Bifidobacterium in anticancer immunochemotherapy: friend or foe?
Abstract
The gut microbiome has received a crescendo of attention in recent years due to myriad influences on human pathophysiology, including cancer. Anticancer therapy research is constantly looking for new hints to improve response to therapy while reducing the risk of relapse. In this scenario, Bifidobacterium, which inhabits the gut microbial ecosystem (especially that of children) and is considered a health-associated microbe, has emerged as a key target to assist anticancer treatments for a better prognosis. However, some researchers have recently hypothesized an unfavorable role of Bifidobacterium spp. in anticancer immunochemotherapy, leading to some confusion in the field. This narrative review summarizes the current knowledge on the role of Bifidobacterium spp. in relation to anticancer treatments, discussing the pros and cons of its presence in the gut microbiome of cancer patients. The current intervention strategies based on the administration of probiotic strains of Bifidobacterium are then discussed. Finally, the need to conduct further studies, especially functional ones, is underlined to provide robust experimental evidence, especially on the underlying molecular mechanisms, and thus resolve the controversies on this microbe for the long-term success of immunochemotherapy.
Keywords
INTRODUCTION
Bifidobacterium are non-spore-forming Gram-positive bacteria with a bifurcated (“bifid”) shape, belonging to the Actinomycetota phylum. Since 1900, when they were first isolated from the feces of breastfed infants[1], about 118 species have been identified and included in the Bifidobacterium genus[2]
Bifidobacterium can be found in several ecological niches, including the gastrointestinal tract of humans and other mammals, insects, and birds[3,4]. Bifidobacterium spp. are the first colonizers of the gut microbiota (GM) of infants, in particular of those breastfed, where they abound due to their ability to metabolize human milk oligosaccharides (HMOs)[5]. Later, with the cessation of breastfeeding and the introduction of solid foods, the near monodominance of Bifidobacterium spp. comes to an end and the GM begins to be colonized by other (more strictly anerobic) microorganisms, assuming a more adult-like composition[6]. In most adult-type GMs, Bifidobacterium still constitutes a relevant component, albeit in much lower proportions (mean relative abundance, 2-14%)[7]. Further decline of Bifidobacterium spp. can be observed with aging, along with a decrease in species diversity, which has been correlated with immunosenescence[8-10]. In contrast, a higher occurrence or prevalence of Bifidobacterium was found in centenarians (and beyond) worldwide, suggesting an association with healthy aging and longevity[10-12].
The ecological fitness of Bifidobacterium can be explained by its ability to metabolize dietary and host-derived carbohydrates, leading to the production of short-chain fatty acids (SCFAs) with a key and multifactorial role in human physiology[2,13,14]. The glycobiome of Bifidobacterium is indeed one of the largest among other gut commensals, comprising several glycosyl hydrolases, glycosyltransferases, and carbohydrate esterases[15]. Additionally, certain Bifidobacterium spp. can synthesize folates and tryptophan-derived indoles[16]. The former are involved in cell metabolism, immune development, and epigenetic modifications[17-19], while the latter improve the integrity of the intestinal epithelium and regulate intestinal mucosa immune responses as aryl hydrocarbon receptor ligands[20-22]. Bifidobacterium can also limit the growth of pathogens by modulating the immune system and producing antibacterial peptides (i.e., bacteriocins) and organic acids (i.e., lactic and acetic acids)[23]. In particular, the accumulation of organic acids leads to acidification of the surrounding environment, thus inhibiting the growth of low pH-sensitive bacteria[24,25].
All these activities underlie the beneficial effects of Bifidobacterium spp. and their use as probiotics in different healthcare settings[26,27]. Unsurprisingly, GM alterations (i.e., dysbiosis) often include, regardless of age, an underrepresentation of Bifidobacterium[28-31]. Furthermore, various studies have reported that the administration of Bifidobacterium spp., alone or in combination with other lactic acid bacteria (particularly Lactobacillus), helps to relieve the symptoms of several intestinal and extraintestinal disorders, e.g., inflammatory bowel disease[32], irritable bowel syndrome[33], antibiotic-associated diarrhea[34] and Clostridioides difficile-associated diarrhea[35], necrotizing enterocolitis[36], allergy[37], systemic lupus erythematosus[38], and atopic dermatitis[39].
While in the aforementioned pathological contexts, the benefits of Bifidobacterium spp. are well established, their role in the cancer setting is still controversial, with some evidence suggesting they may improve immune function and reduce postoperative complications, and more recent evidence suggesting an association with immunochemoresistance and treatment failure[40] [Figure 1].
Figure 1. Bifidobacterium and its controversial role in the response to anticancer immunochemotherapy. Bifidobacterium spp. have been associated with response to immunochemotherapy through stimulation and activation of effector T lymphocytes and dendritic cells. On the other hand, Bifidobacterium spp. have been associated with induction of Treg cells, stimulation of anti-inflammatory cytokines, and general immunosuppressive effects (also mediated by short-chain fatty acids – SCFAs). Furthermore, the ability of Bifidobacterium to produce lactate suggests a hypothetical link with the “Warburg effect”, known to promote tumor growth, thus potentially liming the effect of immunochemotherapy. The figure was partly generated using Servier Medical Art, provided by Servier, licensed under a Creative Commons Attribution 3.0 unported license and images from Flaticon resources.
In this review, after summarizing the relationship between GM and immunochemotherapy, we discuss the latest research evaluating the impact of the presence of Bifidobacterium in the GM of cancer patients, as well as its administration in probiotic formulations on therapeutic response and key clinical outcomes. Finally, we stress the need to conduct further studies, especially of a functional nature, in order to move from purely associative observations to mechanistic glimpses, which provide robust experimental evidence on the role of Bifidobacterium in the anticancer immunochemotherapy landscape. The definition of this role will make it possible to improve current intervention strategies for truly successful precision medicine approaches.
THE RELATIONSHIP OF THE GUT MICROBIOME WITH RESPONSE TO IMMUNOCHEMOTHERAPY
In recent years, several studies have highlighted a bidirectional relationship between GM and immunochemotherapy in different types of cancer. Indeed, therapeutic efficacy was significantly reduced in the absence of GM, thus suggesting that commensal microbes may modulate anticancer immune responses through several mechanisms[40,41]. The first example of this intricate interconnection between gut microbes and anticancer treatment involves cyclophosphamide, an approved chemotherapeutic drug. It has been shown that cyclophosphamide can alter GM composition in mice and promote the translocation of specific Gram-positive bacteria into secondary lymphoid organs, stimulating the production of Th17 cells[42,43]. Indeed, germ-free mice or mice treated with broad-spectrum antibiotics showed resistance to cyclophosphamide-based therapies[43]. In particular, the antitumor response was associated with increased levels of Lactobacillus johnsonii, Enterococcus hirae and Barnesiella intestinihominis[43]. A few years later, elegant work by Daillere et al. revealed the mechanisms by which E. hirae and B. intestinihominis were able to stimulate the immune response against tumor cells[44]. The former translocated from the small intestine to secondary lymphoid organs and increased the intratumor CD8/Treg ratio, while the latter was overabundant in the colon where it promoted the infiltration of interferon-gamma (IFN-γ)-producing T cells into cancer lesions. Further confirming these findings, the antitumor activity of cyclophosphamide was restored in murine models receiving an oral gavage of E. hirae after antibiotic treatment.
GM can also modulate the efficacy of immunotherapy[45-47]. Since their development, immune checkpoint inhibitors have revolutionized the anticancer therapeutic landscape, positively changing the clinical outcomes of several cancers such as melanoma[48] and renal cell carcinoma[49], as well as malignancies considered non-immunogenic such as non-small-cell lung cancer (NSCLC)[50,51] or mismatch-repair-deficient colorectal cancer (CRC)[52]. Immune checkpoint therapy targets regulatory pathways in T cells by removing their inhibitory signals, thereby enabling tumor-reactive T cells to unleash an effective antitumor response[53]. An early study in antibiotic-treated mice showed altered GM that impaired both CpG-oligonucleotide immunotherapy and platinum-based chemotherapy. On the other hand, the reduction of tumor growth through the production of tumor necrosis factor alpha (TNFα) by myeloid cells and T cells was shown in mice not receiving antibiotics. Indeed, antibiotic treatment impaired the production of TNFα and other cytokines by immune cells including monocytes, macrophages, and dendritic cells, and reduced tumor regression[42]. Furthermore, the antitumor effect of anti-cytotoxic T-lymphocyte-associated protein 4 (anti-CTLA-4) antibodies was associated with the presence of distinct Bacteroides species that were able to stimulate the T cell response against melanoma. Indeed, germ-free and antibiotic-treated mice did not respond to anti-CTLA-4, while when they were gavaged with Bacteroides fragilis, a restoration of the efficacy of the anticancer therapy was observed. The same anticancer outcomes were obtained only by immunizing the same murine models with B. fragilis polysaccharides, or by adoptive transfer of B. fragilis-specific T cells. In particular, B. fragilis was associated with Th1 immune responses in lymph nodes and dendritic cell maturation in the tumor environment, thereby reverting CTLA-4 blockade. Similar results were obtained in melanoma patients in whom anti-CTLA-4 efficacy was associated with T cell responses mediated by an overabundance of B. fragilis or Bacteroides thetaiotaomicron[48]. Fecal microbial transplantation from humans to murine models further confirmed that anti-CTLA-4 treatment favored the outgrowth of B. fragilis with all the anticancer properties discussed above. More recently, GM analysis of metastatic melanoma patients receiving ipilimumab, a CTLA-4-targeted immune checkpoint inhibitor, revealed that elevated levels of Faecalibacterium and other members of the phylum Firmicutes were associated with not only longer survival but also reduced occurrence of ipilimumab-induced colitis[54]. Similarly, programmed cell death protein 1 (PD-1) is one of the inhibitory receptors that downregulate effector functions and suppress the immune response leading to non-activation of the immune cascade against tumors. Immunotherapy against PD-1 allowed to achieve tumor regression in a subset of cancers[55-59]. As for GM, studies in renal and lung cancers showed that non-responders were characterized by reduced levels of Akkermansia muciniphila, which promoted the recruitment of activated T lymphocytes into the tumor microenvironment via the IL-12 pathway[47]. A recent study confirmed the relevance of Akkermansia as a prognostic factor for NSCLC patients treated with immune checkpoint inhibitors[60]. Specifically, the authors found that baseline relative abundance of Akkermansia was linked to therapeutic advantages, namely increased objective response rates and overall survival. Furthermore, the intestinal residence of Akkermansia was found to be a proxy for the richness of the gut ecosystem, which is generally related to more favorable outcomes. Finally, two studies in melanoma patients have identified other commensal microbes potentiating the antitumor effects of PD-1 blockade in responders[45,46]. Notably, responders exhibited greater interindividual diversity (which suggests retention of one’s GM fingerprint or uniqueness) and higher levels of Faecalibacterium and other health-associated Ruminococcaceae members, which were involved in enhanced antigen presentation and T cell function in the tumor microenvironment[45]. For a list of bacteria other than Bifidobacterium that have been suggested to date to play a role in the success (or failure) of immunochemotherapy, please consult the relevant literature (e.g., Table 1 from Gupta et al. 2021[61], including pathogenic and non-pathogenic bacteria mediating cancer immunotherapy, and Tang et al. 2022[62], who also discussed engineered bacteria with enhanced tumor tropism, significant immunomodulation and improved safety profile, for enhanced therapeutic outcomes in different cancer models).
Clinical trials registered in the last two years on ClinicalTrials.gov (as accessed in March 2023) involving the use of Bifidobacterium spp. as adjuvant therapy in cancer patients. Search terms included “cancer” or “tumor” in combination with “Bifidobacterium”
Title | Status | Results | Condition | Intervention | Location | URL |
Safety and Efficacy of Bifidobacterium Therapy in Patients With Advanced Liver Cancer Receiving Immunotherapy | Recruiting | Not available | Advanced Hepatocellular Carcinoma | Bifidobacterium bifidum oral product | China | https://clinicaltrials.gov/ct2/show/NCT05620004 |
Clinical Study on BIFICO Accelerating Postoperative Liver Function Recovery in Patients With Hepatocellular Carcinoma | Completed | Not available | Hepatocellular Carcinoma | BIFICO (Bifidobacterium-based product) | China | https://clinicaltrials.gov/ct2/show/NCT05178524 |
Lactobacillus Bifidobacterium V9 (Kex02) Improving the Efficacy of Carilizumab Combined With Platinum in Non-small Cell Lung Cancer Patients | Recruiting | Not available | Non-Small Cell Lung Cancer | Bifidobacterium and Lactobacillus; Placebo | China | https://clinicaltrials.gov/ct2/show/NCT05094167 |
Effect of Live Combined Bifidobacterium, Lactobacillus and Enterococcus Capsules on Oral Mucositis in NasopharyngealCarcinoma Patients Receiving Radiotherapy | Unknown status | Not available | Oral mucositis in Nasopharyngeal Carcinoma | Lactobacillus, Bifidobacterium and Enterococcus | China | https://clinicaltrials.gov/ct2/show/NCT03112837 |
BIFIDOBACTERIUM AND THE IMMUNOCHEMOTHERAPY LANDSCAPE
In this context, Bifidobacterium deserves special attention as it has been directly involved in the response to immunochemotherapy[63,64]. For example, in 2015, Sivan et al. compared antitumor T lymphocyte responses in murine models purchased from two different facilities[65], namely Jackson Laboratory (JAX) and Taconic Farms (TAC) mice. Compared to TAC mice, JAX mice developed adequate antitumor immunity. Interestingly, the mice also differed in GM composition, with Bifidobacterium being overabundant in JAX mice. After induction of melanoma in both murine models, JAX mice exhibited reduced tumor cell growth and enhanced T cell-mediated immune surveillance. Overabundance of Bifidobacterium, particularly species B. breve, B. longum and B. adolescentis, was positively associated with the antitumor response mediated by activation of T cell effectors. Furthermore, oral administration of B. breve and B. longum to mice with Bifidobacterium depletion was sufficient to reduce natural melanoma growth alone and, in association with immunotherapy (anti-PD-L1), to restore specific antitumor T cell responses and almost abolish tumor outgrowth. Increased dendritic cell function leading to T cell activation in the tumor microenvironment was shown in mice receiving B. breve or B. longum, compared to germ-free mice or mice natively without Bifidobacterium in the GM. These findings were validated in a cohort of patients with metastatic melanoma, where metagenomic sequencing revealed pre-treatment enrichment of B. longum, along with A. muciniphila, Collinsella aerofaciens, and Enterococcus faecium, in those who responded to anti-PD-L1 immunotherapy[46]. Furthermore, fecal microbiota transplantation into germ-free mice with responder GM led to the restoration of anti-PD-L1 treatment efficacy. A study by Rong et al. suggested that Bifidobacterium may potentiate the antitumor immune response by promoting the long-term survival of CD8+ T cells as memory cells and thus stimulating the immune response in some immunotherapy treatments[66]. In another recent study, it was demonstrated that Bifidobacterium strains induce potent CD8+ T cell-mediated antitumor immunity in animal models, thus enhancing the treatment with immune checkpoint inhibitors[67]. Interestingly, several years earlier, Li et al. suggested a direct role of Bifidobacterium spp. at the tumor site[68], by demonstrating their translocation from the gastrointestinal tract into the bloodstream and selective accumulation in tumors, due to their ability to survive in the hypoxic, nutrient-rich environment created by tumor cells[69]. Furthermore, the potential of B. adolescentis as a highly specific vector for the transport of anticancer genes to a target tumor has been demonstrated. In murine models subcutaneously implanted with liver cancer cells, intravenous injection of B. adolescentis previously transformed to express a gene encoding the antiangiogenic protein endostatin resulted in germination and proliferation of microbes within the tumor bed, but not in non-malignant tissues. In addition, intratumoral expression of endostatin and thus inhibition of tumor growth were observed.
On the other hand, evidence is also accumulating on a not entirely favorable role of Bifidobacterium in the response to immunochemotherapy. For example, Bifidobacterium were found to be overabundant in the GM of platinum-resistant patients treated for epithelial ovarian cancer[70]. As speculated by the authors, the lactate produced by these microbes as part of their metabolism could fuel the “Warburg effect” (i.e., the production of lactate by aerobic glycolysis)[71,72]. Increased lactate production is frequently observed in tumor cells, where it promotes angiogenesis, tumor growth, inflammation, metastasis, epithelial-mesenchymal transition and immune evasion. The hypothesis put forward by the authors is therefore that Bifidobacterium, and potentially other lactic acid bacteria, are capable of interfering with the lactate cycle, increasing its local and systemic bioavailability and thus influencing tumor progression, as well as the efficacy of chemotherapy. While fascinating, it should be noted that these speculations are based on the detection of increased proportions of Bifidobacterium and other potential lactate producers (and predicted lactate production pathways) while decreased proportions of lactate utilizers in platinum-resistant vs. platinum-sensitive patients, without direct measurement of lactate levels (and its isoforms). In this regard, the lactate levels typically produced in the intestine are much lower than those obtained at the tumor site by the Warburg effect[72], further underlining the need to verify the proposed link. Furthermore, it cannot be ruled out that GM alterations in potential lactate producers/utilizers are only a side effect of chemotherapy, in combination with other host factors, with no direct role in treatment response. However, there is previous evidence that similarly demonstrated an increase in lactic acid bacteria, including Bifidobacterium, in patients with gastrointestinal cancer, and suggested a role for them in influencing tumor development, also through exogenous lactate supply[73]. Once again, these are purely associative observations, which makes proof of concept mandatory, especially in extra-intestinal cancers.
In support of the latter speculations, it should be mentioned that Bifidobacterium has a known anti-inflammatory role, mediated by the production of SCFAs and induction of Treg cells and IL-10, so it is not entirely unreasonable to doubt its ability to promote antitumor immune responses. Perhaps the discriminating factor, as the studies commented above suggest, is the use of immune vs. chemotherapy protocols. In the context of immunotherapy, we hypothesize that Bifidobacterium-induced Treg cells in the intestine might migrate into the tumor microenvironment and promote tumor cell evasion mechanisms from immunosurveillance. In this regard, in a very elegant and recent study in murine models, Fidelle et al. revealed that enterotropic T cells can actually relocate to distant tumors and impair the therapeutic efficacy of immune checkpoint inhibitors[74]. Notably, such a relocation was favored by gut dysbiosis-induced downregulation of the expression of mucosal addressin cell adhesion molecule 1 (MAdCAM-1) on intestinal endothelial cells, which usually helps retain an immunosuppressive set of T cells (Treg17 cells) within the gut, through interaction with integrin 47. Disruption of MAdCAM-1-47 interaction triggered the migration of Treg17 cells from the ileal lamina propria and gut-associated lymphoid tissues to distant tumors and tumor-draining lymph nodes, where they impaired the therapeutic efficacy of immune checkpoint inhibitors by producing immunosuppressive molecules such as IL-10, CD39 and CD73. Moreover, the tumor microenvironment was found to be associated with metabolic reprogramming of tumor-infiltrating Treg cells, which increases their reliance on fatty acid (instead of glucose) metabolism, further contributing to the Warburg effect[75]. However, the process of Treg cell migration to tumor sites and the interaction of migrated immune cells with cancer cells (including factors driving their metabolic behavior) are highly complex processes not yet fully understood. More evidence is needed to better elucidate the role of gut-primed Tregs in cancer immunosurveillance and whether/how Bifidobacterium (or other GM components) is involved in this intricate picture.
GUT MICROBIOME-BASED ANTICANCER INTERVENTION STRATEGIES INVOLVING Bifidobacterium SPP
The results of clinical and preclinical studies investigating the functional roles of Bifidobacterium spp. in the cancer setting have so far been sadly inconclusive. However, the use of Bifidobacterium as a probiotic, alone or in combination with Lactobacillus spp., has led to numerous benefits in multiple pathological contexts[76]. With specific regard to cancer, the intake of Bifidobacterium spp. conferred protection against CRC development in mice[77] and improved immune function in CRC patients[78]. Furthermore, Bifidobacterium mitigated the secondary effects of surgery and chemotherapy in patients with CRC, as well as in those undergoing colectomy or resection of liver metastases[79,80]. In particular, B. breve reduced post-chemotherapy GM dysbiosis and limited the development of infections in a pediatric cohort[81]. In contrast, another study focused on head and neck cancer patients treated with a cocktail of Bifidobacterium spp. and Lactobacillus spp. showed no improvement in patients’ clinical outcomes (i.e., inflammatory markers and gut permeability)[82]. However, it should be mentioned that these conflicting results may be partly explained by interindividual variations in GM and host genomes. Indeed, several studies confirmed that the intestinal colonization and functionality of probiotics are strongly influenced by the individual GM, the host gene expression profile, and other exogenous factors[83-85].
Notwithstanding the above, a number of clinical trials have been planned (some of which are still ongoing) to examine the therapeutic potential of Bifidobacterium interventions in cancer patients. A list of clinical studies registered in the previous two years is shown in Table 1. In an ongoing interventional randomized study of 30 participants with advanced liver cancer receiving immunotherapy, Xie et al. are profiling the GM of patients receiving or not a cocktail of lactic acid bacteria, including Bifidobacterium spp. (NCT05620004). Another interventional randomized clinical trial was designed to evaluate the impact of oral administration of Lactobacillus and Bifidobacterium on 46 participants with NSCLC on the efficacy of immunochemotherapy (NCT05094167). Other ongoing studies are investigating the role of Bifidobacterium in improving the conditions of patients before and after cancer removal surgery, by mitigating therapy-related side effects[86,87]. Furthermore, in a randomized controlled clinical trial of 180 patients with hepatocellular carcinoma (NCT05178524), investigators used Bifidobacterium-rich BIFICO as an intervention strategy to sustain medication in the perioperative period of hepatectomy and observed postoperative liver functionality recovery. The first published research on this project revealed that the GM profile influenced the rate at which liver function recovered after hepatectomy, and, again, Bifidobacterium was a major player. In an interventional randomized clinical trial (NCT03112837), investigators evaluated the impact of combined live capsules of Bifidobacterium, Lactobacillus and Enterococcus in patients with nasopharyngeal carcinoma. In particular, the aim of this study was to determine whether GM modulation reduced the severity of radiation-induced mucositis in patients receiving radical dose radiotherapy. Indeed, radiation-induced mucositis is an acute mucosal reaction of patients undergoing head and neck radiotherapy, which leads to dose-limiting and debilitating side effects.
CONCLUSIONS AND FUTURE TRENDS
GM has now taken a leading role in research focused on maintaining host well-being, including the success of anticancer therapies. In this scenario, several works have linked Bifidobacterium to improved response to immunochemotherapy. However, its well-known anti-inflammatory and immunosuppressive properties and its recent association with platinum resistance do not allow definitive conclusions to be drawn. Nevertheless, some clinical trials are already underway involving the intake of Bifidobacterium directly in cancer patients. It goes without saying that further studies in large cohorts are needed to fill the many knowledge gaps and provide robust experimental evidence. Such studies should possibly be conducted with different omics approaches (including metabolomics) and animal models to finally go beyond simple associations and uncover the underlying molecular mechanisms. Only the achievement of such a goal will truly allow precision strategies to be implemented. In the near future, it is possible to foresee not only that Bifidobacteriuml strains will be rationally used to induce certain anticancer effects in a given context, but that they will eventually be engineered to either boost or hinder some functionalities instrumental to the success of immunochemotherapy protocols. Not least, it is possible to envisage the use of Bifidobacterium-derived postbiotics[88] to specifically confer beneficial effects by directly using the molecular actors involved. This could be particularly relevant in the case of cancer patients, often immunocompromised and with an increased risk of infections and sepsis.
DECLARATIONS
Authors’ contributionsWrote the original draft: Procaccianti G, Roggiani S, Conti G
Reviewed and edited the manuscript: Turroni S, D’Amico F
Supervised during the draft preparation: D’Amico F, Turroni S, Brigidi P
All authors have read and agreed to the published version of the manuscript.
Availability of data and materialsNot applicable.
Financial support and sponsorshipNone.
Conflicts of interestAll authors declared that there are no conflicts of interest.
Ethical approval and consent to participateNot applicable.
Consent for publicationNot applicable.
Copyright© The Author(s) 2023.
REFERENCES
1. Henry T. Recherches sur la flore intestinale des nourrissons:(état normal et pathologique). Available from: https://books.google.com.hk/books/about/Recherches_sur_la_flore_intestinale_des.html?id=jrdkcgAACAAJ&redir_esc=y. [Last accessed on 5 Jul 2023].
2. Sakanaka M, Gotoh A, Yoshida K, et al. Varied pathways of infant gut-associated bifidobacterium to assimilate human milk oligosaccharides: prevalence of the gene set and its correlation with bifidobacteria-rich microbiota formation. Nutrients 2019;12:71.
3. Alberoni D, Gaggìa F, Baffoni L, Modesto MM, Biavati B, Di Gioia D. Bifidobacterium xylocopae sp. nov. and bifidobacterium aemilianum sp. nov., from the carpenter bee (xylocopa violacea) digestive tract. Syst Appl Microbiol 2019;42:205-16.
4. Modesto M, Puglisi E, Bonetti A, et al. Bifidobacterium primatium sp. nov., bifidobacterium scaligerum sp. nov., bifidobacterium felsineum sp. nov. and bifidobacterium simiarum sp. nov.: four novel taxa isolated from the faeces of the cotton top tamarin (saguinus oedipus) and the emperor tamarin (saguinus imperator). Syst Appl Microbiol 2018;41:593-603.
5. Macpherson AJ, de Agüero MG, Ganal-Vonarburg SC. How nutrition and the maternal microbiota shape the neonatal immune system. Nat Rev Immunol 2017;17:508-17.
6. Stewart CJ, Ajami NJ, O'Brien JL, et al. Temporal development of the gut microbiome in early childhood from the TEDDY study. Nature 2018;562:583-8.
7. Odamaki T, Kato K, Sugahara H, et al. Age-related changes in gut microbiota composition from newborn to centenarian: a cross-sectional study. BMC Microbiol 2016;16:90.
8. Hopkins MJ, Macfarlane GT. Changes in predominant bacterial populations in human faeces with age and with clostridium difficile infection. J Med Microbiol 2002;51:448-54.
9. Woodmansey EJ, McMurdo ME, Macfarlane GT, Macfarlane S. Comparison of compositions and metabolic activities of fecal microbiotas in young adults and in antibiotic-treated and non-antibiotic-treated elderly subjects. Appl Environ Microbiol 2004;70:6113-22.
10. Biagi E, Franceschi C, Rampelli S, et al. Gut microbiota and extreme longevity. Curr Biol 2016;26:1480-5.
11. Biagi E, Rampelli S, Turroni S, Quercia S, Candela M, Brigidi P. The gut microbiota of centenarians: signatures of longevity in the gut microbiota profile. Mech Ageing Dev 2017;165:180-4.
12. Rampelli S, Soverini M, D'Amico F, et al. Shotgun metagenomics of gut microbiota in humans with up to extreme longevity and the increasing role of xenobiotic degradation. mSystems 2020;5:e00124-20.
13. Rivière A, Selak M, Lantin D, Leroy F, De Vuyst L. Bifidobacteria and butyrate-producing colon bacteria: importance and strategies for their stimulation in the human gut. Front Microbiol 2016;7:979.
14. Koh A, De Vadder F, Kovatcheva-Datchary P, Bäckhed F. From dietary fiber to host physiology: short-chain fatty acids as key bacterial metabolites. Cell 2016;165:1332-45.
15. Milani C, Lugli GA, Duranti S, et al. Bifidobacteria exhibit social behavior through carbohydrate resource sharing in the gut. Sci Rep 2015;5:15782.
16. Wong CB, Odamaki T, Xiao JZ. Insights into the reason of human-residential bifidobacteria (HRB) being the natural inhabitants of the human gut and their potential health-promoting benefits. FEMS Microbiol Rev 2020;44:369-85.
17. Sugahara H, Odamaki T, Hashikura N, Abe F, Xiao JZ. Differences in folate production by bifidobacteria of different origins. Biosci Microbiota Food Health 2015;34:87-93.
18. D’Aimmo MR, Mattarelli P, Biavati B, Carlsson NG, Andlid T. The potential of bifidobacteria as a source of natural folate. J Appl Microbiol 2012;112:975-84.
19. Jacob RA. Folate, DNA methylation, and gene expression: factors of nature and nurture. Am J Clin Nutr 2000;72:903-4.
20. Meng D, Sommella E, Salviati E, et al. Indole-3-lactic acid, a metabolite of tryptophan, secreted by bifidobacterium longum subspecies infantis is anti-inflammatory in the immature intestine. Pediatr Res 2020;88:209-17.
21. Hubbard TD, Murray IA, Bisson WH, et al. Adaptation of the human aryl hydrocarbon receptor to sense microbiota-derived indoles. Sci Rep 2015;5:12689.
22. Lanz TV, Becker S, Mohapatra SR, Opitz CA, Wick W, Platten M. Suppression of Th1 differentiation by tryptophan supplementation in vivo. Amino Acids 2017;49:1169-75.
23. Lim HJ, Shin HS. Antimicrobial and immunomodulatory effects of bifidobacterium strains: a review. J Microbiol Biotechnol 2020;30:1793-800.
24. Kovanda L, Zhang W, Wei X, et al. In vitro antimicrobial activities of organic acids and their derivatives on several species of gram-negative and gram-positive bacteria. Molecules 2019;24:3770.
25. Fukuda S, Toh H, Hase K, et al. Bifidobacteria can protect from enteropathogenic infection through production of acetate. Nature 2011;469:543-7.
26. Yao S, Zhao Z, Wang W, Liu X. Bifidobacterium longum: protection against inflammatory bowel disease. J Immunol Res 2021;2021:8030297.
27. Wieërs G, Verbelen V, Van Den Driessche M, et al. Do probiotics during in-hospital antibiotic treatment prevent colonization of gut microbiota with multi-drug-resistant bacteria? A randomized placebo-controlled trial comparing
28. Hevia A, Milani C, López P, et al. Allergic patients with long-term asthma display low levels of bifidobacterium adolescentis. PLoS One 2016;11:e0147809.
29. Gao X, Jia R, Xie L, Kuang L, Feng L, Wan C. Obesity in school-aged children and its correlation with gut e.coli and bifidobacteria: a case-control study. BMC Pediatr 2015;15:64.
30. Taverniti V, Guglielmetti S. Methodological issues in the study of intestinal microbiota in irritable bowel syndrome. World J Gastroenterol 2014;20:8821-36.
31. Murri M, Leiva I, Gomez-Zumaquero JM, et al. Gut microbiota in children with type 1 diabetes differs from that in healthy children: a case-control study. BMC Med 2013;11:46.
32. Groeger D, O'Mahony L, Murphy EF, et al. Bifidobacterium infantis 35624 modulates host inflammatory processes beyond the gut. Gut Microbes 2013;4:325-39.
33. Agrawal A, Houghton LA, Morris J, et al. Clinical trial: the effects of a fermented milk product containing Bifidobacterium lactis DN-173 010 on abdominal distension and gastrointestinal transit in irritable bowel syndrome with constipation. Aliment Pharmacol Ther 2009;29:104-14.
34. Corrêa NB, Péret Filho LA, Penna FJ, Lima FM, Nicoli JR. A randomized formula controlled trial of bifidobacterium lactis and streptococcus thermophilus for prevention of antibiotic-associated diarrhea in infants. J Clin Gastroenterol 2005;39:385-9.
35. Plummer S, Weaver MA, Harris JC, Dee P, Hunter J. Clostridium difficile pilot study: effects of probiotic supplementation on the incidence of c. difficile diarrhoea. Int Microbiol 2004;7:59-62.
36. Lin HC, Hsu CH, Chen HL, et al. Oral probiotics prevent necrotizing enterocolitis in very low birth weight preterm infants: a multicenter, randomized, controlled trial. Pediatrics 2008;122:693-700.
37. Singh A, Hacini-Rachinel F, Gosoniu ML, et al. Immune-modulatory effect of probiotic Bifidobacterium lactis NCC2818 in individuals suffering from seasonal allergic rhinitis to grass pollen: an exploratory, randomized, placebo-controlled clinical trial. Eur J Clin Nutr 2013;67:161-7.
38. López P, de Paz B, Rodríguez-Carrio J, et al. Th17 responses and natural IgM antibodies are related to gut microbiota composition in systemic lupus erythematosus patients. Sci Rep 2016;6:24072.
39. Yeşilova Y, Çalka Ö, Akdeniz N, Berktaş M. Effect of probiotics on the treatment of children with atopic dermatitis. Ann Dermatol 2012;24:189-93.
40. D’Amico F, Barone M, Tavella T, Rampelli S, Brigidi P, Turroni S. Host microbiomes in tumor precision medicine: how far are we? Curr Med Chem 2022;29:3202-30.
41. Roy S, Trinchieri G. Microbiota: a key orchestrator of cancer therapy. Nat Rev Cancer 2017;17:271-85.
42. Viaud S, Flament C, Zoubir M, et al. Cyclophosphamide induces differentiation of Th17 cells in cancer patients. Cancer Res 2011;71:661-5.
43. Viaud S, Saccheri F, Mignot G, et al. The intestinal microbiota modulates the anticancer immune effects of cyclophosphamide. Science 2013;342:971-6.
44. Daillère R, Vétizou M, Waldschmitt N, et al. Enterococcus hirae and barnesiella intestinihominis facilitate cyclophosphamide-induced therapeutic immunomodulatory effects. Immunity 2016;45:931-43.
45. Gopalakrishnan V, Spencer CN, Nezi L, et al. Gut microbiome modulates response to anti-PD-1 immunotherapy in melanoma patients. Science 2018;359:97-103.
46. Matson V, Fessler J, Bao R, et al. The commensal microbiome is associated with anti-PD-1 efficacy in metastatic melanoma patients. Science 2018;359:104-8.
47. Routy B, Le Chatelier E, Derosa L, et al. Gut microbiome influences efficacy of PD-1-based immunotherapy against epithelial tumors. Science 2018;359:91-7.
48. Vétizou M, Pitt JM, Daillère R, et al. Anticancer immunotherapy by CTLA-4 blockade relies on the gut microbiota. Science 2015;350:1079-84.
49. Motzer RJ, Escudier B, McDermott DF, et al. Checkmate 025 investigators. Nivolumab versus everolimus in advanced renal-cell carcinoma. N Engl J Med 2015;373:1803-13.
50. Borghaei H, Paz-Ares L, Horn L, et al. Nivolumab versus docetaxel in advanced nonsquamous non-small-cell lung cancer. N Engl J Med 2015;373:1627-39.
51. Carbone DP, Reck M, Paz-Ares L, et al. CheckMate 026 Investigators. First-Line Nivolumab in Stage IV or Recurrent Non-Small-Cell Lung Cancer. N Engl J Med 2017;376:2415-26.
52. Le DT, Uram JN, Wang H, et al. PD-1 blockade in tumors with mismatch-repair deficiency. N Engl J Med 2015;372:2509-20.
54. Chaput N, Lepage P, Coutzac C, et al. Baseline gut microbiota predicts clinical response and colitis in metastatic melanoma patients treated with ipilimumab. Ann Oncol 2017;28:1368-79.
55. Ascierto PA, Long GV, Robert C, et al. Survival outcomes in patients with previously untreated braf wild-type advanced melanoma treated with nivolumab therapy: three-year follow-up of a randomized phase 3 trial. JAMA Oncol 2019;5:187-94.
56. Vokes EE, Ready N, Felip E, et al. Nivolumab versus docetaxel in previously treated advanced non-small-cell lung cancer (checkmate 017 and checkmate 057): 3-year update and outcomes in patients with liver metastases. Ann Oncol 2018;29:959-65.
57. Escudier B, Motzer RJ, Sharma P, et al. Treatment beyond progression in patients with advanced renal cell carcinoma treated with nivolumab in checkmate 025. Eur Urol 2017;72:368-76.
58. Topalian SL, Sznol M, McDermott DF, et al. Survival, durable tumor remission, and long-term safety in patients with advanced melanoma receiving nivolumab. J Clin Oncol 2014;32:1020-30.
59. Weber JS, D'Angelo SP, Minor D, et al. Nivolumab versus chemotherapy in patients with advanced melanoma who progressed after anti-CTLA-4 treatment (checkmate 037): a randomised, controlled, open-label, phase 3 trial. Lancet Oncol 2015;16:375-84.
60. Derosa L, Routy B, Thomas AM, et al. Intestinal akkermansia muciniphila predicts clinical response to PD-1 blockade in patients with advanced non-small-cell lung cancer. Nat Med 2022;28:315-24.
61. Gupta KH, Nowicki C, Giurini EF, Marzo AL, Zloza A. Bacterial-based cancer therapy (BBCT): recent advances, current challenges, and future prospects for cancer immunotherapy. Vaccines 2021;9:1497.
62. Tang Q, Peng X, Xu B, Zhou X, Chen J, Cheng L. Current status and future directions of bacteria-based immunotherapy. Front Immunol 2022;13:911783.
63. Cheng WY, Wu CY, Yu J. The role of gut microbiota in cancer treatment: friend or foe? Gut 2020;69:1867-76.
64. Longhi G, van Sinderen D, Ventura M, Turroni F. Microbiota and cancer: the emerging beneficial role of bifidobacteria in cancer immunotherapy. Front Microbiol 2020;11:575072.
65. Sivan A, Corrales L, Hubert N, et al. Commensal bifidobacterium promotes antitumor immunity and facilitates anti-pd-l1 efficacy. Science 2015;350:1084-9.
66. Rong Y, Dong Z, Hong Z, et al. Reactivity toward bifidobacterium longum and enterococcus hirae demonstrate robust CD8+ t cell response and better prognosis in hbv-related hepatocellular carcinoma. Exp Cell Res 2017;358:352-9.
67. Luu M, Riester Z, Baldrich A, et al. Microbial short-chain fatty acids modulate CD8+ T cell responses and improve adoptive immunotherapy for cancer. Nat Commun 2021;12:4077.
68. Li X, Fu GF, Fan YR, et al. Bifidobacterium adolescentis as a delivery system of endostatin for cancer gene therapy: selective inhibitor of angiogenesis and hypoxic tumor growth. Cancer Gene Ther 2003;10:105-11.
69. Cronin M, Akin AR, Collins SA, et al. High resolution in vivo bioluminescent imaging for the study of bacterial tumour targeting. PLoS One 2012;7:e30940.
70. D'Amico F, Perrone AM, Rampelli S, et al. Gut microbiota dynamics during chemotherapy in epithelial ovarian cancer patients are related to therapeutic outcome. Cancers 2021;13:3999.
71. San-Millán I, Brooks GA. Reexamining cancer metabolism: lactate production for carcinogenesis could be the purpose and explanation of the warburg effect. Carcinogenesis 2017;38:119-33.
72. la Cruz-López KG, Castro-Muñoz LJ, Reyes-Hernández DO, García-Carrancá A, Manzo-Merino J. Lactate in the regulation of tumor microenvironment and therapeutic approaches. Front Oncol 2019;9:1143.
73. Vinasco K, Mitchell HM, Kaakoush NO, Castaño-Rodríguez N. Microbial carcinogenesis: lactic acid bacteria in gastric cancer. Biochim Biophys Acta Rev Cancer 2019;1872:188309.
74. Fidelle M, Rauber C, Alves Costa Silva C, et al. A microbiota-modulated checkpoint directs immunosuppressive intestinal T cells into cancers. Science 2023;380:eabo2296.
75. Sarkar T, Dhar S, Sa G. Tumor-infiltrating t-regulatory cells adapt to altered metabolism to promote tumor-immune escape. Curr Res Immunol 2021;2:132-41.
76. Hidalgo-Cantabrana C, Delgado S, Ruiz L, Ruas-Madiedo P, Sánchez B, Margolles A. Bifidobacteria and their health-promoting effects. Microbiol Spectr 2017;5(3).
77. Leu RK, Hu Y, Brown IL, Woodman RJ, Young GP. Synbiotic intervention of bifidobacterium lactis and resistant starch protects against colorectal cancer development in rats. Carcinogenesis 2010;31:246-51.
78. Roller M, Clune Y, Collins K, Rechkemmer G, Watzl B. Consumption of prebiotic inulin enriched with oligofructose in combination with the probiotics Lactobacillus rhamnosus and Bifidobacterium lactis has minor effects on selected immune parameters in polypectomised and colon cancer patients. Br J Nutr 2007;97:676-84.
79. Liu Z, Li C, Huang M, et al. Positive regulatory effects of perioperative probiotic treatment on postoperative liver complications after colorectal liver metastases surgery: a double-center and double-blind randomized clinical trial. BMC Gastroenterol 2015;15:34.
80. Liu ZH, Huang MJ, Zhang XW, et al. The effects of perioperative probiotic treatment on serum zonulin concentration and subsequent postoperative infectious complications after colorectal cancer surgery: a double-center and double-blind randomized clinical trial. Am J Clin Nutr 2013;97:117-26.
81. Wada M, Nagata S, Saito M, et al. Effects of the enteral administration of Bifidobacterium breve on patients undergoing chemotherapy for pediatric malignancies. Support Care Cancer 2010;18:751-9.
82. Lages PC, Generoso SV, Correia MITD. Postoperative symbiotic in patients with head and neck cancer: a double-blind randomised trial. Br J Nutr 2018;119:190-5.
83. Suez J, Zmora N, Zilberman-Schapira G, et al. Post-antibiotic gut mucosal microbiome reconstitution is impaired by probiotics and improved by autologous FMT. Cell 2018;174:1406-1423.e16.
84. Zmora N, Zilberman-Schapira G, Suez J, et al. Personalized gut mucosal colonization resistance to empiric probiotics is associated with unique host and microbiome features. Cell 2018;174:1388-1405.e21.
85. Morgan XC, Segata N, Huttenhower C. Biodiversity and functional genomics in the human microbiome. Trends Genet 2013;29:51-8.
86. Bajramagic S, Hodzic E, Mulabdic A, Holjan S, Smajlovic SV, Rovcanin A. Usage of probiotics and its clinical significance at surgically treated patients sufferig from colorectal carcinoma. Med Arch 2019;73:316-20.
87. Xie X, He Y, Li H, et al. Effects of prebiotics on immunologic indicators and intestinal microbiota structure in perioperative colorectal cancer patients. Nutrition 2019;61:132-42.
Cite This Article
Export citation file: BibTeX | RIS
OAE Style
Procaccianti G, Roggiani S, Conti G, Brigidi P, Turroni S, D’Amico F. Bifidobacterium in anticancer immunochemotherapy: friend or foe?. Microbiome Res Rep 2023;2:24. http://dx.doi.org/10.20517/mrr.2023.23
AMA Style
Procaccianti G, Roggiani S, Conti G, Brigidi P, Turroni S, D’Amico F. Bifidobacterium in anticancer immunochemotherapy: friend or foe?. Microbiome Research Reports. 2023; 2(3): 24. http://dx.doi.org/10.20517/mrr.2023.23
Chicago/Turabian Style
Procaccianti, Giorgia, Sara Roggiani, Gabriele Conti, Patrizia Brigidi, Silvia Turroni, Federica D’Amico. 2023. "Bifidobacterium in anticancer immunochemotherapy: friend or foe?" Microbiome Research Reports. 2, no.3: 24. http://dx.doi.org/10.20517/mrr.2023.23
ACS Style
Procaccianti, G.; Roggiani S.; Conti G.; Brigidi P.; Turroni S.; D’Amico F. Bifidobacterium in anticancer immunochemotherapy: friend or foe?. Microbiome. Res. Rep. 2023, 2, 24. http://dx.doi.org/10.20517/mrr.2023.23
About This Article
Special Issue
Copyright
Data & Comments
Data
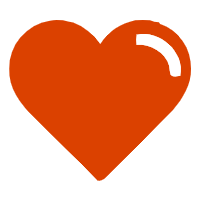

Comments
Comments must be written in English. Spam, offensive content, impersonation, and private information will not be permitted. If any comment is reported and identified as inappropriate content by OAE staff, the comment will be removed without notice. If you have any queries or need any help, please contact us at support@oaepublish.com.