Parathyroid carcinoma
Abstract
Parathyroid carcinoma is a rare but clinically-aggressive tumor. While most cases are sporadic, parathyroid cancer is overrepresented in hyperparathyroidism-jaw tumor syndrome, or rarely other heritable syndromes. Evidence suggests that sporadic parathyroid carcinomas rarely, if ever, evolve through an identifiable benign tumor intermediate. A few genes have been directly implicated in the pathogenesis of sporadic parathyroid cancer; somatic (and less common germline) mutations in the CDC73 tumor suppressor gene are the most frequent finding and the only firmly established molecular drivers of parathyroid cancer. Alterations in other important human cancer genes, including CCND1/cyclin D1, PIK3CA, MTOR and PRUNE2 have also been described in parathyroid cancer, however their abilities to drive malignant parathyroid tumorigenesis remains to be demonstrated experimentally.
Keywords
Introduction
Parathyroid cancer is a rare, but aggressive, cause of primary hyperparathyroidism, accounting for less than 1% of cases of this relatively common endocrine disorder. Parathyroid carcinoma may be suspected, prior to surgery, on the basis of clinical features. Parathyroid cancer presents equally in men and women, in contrast to the 3.5:1 female-to-male ratio seen with benign parathyroid tumors. Serum calcium levels are often markedly elevated in parathyroid cancer, with patients exhibiting renal and/or bone symptoms including nephrolithiasis, osteitis fibrosa cystica, osteoporosis and fracture. Palpable neck mass is common. While the majority of patients with parathyroid carcinoma are symptomatic, rare non-functioning parathyroid carcinomas have been reported. Histopathologic diagnosis of parathyroid cancer can be difficult and unequivocal diagnosis of malignancy depends on the presence of marked invasion into adjacent structures and/or distant metastasis. Features such as fibrous banding, mitotic figures and necrosis are suggestive of, but not diagnostic for, parathyroid carcinoma[1]. Historic inconsistencies in diagnostic criteria for parathyroid cancer, in addition to its rarity, have hindered efforts to understand the genetic basis of this important endocrine malignancy.
Genetic alterations in parathyroid cancer
A hallmark of many solid tumor malignancies is their progression from normal to malignant disease through clinically and histologically-identifiable stages of tumorigenesis, caused by incremental accumulation of acquired genetic abnormalities. Surprisingly, genetic evidence argues against such a progression for parathyroid carcinoma. Genomic and genetic alterations common in benign parathyroid adenomas, most prominently loss of chromosome 11q and accompanying mutation of MEN1, which occur in 35% of parathyroid adenomas[2-8], are rarely, if ever, seen in parathyroid cancer[3,4,8,9]. The clearly distinguishable patterns of genomic and genetic alterations present in parathyroid adenomas versus carcinomas suggests that parathyroid cancer most commonly arises de novo, rather than evolving from a preexisting benign adenoma[8].
Hereditary parathyroid cancer
A possible exception to the predominant process of de novo parathyroid carcinogenesis is that of hereditary parathyroid cancer. Primary hyperparathyroidism is a feature of several hereditary tumor-predisposition syndromes [Table 1]. While the large majority of parathyroid tumors in these syndromes are benign, rare parathyroid carcinomas have been reported in multiple endocrine neoplasia type 1 (MEN1) and type 2a (MEN2a). Somatic mutations of the causative genes, MEN1 and RET, respectively do not appear to contribute to sporadic parathyroid carcinomas. A somatic mutation in CDKN2C/p18, which may cause a subset of cases of MEN1 or a closely related syndrome[10], has been reported in a single parathyroid carcinoma[11].
Hereditary parathyroid tumor predisposition syndromes
Syndrome | Gene | Parathyroid cancer | Other features | Somatic mutations in sporadic parathyroid tumors |
---|---|---|---|---|
MEN1a | MEN1 | Rare | Pituitary and enteropancreatic endocrine tumors | Adenoma ~35% |
CDKN1Ab | None reported | None reported | ||
CDKN2Bb | None reported | None reported | ||
CDKN2Cb | None reported | Adenoma ~1.5% | ||
MEN4a | CDKN1Bb | None reported | Adenoma ~5% | |
MEN2A | RET | Rare | Medullary thyroid cancer, pheochromocytoma | None reported |
HPT-JT | CDC73 | ~10%-15% | Fibro-osseous jaw tumors, uterine tumors, renal lesions | Carcinoma ~77% |
Adenoma ~1.5% | ||||
NSHPT | CASR | None reported | None reported | |
FIHP | GCM2, others | None reported | None reported |
Parathyroid carcinoma is highly overrepresented in the hyperparathyroidism-jaw tumor syndrome (HPT-JT), caused by germline mutations in CDC73 (previously called HRPT2). Approximately 10%-15% of parathyroid tumors in HPT-JT are malignant and affected patients have a lifetime risk of developing parathyroid cancer approaching 40%[12]. In the setting of a germline parathyroid tumor-predisposing mutation, particularly of CDC73, patients may occasionally develop parathyroid carcinomas that have evolved from preexisting benign or atypical adenomas. Such cases likely explain the few rare reports of apparent malignant parathyroid tumor progression.
CDC73
A large percentage of sporadic parathyroid carcinomas also harbor mutations in the CDC73 tumor suppressor gene. A wide range of mutation frequencies (13%-100%) have been reported[13-16] across studies, likely due to inconsistencies in selection criteria. Among studies using the most stringent diagnostic criteria for parathyroid cancer, namely extracapsular invasion and/or distant metastasis, the mutation frequency is 77%[13-15]. In addition to intragenic mutations, gross deletions of CDC73 have also been reported[17-19]. Biallelic inactivation of CDC73 can be demonstrated in many parathyroid cancers[13-15]. A substantial subset of patients with sporadically-presenting parathyroid carcinoma possess germline CDC73 mutations, and may represent new index cases of HPT-JT or a phenotypic variant[13,15,20,21]. Most parathyroid carcinomas also exhibit aberrant immunohistochemical staining for parafibromin, the protein product of CDC73; complete loss of parafibromin expression is the most common staining pattern. As the large majority of benign parathyroid tumors (except in the setting of germline CDC73 mutation) display normal parafibromin staining, parafibromin immunohistochemistry may be considered as a diagnostic adjunct for parathyroid cancer in otherwise equivocal cases[22-24] but aberrant parafibromin staining alone is insufficient as a diagnostic marker of parathyroid carcinoma[25].
Parafibromin is a ubiquitously expressed, evolutionarily conserved 531 amino acid protein with predominantly nuclear expression. Cytoplasmic expression of parafibromin has also been described and may have functions different than nuclear parafibromin[26,27]. Parafibromin’s C-terminal region contains moderate sequence similarity to yeast Cdc73p, a cell-division protein that comprises part of the polymerase-associated factor 1 complex (Paf1c). The human PAF1 complex (hPAF1C) contains homologs of most of the same subunits and shares similar functions. hPAF1C associates with RNA polymerase II during transcriptional initiation and elongation and participates in some histone modifications and posttranscriptional events, including modification of the poly (a) tail. Cdc73p homologs in higher-level organisms contain a metazoan-specific N-terminal domain, capable of directly binding β-catenin, and function in Wnt signaling, a central regulator of development and proliferation[28]. Although parafibromin’s precise role in Wnt signaling, which might vary by cell type[28-30], has yet to be established, the involvement of parafibromin in canonical Wnt/β-catenin signaling provides a possible mechanism for parafibromin’s tumor suppressive function(s). Activation of canonical Wnt signaling leads to β-catenin-mediated gene transcription; targets of Wnt signaling include cyclin D1, a parathyroid oncogene (described further below)[31,32]. Parafibromin can inhibit cancer cell growth and cause G1 phase arrest in vitro, in part through effects on cyclin D1[33,34]. Loss of Wnt pathway components APC and GSK3β[35] and accumulation of β-catenin have also been described in parathyroid cancer[36]. Cytoplasmic parafibromin interacts with cytoskeletal proteins[26] and p53 mRNA, modulating p53-mediated apoptosis[27]. Parafibromin can also interact directly with the SV40 large T antigen; cell lines expressing SV40 large T exhibit different effects on proliferation subsequent to perturbation of parafibromin levels[37], a finding which has complicated interpretation of some in vitro functional analyses.
Conventional and conditional transgenic mouse knockouts of CDC73 have been developed. Homozygous germline deletion of CDC73 is embryonic lethal and germline deletion of CDC73 at later stages of development led to death within 20 days; increased apoptosis was observed in many tissues[38]. No parathyroid gland abnormalities were initially described in either CDC73 knockout[38]. A later study, which followed heterozygous CDC73 knockout mice out to 21 months, reported increased parathyroid proliferation and histologic abnormalities commonly observed in atypical parathyroid adenomas and parathyroid carcinomas in humans; frank features of parathyroid cancer, such as local invasion or distant metastasis were not described. Deletion of CDC73 targeted to the parathyroid glands, by crossing floxed-CDC73 mice with PTH-Cre mice, resulted in similar parathyroid gland abnormalities; heterozygous and homozygous null mice were both affected[39]. Further studies are necessary to understand how loss of CDC73 expression promotes parathyroid tumorigenesis.
Cyclin D1
CCND1, encoding cyclin D1, is a well-established oncogenic contributor to benign parathyroid adenomas and DNA amplifications or gene rearrangements have been demonstrated in a variety of tumor types. CCND1 gene amplification[40,41] and cyclin D1 overexpression[40,42] are common in parathyroid carcinoma. Parathyroid-targeted cyclin D1 transgenic mice develop chronic biochemical hyperparathyroidism and parathyroid gland hypercellularity, but parathyroid carcinoma has not been observed[43]. These finding suggest that cyclin D1 overexpression alone may be insufficient to drive malignant parathyroid tumorigenesis. The precise mechanisms through which cyclin D1 drives tumorigenesis remain controversial. Cyclin D1’s primary function is as a regulator of cell cycle progression, binding to, and activating, the cyclin-dependent kinases CDK4/CDK6, which can then phosphorylate pRB, promoting G1-S phase transition. Loss of pRB expression is also a frequent finding in parathyroid carcinomas[44,45]. Cyclin D1 levels are tightly regulated at multiple levels and dysfunction of any of these control mechanisms may contribute to tumorigenesis[46]. Cyclin D1 also has CDK-independent functions, such as a role in chromosomal stability, which may also contribute to cyclin D1’s ability to drive tumorigenesis[47]. While it remains to be determined which functions of cyclin D1 are most relevant, the frequent loss of pRB expression and occasional inactivation of CDK inhibitor genes in parathyroid cancer underscore the importance of cell cycle dysregulation to the promotion of malignant parathyroid tumorigenesis.
PRUNE2
PRUNE2 has recently been identified as a likely tumor suppressor gene subject to recurrent mutation in parathyroid carcinoma[41,48]. Whole exome sequencing revealed one parathyroid carcinoma with a germline missense PRUNE2 mutation accompanied by allelic loss[48] and two carcinomas harboring biallelic, somatic nonsense mutations[41,48]. Sanger sequencing, limited to exon 8 of PRUNE2, uncovered two additional somatic missense mutations. PRUNE2 functions to suppress Ras homolog family member A (RhoA) activity, resulting in suppression of oncogenic cellular transformation, consistent with a tumor suppressive role in parathyroid carcinoma. Functional studies will be needed to determine if loss of PRUNE2 is capable of, or sufficient to, driving malignant parathyroid tumorigenesis.
PI3K/mTOR
The phosphatidylinositol 3-kinase (PI3K)/mammalian target of rapamycin (mTOR) pathway is an important regulator of cell cycle progression, cell growth and survival, and is frequently subject to alteration in human cancers. PIK3CA, encoding the p110-alpha subunit of PI3K, has been recognized as an oncogene capable of driving tumorigenesis in many types of human tumors. A heterozygous PIK3CA mutation, resulting in a glutamic acid to lysine change at amino acid 545 (p.E545K), was identified in a parathyroid carcinoma subjected to whole genome sequencing, but interestingly this mutation was absent from two recurrent lesions from the same patient[11]. Whole exome sequencing revealed another established activating PIK3CA mutation (p.K111E) in a parathyroid carcinoma[41]. Targeted sequencing revealed two additional known activating PIK3CA mutations, p.H1047R and p.E545A. Interestingly, across the two studies, PIK3CA and CDC73 mutations were mutually exclusive, although the sample sizes were too low to determine statistical significance. Activating mutations of MTOR, also commonly altered in human tumors, were seen in three parathyroid carcinomas in two next-generation sequencing studies[11,41]. Functional studies are required to determine if activating mutations of the PI3K/mTOR pathway are indeed capable of driving malignant parathyroid tumorigenesis.
Additional genetic and genomic considerations
A number of studies have sought to identify regions of genomic gains and losses relevant to the pathogenesis of parathyroid carcinoma. Recurrent regions of allelic loss have been reported on chromosomes 1p, 3, 13q and 14, and recurrent regions of allelic gain on chromosomes 1q and 16[3-5,9,49]. Such regions of recurrent genomic alteration are expected to harbor important tumor suppressor genes and oncogenes, however both targeted sequencing and whole genome/exome analyses have yet to uncover commonly altered tumor suppressor genes or oncogenes within those predicted genomic locations[11,41,44,48,50-54]. Notably, despite the high frequency of both allelic loss at the RB1 locus on chromosome 13q[44,54-57] and loss of pRB expression[44,45], intragenic mutation of RB1 has yet to be identified in parathyroid cancer[11,41,48,54]. Preferential amplification of mutant CDC73 alleles has been reported, which could account, at least in part, for observed alleic gain of chromosome 1q[48]. Whole genome/exome next-generation sequence analyses have identified a number of genes that may be important to the pathogenesis of parathyroid cancer and merit further study. Recurrent mutations have been reported in AKAP9, a gene frequently altered in epithelial cancers, ADCK1, a putative kinase, NOTCH1, which may function as either an oncogene or tumor suppressor gene, and ZEB1, a transcriptional regulator of epithelial-mesenchymal transition[41]. Several mutations which were identified in only one tumor but affecting genes linked to other types of human cancer were also identified. These genes included MLL2, a MEN1-interacting tumor suppressor gene, THRAP3, a gene involved in regulating cyclin D1 expression, and the canonical Wnt pathway genes APC and RNF43. Several genes encoding kinases with postulated roles in cell migration and invasion, including MAP3K11, JAK1 and RIOK3, and chromatin structure-regulating genes, including ARID2, ARID4A, KDM5C, KDM4C, KDM4E, JMJD1C and SETD1B were also mutated. Identification of these novel mutations is an important next step in furthering our knowledge of the molecular pathogenesis of parathyroid cancer. It remains to be determined, through further sequence analysis and functional studies, which of these mutated genes will emerge as important driver genes in parathyroid cancer.
Conclusion
Several important advances have been made towards the goal of understanding the molecular basis of parathyroid cancer. Observations of mutational and allelic imbalance patterns suggest that parathyroid cancer generally arises de novo, rather than evolving from a preexisting typical benign adenoma. Mutations in the CDC73 tumor suppressor gene are the most common finding in malignant parathyroid carcinomas. Alterations in additional genes such as CCND1/CyclinD1, PIK3CA, MTOR and PRUNE2 and others identified by next-generation sequencing methods have also been described in parathyroid cancer, however their abilities to drive malignant parathyroid tumorigenesis remains to be demonstrated experimentally. Additional genes important to the development of parathyroid carcinoma are likely to be identified and the extent and nature of their involvement will need to be carefully examined and validated with genetic and experimental-functional approaches.
Declarations
Authors’ contributionsCosta-Guda J. contributed solely to the paper.
Financial support and sponsorshipThis work was supported in part by the Murray-Heilig Fund in Molecular Medicine.
Conflicts of interestThere are no conflicts of interest.
Patient consentNot applicable.
Ethics approvalNot applicable.
Copyright© The Author(s) 2018.
REFERENCES
1. DeLellis RA, Arnold A, Bilezikian JP, Eng C, Larsson C, Lloyd RV, Mete O. Parathyroid Carcinoma. In: WHO Classification of Tumours of Endocrine Organs. Lyon: IARC; 2017.
2. Tahara H, Smith AP, Gas RD, Cryns VL, Arnold A. Genomic localization of novel candidate tumor suppressor gene loci in human parathyroid adenomas. Cancer Res 1996;56:599-605.
3. Agarwal SK, Schrock E, Kester MB, Burns AL, Heffess CS, Ried T, Marx SJ. Comparative genomic hybridization analysis of human parathyroid tumors. Cancer Genet Cytogenet 1998;106:30-6.
4. Farnebo F, Kytola S, Teh BT, Dwight T, Wong FK, Hoog A, Elvius M, Wassif WS, Thompson NW, Farnebo LO, Sandelin K, Larsson C. Alternative genetic pathways in parathyroid tumorigenesis. J Clin Endocrinol Metab 1999;84:3775-80.
5. Hunt JL, Carty SE, Yim JH, Murphy J, Barnes L. Allelic loss in parathyroid neoplasia can help characterize malignancy. Am J Surg Pathol 2005;29:1049-55.
6. Cromer MK, Starker LF, Choi M, Udelsman R, Nelson-Williams C, Lifton RP, Carling T. Identification of somatic mutations in parathyroid tumors using whole-exome sequencing. J Clin Endocrinol Metab 2012;97:E1774-81.
7. Newey PJ, Nesbit MA, Rimmer AJ, Attar M, Head RT, Christie PT, Gorvin CM, Stechman M, Gregory L, Mihai R, Sadler G, McVean G, Buck D, Thakker RV. Whole-exome sequencing studies of nonhereditary (sporadic) parathyroid adenomas. J Clin Endocrinol Metab 2012;97:E1995-2005.
8. Costa-Guda J, Imanishi Y, Palanisamy N, Kawamata N, Phillip Koeffler H, Chaganti RS, Arnold A. Allelic imbalance in sporadic parathyroid carcinoma and evidence for its de novo origins. Endocrine 2013;44:489-95.
9. Kytola S, Farnebo F, Obara T, Isola J, Grimelius L, Farnebo LO, Sandelin K, Larsson C. Patterns of chromosomal imbalances in parathyroid carcinomas. Am J Pathol 2000;157:579-86.
10. Costa-Guda J, Soong CP, Parekh VI, Agarwal SK, Arnold A. Germline and somatic mutations in cyclin-dependent kinase inhibitor genes CDKN1A, CDKN2B, and CDKN2C in sporadic parathyroid adenomas. Horm Cancer 2013;4:301-7.
11. Kasaian K, Wiseman SM, Thiessen N, Mungall KL, Corbett RD, Qian JQ, Nip KM, He A, Tse K, Chuah E, Varhol RJ, Pandoh P, McDonald H, Zeng T, Tam A, Schein J, Birol I, Mungall AJ, Moore RA, Zhao Y, Hirst M, Marra MA, Walker BA, Jones SJ. Complete genomic landscape of a recurring sporadic parathyroid carcinoma. J Pathol 2013;230:249-60.
12. Mehta A, Patel D, Rosenberg A, Boufraqech M, Ellis RJ, Nilubol N, Quezado MM, Marx SJ, Simonds WF, Kebebew E. Hyperparathyroidism-jaw tumor syndrome: results of operative management. Surgery 2014;156:1315-24; discussion 1324-5.
13. Shattuck TM, Valimaki S, Obara T, Gaz RD, Clark OH, Shoback D, Wierman ME, Tojo K, Robbins CM, Carpten JD, Farnebo LO, Larsson C, Arnold A. Somatic and germ-line mutations of the HRPT2 gene in sporadic parathyroid carcinoma. N Engl J Med 2003;349:1722-9.
14. Howell VM, Haven CJ, Kahnoski K, Khoo SK, Petillo D, Chen J, Fleuren GJ, Robinson BG, Delbridge LW, Philips J, Nelson AE, Krause U, Hammje K, Dralle H, Hoang-Vu C, Gimm O, Marsh DJ, Morreau H, Teh BT. HRPT2 mutations are associated with malignancy in sporadic parathyroid tumours. J Med Genet 2003;40:657-63.
15. Cetani F, Pardi E, Borsari S, Viacava P, Dipollina G, Cianferotti L, Ambrogini E, Gazzerro E, Colussi G, Berti P, Miccoli P, Pinchera A, Marcocci C. Genetic analyses of the HRPT2 gene in primary hyperparathyroidism: germline and somatic mutations in familial and sporadic parathyroid tumors. J Clin Endocrinol Metab 2004;89:5583-91.
16. Haven CJ, van Puijenbroek M, Tan MH, Teh BT, Fleuren GJ, van Wezel T, Morreau H. Identification of MEN1 and HRPT2 somatic mutations in paraffin-embedded (sporadic) parathyroid carcinomas. Clin Endocrinol (Oxf) 2007;67:370-6.
17. Cascon A, Huarte-Mendicoa CV, Javier Leandro-Garcia L, Leton R, Suela J, Santana A, Costa MB, Comino-Mendez I, Landa I, Sanchez L, Rodriguez-Antona C, Cigudosa JC, Robledo M. Detection of the first gross CDC73 germline deletion in an HPT-JT syndrome family. Genes Chromosomes Cancer 2011;50:922-9.
18. Domingues R, Tomaz RA, Martins C, Nunes C, Bugalho MJ, Cavaco BM. Identification of the first germline HRPT2 whole-gene deletion in a patient with primary hyperparathyroidism. Clin Endocrinol (Oxf) 2012;76:33-8.
19. Bricaire L, Odou MF, Cardot-Bauters C, Delemer B, North MO, Salenave S, Vezzosi D, Kuhn JM, Murat A, Caron P, Sadoul JL, Silve C, Chanson P, Barlier A, Clauser E, Porchet N, Groussin L. Frequent large germline HRPT2 deletions in a French National cohort of patients with primary hyperparathyroidism. J Clin Endocrinol Metab 2013;98:E403-8.
20. Guarnieri V, Scillitani A, Muscarella LA, Battista C, Bonfitto N, Bisceglia M, Minisola S, Mascia ML, D'Agruma L, Cole DE. Diagnosis of parathyroid tumors in familial isolated hyperparathyroidism with HRPT2 mutation: implications for cancer surveillance. J Clin Endocrinol Metab 2006;91:2827-32.
21. Arnold A, Marx SJ. Familial Hyperparathyroidism. In: Primer on the metabolic bone diseases and disorders of mineral metabolism 2008:361-6.
22. Tan MH, Morrison C, Wang P, Yang X, Haven CJ, Zhang C, Zhao P, Tretiakova MS, Korpi-Hyovalti E, Burgess JR, Soo KC, Cheah WK, Cao B, Resau J, Morreau H, Teh BT. Loss of parafibromin immunoreactivity is a distinguishing feature of parathyroid carcinoma. Clin Cancer Res 2004;10:6629-37.
23. Gill AJ, Clarkson A, Gimm O, Keil J, Dralle H, Howell VM, Marsh DJ. Loss of nuclear expression of parafibromin distinguishes parathyroid carcinomas and hyperparathyroidism-jaw tumor (HPT-JT) syndrome-related adenomas from sporadic parathyroid adenomas and hyperplasias. Am J Surg Pathol 2006;30:1140-9.
24. Cetani F, Ambrogini E, Viacava P, Pardi E, Fanelli G, Naccarato AG, Borsari S, Lemmi M, Berti P, Miccoli P, Pinchera A, Marcocci C. Should parafibromin staining replace HRTP2 gene analysis as an additional tool for histologic diagnosis of parathyroid carcinoma? Eur J Endocrinol 2007;156:547-54.
26. Agarwal SK, Simonds WF, Marx SJ. The parafibromin tumor suppressor protein interacts with actin-binding proteins actinin-2 and actinin-3. Mol Cancer 2008;7:65.
27. Jo JH, Chung TM, Youn H, Yoo JY. Cytoplasmic parafibromin/hCdc73 targets and destabilizes p53 mRNA to control p53-mediated apoptosis. Nat Commun 2014;5:5433.
28. Mosimann C, Hausmann G, Basler K. Parafibromin/Hyrax activates Wnt/Wg target gene transcription by direct association with beta-catenin/Armadillo. Cell 2006;125:327-41.
29. James RG, Biechele TL, Conrad WH, Camp ND, Fass DM, Major MB, Sommer K, Yi X, Roberts BS, Cleary MA, Arthur WT, MacCoss M, Rawlings DJ, Haggarty SJ, Moon RT. Bruton's tyrosine kinase revealed as a negative regulator of Wnt-beta-catenin signaling. Sci Signal 2009;2:ra25.
30. Takahashi A, Tsutsumi R, Kikuchi I, Obuse C, Saito Y, Seidi A, Karisch R, Fernandez M, Cho T, Ohnishi N, Rozenblatt-Rosen O, Meyerson M, Neel BG, Hatakeyama M. SHP2 tyrosine phosphatase converts parafibromin/Cdc73 from a tumor suppressor to an oncogenic driver. Mol Cell 2011;43:45-56.
31. Shtutman M, Zhurinsky J, Simcha I, Albanese C, D'Amico M, Pestell R, Ben-Ze'ev A. The cyclin D1 gene is a target of the beta-catenin/LEF-1 pathway. Proc Natl Acad Sci U S A 1999;96:5522-7.
32. Tetsu O, McCormick F. Beta-catenin regulates expression of cyclin D1 in colon carcinoma cells. Nature 1999;398:422-6.
33. Woodard GE, Lin L, Zhang JH, Agarwal SK, Marx SJ, Simonds WF. Parafibromin, product of the hyperparathyroidism-jaw tumor syndrome gene HRPT2, regulates cyclin D1/PRAD1 expression. Oncogene 2005;24:1272-6.
34. Lin L, Zhang JH, Panicker LM, Simonds WF. The parafibromin tumor suppressor protein inhibits cell proliferation by repression of the c-myc proto-oncogene. Proc Natl Acad Sci U S A 2008;105:17420-5.
35. Juhlin CC, Haglund F, Villablanca A, Forsberg L, Sandelin K, Branstrom R, Larsson C, Hoog A. Loss of expression for the Wnt pathway components adenomatous polyposis coli and glycogen synthase kinase 3-beta in parathyroid carcinomas. Int J Oncol 2009;34:481-92.
36. Svedlund J, Auren M, Sundstrom M, Dralle H, Akerstrom G, Bjorklund P, Westin G. Aberrant WNT/beta-catenin signaling in parathyroid carcinoma. Mol Cancer 2010;9:294.
37. Iwata T, Mizusawa N, Taketani Y, Itakura M, Yoshimoto K. Parafibromin tumor suppressor enhances cell growth in the cells expressing SV40 large T antigen. Oncogene 2007;26:6176-83.
38. Wang P, Bowl MR, Bender S, Peng J, Farber L, Chen J, Ali A, Zhang Z, Alberts AS, Thakker RV, Shilatifard A, Williams BO, Teh BT. Parafibromin, a component of the human PAF complex, regulates growth factors and is required for embryonic development and survival in adult mice. Mol Cell Biol 2008;28:2930-40.
39. Walls GV, Stevenson M, Lines KE, Newey PJ, Reed AAC, Bowl MR, Jeyabalan J, Harding B, Bradley KJ, Manek S, Chen J, Wang P, Williams BO, Teh BT, Thakker RV. Mice deleted for cell division cycle 73 gene develop parathyroid and uterine tumours: model for the hyperparathyroidism-jaw tumour syndrome. Oncogene 2017;36:4025-36.
40. Zhao L, Sun LH, Liu DM, He XY, Tao B, Ning G, Liu JM, Zhao HY. Copy number variation in CCND1 gene is implicated in the pathogenesis of sporadic parathyroid carcinoma. World J Surg 2014;38:1730-7.
41. Pandya C, Uzilov AV, Bellizzi J, Lau CY, Moe AS, Strahl M, Hamou W, Newman LC, Fink MY, Antipin Y, Yu W, Stevenson M, Cavaco BM, Teh BT, Thakker RV, Morreau H, Schadt EE, Sebra R, Li SD, Arnold A, Chen R. Genomic profiling reveals mutational landscape in parathyroid carcinomas. JCI Insight 2017;2:e92061.
42. Vasef MA, Brynes RK, Sturm M, Bromley C, Robinson RA. Expression of cyclin D1 in parathyroid carcinomas, adenomas, and hyperplasias: a paraffin immunohistochemical study. Mod Pathol 1999;12:412-6.
43. Imanishi Y, Hosokawa Y, Yoshimoto K, Schipani E, Mallya S, Papanikolaou A, Kifor O, Tokura T, Sablosky M, Ledgard F, Gronowicz G, Wang TC, Schmidt EV, Hall C, Brown EM, Bronson R, Arnold A. Primary hyperparathyroidism caused by parathyroid-targeted overexpression of cyclin D1 in transgenic mice. J Clin Invest 2001;107:1093-102.
44. Cryns VL, Thor A, Xu HJ, Hu SX, Wierman ME, Vickery AL, Jr. , Benedict WF, Arnold A. Loss of the retinoblastoma tumor-suppressor gene in parathyroid carcinoma. N Engl J Med 1994;330:757-61.
45. Cetani F, Pardi E, Viacava P, Pollina GD, Fanelli G, Picone A, Borsari S, Gazzerro E, Miccoli P, Berti P, Pinchera A, Marcocci C. A reappraisal of the Rb1 gene abnormalities in the diagnosis of parathyroid cancer. Clin Endocrinol (Oxf) 2004;60:99-106.
46. Kim JK, Diehl JA. Nuclear cyclin D1: an oncogenic driver in human cancer. J Cell Physiol 2009;220:292-6.
47. Casimiro MC, Arnold A, Pestell RG. Kinase independent oncogenic cyclin D1. Aging (Albany NY) 2015;7:455-6.
48. Yu W, McPherson JR, Stevenson M, van Eijk R, Heng HL, Newey P, Gan A, Ruano D, Huang D, Poon SL, Ong CK, van Wezel T, Cavaco B, Rozen SG, Tan P, Teh BT, Thakker RV, Morreau H. Whole-exome sequencing studies of parathyroid carcinomas reveal novel PRUNE2 mutations, distinctive mutational spectra related to APOBEC-catalyzed DNA mutagenesis and mutational enrichment in kinases associated with cell migration and invasion. J Clin Endocrinol Metab 2015;100:E360-4.
49. Sulaiman L, Haglund F, Hashemi J, Obara T, Nordenstrom J, Larsson C, Juhlin CC. Genome-wide and locus specific alterations in CDC73/HRPT2-mutated parathyroid tumors. PLoS One 2012;7:e46325.
50. Friedman E, Bale AE, Marx SJ, Norton JA, Arnold A, Tu T, Aurbach GD, Spiegel AM. Genetic abnormalities in sporadic parathyroid adenomas. J Clin Endocrinol Metab 1990;71:293-7.
51. Yoshimoto K, Iwahana H, Fukuda A, Sano T, Katsuragi K, Kinoshita M, Saito S, Itakura M. ras mutations in endocrine tumors: mutation detection by polymerase chain reaction-single strand conformation polymorphism. Jpn J Cancer Res 1992;83:1057-62.
52. Cryns VL, Rubio MP, Thor AD, Louis DN, Arnold A. p53 abnormalities in human parathyroid carcinoma. J Clin Endocrinol Metab 1994;78:1320-4.
53. Hakim JP, Levine MA. Absence of p53 point mutations in parathyroid adenoma and carcinoma. J Clin Endocrinol Metab 1994;78:103-6.
54. Shattuck TM, Kim TS, Costa J, Yandell DW, Imanishi Y, Palanisamy N, Gaz RD, Shoback D, Clark OH, Monchik JM, Wierman ME, Hollenberg A, Tojo K, Chaganti RS, Arnold A. Mutational analyses of RB and BRCA2 as candidate tumour suppressor genes in parathyroid carcinoma. Clin Endocrinol (Oxf) 2003;59:180-9.
55. Dotzenrath C, Teh BT, Farnebo F, Cupisti K, Svensson A, Toell A, Goretzki P, Larsson C. Allelic loss of the retinoblastoma tumor suppressor gene: a marker for aggressive parathyroid tumors? J Clin Endocrinol Metab 1996;81:3194-6.
56. Pearce SH, Trump D, Wooding C, Sheppard MN, Clayton RN, Thakker RV. Loss of heterozygosity studies at the retinoblastoma and breast cancer susceptibility (BRCA2) loci in pituitary, parathyroid, pancreatic and carcinoid tumours. Clin Endocrinol (Oxf) 1996;45:195-200.
Cite This Article

How to Cite
Download Citation
Export Citation File:
Type of Import
Tips on Downloading Citation
Citation Manager File Format
Type of Import
Direct Import: When the Direct Import option is selected (the default state), a dialogue box will give you the option to Save or Open the downloaded citation data. Choosing Open will either launch your citation manager or give you a choice of applications with which to use the metadata. The Save option saves the file locally for later use.
Indirect Import: When the Indirect Import option is selected, the metadata is displayed and may be copied and pasted as needed.
About This Article
Special Issue
Copyright
Data & Comments
Data
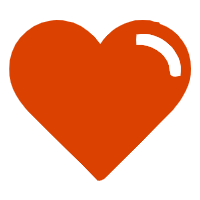
Comments
Comments must be written in English. Spam, offensive content, impersonation, and private information will not be permitted. If any comment is reported and identified as inappropriate content by OAE staff, the comment will be removed without notice. If you have any queries or need any help, please contact us at [email protected].