Cotton pillow samplers for assessment of thirdhand smoke in homes of smokers and nonsmokers with children
Abstract
Secondhand smoke (SHS) exposure is a major cause of illnesses in children and leaves a persistent and toxic residue indoors called thirdhand smoke (THS) that adheres to various surfaces, permeates materials, accumulates in household dust, and is subsequently re-emitted into the air. THS, like SHS, has been shown to contain multiple toxic chemicals, including carcinogenic tobacco-specific nitrosamines (TSNAs). Children are highly susceptible to tobacco smoke pollutants, and simple methods for assessing children’s SHS and THS exposure are needed. Therefore, we evaluated the performance of a cotton pillow used as a passive sampler in homes of children with caregivers who smoke tobacco, with and without home smoking bans, as well as nonsmokers. We deployed a commercially available organic cotton travel pillow, which was left in the home for a median of 9.1 days. Pillow component nicotine levels were significantly higher in homes of smokers without a ban as compared to smokers and nonsmokers who had a ban (e.g., median pillowcase nicotine 337.7 ng/g per day vs. 72.5 ng/g per day and 0.1 ng/g per day, respectively) and differences were similar to those for air nicotine. Pillowcase TSNAs were detected mainly in the homes of smokers without a smoking ban. Pillow component (pillowcase, fabric, and filling) nicotine levels were highly correlated with air nicotine levels (rho = 0.76-0.88, all P < 0.001). Nicotine in the pillow components was also highly correlated with urinary cotinine in the children (rho = 0.65 to 0.81, all P < 0.001) and other measures of tobacco smoke exposure. Pillow performance as a sampler is promising, given the ease and simplicity of sample deployment.
Keywords
INTRODUCTION
SHS exposure is a major source of respiratory infections, asthma, ear infections, and many other illnesses in children[1]. Despite advances made in banning tobacco smoking in public spaces, children are still exposed to SHS at home through their caregivers[2]. In addition to being exposed to secondhand smoke via inhalation, it has recently been shown that children are exposed to the residue of tobacco smoke that deposits on surfaces, is absorbed into furniture and other materials in the home, accumulates in house dust, and is re-emitted into the air via inhalation, ingestion, and dermal absorption[3-5]. This residue is called THS, and in addition to contaminating indoor environments in homes of smokers that smoke indoors, THS has been shown to contaminate homes even when caregivers smoke outside, likely due to the residue that clings to their clothes and skin and is transported indoors[6,7]. THS can also persist in homes for months to years after smoking has ceased[8-10]. THS has been shown to contain some of the most toxic components of SHS, including but not limited to the potent carcinogen and tobacco-specific nitrosamine (TSNA) 4-(methylnitrosamino)-1-(3-pyridyl)-1-butanone (NNK)[11,12], mutagenic polycyclic aromatic hydrocarbons (PAHs)[13-15], and the heavy metals lead and cadmium[16]. Children are highly susceptible to pollutants in house dust and on surfaces due to their hand-to-mouth behaviors, activity near the floor, thin dermis, high inhalation rate relative to body weight, and developing organs[17]. For this reason, interest in assessing children’s THS exposure and SHS exposure, called total tobacco smoke exposure, is increasing.
Simple, easy-to-deploy, and retrievable samplers for THS and tobacco smoke will help in the translation of tobacco control efforts to discover and intervene in children’s tobacco smoke exposure. The most common method for assessing SHS in home environments is the measurement of airborne nicotine or respirable particles in the air[18]. Nicotine is usually measured with passive "Hammond" monitors that are placed in the home for a week[19], but also by active monitoring with a pump at a known flow rate connected to a tube that collects nicotine for later analysis[20]. For personal exposure, the most common method for assessing tobacco smoke exposure is the measurement of cotinine, a metabolite of nicotine, in urine or saliva. In addition, the metabolite of the TSNA NNK, 4-(methylnitrosamino)-1-(3-pyridyl)-1-butanol (NNAL), is also measured in urine as an indicator of exposure to this carcinogenic compound in tobacco[21]. THS pollution has typically been measured in dust sampled through vacuum collection[3,22,23] and on surfaces using a surface wipe protocol[9,24]. Here, we investigate the application of a simple cotton pillow left in a home for approximately a week as a passive sampler for THS.
The cotton pillow sampler, first described by Matt et al., 2019, consists of a small organic cotton travel pillow with a pillowcase and organic cotton stuffing[25]. Matt et al. deployed this pillow in the homes of eight former tobacco smokers who were verified to have quit smoking one week prior, and left the pillow there for three weeks, as well as deploying a pillow for the same amount of time in the homes of four nonsmokers[25]. Participants were instructed to keep the pillow on the living room sofa/couch and treat it like any other pillow. The pillows were shown to become contaminated with nicotine even in the absence of active smoking, providing the first direct evidence that THS reservoirs in the home can pollute new, clean items brought into the home. This demonstrated that deep reservoirs of THS persist in former smokers' homes and present an exposure risk even in the absence of active smoking.
In the present study, we further investigate the ability of the cotton pillow as a sampler to reflect THS contamination of the pillow resulting from SHS and THS in homes of children of caregivers who actively smoke tobacco without an indoor ban and from THS in homes of smokers and nonsmokers with an indoor ban. With the large volume of fabrics and materials in homes of active smokers (e.g., carpets, upholstery, stuffing, curtains, toys, clothes, blankets), the potential exists to accumulate significant reservoirs of THS toxicants, which can serve as exposure sources for children. This study expands the endpoints measured in the initial study of the pillow cotton sampler to include other tobacco toxicants (i.e., TSNAs) and other exposure measures in children. We compare nicotine and TSNAs in the cotton pillow sampler to other known measures of SHS and THS contamination and exposure, namely airborne nicotine in the home and personal exposure markers in the children: nicotine in worn silicone wristbands, nicotine in hand wipes, and urinary cotinine and NNAL.
MATERIALS AND METHODS
Participants
Participants in this study were a subset of families participating in the NIH-funded Healthy Families Study (Dr. Mahabee-Gittens, PI, R01HD083554) who agreed to additionally participate in this substudy (Drs. Mahabee-Gittens and Matt, MPIs, R01ES027815S1). Healthy Families was a study of caregivers who smoked combustible tobacco and their children who were recruited in pediatric emergency settings in Ohio to participate in a tobacco cessation intervention. The families were screened and recruited in the clinical setting and visited two times at later time intervals at six weeks and six months (further details are given in[26,27]). At the six-month visit, participating families in the pillow substudy deployed a clean, prescreened cotton pillow in the home in the room where the participating caregiver smoked the most or in the room where the child spent the most time (if the home had a smoking ban). A passive nicotine air monitor was placed in the same room, and the participating child was given a cleaned silicone wristband to wear. Caregiver assessments were also conducted, which included information regarding smoking behaviors in the home. Approximately one week later (for pillows, median 9.1 days, range 5.9-23.0 days), the home was visited to collect the pillow, wristband, and air sampler, and urine and hand wipe samples were obtained from the children. On a few occasions, the various samples were collected at different times due to the need for the child to be present or other scheduling factors. For the pillows, three samplers were deployed significantly longer than the air sampler (approximately 5, 7, and 14 days longer), but all were deployed at times similar to the wristband sampler. After excluding participants who had missing data for pillows (one participant lost the pillow, and one sample was not analyzed), we analyzed data for pillow deployment in 45 homes. Institutional Review Board (IRB) approval for the collection of samples was obtained from the Cincinnati Children’s Hospital Medical Center, #2017-5157, and all samples and data were deidentified before being sent to the Environmental Health laboratory at San Diego State University (SDSU) for analysis.
Cotton pillow samplers
The pillow samplers and analysis of nicotine were previously described in detail by Matt et al., 2019[25]. Briefly, the pillows were obtained from White Lotus Home (Pure Cotton Sleep Pillow, Travel Pillow 12 × 16; Highland Park, NJ; https://www.whitelotushome.com/bedding/organic-pillows-100-usda-certified-cotton.html). According to the manufacturer, the pillows and pillowcases were manufactured with 100% organic cotton with unprocessed fibers, and no treatments were applied to the pillows, pillow filling, or pillowcases. Based on one representative pillow, the pillowcase measured 0.31 m × 0.43 m and weighed 47 g. The pillow fabric cover measured 0.31 m × 0.43 m and weighed 37 g, and the cotton stuffing weighed 342 g. The pillows ordered in a batch were prescreened for nicotine contamination at the SDSU Environmental Health Laboratory using nicotine isotope dilution liquid chromatography-tandem mass spectrometry (LC-MS/MS) methods described previously[25,28]. Pillows were sent by mail with additional pillows to be used for transport/field blanks. At home, field blanks were deployed at the time of collection (blanks opened, handled in the same manner as sample pillows, then returned to storage). Of these blanks, 20% were analyzed. Blank values were subtracted from the pillow nicotine values before reporting results. Specifically, the median nicotine values in the pillow blanks were: (1) pillowcase median = 0.7 ng (25th and 75th percentiles 0.0-2.4 ng); (2) pillow fabric median = 1.5 ng (25th and 75th percentiles 0.0-6.0 ng); (3) pillow cotton stuffing median = 0.1 ng (25th and 75th percentiles 0.0-1.1 ng). Pillows were cut into fourths, then placed in a zip-style plastic freezer bag and stored at -80 °C until being shipped to SDSU on dry ice, where they were stored at -20 °C until analysis for nicotine and TSNAs.
Prior to extraction, samples for nicotine analysis were spiked with a known amount of deuterated nicotine and then extracted as in Matt et al., 2019, and the extract was stored at -20 °C until analysis by Agilent 6,460 isotope dilution liquid chromatography-tandem mass spectrometry (LC-MS/MS)[25]. The estimated method detection limit (MDL) was 0.19 ng/sample. For nicotine, 100% of pillow component samples were over the MDL. For TSNA analysis, the following four TSNAs were measured: NNK, N′-nitrosonornicotine (NNN), N′-nitrosoanabasine (NAB), and N′-nitrosoanatabine (NAT). The sample was analyzed following the published method for TSNAs in silicone wristbands[29]. Briefly, the sample was measured and weighed, then spiked with the four internal standards (NNK-d4, NAB-d4, NAT-d4, and NNN-d4). The estimated MDL was 0.10 ng/sample. Concentrations were summed to calculate total TSNAs (pg/g). Percent detection in the samples is given in Supporting information.
Silicone wristband samplers
Silicone wristband samplers for assessing tobacco exposure were prepared and deployed as described in Quintana et al., 2021, except that silicone wristbands were purchased commercially from https://24hourwristbands.com/ as in[30] in sizes extra small to large, then wristbands were cleaned with solvents and screened for nicotine contamination before being shipped[29]. Wristbands were stored and transported in clear glass jars with polytetrafluoroethylene–faced caps, with two per jar, one for the sample and one for the blank, and were stored at room temperature until deployment. Participants were instructed to wear the wristbands at all times, except if they interfered with sports or when swimming in a pool. Field blanks were obtained when wristbands were deployed by handling them similarly and then returning them to the container. Samples were shipped separately in pre-cleaned amber vials to the SDSU Environmental Health Laboratory on ice and then stored at -20 °C until analysis. Wristbands were cut into pieces, spiked with deuterated nicotine, and extracted as in[28], and extracts were stored at -20 °C until analysis for nicotine. The estimated MDL was 0.19 ng/wristband. The LOQ was approximately 1 ng/wristband. Due to the children wearing varying wristband sizes, the wristbands were weighed in the laboratory, and nicotine data in the wristband was presented as ng/g silicone. Of the 45 subjects with valid pillow data, 10 children reported losing their wristbands during the study.
Passive air monitors
The air monitors were the widely-used Hammond-style nicotine monitors consisting of 37 mm sodium bisulfate-coated Teflon filters in a cartridge with a windscreen. Monitors were provided to Cincinnati Children’s Hospital Medical Center (CCHMC), deployed with a field blank handled in the same manner, then returned to SDSU on ice and stored at -20 °C until analysis. Filters were spiked with deuterated nicotine and then extracted and analyzed by Agilent 6,460 isotope dilution (LC-MS/MS) as in our previous studies. Approximately 20% of blanks were analyzed, and all were under the estimated MDL at 0.13 ng/badge. The air nicotine level from one nonsmoker home was under the MDL.
Hand wipe collection and analysis
Hand wipes were collected as in previous studies[26,27,31,32], and a detailed protocol is given here[33]. Briefly, a cotton facial wipe (from a batch prescreened to ensure low nicotine contamination) was moistened with 1% ascorbic acid and wiped vertically and horizontally across the child’s dominant hand. A field blank was collected for each sample, and approximately 20% were analyzed and values subtracted from samples before final calculations. Field blanks had a mean of 1.1 ng nicotine/wipe and a range of 0.9-1.2ng. The length and width of the child’s hand were measured, and the area was used to express values in µg nicotine per m2. For five subjects (all nonsmokers), the hand area values were missing, and the median hand area of all subjects was used to calculate µg nicotine per m2. Samples were spiked with deuterated nicotine before extraction, and analysis was performed by Agilent 6,460 isotope dilution LC-MS/MS as described above. The estimated MDL was 0.19 ng/wipe, and all samples were greater than this value.
Urine collection and analysis
Urine was collected from the child participants, which has been detailed in prior work[26]. Urine samples were collected at the visit when the pillows were collected, immediately frozen at -80 °C, and shipped on dry ice to the Jacob Laboratory at the University of California at San Francisco. Samples were stored at -20 °C until analysis for cotinine levels and NNAL (total NNAL, free + glucuronide) levels with LC-MS/MS[21,34]. The limit of quantitation (LOQ) was 0.02 ng/ml for cotinine, and all samples were greater than the LOQ. The LOQ was 0.25 pg/mL for NNAL, and four samples of nonsmokers were less than the LOQ and were replaced with 0.18 pg/mL (LOQ/SQRT 2).
Data analysis
Field blank-corrected sample data were imported into SPSS version 28 for analysis. The pillow nicotine and TSNA data in ng/g or µg/m2 were corrected for deployment time by dividing by the number of days it was exposed in the home. The wristband nicotine data in ng/g silicone was corrected for the number of days reportedly worn by the child. Differences between groups (participants without a ban on smoking in the home at the time of the visit vs. participants with a ban on smoking in the home) were determined by the Mann-Whitney test. Correlations between measures were determined with Spearman’s rank correlation. The Type I error rate was set at α = 0.05.
RESULTS
Participant and home characteristics
Table 1 provides the characteristics of the participants and their homes. Most children in the study (n = 45) were African-American/Black, non-Hispanic (60.0%), followed by Caucasian, non-Hispanic (28.9%). Two-thirds of subjects had incomes below $15,000 per year, and just over half had an education level of some college or more. Most (84.4%) had public insurance (Medicaid). The majority of homes were apartments (31.1%) or other multiunit housing (24.4%), with 44.4% having less than 950 home square footage. Aside from five non-smoking caregivers (11.1%), most children had caregivers who smoked cigarettes (68.9%), small cigars (Black & Milds) (11.1%), or both (2.2%). About one-third of caregivers (35.8%) reported a ban on smoking in their homes. Of these, one had quit smoking since the initial study visit, but for simplicity, the category is referred to as "smokers with a ban on smoking inside the home" in the analyses below.
Participant demographics, home characteristics, and tobacco use behaviors (n = 45 participant homes)
Characteristics | n | % in category |
Child age (years, at baseline) | ||
0-1 years | 9 | 20.0 |
2-4 years | 9 | 20.0 |
5-9 years | 15 | 33.3 |
10-17 years | 12 | 26.7 |
Child gender assigned at birth (female) | 22 | 48.9 |
Child race/ethnicity | ||
African American/ Black | 27 | 60.0 |
Caucasian, non-Hispanic | 13 | 28.9 |
Other/unknown | 2 | 4.4 |
API, AI, mixed race | 3 | 6.7 |
Family income, annual | ||
< $5,000 | 17 | 37.8 |
$5,000-$15,000 | 13 | 28.9 |
> $15,000 | 15 | 33.3 |
Caregiver education level | ||
< High school | 9 | 20.0 |
High school graduate | 13 | 28.9 |
Some college and above | 23 | 51.1 |
Child insurance type | ||
Commercial | 7 | 15.6 |
Public (Medicaid) | 38 | 84.4 |
Home type | ||
Multiunit housing-apartment | 14 | 31.1 |
Multiunit housing townhouse/ | 11 | 24.4 |
condominium | 13 | 28.9 |
Single-family (including trailer) | 2 | 4.4 |
Other (including hotel) | 5 | 11.1 |
Home square feet | ||
≤ 950 | 20 | 44.4 |
> 950 | 20 | 44.4 |
missing | 5 | 11.1 |
Caregiver smoking | ||
Nonsmokers | 5 | 11.1 |
Former smoker (quit < 6 mo) | 3 | 6.7 |
Current smoker-Cigarettes | 31 | 68.9 |
Current Smoker-Black and Milds (small cigars) | 5 | 11.1 |
Current Smoker-Both cigarettes and B&M | 1 | 2.2 |
Smoking ban indoors at home (at time of visit) | ||
No | 29 | 64.4 |
Yes | 16 | 35.6 |
Nicotine and TSNA in the pillow components
Table 2 displays the nicotine and TSNA contamination in the pillow components. Nicotine contamination was detected in all components of the pillow sampler, with 100% of samples > LOQ. The pillowcase absorbed a median of 185.2 ng/g per day of nicotine, more than the pillow fabric cover (median 59.1 ng/g per day) and the pillow cotton filling (median 13.0 ng/g per day) [Table 2]. Although detectable in nonsmokers’ home pillows, the levels were very low, with a median of 0.1, 0.2, and 0.1 ng/g per day, respectively. Levels of nicotine in the pillow were highest in homes of smokers without a smoking ban (median 333.7 ng/g per day in the pillowcase, 4.7 times greater than the concentration in homes of smokers with a ban on indoor smoking, with a median 72.5 ng/g per day, and pillowcase nicotine from homes without a smoking ban was significantly higher than homes with a ban (P < 0.001). Levels in the pillow fabric cover were also greater in homes of smokers without a ban (114.0 ng/g per day vs. 30.2 ng/g per day) and in the pillow filling (20.8 ng/g per day vs. 9.0 ng/g per day), and differences between homes without and a ban were also significant (P < 0.001). When expressed as loading, pillowcase nicotine was a median of 30.1 µg/m2-day and was also greater in homes of smokers with no ban on indoor smoking (median 54.7 µg/m2-day vs. 14.8 µg/m2-day), as was nicotine in the pillow fabric cover. The majority of pillows were placed in the living room: in homes of smokers with no ban, 89.7% were placed in the living room, compared to 81.3% in homes with a smoking ban, and this difference was not significant.
Pillow nicotine and TSNAs, overall and by smoking ban status, and other tobacco-related exposure measures
All samples median (n) Min, 25th, 75th, Max | Nonsmokers median (n) Min, 25th, 75th, Max | Smokers with ban median (n) Min, 25th, 75th, Max | Smokers without ban median (n) Min, 25th, 75th, Max | |
Pillow Component-Nicotine concentration, ng/g-day | ||||
Pillowcase | 185.2 (44) 0.0, 55.2, 545.4, 4188.1 | 0.1 (5) 0.0, 0.0, 0.3, 0.5 | 72.5 (11) 17.2,37.3, 105.9, 1281.4 | 337.7*** (28) 39.0, 179.7, 1045.3, 4188.1 |
Pillow fabric | 59.1 (42) 0.0, 15.3, 171.3, 566.3 | 0.2 (5) 0.0, 0.1, 0.3, 0.3 | 30.2 (11) 7.0, 9.8, 57.2, 182.3 | 114.0*** (28) 14.8, 53.0, 244.1, 566.3 |
Pillow filling | 13.0 (45) 0.1, 2.9, 34.5, 316.7 | 0.1 (5) 0.1, 0.1, 0.2, 0.2 | 9.0 (11) 0.4, 3.7, 22.3, 35.1 | 20.8*** (28) 1.2, 5.2, 54.7, 316.7 |
Pillow Component-Nicotine loading, µg/m2-day | ||||
Pillowcase | 30.1 (44) 0.0, 11.5, 89.7, 627.7 | 0.0 (5) 0.0, 0.0, 0.0, 0.1 | 14.8 (11) 5.8, 10.7, 22.9, 189.8 | 54.7*** (28) 6.1, 28.1, 195.7, 627.7 |
Pillow fabric | 12.6 (42) 0.0, 4.5, 30.6, 163.7 | 0.0 (5) 0.0,0.0, 0.0, 0.0 | 8.7 (11) 1.1, 3.9, 12.3, 68.0 | 25.6*** (26) 2.4, 10.2, 42.5, 163.7 |
Pillow Component-Total TSNA concentration, pg/g-day | ||||
Pillowcase | 0.00 (42) 0.00, 0.00, 0.02, 0.25 | 0.00 (5) 0.00, 0.00, 0.00, 0.00 | 0.00 (10) 0.00, 0.00, 0.00, 0.12 | 0.00* (27) 0.00, 0.00, 0.06, 0.25 |
Other sample types | ||||
Air nicotine, µg/m3 | 1.42 (44) 0.0, 0.49, 3.2, 13.5 | 0.00 (4) 0.00, 0.00, 0.00, 0.00 | 0.52 (11) 0.03, 0.10, 1.08, 2.73 | 2.38*** (29) 0.30, 1.0, 4.1, 13.5 |
Child wristband nicotine ng/g-day | 101.0 (35) 0.0, 46.2, 210.4, 1435.4 | 0.1 (4) 0.0, 0.05, 0.5, 0.6 | 88.6 (11) 8.9. 14.9, 101.6, 210.4 | 185.7*** (20) 44.6, 90.0, 378.7, 1435.4 |
Child hand nicotine, µg/m2 | 7.8 (43) 0.0, 2.4, 26.9, 334.3 | 0.0 (4) 0.0, 0.0, 0.1, 0.1 | 12.1 (11) 0.4, 1.1, 26.9, 49.8 | 10.8* (27) 0.5, 4.6, 32.5, 334.3 |
Child urinary cotinine, ng/mL | 11.6 (42) 0.4, 3.8, 20.0, 56.1 | 0.04 (5) 0.04, 0.04, 0.06, 0.09 | 6.2 (11) 0.5, 1.7, 12.4, 32.6 | 15.6*** (26) 1.2, 10.6, 22.1, 56.2 |
Child urinary NNAL, pg/mL | 31.7 (42) 0.2, 10.4, 42.9, 254.9 | 0.2 (5) 0.2, 0.2, 0.2, 0.3 | 24.2 (11) 1.5, 7.1, 42.8, 70.7 | 39.0** (26) 4.7, 14.1, 48.9, 254.9 |
We measured TSNAs in the pillowcase only. The sum of the four TSNAs measured, the known carcinogens NNK and NNN, as well as NAB and NAT, had a low overall detection rate (28.6%) and were mainly detected in the pillows deployed in homes of smokers with no smoking ban (40.7% detection, vs. 10% in homes of smokers and former smokers with no ban, supporting online material) [Supplementary Table 1]. Concentrations of individual TSNAs are given in supporting online material [Supplementary Table 1]. Pillowcase TSNAs were significantly higher in the group of smokers with no ban on smoking inside the home compared to homes with a ban (P < 0.05) [Table 2].
Other measures of contamination and exposure in the home
Table 2 presents levels of tobacco-related pollutants in air, in silicone wristbands worn by the children, in hand wipes, and in children’s urine. Indoor air average concentrations of nicotine were approximately 4.6 times higher in homes of smokers with no ban on indoor smoking, similar to the pillowcase nicotine, with a median concentration of 2.38 µg/m3, as compared to 0.52 µg/m3 in homes of smokers with an indoor ban. Levels of air nicotine were significantly higher in homes of smokers with no ban on indoor smoking (P < 0.001). Wristband nicotine levels were also significantly higher when worn by children residing in homes of smokers with no indoor ban on smoking by a factor of 2.1, median of 185.7 ng/g per day vs. median of 88.6 ng/g per day (P < 0.001). For child’s hand nicotine, a measure of the child’s exposure to THS, differences were less marked between homes of smokers with no ban and those homes with a ban on smoking indoors (medians were similar, at 10.8 µg/m2 and 12.1 µg/m2), though the group as a whole with no ban on smoking in the home had higher nicotine on the hands, P < 0.05). In child’s urine, sampled at the time pillows were retrieved, cotinine levels were 2.5 fold higher (P < 0.001) in children living in homes of smokers with no ban as compared to homes with a ban on smoking indoors (median 15.6 ng/ml vs. 6.2 ng/mL, respectively). Levels of urinary NNAL were higher among children living in homes with no ban on smoking as compared to children living in homes with a ban on smoking (P < 0.01, median 39.0 pg/mL and median 24.2 pg/mL, respectively).
Associations of pillow nicotine and TSNAs with other tobacco smoke contamination and exposure measures
Table 3 displays the correlation between tobacco-related measures in this study. The pillowcase nicotine concentration was highly positively correlated with the pillow fabric concentration (rho = 0.894, P < 0.001) and the pillow stuffing concentration (rho = 0.731, P < 0.001). All three components were significantly positively correlated with total TSNA concentrations in the pillow components, although to a lesser extent (rho = 0.536-0.575, all P < 0.001). All components of pillow nicotine were also significantly correlated with air nicotine (rho = 0.759-0.882, all P < 0.001). Pillow component nicotine concentrations were all significantly correlated with the measures of the child’s personal exposure to tobacco pollution, wristband nicotine concentration (rho = 0.687-0.792, all P < 0.001), hand nicotine loading (rho = 0.618-0.681, all P < 0.001), urinary cotinine (rho = 0.648-0.811, all P < 0.01), and urinary NNAL (rho = 0.615-0.731, all P < 0.001). Pillowcase TSNA concentrations, to a lower extent, were correlated with air levels of nicotine (rho = 0.481, P < 0.01) as well as the personal exposure measures wristband nicotine concentrations (rho = 0.594, P < 0.001), hand nicotine loading (rho = 0.336, P < 0.05), and urinary cotinine, (rho = 0.334, P < 0.05), although not with urinary NNAL, a measure of exposure to NNK, a component of the total TSNAs. We also examined the correlation between NNK concentrations in the pillowcase and NNAL in the child’s urine, as NNK is the parent compound to NNAL, and this correlation was low and not significant (rho = 0.136, P > 0.05).
Correlation between tobacco-related pillow components and other secondhand/thirdhand tobacco smoke exposure measures
Pillowcase nicotine, µg/g-day | Pillow fabric nicotine, µg/g-day | Pillow filling nicotine, µg/g-day | Pillowcase total TSNAs, ng/g-day | Air nicotine, µg/m3 | Wristband nicotine, ng/g-day | Hand nicotine, µg/m2 | Urine cotinine, ng/mL | Urine NNAL, pg/mL | |
Pillowcase nicotine, µg/g-day | |||||||||
Pillow fabric nicotine, µg/g-day | 0.894*** 41 | ||||||||
Pillow filling nicotine, µg/g-day | 0.731*** 44 | 0.785*** 42 | |||||||
Pillowcase total TSNAs, ng/g-day | 0.536*** 42 | 0.567*** 38 | 0.575** 42 | ||||||
Air nicotine, µg/m3 | 0.779*** 43 | 0.882*** 41 | 0.759** 44 | 0.481** 41 | |||||
Wristband nicotine, ng/g-day | 0.714*** 34 | 0.792*** 32 | 0.687** 35 | 0.594*** 32 | 0.749*** 35 | ||||
Hand nicotine, µg/m2 | 0.681*** 42 | 0.650*** 40 | 0.618*** 43 | 0.336* 40 | 0.606* 42 | 0.580*** 34 | |||
Urine cotinine, ng/ mL | 0.710*** 42 | 0.811*** 39 | 0.648** 42 | 0.334* 39 | 0.805*** 41 | 0.693*** 33 | 0.617*** 42 | ||
Urine NNAL, pg/mL | 0.615*** 41 | 0.731*** 39 | 0.674*** 42 | 0.306 39 | 0.678*** 41 | 0.726*** 33 | 0.510*** 42 | 0.749*** 41 |
DISCUSSION
Nicotine levels in the simple cotton pillow samplers were similar to other established and validated measures of tobacco pollution in the same home. Pillow component nicotine levels (concentration, ng/g per day) were significantly higher in homes of smokers without a ban as compared to current smokers and nonsmokers with a ban, and the differences between the groups were similar in magnitude to that shown by air nicotine levels in the home. Nicotine in pillow components was also highly correlated with air nicotine levels (all rho > 0.750). Urinary cotinine in the children was significantly positively correlated with the amount of nicotine in the pillow almost to the same extent as urinary cotinine was correlated with air nicotine (correlations of urinary cotinine and pillow components rho = 0.648-0.811 vs. the correlation of air nicotine with cotinine rho = 0.805). Wristband nicotine, which has previously been shown to track well with children’s urine cotinine, was also significantly correlated with pillow component nicotine (e.g., wristband nicotine and pillowcase nicotine, rho = 0.714). Wristband nicotine, urinary cotinine, and urinary NNAL are personal exposure measures that reflect exposures from other locations besides the home, in contrast to air nicotine and pillow nicotine, which reflect exposures in the home only. The high correlation between nicotine in the pillow sampler and the personal exposure measures is likely due to the fact that the children receive most of their exposure at home. The performance of pillows as sampling devices is surprising, given the ease of sample deployment (i.e., participants were instructed to use the pillow as they wished).
A large amount of nicotine was absorbed by the clean pillow during deployment in the home (median 9.1 days). Supplementary Table 2 presents estimates of the total nicotine absorbed per day by each pillow, both as total nicotine quantity per pillow and mg nicotine per day exposed in the home. Pillows absorbed a median of 167 µg of nicotine in total (a maximum of 2,843 µg) and a median of 15.5 µg nicotine/day, with a median of 30.5 µg nicotine /day in homes of caregivers who smoke and have no smoking ban inside the home. For comparison, the cotton pillows (same size and type) deployed for three weeks in the earlier study conducted by Matt et al. in 2019 absorbed approximately 1 µg/day (median)[25]. These homes had no active smoking inside, and all nicotine in the pillow was associated with re-emission from thirdhand smoke reservoirs in homes of former smokers, either from air nicotine, nicotine bound to resuspended particles, or contact of the pillow with THS contaminated occupants or furnishings. Nicotine air levels in the study by Matt et al. in 2019 were much lower, at a median of 10.1 ng/m3[25]. In our study, airborne nicotine levels were a median of 2.4 µg/m3 or approximately 240 times higher in homes of active smokers and 0.5 µg/m3 or 50 times higher in homes of smokers without a ban. We wish to note that the airborne nicotine samples in Matt et al.’s study in 2019 were collected for a period of 2 days by an active air sampler operated at 1.5 LPM into an XAD-4 tube (followed by the same analysis for nicotine as in this study, isotope dilution LC-MS/MS,) as compared to the 7+ days that the passive air samplers collected airborne nicotine levels in the data reported here[25]. Therefore, the airborne nicotine data is not directly comparable given the time period assessed and different methods used; however, the comparison is offered to illustrate the potential for high absorption of nicotine into the pillow in a home with active smoking. Similar to Matt et al.’s study in 2019, the fraction of nicotine in the pillow was highest in the pillowcase, followed by the pillow cotton filling, and finally, the pillow cover (all P < 0.001)[25] [Supplementary Table 2].
Nicotine in the pillow reflects direct THS contamination in the home. In just a week or so, the fact that just this one small pillow absorbed a median of 30.5 µg nicotine/day illustrates the potential of furnishings, clothing, toys, etc. to absorb and retain nicotine and other THS components in the homes of smokers. In chamber studies, nicotine has been shown to quickly adhere to furnishings upon emission and to be released at a slower rate[35]. In addition to nicotine, other toxic contaminants such as PAHs have been demonstrated to swiftly adhere to fabrics and materials placed inside a chamber[14], although sorption differs by fabric type, with natural fibers holding and releasing more nicotine[36]. It is, therefore, likely that the cotton fabric and stuffing are a reliable substrate to measure the absorption of nicotine in indoor environments. Nicotine on surfaces has the potential to form carcinogenic TSNAs[37] through secondary reactions with ambient oxidants, making exposure to children a concern.
We compared the effective sampling rate of the pillow to the sampling rate of the passive Hammond nicotine air monitor used side by side in this study to estimate a sampling rate if all nicotine came from the air. This calculation assumes nicotine transfer from the body or surfaces to the pillow is negligible. In homes without a ban on smoking, the median nicotine level collected on the air filter was 0.0821 µg/nicotine per day (in the same homes for which data on all pillow components was available). When compared to the Hammond monitor sampling rate of 25 ml/minute, and assuming all the nicotine was coming from the air, the pillow was absorbing and retaining nicotine at 356 times the rate of the Hammond sampler, or 9.3 liters per minute. For non-smoking homes, with an estimated median of 8.2 µg/day on the pillow and a median of 0.0188 µg/day collected on the Hammond air filter, the estimated rate was 10.9 liters per minute. The difference in surface area (flat dimension) between the pillow and the 37 mm diameter air monitor filter is an estimated factor of 124 times higher in the pillow sampler assuming a flat surface. The pillow likely has a higher surface area than that calculated from the rough dimensions of the pillow due to the 3D structure and thick cotton cover. In a study of air uptake into cotton and rayon cloth hung in contaminated homes of the indoor air contaminants phthalates and halogenated flame retardants, uptake rates were reported as sampling 35-92 m3/day per m2[38]. Estimated sampling rates for the total pillow in this study were 100.5-116.7m3/per m2, assuming all nicotine came from the air. In another comparison, examining the pillowcase only, nicotine loading in µg/m2 per day was similar to or slightly lower than that collected on the Hammond badge when adjusted for surface area. The pillow sampler appears, therefore, to be retaining nicotine at a high rate similar to the Hammond air monitor, which is surprising as the air monitor has a filter treated with sodium bisulfite to retain all nicotine that contacts it, whereas the nicotine can both adsorb to and desorb from the cotton pillow sampler. If significant contamination by nicotine in the pillow were arising from sources other than air, then this sampling rate would be an overestimate. However, air uptake rates are not dissimilar to those reported for phthalates and halogenated flame retardants, as previously mentioned in this paragraph. In addition, the estimated median uptake rates (9.3 LPM and 10.9 LPM) were fairly similar when calculated for homes without a smoking ban and homes with a smoking ban, which might be expected to differ in the amount of physical transfer. While we cannot rule out that some transfer of nicotine may have taken place from residents' bodies or other surfaces, the substantial correlation between pillow nicotine concentrations and air nicotine concentration suggests that the contributions were small, certainly substantially smaller than from air nicotine.
The surface of the pillowcase was significantly contaminated with nicotine compared to values collected through the surface wipe technique in homes in our previous studies. The total surface loading (not adjusted by day) for the pillowcase and pillow cover is given in Supplementary Table 3. The surface loading in the pillowcases in this study (median 288 µg/m2 overall and a median 682 µg/m2 in homes of active smokers with no ban on smoking in the home, SI) [Supplementary Table 3] is higher than the geometric mean levels wiped from surfaces in homes of smokers who smoked inside (median 98.7 µg/m2 nicotine in one study[8], geometric mean 145.6 µg/m2 in a multiunit subsidized housing study)[39], and on surfaces in homes of smokers before quitting (geometric mean 31.2 µg/m2)[10]. This is surprising since these pillows were clean prior to being deployed in the home for a little over a week. One explanation is that the surface wipe technique does not collect all the nicotine in the material being wiped, and significant reservoirs of nicotine are present in fabrics in the homes, as well as other porous materials such as drywall. However, since we did not collect surface wipes for nicotine in these same homes, we cannot conclude that this is the case.
The cotton pillow sampler absorbed the toxic THS compounds TSNAs in addition to nicotine, although fewer than 30% had detectable levels. Pillowcases contained NNK, a known lung carcinogen, which was only present in pillows from homes of smokers with no smoking ban, and levels of total TSNAs were significantly higher in pillowcases from homes of this group. Other TSNAs (NNN, NAB, NAT) were present in pillowcases from smokers and former smokers with indoor smoking bans. This demonstrates that children could be exposed to these toxic contaminants through THS. The levels of nicotine wiped from the hands of the children living with smokers with a ban were more similar to those living with smokers without a ban, as opposed to the differences seen in other measures. Although children presumably clean their hands daily, the levels are only 10-fold or less than those wiped from surfaces in homes of smokers smoking indoors, as given in the previous paragraph. This demonstrates the potential for children to be exposed through touching THS-contaminated environments. Although total TSNAs in pillows were correlated with air nicotine, urine cotinine, and wristband nicotine, levels were not significantly correlated with urinary NNAL, a metabolite of NNK, and neither were NNK levels. This could be due to the low level of detection of the TSNAs in the pillowcase.
Even though the pillow sampler is simple to deploy and retrieve, there are disadvantages. A pillow was lost in the study and could not be retrieved. There were no losses of the air monitor; however, more wristbands were lost (10/45) than any other sampler used. This may have been due to a lack of age-appropriate wristband sizes for the children in this study or a selection of non-preferred colors. Additionally, the pillow sampler (similar to the air and wristband samplers) requires a period of deployment in the home to absorb THS, a median of nine days in this study. Other measures, such as urine sampling or surface wipes, can be obtained in one visit. However, the pillow sampler is uncontaminated when deployed and left for a defined period of time, while surface wipes, house dust samples, and urinary cotinine may measure differing time scales. The persistence of nicotine on surfaces and in house dust is unknown, but it is known to persist for months, even after cleaning when new non-smoking tenants move into smoker’s homes, and for years after smoking has ceased in the home[8-10]. For urinary NNAL, the half-life is 2-3 weeks, and for urinary cotinine 16- 20 h[40,41]. A limitation of our study is that we did not compare results to dust or surface wipe sampling in the same home, which can give us a robust measure of existing THS contamination in the home. In addition, for the pillow and the wristband samplers, we did not have a record of behaviors that might affect the nicotine concentrations (e.g., wearing the wristband while showering, and handling the pillow). However, as we were interested in these samplers as simple community-friendly devices, we wished to put few limitations on use and ask as few intrusive questions as possible.
The choice of the best measure of SHS/THS in the home would depend on several factors, including practical concerns such as the number of visits to the house by the sampling team or if visits are possible at all, whether personal measurements or home-based measurements are desired, and whether sampling equipment and trained personnel are available. Home-based measures include the cotton pillow sampler detailed in this study, air nicotine measurements, and nicotine and other tobacco pollutants measured in collected house dust and surface samples. Personal measurements include urine, saliva, silicone wristbands, and hand wipes. The cotton pillow sampler is easy to send to interested parties, easy to deploy, and is a robust sampler, meaning that it can be placed where people in the home can access it. It also gives a measure of contamination for the defined sampling period and can reflect both THS and SHS exposure. Two visits are needed, to deploy the pillow and to pick it up, but it is possible that the pillow sampler could be mailed back, although this has not yet been tested. Nicotine in air measurement using the passive Hammond sampler is the accepted standard for air concentrations of nicotine due to SHS, but it may not measure low levels of nicotine coming from THS contamination only, due to a low sampling rate. The passive "Hammond" air samplers must be protected from handling as well. For THS assessment, where a defined exposure time is not of interest, measurement of nicotine and/or TSNAs on house dust or surfaces allows for a single visit to the home, and reflects THS, not SHS, contamination only. Therefore, the use of the pillow sampler may be best suited for community-based activities regarding SHS/THS exposure, with untrained participants collecting their own samples.
In conclusion, the cotton pillow sampler performance is promising for measuring THS, given the ease and informality of sample deployment. One of the contributions of the data provided in this paper is showing how much THS in the form of nicotine can be absorbed by the small pillow in homes of smokers, thus illustrating the large amounts of nicotine that can persist in a home in the form of THS. The high concordance of nicotine in the pillow with established air sampling methods for nicotine in the home and strong correlations with other measures of total tobacco smoke exposure, including urinary and the silicone wristband measures, indicate that the pillow sampler is a community-friendly tool to add to existing methods for assessing THS and SHS contamination.
DECLARATIONS
Authors’ contributions
Provided input on study design, performed the statistical analysis, drafted the manuscript, and provided final edits: Quintana PJE
Coordinated sample processing and analysis and contributed to writing and editing: Lopez-Galvez N
Contributed to the acquisition of funding for the project, led the study design, contributed to the analysis and interpretation of data and writing and editing of the manuscript: Matt GE
Curated the database and coding of data, provided writing and edits: Merianos AL
Developed analytical methods for TSNA detection in pillows and oversaw laboratory analysis of cotinine: Dodder NG
Gave input into study design, managed and oversaw all laboratory analysis, and contributed to writing and edits: Hoh E
Trained field staff, oversaw field data collection, processing and shipping of field samples, and contributed to edits: Stone L
Performed field data collection and processed samples: Wullenweber CA
Acquired funding for the project, co-led the study design, oversaw data collection in the field, contributed to the analysis and interpretation of data, and the writing and editing of the manuscript: Mahabee-Gittens EM
Availability of data and materials
The data that support the findings of this study are available from the corresponding author, Quintana PJE, upon reasonable request.
Financial support and sponsorship
This research was supported by funds from the California Tobacco-Related Disease Research Program of the University of California 28PT-0079 (PI Quintana PJE); T33PC6863, T32PT6244 (PI Matt GE); NIH R01HD083554 (Dr. Mahabee-Gittens EM); R01ES027815S1 (Drs. Mahabee-Gittens EM and Matt GE, MPIs); R01ES030743 (Dr. Mahabee-Gittens EM, PI); R21ES032161 (Dr. Merianos AL, PI); K01DA044313 (Dr. Merianos AL, PI).
Conflicts of interest
All authors declared that there are no conflicts of interest.
Ethical approval and consent to participate
This study was conducted with the approval of the CCHMC and the San Diego State University Institutional Review Board.
Consent for publication
Not applicable.
Copyright
© The Author(s) 2023.
Supplementary Materials
REFERENCES
1. Office on Smoking and Health (US). The health consequences of involuntary exposure to tobacco smoke: a report of the surgeon general. Atlanta (GA): Centers for Disease Control and Prevention (US); 2006.
2. Agaku IT, Odani S, King BA, Armour BS. Prevalence and correlates of secondhand smoke exposure in the home and in a vehicle among youth in the United States. Prev Med 2019;126:105745.
3. Jacob P 3rd, Benowitz NL, Destaillats H, et al. Thirdhand smoke: new evidence, challenges, and future directions. Chem Res Toxicol 2017;30:270-94.
4. Arfaeinia H, Ghaemi M, Jahantigh A, Soleimani F, Hashemi H. Secondhand and thirdhand smoke: a review on chemical contents, exposure routes, and protective strategies. Environ Sci Pollut Res Int 2023;30:78017-29.
5. Díez-Izquierdo A, Cassanello-Peñarroya P, Lidón-Moyano C, Matilla-Santander N, Balaguer A, Martínez-Sánchez JM. Update on thirdhand smoke: a comprehensive systematic review. Environ Res 2018;167:341-71.
6. Matt GE, Quintana PJ, Hovell MF, et al. Households contaminated by environmental tobacco smoke: sources of infant exposures. Tob Control 2004;13:29-37.
7. Kelley ST, Liu W, Quintana PJE, et al. Altered microbiomes in thirdhand smoke-exposed children and their home environments. Pediatr Res 2021;90:1153-60.
8. Matt GE, Quintana PJ, Zakarian JM, et al. When smokers move out and non-smokers move in: residential thirdhand smoke pollution and exposure. Tob Control 2011;20:e1.
9. Matt GE, Quintana PJE, Hoh E, et al. Persistent tobacco smoke residue in multiunit housing: Legacy of permissive indoor smoking policies and challenges in the implementation of smoking bans. Prev Med Rep 2020;18:101088.
10. Matt GE, Quintana PJE, Zakarian JM, et al. When smokers quit: exposure to nicotine and carcinogens persists from thirdhand smoke pollution. Tob Control 2017;26:548-56.
11. Pozuelos GL, Jacob P 3rd, Schick SF, Omaiye EE, Talbot P. Adhesion and removal of thirdhand smoke from indoor fabrics: a method for rapid assessment and identification of chemical repositories. Int J Environ Res Public Health 2021;18:3592.
12. Tang X, Benowitz N, Gundel L, et al. Thirdhand exposures to tobacco-specific nitrosamines through inhalation, dust ingestion, dermal uptake, and epidermal chemistry. Environ Sci Technol 2022;56:12506-16.
13. Hoh E, Hunt RN, Quintana PJE, et al. Environmental tobacco smoke as a source of polycyclic aromatic hydrocarbons in settled household dust. Environ Sci Technol 2012;46:4174-83.
14. Schick SF, Farraro KF, Perrino C, et al. Thirdhand cigarette smoke in an experimental chamber: evidence of surface deposition of nicotine, nitrosamines and polycyclic aromatic hydrocarbons and de novo formation of NNK. Tob Control 2014;23:152-9.
15. Matt GE, Greiner L, Record RA, et al. Policy-relevant differences between secondhand and thirdhand smoke: strengthening protections from involuntary exposure to tobacco smoke pollutants. Tob Control 2023:1-9.
16. Matt GE, Quintana PJE, Hoh E, et al. Tobacco smoke is a likely source of lead and cadmium in settled house dust. J Trace Elem Med Biol 2021;63:126656.
17. US EPA. Child-Specific Exposure Factors Handbook (2008, Final Report). U.S. Environmental Protection Agency, Washington, DC, EPA/600/R-06/096F, 2008. Available from: https://cfpub.epa.gov/ncea/risk/recordisplay.cfm?deid=199243 [Last accessed on 8 Nov 2023].
18. Apelberg BJ, Hepp LM, Avila-tang E, et al. Environmental monitoring of secondhand smoke exposure. Tob Control 2013;22:147-55.
19. Hammond SK, Leaderer BP. A diffusion monitor to measure exposure to passive smoking. Environ Sci Technol 1987;21:494-7.
20. Matt GE, Quintana PJE, Hoh E, et al. A Casino goes smoke free: a longitudinal study of secondhand and thirdhand smoke pollution and exposure. Tob Control 2018;27:643-9.
21. Jacob P 3rd, Havel C, Lee DH, Yu L, Eisner MD, Benowitz NL. Subpicogram per milliliter determination of the tobacco-specific carcinogen metabolite 4-(methylnitrosamino)-1-(3-pyridyl)-1-butanol in human urine using liquid chromatography-tandem mass spectrometry. Anal Chem 2008;80:8115-21.
22. Continente X, Henderson E, López-González L, et al. TackSHS Project Investigators. Exposure to secondhand and thirdhand smoke in private vehicles: Measurements in air and dust samples. Environ Res 2023;235:116681.
23. Matt GE, Quintana PJE, Hoh E, et al. Remediating thirdhand smoke pollution in multiunit housing: temporary reductions and the challenges of persistent reservoirs. Nicotine Tob Res 2021;23:364-72.
24. Quintana PJE, Matt GE, Chatfield D, Zakarian JM, Fortmann AL, Hoh E. Wipe sampling for nicotine as a marker of thirdhand tobacco smoke contamination on surfaces in homes, cars, and hotels. Nicotine Tob Res 2013;15:1555-63.
25. Matt GE, Hoh E, Quintana PJE, Zakarian JM, Arceo J. Cotton pillows: a novel field method for assessment of thirdhand smoke pollution. Environ Res 2019;168:206-10.
26. Mahabee-Gittens EM, Merianos AL, Jandarov RA, Quintana PJE, Hoh E, Matt GE. Differential associations of hand nicotine and urinary cotinine with children's exposure to tobacco smoke and clinical outcomes. Environ Res 2021;202:111722.
27. Mahabee-Gittens EM, Merianos AL, Stone L, et al. Hand nicotine as an independent marker of thirdhand smoke pollution in children's environments. Sci Total Environ 2022;849:157914.
28. Quintana PJE, Hoh E, Dodder NG, et al. Nicotine levels in silicone wristband samplers worn by children exposed to secondhand smoke and electronic cigarette vapor are highly correlated with child's urinary cotinine. J Expo Sci Environ Epidemiol 2019;29:733-41.
29. Quintana PJE, Lopez-Galvez N, Dodder NG, et al. Nicotine, cotinine, and tobacco-specific nitrosamines measured in children's silicone wristbands in relation to secondhand smoke and e-cigarette vapor exposure. Nicotine Tob Res 2021;23:592-9.
30. O'Connell SG, Kincl LD, Anderson KA. Silicone wristbands as personal passive samplers. Environ Sci Technol 2014;48:3327-35.
31. Mahabee-Gittens EM, Merianos AL, Hoh E, Quintana PJ, Matt GE. Nicotine on children's hands: limited protection of smoking bans and initial clinical findings. Tob Use Insights 2019;12:1179173X18823493.
32. Mahabee-Gittens EM, Merianos AL, Matt GE. Preliminary evidence that high levels of nicotine on children's hands may contribute to overall tobacco smoke exposure. Tob Control 2018;27:217-9.
33. Mahabee-Gittens EM, Quintana PJE, Hoh E, et al. Collecting Hand Wipe Samples to Assess Thirdhand Smoke Exposure. Front Public Health 2021;9:770505.
34. Jacob P 3rd, Yu L, Duan M, Ramos L, Yturralde O, Benowitz NL. Determination of the nicotine metabolites cotinine and trans-3'-hydroxycotinine in biologic fluids of smokers and non-smokers using liquid chromatography-tandem mass spectrometry: biomarkers for tobacco smoke exposure and for phenotyping cytochrome P450 2A6 activity. J Chromatogr B Analyt Technol Biomed Life Sci 2011;879:267-76.
35. Singer BC, Hodgson AT, Guevarra KS, Hawley EL, Nazaroff WW. Gas-phase organics in environmental tobacco smoke. 1. Effects of smoking rate, ventilation, and furnishing level on emission factors. Environ Sci Technol 2002;36:846-53.
36. Borujeni ET, Yaghmaian K, Naddafi K, Hassanvand MS, Naderi M. Identification and determination of the volatile organics of third-hand smoke from different cigarettes and clothing fabrics. J Environ Health Sci Eng 2022;20:53-63.
37. Sleiman M, Gundel LA, Pankow JF, Jacob P 3rd, Singer BC, Destaillats H. Formation of carcinogens indoors by surface-mediated reactions of nicotine with nitrous acid, leading to potential thirdhand smoke hazards. Proc Natl Acad Sci U S A 2010;107:6576-81.
38. Saini A, Okeme JO, Mark Parnis J, McQueen RH, Diamond ML. From air to clothing: characterizing the accumulation of semi-volatile organic compounds to fabrics in indoor environments. Indoor Air 2017;27:631-41.
39. Hood NE, Ferketich AK, Klein EG, Pirie P, Wewers ME. Associations between self-reported in-home smoking behaviours and surface nicotine concentrations in multiunit subsidised housing. Tob Control 2014;23:27-32.
40. Goniewicz ML, Eisner MD, Lazcano-Ponce E, et al. Comparison of urine cotinine and the tobacco-specific nitrosamine metabolite 4-(methylnitrosamino)-1-(3-pyridyl)-1-butanol (NNAL) and their ratio to discriminate active from passive smoking. Nicotine Tob Res 2011;13:202-8.
Cite This Article
How to Cite
Quintana, P. J. E.; Lopez-Galvez N.; Matt G. E.; Merianos A. L.; Dodder N. G.; Hoh E.; Stone L.; Wullenweber C. A.; Mahabee-Gittens E. M. Cotton pillow samplers for assessment of thirdhand smoke in homes of smokers and nonsmokers with children. J. Environ. Expo. Assess. 2024, 3, 1. http://dx.doi.org/10.20517/jeea.2023.28
Download Citation
Export Citation File:
Type of Import
Tips on Downloading Citation
Citation Manager File Format
Type of Import
Direct Import: When the Direct Import option is selected (the default state), a dialogue box will give you the option to Save or Open the downloaded citation data. Choosing Open will either launch your citation manager or give you a choice of applications with which to use the metadata. The Save option saves the file locally for later use.
Indirect Import: When the Indirect Import option is selected, the metadata is displayed and may be copied and pasted as needed.
About This Article
Special Issue
Copyright
Data & Comments
Data
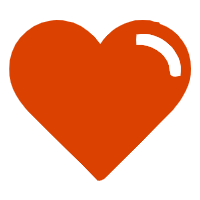
Comments
Comments must be written in English. Spam, offensive content, impersonation, and private information will not be permitted. If any comment is reported and identified as inappropriate content by OAE staff, the comment will be removed without notice. If you have any queries or need any help, please contact us at support@oaepublish.com.