bowel disease. Microorganisms 2020;8:1715.
32. Manor O, Dai CL, Kornilov SA, et al. Health and disease markers correlate with gut microbiome composition across thousands of people. Nat Commun 2020;11:5206.
33. Brahe LK, Le Chatelier E, Prifti E, et al. Specific gut microbiota features and metabolic markers in postmenopausal women with obesity. Nutr Diabetes 2015;5:e159.
34. Bouchau R, Cariou E, Deney A, et al. Sports participation and myocarditis: Influence of sport types on disease severity. Int J Cardiol Heart Vasc 2021;37:100895.
35. Mohr AE, Jäger R, Carpenter KC, et al. The athletic gut microbiota. J Int Soc Sports Nutr 2020;17:24.
36. Marttinen M, Ala-Jaakkola R, Laitila A, Lehtinen MJ. Gut microbiota, probiotics and physical performance in athletes and physically active individuals. Nutrients 2020;12:2936.
Cite This Article
Export citation file: BibTeX | RIS
OAE Style
Sabater C, Iglesias-Gutiérrez E, Ruiz L, Margolles A. Next-generation sequencing of the athletic gut microbiota: a systematic review. Microbiome Res Rep 2023;2:5. http://dx.doi.org/10.20517/mrr.2022.16
AMA Style
Sabater C, Iglesias-Gutiérrez E, Ruiz L, Margolles A. Next-generation sequencing of the athletic gut microbiota: a systematic review. Microbiome Research Reports. 2023; 2(1): 5. http://dx.doi.org/10.20517/mrr.2022.16
Chicago/Turabian Style
Sabater, Carlos, Eduardo Iglesias-Gutiérrez, Lorena Ruiz, Abelardo Margolles. 2023. "Next-generation sequencing of the athletic gut microbiota: a systematic review" Microbiome Research Reports. 2, no.1: 5. http://dx.doi.org/10.20517/mrr.2022.16
ACS Style
Sabater, C.; Iglesias-Gutiérrez E.; Ruiz L.; Margolles A. Next-generation sequencing of the athletic gut microbiota: a systematic review. Microbiome. Res. Rep. 2023, 2, 5. http://dx.doi.org/10.20517/mrr.2022.16
About This Article
Copyright
Data & Comments
Data
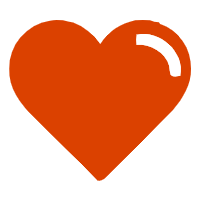

Comments
Comments must be written in English. Spam, offensive content, impersonation, and private information will not be permitted. If any comment is reported and identified as inappropriate content by OAE staff, the comment will be removed without notice. If you have any queries or need any help, please contact us at support@oaepublish.com.