Novel and legacy brominated flame retardants in human breast milk and house dust from Denmark
Abstract
A suite of novel brominated flame retardants (NBFRs), hexabromocyclododecanes (HBCDDs), polybrominated diphenyl ethers (PBDEs) and dechlorane plus were measured in matched samples of house dust
Keywords
INTRODUCTION
Brominated flame retardants (BFRs) have been used in consumer products (e.g., home furnishings, electronics, and building materials) to reduce their flammability and slow down or prevent fires. Polybrominated diphenyl ethers (PBDEs) were among the first classes of additive flame retardants (FRs) used worldwide since the 1970s and typically applied in one of three technical mixtures: Penta-, Octa-, and Deca-BDE[1]. Due to evidence of persistence, bioaccumulation, long-range transport, and toxicity, the Penta- and Octa-BDE mixtures were banned in the EU and phased out in the USA from the mid-2000s[2], with their eventual global ban under the Stockholm Convention on Persistent Organic Pollutants (POPs) in 2009[3]. The use of DecaBDE in electronic applications has been restricted in the European Union (EU) since 2008, and DecaBDE was added to the Stockholm Convention in 2017[4]. In the early 2000s, hexabromocyclododecane (HBCDD) was the second most prominent BFR on the EU market. It was mainly used in polystyrene, but also as a replacement for PBDEs[5]. It was banned in the EU and worldwide in 2013 due to its POP- characteristics. The ban of PBDEs and HBCDD in consumer products led to an increased demand for alternatives, which include other brominated chemicals often referred to as novel brominated FRs (NBFRs) and the chlorinated flame retardant dechlorane plus (DP, also known as DDC-CO), which was recently added to the Stockholm Convention[6]. The use patterns of the different FR classes has varied between regions with a notable preference for PentaBDE in North America, HBCDD in Europe, TBBPA in Asia, and DecaBDE in all three regions[7]. However, there have also been temporal changes in consumption of the commercial mixtures of PBDEs, and later export of waste from more to less industrialized countries has altered the emission patterns[8]. Similar information on use patterns has not been identified for NBFRs.
Due to their persistence and the long lifetime of many consumer products, PBDEs and HBCDDs are still detected in the environment, including wildlife and abiotic environmental media such as air, soil, and indoor dust[9-11]. At the same time, NBFRs have also been widely detected in environmental samples, consumer products, and food[12,13]. In humans, PBDEs have been measured all over the world in a variety of matrices, including breast milk, blood, placenta, and adipose tissue, while reports on HBCDDs are less frequent and only few measurements exist on NBFRs in human matrices[14-18]. NBFRs have also been detected in upholstered furniture foam and house dust, and a urinary metabolite of 2-ethylhexyl 2,3,4,5-tetrabromoethylhexylbenzoate (EH-TBB) has been frequently found in both adults and children[19-22]. However, breast milk measurements of NBFRs have been rare and have only been conducted in the UK, USA, and China to date[23-26]. Overall, both legacy and current-use BFRs are omnipresent in our environment, suggesting chronic exposure and consequently concern about potential adverse health impacts.
PBDE congeners have been associated with a variety of negative health outcomes such as thyroid hormone disruption and neurodevelopmental deficits in both human and animal studies[27-30]. In particular, prenatal and early-life PBDE exposure has been linked to various adverse effects on neurobehavioral development in young children, such as poorer attention, fine motor coordination, and cognition as well as lower IQ[28,31,32]. Much less is known about the NBFRs[13] and their potential toxicity, but for several of the better-studied NBFRs, neurotoxic potential has been shown, possibly linked to endocrine disruption[33]. Specifically, components of the Firemaster® 550 mixture, which includes both brominated and organophosphate compounds, have been associated with metabolic and endocrine disruption and activation of nuclear receptors regulating adipogenic pathways in in vitro studies[34-36]. Given the long-term effects of PBDE exposure and the growing prominence of NBFRs in consumer product use and environmental presence, there is a need to elucidate dominant pathways of exposure for these brominated compounds. This is of particular concern since children tend to have higher levels of exposure to chemicals including PBDEs, compared to adults[37].
Here, we report concentrations of PBDEs, HBCDDs, as well as NBFRs and dechlorane plus in breast milk collected from women in the Copenhagen area in 2007-2008. This was a critical point in BFR history with the phase-out and global ban of PBDEs in 2009 changing the use pattern of BFRs. Further, the breast milk concentrations were compared to house dust concentrations of HBCDDs and NBFRs collected at the same time as well as and to previously published paired PBDE concentrations in placenta tissue as well as maternal and cord blood. Exposure estimates for breast milk and dust were investigated and compared for the infants. These data present a retrospective assessment of breast milk concentrations in Denmark immediately following the international PBDE ban and prior to the HBCDD-ban, thus providing a benchmark for more recent and future measurements of BFR concentrations in breast milk.
EXPERIMENTAL
Study population and sample collection
Study participants were recruited among healthy, pregnant women scheduled for caesarean section at Copenhagen University Hospital from March to December 2007; further details are given elsewhere[15,38]. Caesarean section might have subtle influences on nutrient composition in breast milk[39], but potential influences on contaminant levels are unknown. In combination with a relatively high rate (approximately 20%) of caesarean sections in Denmark[40], the samples are likely to represent typical Danish FR levels at the time. The women were asked to sample breast milk once a week for 3 months after giving birth using a manual breast milk pump supplied by the study; after sampling, the milk was transferred to a pre-clean amber glass. The weekly samples were combined in a composite sample stored in the participants' household freezer. Each participant recorded the dates of sampling. Three months after birth, both milk samples and the participants’ vacuum cleaner bags were collected. The content of the bags was sieved to
Chemical analysis
The samples were first analyzed for PBDEs and then later for NBFRs, DPs, and HBCDDs; due to a limited sample volume, a few samples were only analyzed for PBDEs; an overview of the different analyses is given in Supplementary Figure 1. For the analysis of PBDEs in breast milk, 20 mL of breast milk was dried with diatomaceous earth, spiked with 13C-labelled BDE-209 and octachlorobiphenyl (PCB-198) and extracted using Soxhlet with hexane:acetone (4:1). The milk extracts were cleaned on a multilayer column including sulfuric acid-impregnated silica eluted with 250 mL hexane as described in Vorkamp et al.[44]. The cleaned extract was reduced in volume by rotary evaporator, and the final volume was approximated to 0.5 mL and analyzed by gas chromatography with electron capture negative ionization-mass spectrometry
The chemical analyses of HBCDDs and NBFRs included α-, β-, and γ-HBCDD, EH-TBB, 2,3-dibromopropyl 2,4,6-tribromophenyl ether (DPTE, also known as TBP-DBPE), bis(2-ethylhexyl) tetrabromophthalate (BEH-TEBP), decabromodiphenyl ethane (DBDPE), as well as syn- and anti-DP. Their analysis in breast milk and dust followed the method described in Vorkamp et al., with some modifications[45]. In brief, sub-samples of approximately 0.5 g of house dust or 20 mL breast milk (after heating to 37 °C) were dried with diatomaceous earth, spiked with isomer-specific internal standards of 13C-HBCDD as well as 13C-BEH-TEBP (where relevant) and extracted by Pressurized Liquid Extraction (dust) or Soxhlet (milk) using hexane:dichloromethane (1:1). Dust samples were cleaned up on a column consisting of 2 g activated aluminum oxide, 2 g deactivated silica and some Na2SO4 and eluted with 60 mL hexane:dichloromethane (1:1). For the milk extracts, the column clean-up was the same described in
Each analytical batch included QA/QC measures such as procedural blanks, internal or standard reference material (NIST SRM 2585), as described elsewhere[15,41]. For PBDEs in breast milk, a sample of human breast milk from the ring test “Dioxins in Food, 2006” was used (with consensus values for BDE-28, 47, 99, 100, 153, 154, 209)[47]. The obtained results were within ±20% of the consensus values for all congeners except for BDE-209 in one batch, which was 37% above the consensus value, and BDE-154, which was generally overestimated due to the co-elution with BB-153 described above. NIST SRM 2582 was also used for quality control of HBCCDs and NBFRs, based on indicative values published in the literature[22,48]. For the NBFR analyses, PCB-198 [and, in some batches, 13C-polychlorinated naphthalene (PCN)-27] were added for recovery determination prior to extraction. The recovery rate for dust was 91.5% on average, with a range of 68%-116%. The recovery standards were difficult to integrate in the milk samples, leading to an average of 85% (range 52%-120%). However, the low numbers are likely caused by chromatographic challenges due to matrix effects, rather than indicating losses in the process.
Statistical analyses
Analyses were conducted using SAS statistical software (version 9.4; SAS Institute Inc., Cary, NC, USA). Based on examination of distributions of all PBDEs and NBFRs in breast milk and the biological matrices previously analyzed (placenta, fetal and maternal blood), the majority of which were right-skewed, non-parametric statistics or log10-transformed concentrations were used for all analyses. Statistical analyses were conducted only for chemicals with detection frequencies > 65% overall. For any concentrations below the method detection limit (MDL), values were imputed as MDL/2 and normalized in a matrix-specific manner (i.e., breast milk, serum, and placenta by lipid concentration and dust by dry mass).
We evaluated associations between breast milk and dust for NBFRs and between breast milk and dust, placenta, fetal blood, and maternal blood for PBDEs using Spearman correlations. Relationships among NBFRs and PBDEs, separately, were assessed within breast milk samples, also with Spearman correlations. We examined additional relationships between log10-transformed concentrations and various predicting factors using regression analyses. These factors included mother’s age, pre- and term-pregnancy body mass index, and pregnancy weight gain (evaluated as continuous variables) and parity and previous breastfeeding (evaluated as categories, dichotomized based on median reported value). For evaluating associations with breast milk PBDE concentrations, maternal serum and house dust concentrations were split into tertile categories. Beta coefficients from all of these models were exponentiated and used as an estimate of multiplicative change in concentrations for each unit of increase for all continuous variables or relative to the reference category for any categorical variables.
Exposure estimate calculations
Estimates of daily intake were calculated based on breast milk and house dust concentrations of NBFRs and PBDEs with > 65% detection in a matrix. These estimates were calculated for a 3-month-old infant, given that the house dust concentrations were collected in homes at 3 months post-delivery. Estimates are presented in units of ng/day for comparison of exposure pathways and were not normalized to body mass. The US Environmental Protection Agency Exposure Factors Handbook was used for recommended estimated daily consumption of breast milk and for unintentionally ingested house dust for 3-month-old infants. For human milk intake, the weighted mean intake value for exclusively breastfed infants was
Furthermore, intake estimates of PBDEs from air were calculated based on air measurements in the same homes days prior to birth as previously presented in Vorkamp et al.[41]. A long-term exposure value for inhalation of 3.5 m3/d was estimated for infants 1-3 months of age[51]. The equation for intake by inhalation was then:
RESULTS & DISCUSSION
Study population
A total of 51 women were recruited for the overall study. Of these, four women did not participate in the follow-up, and another seven did not wish to or were not able to deliver a breast milk sample. This resulted in a total of 40 breast milk samples and 47 dust samples collected 3 months after birth; details about the overall study population have been described previously[15,41,42]. The mean age of mothers of the subset of participants providing breast milk samples was 32.7 years (range: 24-43 years) [Supplementary Table 2]. The average number of previous children was one (range: 0-3 children) and mothers had previously breastfed for about a year prior to starting the sample collection (mean: 11 months; range: 0-30 months). Further details on descriptors are given in Supplementary Table 2.
Breast milk
Levels
BFRs have only previously been reported in breast milk in Denmark in two studies collecting samples between 1997 and 2002, which included PBDEs in milk and placenta samples[52] and PBDEs and HBCDDs in milk[53]; NBFRs had not previously been measured in breast milk from Denmark. In the current study, we measured 19 PBDE congeners, 3 HBCDD diastereomers, 5 NBFRs, and 2 DP isomers. Of these chemicals, 8 PBDEs, 2 HBCDD diastereomers, and 4 NBFRs were detected in over 65% of the breast milk samples
Descriptive statistics of PBDEs in milk and dust
Breast milk (n = 40) ng/g lipid | House dust (n = 47) ng/g dry weight | ||||||||
Chemical | % Detect | Mean | Median | Range | % Detect | Mean | Median | Range | |
BDE-17 | 85 | 0.035 | 0.024 | < 0.014-0.13 | 60 | 0.36 | 0.22 | < 0.022-3.09 | |
BDE-28 | 85 | 0.076 | 0.047 | < 0.036-0.43 | 83 | 0.66 | 0.35 | < 0.022-6.63 | |
BDE-49 | 43 | 0.039 | n.a. | < 0.012-0.13 | 79 | 1.18 | 0.67 | < 0.022-8.09 | |
BDE-71 | 0 | n.a. | n.a. | n.a. | 0 | n.a. | n.a. | n.a. | |
BDE-47 | 100 | 1.20 | 0.61 | 0.19-9.92 | 100 | 35.4 | 13.7 | 1.12-316 | |
BDE-66 | 38 | 0.021 | n.a. | < 0.008-0.042 | 96 | 1.34 | 0.75 | < 0.082-6.17 | |
BDE-77 | 0 | n.a. | n.a. | n.a. | 0 | n.a. | n.a. | n.a. | |
BDE-100 | 95 | 0.45 | 0.23 | < 0.10-8.14 | 100 | 8.56 | 3.53 | 0.270-60.0 | |
BDE-99 | 100 | 0.28 | 0.18 | 0.065-1.48 | 100 | 44.7 | 19.2 | 1.27-325 | |
BDE-85 | 18 | 0.031 | n.a. | < 0.013-0.10 | 89 | 1.80 | 0.78 | < 0.024-13.5 | |
BDE-154$ | 98 | 0.21 | 0.15 | < 0.095-0.81 | 96 | 3.85 | 1.83 | < 0.029-26.5 | |
BDE-153 | 100 | 1.55 | 0.79 | 0.44-25.3 | 98 | 5.98 | 2.71 | < 0.057-45.7 | |
BDE-183 | 30 | 0.054 | n.a. | < 0.023-0.43 | 100 | 7.19 | 4.14 | 0.36-31.2 | |
BDE-197 | 58 | 0.098 | 0.046 | < 0.051-0.28 | 100 | 3.56 | 2.14 | 0.27-15.3 | |
BDE-203 | 3 | n.a. | n.a. | n.a. | 100 | 2.38 | 1.84 | 0.30-13.5 | |
BDE-208 | 35 | 0.058 | n.a. | < 0.022-0.47 | 87 | 8.12 | 2.92 | < 0.55-175 | |
BDE-207 | 43 | 0.13 | na.a | < 0.045-1.21 | 94 | 22.8 | 5.47 | < 0.95-628 | |
BDE-206 | 3 | n.a. | n.a. | n.a. | 96 | 65.2 | 12.0 | < 1.95-2,220 | |
BDE-209 | 95 | 2.70 | 0.53 | < 0.061-49.8 | 100 | 2641 | 432 | 54.5-79,800 | |
∑PBDE6* | 100 | 3.73 | 2.21 | 0.98-45.2 | 100 | 105 | 53.6 | 4.64-672 |
Descriptive statistics of NBFRs, HBCDD and Dechlorane Plus in milk and dust
Breast milk (n = 37) ng/g lipid | House dust (n = 43a) ng/g dry weight | ||||||||
Chemical | % Detect | Mean | Median | Range | % Detect | Mean | Median | Range | |
DPTE | 91 | 0.13 | 0.11 | < 0.028-0.34 | 93 | 1.36 | 0.99 | < 0.21-5.76 | |
EH-TBB | 43 | 0.12 | n.a. | < 0.018-0.60 | 100 | 12.0 | 6.26 | 1.75-89.1 | |
BTBPE | 100 | 0.087 | 0.064 | 0.026-0.28 | 100 | 31.7 | 7.23 | 2.15-293 | |
BEH-TEBP | 100b | 0.92 | 0.78 | 0.53-1.50 | 100 | 39.1 | 26.7 | 1.79-200 | |
DBDPE | 19 | 0.21 | n.a. | < 0.088-2.06 | 100 | 141 | 86.2 | 10.4-801 | |
Syn-DP | 49 | 0.062 | n.a. | < 0.008-0.44 | 98 | 9.77 | 1.57 | < 0.21-332 | |
Anti-DP | 84 | 0.13 | 0.073 | < 0.022-1.85 | 100 | 8.23 | 4.54 | 0.55-54.3 | |
α - HBCDD | 100 | 0.53 | 0.24 | 0.11-5.68 | 100 | 140 | 92.1 | 11.4-548 | |
β - HBCDD | 6 | 0.051 | n.a. | n.a. | 100 | 52.4 | 32.1 | 5.52-293 | |
γ - HBCDD | 65 | 0.10 | 0.051 | < 0.020-1.13 | 100 | 431 | 177 | 18.8-3,310 |
Of the HBCDD diastereomers, α-HBCDD was detected in all samples and was the most abundant diastereomer (median = 0.24 ng/g lipid), with γ detected in 65% and β detected in only 6% of samples [Table 2]. α-HBCDD was also the most abundant non-PBDE flame retardant that was measured in the breast milk samples if disregarding BEH-TEBP that was only analyzed in five samples. α-HBCDD has also previously been the dominant HBCDD diastereomer in diastereomer-specific analyses of breast milk[58,59]. Compared to a previous Danish study conducted between 1997 and 2002 (n = 435), our study observed slightly lower concentrations of α-HBCDD[53]. Although it is difficult to determine trends in a region from just two time points, data suggest that HBCDD concentrations could have decreased in Denmark in the 2000s, which is in line with Swedish studies indicating decreasing concentrations in the same time period[37]. Other studies examining breast milk samples in Europe from the mid to late 2000s detected similar albeit slightly higher concentrations, including a cohort of Norwegian women[60] and studies conducted in Ireland[61], as well as the recent WHO/UNEP overview[57]. However, other studies conducted in the UK showed substantially higher concentrations of HBCDDs[62,63], whereas HBCDDs were not detected in a study on breast milk from Sweden, possibly related to a GC-MS method, which is less sensitive than the commonly applied LC-MS/MS method[56,64].
Detected in nearly all samples (DF = 91%), the most abundant NBFR in our study was DPTE
Although BEH-TEBP was only measured in a subset of five samples due to degradation in the standard clean-up procedure, the concentrations were among the highest of any BFR measured in the breast milk samples (median = 0.78 ng/g lipid). High levels despite moderate detection frequency were also observed for milk samples collected in Norway, Slovakia, and the Netherlands around the same time[71], which collectively calls for inclusion of this compound in future studies, despite the analytical challenges, as it could be an important pathway of exposure for infants.
The levels of syn- and anti-DP detected here were slightly lower than previously observed across Europe[71] but higher than in Canada[72]. However, the literature on NBFRs in human milk is still very limited and thus limits the comparison of our results to others; a recent review of contaminants in human milk by Chi et al. included several groups of flame retardants but not any of the NBFRs investigated here[73].
Intramatrix correlations
In general, PBDEs, particularly the lower molecular weight congeners and congeners of the commercial PentaBDE mixture, were highly correlated in breast milk [Table 3]. Likewise, the two diastereomers of HBCDD (α and γ) were also highly correlated (rs = 0.64, P < 0.0001). DPTE in breast milk was positively and significantly correlated with BTBPE and anti-DP, but not with the HBCDD diastereomers. Anti-DP was further moderately correlated with the two HBCDD diastereomers, whereas BTBPE was correlated with BDE-28. α-HBCDD was also positively correlated with BDE-28, -47, -99, and -100 (rs = 0.34-0.47, P < 0.05, Table 3). Given their concurrent use as flame retardants, this indicates that HBCDDs and PBDEs could have similar routes of exposure or sources.
Correlation table within milk for flame retardants detected in > 65% of samples (PBDEs: n = 40, NBFRs: n = 37)
BDE-17 | BDE-28 | BDE-47 | BDE-99 | BDE-100 | BDE-153 | BDE-154 | BDE-209 | DPTE | BTBPE | Anti-DP | α - HBCDD | γ - HBCDD | |
BDE-17 | 1 | ||||||||||||
BDE-28 | 0.15 | 1 | |||||||||||
BDE-47 | 0.06 | 0.84*** | 1 | ||||||||||
BDE-99 | 0.34* | 0.69*** | 0.82*** | 1 | |||||||||
BDE-100 | 0.03 | 0.73*** | 0.85*** | 0.63*** | 1 | ||||||||
BDE-153 | 0.27 | 0.17 | 0.18 | 0.17 | 0.35* | 1 | |||||||
BDE-154 | 0.30 | 0.24 | 0.18 | 0.24 | 0.11 | 0.33* | 1 | ||||||
BDE-209 | 0.29 | -0.16 | -0.23 | -0.11 | -0.13 | -0.13 | -0.06 | 1 | |||||
DPTE | 0.07 | 0.19 | 0.08 | 0.14 | 0.14 | 0.00 | -0.03 | 0.00 | 1 | ||||
BTBPE | 0.10 | 0.38* | 0.25 | 0.31 | 0.25 | 0.11 | 0.24 | -0.02 | 0.55*** | 1 | |||
Anti-DP | -0.04 | 0.17 | 0.23 | 0.17 | 0.04 | -0.31 | 0.13 | 0.22 | 0.44** | 0.13 | 1 | ||
α - HBCDD | 0.12 | 0.45** | 0.43** | 0.34* | 0.47** | -0.02 | 0.20 | 0.00 | 0.23 | 0.20 | 0.38* | 1 | |
γ - HBCDD | 0.14 | 0.18 | 0.14 | 0.23 | 0.08 | -0.12 | -0.01 | 0.07 | 0.23 | 0.06 | 0.35* | 0.64*** | 1 |
Relationship with age
Several of the BFR compounds in breast milk were positively associated with the mother’s age. Two NBFRs (BTBPE and anti-DP), α-HBCDD, and BDE-154 concentrations increased by almost 10% per one-year change in the mother’s age [Figure 1]; however, for BDE-154 it is worth noting that it co-elutes with BB-153, which is very persistent and its use dates back further than the PBDEs.
Dust
Among the PBDEs, the dust samples were dominated by BDE-209 (median: 432 ng/g), followed by BDE-99 (median: 19.2 ng/g) and BDE-47 (median: 13.7 ng/g) [Table 1], as previously observed in other European studies[16]. The median level of the ΣHBCDDs was comparable to BDE-209, though the maximum values were not as pronounced for ΣHBCDDs. DBDPE was also found in high levels though not as high as BDE-209, which at the time of sampling was still in use in some applications. Substantial amounts of EH-TBB, BTBPE, and BEH-TEBP were also detected. Although median levels in the literature span many orders of magnitude[13], the medians and ranges in the current study were very similar to levels reported by
Intermatrix correlations of breast milk
Dust
Of the BFRs analyzed in both breast milk and house dust, three of the seven PBDEs in paired samples were significantly correlated (rs = 0.35-0.42, P < 0.05; Table 4), i.e., BDE-28, -47, -99. They are all lower molecular weight PBDEs and prominent congeners of the Penta-BDE commercial mix. This suggests that house dust could be a recent source of exposure together with toxicokinetic processes, which may favor the transfer to and accumulation of lower brominated molecules in milk, despite higher exposure to BDE-209[75]. Positive and significant correlations between milk and house dust have been observed previously for PBDE congeners, although the strength of correlation varied by congener in different geographical regions. Significant associations between breast milk and house dust have been observed in Sweden for
Spearman correlation (rs) between milk vs. dust, placenta, fetal plasma, and maternal plasma for flame retardants detected in > 65% of samples
Breast milk | |||||||||
House dust (n = 40) | PBDEs | BDE-17 | BDE-28 | BDE-47 | BDE-99 | BDE-100 | BDE-153 | BDE-154 | BDE-209 |
BDE-28 | 0.35* | 0.39* | 0.36* | ||||||
BDE-47 | 0.39* | 0.37* | |||||||
BDE-99 | 0.39* | 0.42* | |||||||
BDE-100 | 0.37* | 0.39* | 0.19 | ||||||
BDE-153 | 0.37* | 0.37* | 0.01 | ||||||
BDE-154 | 0.38* | 0.42* | 0.19 | ||||||
BDE-209 | -0.12 | ||||||||
Placenta (n = 39) | BDE-47 | 0.56** | 0.53** | 0.58** | |||||
BDE-99 | 0.37* | 0.32* | 0.09 | 0.36* | |||||
BDE-100 | 0.42* | 0.45* | 0.38* | 0.58** | |||||
BDE-153 | 0.42* | 0.73*** | |||||||
BDE-154 | 0.59*** | ||||||||
BDE-209 | 0.12 | ||||||||
Fetal blood (n = 31) | BDE-28 | 0.83*** | 0.77*** | 0.58*** | 0.67*** | ||||
BDE-153 | 0.64*** | 0.37* | |||||||
Maternal blood (n = 39) | BDE-28 | 0.81*** | 0.74*** | 0.55** | 0.62*** | ||||
BDE-47 | 0.65** | 0.76*** | 0.57** | 0.67*** | |||||
BDE-153 | 0.73*** | ||||||||
BDE-209# | -0.59* | 0.39 |
In general, breast milk concentrations of NBFRs were not significantly correlated with house dust [Supplementary Table 3], although the associations were generally positive with the exception of DPTE being notably negatively correlated (rs = -0.32, P = 0.07). This is unlike PBDE exposure, which has historically been linked fairly strongly to house dust[79]. It may suggest other exposure pathways than dust; however, the transformation processes of NBFRs are not well-understood and might differ from those of the relatively persistent PBDEs. Only one other study was identified addressing correlations between HBCDDs in milk and dust, but the correlation analysis was not possible due to the low detection frequency in breast milk[56].
Maternal serum
Concentrations of PBDEs in maternal serum of the same individuals were previously described and reported in Frederiksen et al.[42]. In brief, five PBDEs were detected in the majority of the samples, and BDE-209 was the most abundant, although it was only measured in 18 samples total, with BDE-153 being the second most abundant PBDE in serum [Supplementary Table 1]. PBDEs in breast milk and maternal serum were significantly and positively correlated for the three PBDE congeners with full sample overlap (i.e. DF > 65%) between milk and serum (rs = 0.73-0.81, P < 0.0001; Table 4), but not for BDE-209. When relationships were adjusted for parity and mother’s age, these relationships were still clearly observed, with mothers who had the highest tertile concentration of BDE in their blood having between 2 to 4 times as high concentrations in breast milk, depending on the congener [Figure 2].
Cord blood
Concentrations of PBDEs in cord blood were previously reported in Frederiksen et al.[42]. Only BDE-28 and -153 were detected in > 65% (cut-off set for statistical analyses) of both the cord blood and breast milk samples. Both congeners were highly correlated between cord blood and breast milk (rs = 0.83 and 0.64,
Placenta
PBDE concentrations in placenta were previously described in Frederiksen et al., with further investigations comparing placenta and dust concentrations in Vorkamp et al.[15,41]. Positive and significant correlations were observed for 4 of the 6 PBDEs detected in both placenta and breast milk (BDE-47, -100, -153, and -154 (rs = 0.53-0.73; P < 0.0001)), and notably, no association was observed for BDE-99 and -209.
Exposure estimates
Exposure estimates for 3-month-old infants in 2007 were calculated based on breast milk and house dust measurements for NBFRs, HBCDDs, DPs, and PBDEs; for PBDEs, intake via air was also calculated. For the NBFRs and PBDEs, with the exception of BDE-209, breast milk contributed substantially more to the daily exposure than house dust [Table 5]. This was in agreement with Toms et al., who reported an intake of BDE-47 from breast milk for Australian infants very similar to our calculations (10-440 ng/day) for samples taken the same year[78]. For BDE-209 and α-HBCDD, median exposure estimates were more similar for dust and breast milk, although slightly higher in breast milk, while the opposite trend was observed for γ-HBCDD. Exposure through inhalation only contributed marginally to the total exposure of PBDEs but was comparable to the exposure via dust for PBDEs with up to four bromine atoms [Table 5]. These data suggest that in addition to in utero exposures, exposure through breast milk plays a dominant role in contributing to the cumulated infant exposure to BFRs compared to house dust (and air), and that this trend also includes the NBFRs. Although these samples were collected in the late 2000s, they provide a valuable point of reference and insight for exploring exposure pathways and prioritizing preventive initiatives, particularly for NBFRs, for which much is still unknown. PBDE and HBCDD levels are expected to continue to decline in the future due to global restrictions, as was recently demonstrated for breast milk BDE-47 and BDE-99 concentrations[80], whereas the expected trend for NBFR is less clear as these chemicals are not yet restricted.
Exposure estimates for 3-month-old infant (ng/day), reported if > 65% detection
Breast milk | House dust | Air* | |||||||
Chemical | Mean | Median | Range | Mean | Median | Range | Mean | Median | Range |
DPTE | 2.71 | 2.55 | 1.18-14.8 | 0.03 | 0.02 | < 0.21-5.76 | n.a. | ||
EH-TBB | 0.24 | 0.13 | 1.75-89.1 | ||||||
BTBPE | 1.90 | 1.34 | 0.85-5.49 | 0.63 | 0.15 | 2.15-293 | |||
BEH-TEBP | 24.4 | 21.5 | 20.3-50.0 | 0.78 | 0.53 | 1.79-200 | |||
DBDPE | 2.82 | 1.72 | 10.41-801 | ||||||
Syn-DP | 0.20 | 0.03 | < 0.21-332 | ||||||
Anti-DP | 3.28 | 1.14 | < 0.17-60.5 | 0.17 | 0.09 | 0.55-54.3 | |||
α - HBCD | 15.2 | 4.67 | 2.69-248 | 2.80 | 1.84 | 11.35-548 | |||
β - HBCD | 1.05 | 0.64 | 5.52-293 | ||||||
γ - HBCD | 2.74 | 0.95 | < 0.429-37.0 | 8.62 | 3.54 | 18.82-3,310 | |||
BDE-17 | 0.59 | 0.52 | < 0.19-1.70 | 0.01 | 0.004 | < 0.001-0.06 | 0.05 | 0.04 | < LOQ-0.09 |
BDE-28 | 1.77 | 1.14 | < 0.19-18.1 | 0.02 | 0.01 | < 0.001-0.13 | 0.05 | 0.04 | 0.01-0.16 |
BDE-49 | 0.03 | 0.01 | < 0.001-0.16 | 0.03 | 0.02 | 0.01-0.12 | |||
BDE-47 | 28.9 | 15.0 | 1.65-419 | 0.67 | 0.26 | 0.02-6.32 | 0.67 | 0.48 | 0.17-2.61 |
BDE-66 | 0.03 | 0.01 | < 0.001-0.12 | 0.02 | 0.01 | < LOQ-0.05 | |||
BDE-100 | 13.6 | 4.73 | < 0.48-344 | 0.16 | 0.07 | 0.01-1.20 | 0.05 | 0.05 | 0.01-0.13 |
BDE-99 | 6.08 | 4.01 | 1.03-62.7 | 0.86 | 0.42 | 0.03-6.50 | 0.29 | 0.23 | 0.06-1.3 |
BDE-85 | 0.04 | 0.02 | < 0.001-0.27 | ||||||
BDE-154$ | 4.40 | 3.23 | < 0.81-14.4 | 0.07 | 0.03 | < 0.001-0.53 | |||
BDE-153 | 44.8 | 17.1 | 6.56-1,070 | 0.11 | 0.05 | < 0.001-0.91 | |||
BDE-183 | 0.08 | 0.08 | 0.01-0.62 | ||||||
BDE-197 | 0.07 | 0.04 | 0.01-0.31 | ||||||
BDE-203 | 0.05 | 0.04 | 0.01-0.27 | ||||||
BDE-208 | 0.17 | 0.06 | < 0.01-3.50 | ||||||
BDE-207 | 0.48 | 0.11 | < 0.01-12.6 | ||||||
BDE-206 | 1.36 | 0.24 | < 0.04-44.4 | ||||||
BDE-209 | 70.9 | 10.2 | < 0.48-1,190 | 49.4 | 8.52 | 0.37-1,596 | 0.81 | 0.42 | < LOQ-6.41 |
The final exposure pathway, dermal uptake, was not assessed directly in the current study as several of the required input data such as dust loadings and skin lipid thickness were not available for infants, making the estimates highly uncertain. For infant skin in particular, the stratum corneum is thinner than adult skin[81] so the permeability coefficients determined on adult skin may not apply. However, estimates of skin permeability of BFRs have shown that dermal uptake of NBFRs does occur, though much is initially trapped in the epidermis[82]. The highest dermal uptake is expected for the BFR with lower log Kow such as DPTE, HBCDD, and the least brominated PBDEs, and it cannot be ruled out that dermal uptake may be a relevant exposure pathway.
CONCLUSION
Despite attention to BFRs over the last few decades, NBFRs have only scarcely been studied with regard to human external and internal exposure. This study shows that the internal exposure levels of NBFRs were similar to those of most PBDEs already at the time of PBDE restrictions. As opposed to PBDEs, NBFRs were not correlated in breast milk and house dust, indicating other prominent exposure pathways than dust ingestion and/or transformation and toxicokinetic processes which are not yet understood. These data add to the knowledge of exposure pathways and estimated total intake for infants that may be a valuable reference point for future BFR studies and assessment of current and future restrictions.
DECLARATIONS
Acknowledgments
The authors would like to thank all study participants for volunteering, Marie Pedersen for the initial recruitment, and Birgit Groth for skillful analyses of NBFRs, DPs, and HBCDDs.
Authors’ contributions
Writing - original draft: Hammel SC, Frederiksen M
Conceptualization: Vorkamp K, Nielsen JB, Sørensen LS, Knudsen LE, Frederiksen M
Writing - review & editing: Vorkamp K, Nielsen JB, Sørensen LS, Knudsen LE
Supervision: Vorkamp K, Knudsen LE
Funding acquisition: Vorkamp K, Nielsen JB, Sørensen LS, Knudsen LE, Frederiksen M
Methodology, resources: Vorkamp K, Knudsen LE, Frederiksen M
Data curation, project administration: Vorkamp K, Frederiksen M
Visualization, formal analysis: Hammel SC
Investigation: Frederiksen M
Availability of data and materials
Fully anonymized data can be shared upon justified request.
Financial Support and sponsorship
The study was supported by the Danish Agency for Science, Technology and Innovation (271-06-0355) and Danish Council for Independent Research (DFF - 1333-00034), the data analyses and publication were further supported by FFIKA (Focused Research Effort on Chemicals in the Working Environment) from the Danish Government.
Conflict of Interest
All authors declare that there are no conflicts of interest.
Ethical approval and consent to participate
The study was approved by the Regional Ethics Committee of the Capital Region of Denmark (H-KF-327603) and the Danish Data Protection Agency. All participants provided written consent to participate.
Consent for publication
Not applicable.
Copyright
© The Author(s) 2024.
Supplementary Materials
REFERENCES
1. Alaee M, Arias P, Sjödin A, Bergman A. An overview of commercially used brominated flame retardants, their applications, their use patterns in different countries/regions and possible modes of release. Environ Int 2003;29:683-9.
3. UNEP. Report of the Conference of the Parties of the Stockholm Convention on Persistent Organic Pollutants on the work of its fourth meeting. 2009. Available from: http://chm.pops.int/portals/0/repository/cop4/unep-pops-cop.4-38.english.pdf [Last accessed on 21 Feb 2024].
4. UNEP. Report of the Conference of the Parties of the Stockholm Convention on Persistent Organic Pollutants on the work of its ninth meeting. 2017. Available from: https://chm.pops.int/TheConvention/ConferenceoftheParties/Meetings/COP9/tabid/7521/Default.aspx [Last accessed on 21 Feb 2024].
5. Covaci A, Gerecke AC, Law RJ, et al. Hexabromocyclododecanes (HBCDs) in the environment and humans: a review. Environ Sci Technol 2006;40:3679-88.
6. UNEP. The new POPs under the Stockholm Convention. 2023. Available from: https://chm.pops.int/TheConvention/ThePOPs/TheNewPOPs/tabid/2511/Default.aspx [Last accessed on 21 Feb 2024].
7. Law RJ, Allchin CR, de Boer J, et al. Levels and trends of brominated flame retardants in the European environment. Chemosphere 2006;64:187-208.
8. Abbasi G, Li L, Breivik K. Global historical stocks and emissions of PBDEs. Environ Sci Technol 2019;53:6330-40.
9. Akortia E, Okonkwo JO, Lupankwa M, et al. A review of sources, levels, and toxicity of polybrominated diphenyl ethers (PBDEs) and their transformation and transport in various environmental compartments. Environ Rev 2016;24:253-73.
10. Zhu L, Hajeb P, Fauser P, Vorkamp K. Endocrine disrupting chemicals in indoor dust: a review of temporal and spatial trends, and human exposure. Sci Total Environ 2023;874:162374.
11. Zhu L, Fauser P, Mikkelsen L, Sanderson H, Vorkamp K. Suspect and non-target screening of semi-volatile emerging contaminants in indoor dust from Danish kindergartens. Chemosphere 2023;345:140451.
12. Vorkamp K, Balmer J, Hung H, Letcher RJ, Rigét FF, de Wit CA. Current-use halogenated and organophosphorous flame retardants: a review of their presence in Arctic ecosystems. Emerg Contam 2019;5:179-200.
13. Zuiderveen EAR, Slootweg JC, de Boer J. Novel brominated flame retardants - a review of their occurrence in indoor air, dust, consumer goods and food. Chemosphere 2020;255:126816.
14. Sjödin A, Patterson DG Jr, Bergman A. A review on human exposure to brominated flame retardants - particularly polybrominated diphenyl ethers. Environ Int 2003;29:829-39.
15. Frederiksen M, Thomsen M, Vorkamp K, Knudsen LE. Patterns and concentration levels of polybrominated diphenyl ethers (PBDEs) in placental tissue of women in Denmark. Chemosphere 2009;76:1464-9.
16. Frederiksen M, Vorkamp K, Thomsen M, Knudsen LE. Human internal and external exposure to PBDEs - a review of levels and sources. Int J Hyg Environ Health 2009;212:109-34.
17. Govarts E, Gilles L, Rodriguez Martin L, et al. Harmonized human biomonitoring in European children, teenagers and adults: EU-wide exposure data of 11 chemical substance groups from the HBM4EU Aligned Studies (2014-2021). Int J Hyg Environ Health 2023;249:114119.
18. van der Schyff V, Kalina J, Govarts E, et al. Exposure to flame retardants in European children - results from the HBM4EU aligned studies. Int J Hyg Environ Health 2023;247:114070.
19. Cooper EM, Kroeger G, Davis K, Clark CR, Ferguson PL, Stapleton HM. Results from screening polyurethane foam based consumer products for flame retardant chemicals: assessing impacts on the change in the furniture flammability standards. Environ Sci Technol 2016;50:10653-60.
20. Hoffman K, Fang M, Horman B, et al. Urinary tetrabromobenzoic acid (TBBA) as a biomarker of exposure to the flame retardant mixture Firemaster® 550. Environ Health Persp 2014;122:963-9.
21. Butt CM, Congleton J, Hoffman K, Fang M, Stapleton HM. Metabolites of organophosphate flame retardants and 2-ethylhexyl tetrabromobenzoate in urine from paired mothers and toddlers. Environ Sci Technol 2014;48:10432-8.
22. Stapleton HM, Allen JG, Kelly SM, et al. Alternate and new brominated flame retardants detected in U.S. house dust. Environ Sci Technol 2008;42:6910-6.
23. Tao F, Abou-Elwafa Abdallah M, Ashworth DC, Douglas P, Toledano MB, Harrad S. Emerging and legacy flame retardants in UK human milk and food suggest slow response to restrictions on use of PBDEs and HBCDD. Environ Int 2017;105:95-104.
24. Shi Z, Zhang L, Li J, et al. Novel brominated flame retardants in food composites and human milk from the Chinese total diet study in 2011: concentrations and a dietary exposure assessment. Environ Int 2016;96:82-90.
25. Chen T, Huang M, Li J, Li J, Shi Z. Polybrominated diphenyl ethers and novel brominated flame retardants in human milk from the general population in Beijing, China: Occurrence, temporal trends, nursing infants' exposure and risk assessment. Sci Total Environ 2019;689:278-86.
26. Schreder E, Zheng G, Sathyanarayana S, Gunaje N, Hu M, Salamova A. Brominated flame retardants in breast milk from the United States: first detection of bromophenols in U.S. breast milk. Environ Pollut 2023;334:122028.
27. Zhou T, Taylor MM, DeVito MJ, Crofton KM. Developmental exposure to brominated diphenyl ethers results in thyroid hormone disruption. Toxicol Sci 2002;66:105-16.
28. Herbstman JB, Sjödin A, Kurzon M, et al. Prenatal exposure to PBDEs and neurodevelopment. Environ Health Persp 2010;118:712-9.
29. Costa LG, Giordano G. Developmental neurotoxicity of polybrominated diphenyl ether (PBDE) flame retardants. Neurotoxicology 2007;28:1047-67.
30. Kim YR, Harden FA, Toms LM, Norman RE. Health consequences of exposure to brominated flame retardants: a systematic review. Chemosphere 2014;106:1-19.
31. Eskenazi B, Chevrier J, Rauch SA, et al. In utero and childhood polybrominated diphenyl ether (PBDE) exposures and neurodevelopment in the CHAMACOS study. Environ Health Persp 2013;121:257-62.
32. Vuong AM, Yolton K, Cecil KM, Braun JM, Lanphear BP, Chen A. Flame retardants and neurodevelopment: an updated review of epidemiological literature. Curr Epidemiol Rep 2020;7:220-36.
33. Dong L, Wang S, Qu J, You H, Liu D. New understanding of novel brominated flame retardants (NBFRs): neuro(endocrine) toxicity. Ecotox Environ Safe 2021;208:111570.
34. Belcher SM, Cookman CJ, Patisaul HB, Stapleton HM. In vitro assessment of human nuclear hormone receptor activity and cytotoxicity of the flame retardant mixture FM 550 and its triarylphosphate and brominated components. Toxicol Lett 2014;228:93-102.
35. Patisaul HB, Roberts SC, Mabrey N, et al. Accumulation and endocrine disrupting effects of the flame retardant mixture Firemaster® 550 in rats: an exploratory assessment. J Biochem Mol Toxicol 2013;27:124-36.
36. Pillai HK, Fang M, Beglov D, et al. Ligand binding and activation of PPARγ by Firemaster® 550: effects on adipogenesis and osteogenesis in vitro. Environ Health Persp 2014;122:1225-32.
37. Fromme H, Becher G, Hilger B, Völkel W. Brominated flame retardants - exposure and risk assessment for the general population. Int J Hyg Environ Health 2016;219:1-23.
38. Pedersen M, Wichmann J, Autrup H, et al. Increased micronuclei and bulky DNA adducts in cord blood after maternal exposures to traffic-related air pollution. Environ Res 2009;109:1012-20.
39. Samuel TM, Thielecke F, Lavalle L, et al. Mode of neonatal delivery influences the nutrient composition of human milk: results from a multicenter European cohort of lactating women. Front Nutr 2022;9:834394.
40. Rydahl E, Declercq E, Juhl M, Maimburg RD. Cesarean section on a rise - does advanced maternal age explain the increase? A population register-based study. PLoS One 2019;14:e0210655.
41. Vorkamp K, Thomsen M, Frederiksen M, Pedersen M, Knudsen LE. Polybrominated diphenyl ethers (PBDEs) in the indoor environment and associations with prenatal exposure. Environ Int 2011;37:1-10.
42. Frederiksen M, Thomsen C, Frøshaug M, et al. Polybrominated diphenyl ethers in paired samples of maternal and umbilical cord blood plasma and associations with house dust in a Danish cohort. Int J Hyg Environ Health 2010;213:233-42.
43. Frederiksen M, Vorkamp K, Mathiesen L, Mose T, Knudsen LE. Placental transfer of the polybrominated diphenyl ethers BDE-47, BDE-99 and BDE-209 in a human placenta perfusion system: an experimental study. Environ Health 2010;9:32.
44. Vorkamp K, Christensen JH, Glasius M, Riget FF. Persistent halogenated compounds in black guillemots (Cepphus grylle) from Greenland - levels, compound patterns and spatial trends. Mar Pollut Bull 2004;48:111-21.
45. Vorkamp K, Bossi R, Rigét FF, Skov H, Sonne C, Dietz R. Novel brominated flame retardants and dechlorane plus in Greenland air and biota. Environ Pollut 2015;196:284-91.
46. Vorkamp K, Bester K, Rigét FF. Species-specific time trends and enantiomer fractions of hexabromocyclododecane (HBCD) in biota from East Greenland. Environ Sci Technol 2012;46:10549-55.
47. Haug LS, Becher G. Interlaboratory comparison on dioxins in food 2006. Sixth round of an international study. In: Norwegian Institute of Public Health, Oslo, Norway; 2006. Available from https://www.fhi.no/en/publ/2009-and-older/interlaboratory-comparison-on-dioxi-----/ [Last accessed on 22 Feb 2024].
48. Ali N, Harrad S, Muenhor D, Neels H, Covaci A. Analytical characteristics and determination of major novel brominated flame retardants (NBFRs) in indoor dust. Anal Bioanal Chem 2011;400:3073-83.
49. EPA U. Chapter 15- human milk intake. In: Exposure factors handbook 2011 edition (final report). Washington DC: USA. Environmental Protection Agency; 2011. Available from https://www.epa.gov/sites/default/files/2015-09/documents/efh-chapter15.pdf [Last accessed on 22 Feb 2024].
50. EPA U. Update for Chapter 5 of the exposure factors handbook - soil and dust ingestion. In: Exposure factors handbook 2011 edition (final report). Washington, DC: USA. Environmental Protection Agency; 2017. Available from https://www.epa.gov/sites/default/files/2018-01/documents/efh-chapter05_2017.pdf [Last accessed on 22 Feb 2024].
51. USEPA. U.S. EPA. Exposure factors handbook 2011 edition (final report). Environmental Protection Agency, Washington DC, 2011. Available from https://cfpub.epa.gov/ncea/risk/recordisplay.cfm?deid=236252 [Last accessed on 21 Feb 2024].
52. Main KM, Kiviranta H, Virtanen HE, et al. Flame retardants in placenta and breast milk and cryptorchidism in newborn boys. Environ Health Persp 2007;115:1519-26.
53. Antignac JP, Main KM, Virtanen HE, et al. Country-specific chemical signatures of persistent organic pollutants (POPs) in breast milk of French, Danish and Finnish women. Environ Pollut 2016;218:728-38.
54. Geyer HJ, Schramm K-W, Feicht E, et al. Terminal elimination half-lives of the brominated flame retardants TBBPA, HBCD, and lower brominated PBDEs in humans. Organohalogen Compd 2004;66:3820-5. Available from: https://www.osti.gov/etdeweb/biblio/20828431 [Last accessed on 21 Feb 2024].
55. Thomsen C, Stigum H, Frøshaug M, Broadwell SL, Becher G, Eggesbø M. Determinants of brominated flame retardants in breast milk from a large scale Norwegian study. Environ Int 2010;36:68-74.
56. Björklund JA, Sellström U, de Wit CA, Aune M, Lignell S, Darnerud PO. Comparisons of polybrominated diphenyl ether and hexabromocyclododecane concentrations in dust collected with two sampling methods and matched breast milk samples. Indoor Air 2012;22:279-88.
57. Schächtele A, Malisch R, Hardebusch B, et al. WHO- and UNEP-coordinated exposure studies 2000-2019: findings of polybrominated substances (PBDE, HBCDD, PBB 153, PBDD/PBDF). In: Persistent Organic Pollutants in Human Milk. Cham: Springer International Publishing; 2023. pp. 299-342. Available from: https://doi.org/10.1007/978-3-031-34087-1_9 [Last accessed on 22 Feb 2024].
58. Ryan JJ, Rawn DF. The brominated flame retardants, PBDEs and HBCD, in Canadian human milk samples collected from 1992 to 2005; concentrations and trends. Environ Int 2014;70:1-8.
59. Shi Z, Zhang L, Li J, Wu Y. Legacy and emerging brominated flame retardants in China: a review on food and human milk contamination, human dietary exposure and risk assessment. Chemosphere 2018;198:522-36.
60. Eggesbø M, Thomsen C, Jørgensen JV, Becher G, Odland JØ, Longnecker MP. Associations between brominated flame retardants in human milk and thyroid-stimulating hormone (TSH) in neonates. Environ Res 2011;111:737-43.
61. Pratt I, Anderson W, Crowley D, et al. Brominated and fluorinated organic pollutants in the breast milk of first-time Irish mothers: is there a relationship to levels in food? Food Addit Contam Part A 2013;30:1788-98.
62. Abdallah MA, Harrad S. Tetrabromobisphenol-A, hexabromocyclododecane and its degradation products in UK human milk: relationship to external exposure. Environ Int 2011;37:443-8.
63. Harrad S, Abdallah MA. Concentrations of polybrominated diphenyl ethers, hexabromocyclododecanes and tetrabromobisphenol-A in breast milk from United Kingdom women do not decrease over twelve months of lactation. Environ Sci Technol 2015;49:13899-903.
64. Darnerud PO, Lignell S, Aune M, et al. Time trends of polybrominated diphenylether (PBDE) congeners in serum of Swedish mothers and comparisons to breast milk data. Environ Res 2015;138:352-60.
65. Ma Y, Venier M, Hites RA. Tribromophenoxy flame retardants in the Great Lakes atmosphere. Environ Sci Technol 2012;46:13112-7.
66. van der Recke R, Vetter W. Synthesis and characterization of 2,3-dibromopropyl-2,4,6-tribromophenyl ether (DPTE) and structurally related compounds evidenced in seal blubber and brain. Environ Sci Technol 2007;41:1590-5.
67. Möller A, Xie Z, Sturm R, Ebinghaus R. Polybrominated diphenyl ethers (PBDEs) and alternative brominated flame retardants in air and seawater of the European Arctic. Environ Pollut 2011;159:1577-83.
68. Shoeib M, Harner T, Webster GM, Sverko E, Cheng Y. Legacy and current-use flame retardants in house dust from Vancouver, Canada. Environ Pollut 2012;169:175-82.
69. Müller MH, Polder A, Brynildsrud OB, et al. Brominated flame retardants (BFRs) in breast milk and associated health risks to nursing infants in Northern Tanzania. Environ Int 2016;89-90:38-47.
70. Čechová E, Seifertová M, Kukučka P, et al. An effective clean-up technique for GC/EI-HRMS determination of developmental neurotoxicants in human breast milk. Anal Bioanal Chem 2017;409:1311-22.
71. Čechová E, Vojta Š, Kukučka P, et al. Legacy and alternative halogenated flame retardants in human milk in Europe: implications for children's health. Environ Int 2017;108:137-45.
72. Rawn DFK, Quade SC, Corrigan C, et al. Differences in mirex [dechlorane] and dechlorane plus [syn- and anti-] concentrations observed in Canadian human milk. Chemosphere 2023;316:137784.
73. Chi ZH, Goodyer CG, Hales BF, Bayen S. Characterization of different contaminants and current knowledge for defining chemical mixtures in human milk: a review. Environ Int 2023;171:107717.
74. Sahlström LMO, Sellström U, de Wit CA, Lignell S, Darnerud PO. Estimated intakes of brominated flame retardants via diet and dust compared to internal concentrations in a Swedish mother-toddler cohort. Int J Hyg Environ Health 2015;218:422-32.
75. Weijs L, Dirtu AC, Das K, et al. Inter-species differences for polychlorinated biphenyls and polybrominated diphenyl ethers in marine top predators from the Southern North Sea: Part 1. Accumulation patterns in harbour seals and harbour porpoises. Environ Pollut 2009;157:437-44.
76. Wu N, Herrmann T, Paepke O, et al. Human exposure to PBDEs: associations of PBDE body burdens with food consumption and house dust concentrations. Environ Sci Technol 2007;41:1584-9.
77. Coakley JD, Harrad SJ, Goosey E, et al. Concentrations of polybrominated diphenyl ethers in matched samples of indoor dust and breast milk in New Zealand. Environ Int 2013;59:255-61.
78. Toms LM, Hearn L, Kennedy K, et al. Concentrations of polybrominated diphenyl ethers (PBDEs) in matched samples of human milk, dust and indoor air. Environ Int 2009;35:864-9.
79. Jones-Otazo HA, Clarke JP, Diamond ML, et al. Is house dust the missing exposure pathway for PBDEs? An analysis of the urban fate and human exposure to PBDEs. Environ Sci Technol 2005;39:5121-30.
80. der Schyff V, Kalina J, Abballe A, Iamiceli AL, Govarts E, Melymuk L. Has regulatory action reduced human exposure to flame retardants? Environ Sci Technol 2023;57:19106-24.
81. Walters RM, Khanna P, Chu M, Mack MC. Developmental changes in skin barrier and structure during the first 5 years of life. Skin Pharmacol Phys 2016;29:111-8.
Cite This Article
Export citation file: BibTeX | RIS
OAE Style
Hammel SC, Vorkamp K, Nielsen JB, Sørensen LS, Knudsen LE, Frederiksen M. Novel and legacy brominated flame retardants in human breast milk and house dust from Denmark. J Environ Expo Assess 2024;3:8. http://dx.doi.org/10.20517/jeea.2023.51
AMA Style
Hammel SC, Vorkamp K, Nielsen JB, Sørensen LS, Knudsen LE, Frederiksen M. Novel and legacy brominated flame retardants in human breast milk and house dust from Denmark. Journal of Environmental Exposure Assessment. 2024; 3(2): 8. http://dx.doi.org/10.20517/jeea.2023.51
Chicago/Turabian Style
Hammel, Stephanie C., Katrin Vorkamp, Jesper Bo Nielsen, Lars S. Sørensen, Lisbeth E. Knudsen, Marie Frederiksen. 2024. "Novel and legacy brominated flame retardants in human breast milk and house dust from Denmark" Journal of Environmental Exposure Assessment. 3, no.2: 8. http://dx.doi.org/10.20517/jeea.2023.51
ACS Style
Hammel, SC.; Vorkamp K.; Nielsen JB.; Sørensen LS.; Knudsen LE.; Frederiksen M. Novel and legacy brominated flame retardants in human breast milk and house dust from Denmark. J. Environ. Expo. Assess. 2024, 3, 8. http://dx.doi.org/10.20517/jeea.2023.51
About This Article
Special Issue
Copyright
Data & Comments
Data
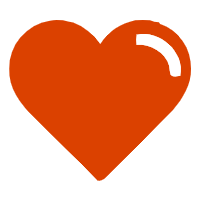

Comments
Comments must be written in English. Spam, offensive content, impersonation, and private information will not be permitted. If any comment is reported and identified as inappropriate content by OAE staff, the comment will be removed without notice. If you have any queries or need any help, please contact us at support@oaepublish.com.