Determination of tetramethylammonium hydroxide in air using ion chromatography or ultra-performance liquid chromatography - high-resolution mass spectrometry
Abstract
Tetramethylammonium hydroxide (TMAH) is extensively utilized in the semiconductor and optoelectronic industries, serving as either a developer or an etchant. TMAH possesses acute toxicity and alkaline corrosiveness, having caused several fatal and injurious accidents in Taiwan. However, there is currently a lack of methods available for assessing TMAH exposure. This study developed a method involving sampling with quartz fiber filters followed by ultrasonic agitation in 10 mL mobile phase, filtering, and analysis using either ion chromatography (IC) or ultra-performance liquid chromatography - high-resolution mass spectrometry (UPLC-HRMS). Utilizing the characteristics of HRMS, the compound analyzed was identified as tetramethylammonium ion (TMA+) through mass spectrometry analysis. The method exhibits excellent trapping capacity and recovery rate. The linear range of TMA+ for IC is 0.3 to 100 μg/mL, with a correlation coefficient of R = 0.9998. The linear range of TMA+ for UPLC-HRMS is 0.002 to 1 μg/mL, with a correlation coefficient of R = 0.996. The recovery rates for IC and HRMS were 116.6% and 90.6%, with corresponding coefficient of variation (CV) of 2.25% and 3.53%, respectively. Samples can be stored at room temperature for 28 days. Verification of this method was conducted at the TMAH factory, and the air concentrations measured in the TMAH filling area were 3.29 and 4.75 μg/m3 from IC and UPLC-HRMS, respectively. This method will be applied in the on-site analysis of occupational hazards for operators in chemical plants, technology factories, and recycling plants, aiming to evaluate risk values to protect the safety and health of workers and prevent occupational diseases.
Keywords
INTRODUCTION
The tetramethylammonium ion (TMA+, C4H13N+), a basic quaternary ammonium compound, was first isolated from a sea anemone in 1923[1]. The hydroxide salt, tetramethylammonium hydroxide [TMAH; (CH3)4NOH, CAS No. 75-59-2], functions as an etchant or developer commonly used in the optoelectronic and semiconductor industries[2]. According to a survey by Taiwan’s Industrial Technology Research Institute, approximately 2,000,000 tons of TMAH are used in Taiwan each year[3]. Numerous semiconductor facilities in mainland China, Japan, South Korea, and the USA also use TMAH. TMAH is known as an alkaline corrosive and cholinergic agonist, capable of causing chemical skin burns and systemic toxicity[4]. Dermal exposure to TMAH can result in fatal intoxication, and it is increasingly becoming a serious concern in Taiwan[5,6,7]. The structure of the TMA+ resembles the cationic component of acetylcholine. TMA+ can stimulate muscarinic or nicotinic autonomic ganglia, potentially leading to a depolarization blockade[2]. The neurological effects of TMA+ intoxication include respiratory muscle paralysis caused by ganglionic blockade. Cholinergic symptoms resulting from acetylcholine accumulation were first reported in Taiwanese patients in 2010[7]. There have also been cases in South Korea where workers died from splashing TMAH[8].
Currently, the detection methods for TMAH mainly focus on testing TMAH in sewage or solutions, which can be divided into acid titration, conductivity measurement, ion chromatography (IC), and liquid chromatography-mass spectrometry (LC-MS/MS). Wang et al. targeted the OH- group in the structure of TMAH compounds, using phenolphthalein as an indicator and titrating with standard hydrochloric acid solution to determine the concentration of TMAH, achieving a measurement accuracy of up to
Hundreds of thousands of workers in Taiwan who are engaged in manufacturing, transportation, storage, or waste reduction face the risk of exposure to TMAH[14]. TMAH has been reported to cause severe burning sensations in the eyes, nose, throat, lungs, and skin[15]. However, there is currently a lack of analytical methods that can assess the TMAH exposure concentrations in labor working environments. Therefore, the goal of this study is to develop a sampling and IC or ultra-performance liquid chromatography - high-resolution mass spectrometry (UPLC-HRMS) analysis method for TMAH in the working environment, and to use UPLC-HRMS to verify the accuracy of the method. This method can be used not only to assess workers’ exposure to TMAH in the workplace environment but also to evaluate environmental hazardous substance exposure and health risk assessment.
EXPERIMENTAL
Materials
Deionized water (18.2 MΩ), prepared using a Milli-Q Plus system (Millipore, USA), was used. Tetramethylammonium chloride (TMACl) 98% was purchased from Thermo Fisher Scientific (Bremen, Germany). Sulphuric acid 95% was purchased from Sigma-Aldrich (St. Louis, MO, USA). Methanesulfonic Acid 98% was purchased from Alfa Aesar (Haverhill, MA, USA). 1.0 μm 37 mm polytetrafluoroethylene (PTFE), GLA-5000 5.0 μm 37 mm polyvinyl chloride (PVC), and 2500QAT-UP 37 mm Tissuquartz Fiber filter were purchased from PALL (Port Washington, NY, USA). 0.8 μm 37 mm mixed-cellulose ester (MCE) filter and 1.0 μm 37 mm Glass Fiber filter were purchased from SKC (Eighty-Four, PA, USA).
IC
A SHINE Model D-120 ion chromatography system with a suppressed conductivity detector (Qingdao, China) was used, consisting of a Model SH-120A pump, a Model SHA-15 auto sample injector, a Model SHY-C-3 suppressor, a Model SHD-6 conductivity detector, a column oven, a SHINE SH-G-1 (50 mm ×
UPLC-HRMS
An Ultimate 3000 ultra-performance liquid chromatography system (Thermo Fisher Scientific, Bremen, Germany) coupled with a Q Exactive Plus Hybrid Quadrupole-Orbitrap mass spectrometry (Thermo Fisher Scientific, Bremen, Germany) was used to obtain the full scan and HRMS data. The samples (5 µL) were chromatographed on a Phenomenex Luna C18 Column (130 Å, 1.7 mm, 2.1 mm × 100 mm) and the column temperature was maintained at 45 °C. The mobile phase consisted of 0.1% formic acid in water with 2% acetonitrile (A) and 0.1% formic acid in acetonitrile (B). The UPLC flow rate was set to 250 μL/min. The UPLC gradient started with 98% A for 1 min, then linearly increased to 95% B over 11 min, and was held at 95% B for 2 min. Finally, the gradient was returned to 98% A for 1 min and held at 98% A for an additional minute to re-equilibrate the system. Positive ionization mode was employed with an electrospray voltage of 4.0 kV. The instrumental settings were as follows: sheath gas flow rate of 35 arbitrary units, auxiliary gas flow rate of 10 arbitrary units, sweep gas flow rate of 0.02 arbitrary units, and capillary temperature of
Sampling method
TMAH dissolved in water solutions exists in an ionic state and can evaporate into the air as aerosol particulate matter. Therefore, the “filter paper collection method” is adopted. However, there are many types of filter paper, and it is necessary to determine which one is suitable. Six different filter papers including Quartz Fiber, PTFE, PVC, Glass Fiber, and MCE will be tested. After obtaining the optimal sampling medium, the maximum amount of TMAH aerosol droplets that can be collected by the medium will be tested. This is to prevent underestimation of measurement results in the future due to TMAH aerosol droplets in the environment exceeding the loading capacity of the medium, and to determine whether the collected TMAH will be washed away during elution. A sampling pump was set to a flow rate of 4 L/min (the highest flow rate typically used for ambient sampling), allowing air to pass through the quartz fiber filter containing TMA+.
Sample preparation
Optimization of sample preparation is conducted as follows: Step 1: Desorption solvent. Due to the higher water solubility of TMAH, desorption solution tests are carried out with water and mobile phase solutions; Step 2: Desorption method. Static settling, shaking, and ultrasonic agitation are tested for 30 min each; Step 3: Desorption time. Testing the effect of desorption efficiency at 5, 15, and 30 min. After obtaining the optimal analytical conditions through the three steps, 25, 50, and 100 μL of 10 mg/mL TMA+ were added to the filter for testing to determine whether the sample pretreatment conditions meet the requirements for different concentrations.
Sample storage
Add 40 μL of 1 mg/mL TMA+ to quartz fiber filters. Store 7 samples at room temperature (20 to 25 °C) and under refrigeration (< 4 °C) (6 with added samples and 1 blank sample) for testing on days 1, 7, 14, 21, and 28, totaling 70 samples. On the preparation day (day 1), analysis is conducted on samples stored at both room temperature and under refrigeration on days 7, 14, 21, and 28. The relative recovery rate is calculated by comparing the average of each test group to the test value from day 1. The average of the test results after day 7 should be within 90%-110% of the average of the first test. Otherwise, the experiment is stopped, and the last successful test day is considered the stable storage period of the sample. If stability is maintained for 28 days, then 28 days will be considered the maximum stable storage period.
RESULTS AND DISCUSSION
Sampling materials
TMAH solution, which is released into the air as water vapor, becomes an aerosol particulate pollutant. Therefore, the “filter collection method” was chosen. However, with many types of filter papers available, it is necessary to determine which type is most suitable. Six different types of filters commonly used for air sampling in occupational hygiene were tested to evaluate their efficiency in collecting TMAH: Quartz Fiber, PTFE, PVC, Glass Fiber, and MCE. On the day before the analysis, 40 μL of 1 mg/mL TMA+ was added to each type of filter paper, resulting in a total of 30 samples (6 samples per filter type). The filters were placed in 15 mL plastic centrifuge tubes. The filters were placed in 15 mL plastic centrifuge tubes, and 10 mL of
Extraction recoveries of five sampling materials (n = 6)
Filter | Recovery (%) | Precision (%) |
Quartz fiber | 100.5 | 0.63 |
PTFE | 101.9 | 0.68 |
PVC | 100.9 | 0.98 |
Glass fiber | 96.5 | 1.22 |
MCE | 72.9 | 2.31 |
Retention efficiency
This test aimed to determine whether TMAH captured by the sampling medium would be washed away during sampling, potentially leading to an underestimation of the measurement results. Forty microliters (40 μL) of 1 mg/mL TMA+ were added to six quartz fiber filters one day before sample preparation. After allowing TMA+ to be fully absorbed, the next day, a sampling pump was set to a flow rate of 4 L/min, and air was passed through the quartz fiber filters containing TMA+ for 6 h. The filters were then placed in 15 mL plastic centrifuge tubes, 10 mL of 5 mM sulfuric acid solution was added, and after standing for 30 min, the sample was filtered and analyzed using IC. The results showed a recovery rate of 97.5% with a coefficient of variation (CV) of 1.84%. This indicates that TMA+ on the quartz fiber filter was not significantly lost after being subjected to a gas flow of 4 L/min for 6 h.
Effect of desorption solvent
The test aimed to determine which solvent would be more effective in desorbing TMA+ from the quartz fiber filters. The day before the analysis, 40 μg of 1 mg/mL TMA+ was added to each quartz fiber filter. Two desorption agents were tested, with a total of 12 samples (6 for each desorption agent). After allowing TMA+ to be fully absorbed, the next day, DI water and 5 mM sulfuric acid solution (mobile phase) were used as desorption solvents. The quartz fiber filters were placed in 15 mL plastic centrifuge tubes. Next, 10 mL of
Desorption condition
The effects of different sample pretreatment methods - stand, agitation, and ultrasonic agitation - on the recovery rate of TMA+ were evaluated. On the day before analysis, 40 μg of 1 mg/mL TMA+ was added to each quartz fiber filter. With three desorption conditions tested, a total of 18 samples were prepared. After allowing TMA+ to fully absorb overnight, the filters were transferred to 15 mL plastic centrifuge tubes the next day and 10 mL of 5 mM sulfuric acid solution was added. Desorption was performed by standing for 30 min, agitation for 30 min, and ultrasonic for 30 min. The samples were filtered and analyzed using IC. The results are shown in Table 2. The recovery rates for stand, agitation, and ultrasonic agitation for 30 min were 95.4%, 100.2%, and 100.0%, respectively, with a CV of 0.59%, 0.86%, and 1.17%, respectively. All three desorption methods achieved recovery rates of over 95%. Considering the effectiveness of desorption, ultrasonic treatment was selected as the preferred method.
Extraction recoveries of stand, agitation, and ultrasonic sample pretreatment methods and ultrasonic times (n = 6)
Extraction | Recovery (%) | Precision (%) |
Stand 30 min | 95.4 | 0.59 |
Agitation 30 min | 100.2 | 0.86 |
Ultrasonic 30 min | 100.0 | 1.17 |
Ultrasonic 5 min | 98.8 | 2.24 |
Ultrasonic 15 min | 99.6 | 1.56 |
Ultrasonic 30 min | 100.4 | 2.16 |
Effect of desorption time
Based on the previous test results, ultrasonic was identified as the optimal desorption method. However, how long does ultrasonic agitation need to effectively desorb TMA+ from the quartz fiber filter? In this study, three different durations of ultrasonic agitation were tested. A total of 18 samples were prepared by adding 40 μL of 1 mg/mL TMA+ to each quartz fiber filter and allowing them to absorb the TMA+ overnight. The next day, the filter papers were placed in 15 mL plastic centrifuge tubes, and 10 mL of 5 mM sulfuric acid solution was added. Ultrasonic was performed for 5, 15, and 30 min, respectively. The results are shown in Table 2. The recovery rates after ultrasonic agitation for 5, 15, and 30 min were 98.8%, 99.6%, and 100.4%, respectively, with CV values of 2.24%, 1.56%, and 2.16%, respectively. The best desorption effect was achieved with 30 min of ultrasonic.
Extraction recovery
Using the optimal pretreatment conditions determined from previous tests (10 mL elution with rinsing solution, followed by 30 min of ultrasonic agitation), the desorption efficiency of low, medium, and high concentrations was tested. Add 25, 50, and 100 μL of 10 mg/mL TMA+ to each quartz fiber filter one day before analysis, including one blank sample for each concentration, resulting in a total of 21 samples. The results are shown in Table 3. The average recovery rate was 116.6% with a CV of 2.25%, and the maximum difference in recovery rates among the concentrations was 1.55%. The sample preparation steps for UPLC-HRMS are the same as those for IC, except that the desorption solution is replaced with an aqueous solution. The average desorption efficiency for UPLC-HRMS is 90.6% and the CV value is 3.53%.
Extraction recoveries of the proposed method at low, medium and high concentration levels (n = 6)
Instrument | Concentration (μg/mL) | Extraction recovery (%) | Average recovery (%) | Precision (%) | Total precision (%) |
IC | 25 | 115.8 | 116.6 | 1.98 | 2.25 |
50 | 116.4 | 2.44 | |||
100 | 117.6 | 2.40 | |||
UPLC-HRMS | 25 | 90.0 | 90.6 | 3.76 | 3.53 |
50 | 91.5 | 4.46 | |||
100 | 90.4 | 2.56 |
Storage
The accuracy of measurements can be significantly affected by the storage environment of samples collected for TMAH aerosol capture. To assess whether the samples deteriorate during transportation or storage and whether there is any diffusion effect leading to sample loss, storage period tests were conducted under both room temperature and refrigeration conditions. Add 40 μL of 1 mg/mL TMA+ to each quartz fiber filter, with 7 samples needed for each storage condition (6 with added TMA+ and 1 blank sample) to be tested on days 1, 7, 14, 21, and 28, resulting in a total of 70 samples. The results are shown in Table 4. The recovery rates after 28 days of storage at room temperature (20 to 25 °C) and under refrigeration (< 4 °C) were comparable, with no significant difference. Therefore, samples collected for TMAH analysis can be stored at room temperature for at least 28 days.
The sample recoveries of storage in refrigeration and room temperature (n = 6)
Day | Refrigeration | Room temperature | ||
Recovery (%) | Precision (%) | Recovery (%) | Precision (%) | |
1 | 93 | 3.60 | 99.7 | 2.90 |
7 | 104.3 | 4.79 | 104.6 | 4.37 |
14 | 101.4 | 0.47 | 107.7 | 0.76 |
21 | 99.7 | 1.60 | 100.9 | 2.04 |
28 | 97.5 | 2.37 | 99.7 | 1.99 |
Air sampling
To verify the capability of the method developed in this study to effectively capture TMAH in the air, we employed a mist generator (JSQ-3310C) to simulate TMA+ aerosols present in workplace air. Sampling was conducted for 2 h using an impinger containing 10 mL of deionized water and quartz fiber filters, respectively, with a sampling pump (Gilian GilAir Plus) set at a flow rate of 1 L/min. Given the high water solubility of TMAH, the TMA+ signal intensity in deionized water was established as the reference (100%). The analysis of the quartz fiber filters yielded 137% of the reference value, indicating potential sources of error, such as the positioning of the sampler or losses in the absorbing liquid. Nonetheless, these results confirm that quartz filters can indeed effectively capture TMAH aerosols in the air.
Method validation
The linear range of quantification refers to the ability to obtain a measurement signal proportional to the concentration of TMA+ in the analysis of samples. Understanding the linear range of quantification of TMA+ can ensure the proper accuracy and precision of the measurement results. The calibration curve was constructed by plotting the peak areas against the concentrations of the TMA+ spiked in 5 mM H2SO4 (IC) or DIW (UPLC-HRMS). The limit of quantification (LOQ) is the lowest concentration on the calibration curve by the desorption volume, then by the conversion factor of TMA+ to TMAH (1.23), and dividing the result by the total sampling volume (sampling flow rate of 4 L/min multiplied by the sampling time of 8 h). The results are shown in Table 5. The linear range of TMA+ of IC is 0.3 to 100 μg/mL, with a correlation coefficient of R = 0.9998. The linear range of TMA+ of UPLC-HRMS is 0.002 to 1 μg/mL, with a correlation coefficient of R = 0.996. The collision energy was set at 35 for UPLC-HRMS analysis and the precursor ion m/z = 74.097 was used to obtain the TMA+ signals.
Linear range, correlation coefficient (r), LOQs of the proposed method
Analytical approach | Linear range (μg/mL) | r | LOQ (μg/m3) |
IC | 0.3~100 | 0.9998 | 1.92 |
UPLC-HRMS | 0.002~1 | 0.9960 | 0.01 |
HRMS verification
Utilizing the characteristics of HRMS, the compound analyzed was identified as TMA+ through mass spectrometry analysis [Figure 1]. The molecular weight of TMA+ is 74.097, and the mass spectrometer indeed detected signals corresponding to the molecular weight of 74.097.
Workplace environmental exposure assessment
To validate that the method we developed can be applied in the TMAH operational environment exposure assessment, we conducted operational environment sampling and analysis at a TMAH supply factory located in northern Taiwan. We carried out sampling in the TMAH Filling Area, Quality Control Laboratory, On-site Office, and Lounge. Sampling was conducted for 6.5 h using quartz fiber filters with a sampling pump set at a flow rate of 4 L/min. After pretreatment, the samples were analyzed using IC and UPLC- HRMS, with the results shown in Table 6. The highest air concentrations measured in the TMAH Filling Area were 3.29 and 4.75 μg/m³ from IC and UPLC-HRMS, respectively. The concentrations at the other sampling locations were below the quantitation limit of IC. However, due to the better quantitation limit of UPLC-HRMS, all samples could be measured. Because of the lower quantitation limit of UPLC-HRMS, the samples from the TMAH Filling Area needed to be diluted. This might be the reason for the discrepancy in measurements of the same sample using IC and UPLC-HRMS.
The results of workplace environmental exposure assessment of the TMAH factory
IC (μg/m3) | UPLC-HRMS (μg/m3) | |
TMAH filling area | 3.29 | 4.75 |
Quality control laboratory | < 2.48 | 0.07 |
On-site office | < 2.46 | 0.15 |
Lounge | < 2.40 | 0.05 |
CONCLUSION
This study developed a method for collecting TMAH in the air using a quartz fiber filter, with a sampling flow rate of 1-4 L/min, followed by elution with a mobile phase solution, 30 min of ultrasonic desorption, and subsequent analysis by IC or UPLC-HRMS for TMA+. Currently, Taiwan’s Ministry of Labor has not established a standard method for TMAH exposure assessment. This method was validated at the TMAH supply factory and can be applied to assess environmental exposure to TMAH, understand health risks, and prevent the occurrence of occupational diseases.
DECLARATIONS
Acknowledgments
The authors gratefully acknowledge the Accurate Detection Co., Ltd. and the Core Facility Center of National Cheng Kung University for their support in ion chromatography and high-resolution mass spectrometry analysis, respectively.
Authors’ contributions
Designed the study, and conducted the original draft writing and revising, data curation and analysis:
Assisted in sampling conditions and ion chromatography experiments: Huang HL
Assisted with high-resolution mass spectrometry experiments: Lin YC, Hsiao PZ
Responsible for revising the manuscript: Chang CW
Oversaw its supervision, administered the project, and revised the manuscript: Liao PC
Availability of data and materials
The data that support the findings of this study are available from the corresponding author upon reasonable request.
Financial support and sponsorship
This work was supported by Grants (E11200024002-006) from the Industrial Development Administration, Ministry of Economic Affairs of Taiwan.
Conflicts of interest
Liao PC is an Editorial Board member of Journal of Environmental Exposure Assessment, while the other authors have declared that there are no conflicts of interest.
Ethical approval and consent to participate
Not applicable.
Consent for publication
Not applicable.
Copyright
© The Author(s) 2024.
REFERENCES
1. Ackermann D, Holtz F, Reinwein H. Purification and constitutional determination of tetramine, a toxin from Actinia equina. Z Biol 1923;78:113. Available from: https://scholar.google.com/scholar_lookup?journal=Z+Biol&title=Reindarstellung+und+konstitutionsemitteelung+der+tetramins,+eines+giftes+aus+Aktina+equina&author=D+Ackermann&author=F+Holtz&author=H+Reinwein&volume=78&publication_year=1923&pages=113& [Last accessed on 18 Sep 2023]
2. Lin CC, Yang CC, Ger J, Deng JF, Hung DZ. Tetramethylammonium hydroxide poisoning. Clin Toxicol 2010;48:213-7.
3. The development overview of resource recycling for major waste in Taiwan’s IC manufacturing industry (Part 2). (in Chinese) Available from: https://www.materialsnet.com.tw/DocView.aspx?id=53664. [Last accessed on 19 Sep 2024].
4. Wu CL, Su SB, Chen JL, Chang CP, Guo HR. Tetramethylammonium ion causes respiratory failure related mortality in a rat model. Resuscitation 2012;83:119-24.
5. Liu CH, Huang CY, Huang CC. Occupational neurotoxic diseases in Taiwan. Saf Health Work 2012;3:257-67.
6. Lee CH, Wang CL, Lin HF, Chai CY, Hong MY, Ho CK. Toxicity of tetramethylammonium hydroxide: review of two fatal cases of dermal exposure and development of an animal model. Toxicol Ind Health 2011;27:497-503.
7. Wu CL, Su SB, Chen JL, Lin HJ, Guo HR. Mortality from dermal exposure to tetramethylammonium hydroxide. J Occup Health 2008;50:99-102.
8. Park SH, Park J, You KH, Shin HC, Kim HO. Tetramethylammonium hydroxide poisoning during a pallet cleaning demonstration. J Occup Health 2013;55:120-4.
9. Wang JJ, Shalyt E, Bai CN, Liang G, Macewan M, Parekh V. Advanced monitoring of TMAH solution. Solid State Phenom 2014;219:81-4.
10. Jie H, Kulkarni A, Kim HU, Kim T. In-line real-time conductivity technique for monitoring of liquid chemical concentration during semiconductor manufacturing. In: ICPT 2017; International Conference on Planarization/CMP Technology; 2017 Oct 11-13; Leuven, Belgium. VDE; 2017. pp. 1-4. Available from: https://ieeexplore.ieee.org/document/8238007. [Last accessed on 18 Sep 2024]
11. Urakami T, Araki H, Kobayashi H. Isolation and identification of tetramethylammonium-biodegrading bacteria. J Ferment Bioeng 1990;70:41-4.
12. Chang KF, Yang SY, You HS, Pan JR. Anaerobic treatment of tetra-methyl ammonium hydroxide (TMAH) containing wastewater. IEEE Trans Semicond Manufact 2008;21:486-91.
13. Koga T, Kashiwabara M, Hirakawa S, Ishibashi Y, Miyawaki T. Development of method determining tetramethylammonium hydroxide and tetramethylammonium salts in industrial wastewater by LC-MS/MS. J Jpn Soc Water Environ 2022;45:115-23. (in Japanese).
14. Wu CL, Su SB, Lien HY, Guo HR. The role of the chemical burns caused by hydroxide ion in the toxicity of dermal exposure to tetramethylammonium ion in a rat model. Burns 2012;38:1051-7.
Cite This Article

How to Cite
Download Citation
Export Citation File:
Type of Import
Tips on Downloading Citation
Citation Manager File Format
Type of Import
Direct Import: When the Direct Import option is selected (the default state), a dialogue box will give you the option to Save or Open the downloaded citation data. Choosing Open will either launch your citation manager or give you a choice of applications with which to use the metadata. The Save option saves the file locally for later use.
Indirect Import: When the Indirect Import option is selected, the metadata is displayed and may be copied and pasted as needed.
About This Article
Copyright
Data & Comments
Data
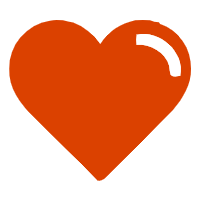
Comments
Comments must be written in English. Spam, offensive content, impersonation, and private information will not be permitted. If any comment is reported and identified as inappropriate content by OAE staff, the comment will be removed without notice. If you have any queries or need any help, please contact us at [email protected].