Pollution status, distribution, source analysis, and risk assessment of OCPs in soil from the Hexi Corridor in Northwest China
Abstract
Since OCPs with different historical usage and atmospheric input may show varied environmental behaviors and risks to the local residents and relevant research on underdeveloped areas in the middle latitudes of China is lacking, it is essential to classify the contamination status and sources of OCPs from these middle latitudes areas and to evaluate the related health risks to humans. Fifty soil samples were collected and analyzed within five cities in the Hexi Corridor in Northwest China. The ranges of ∑24OCPs, ∑DDTs, and ∑HCHs concentrations in the soil samples were 23.1-393 ng/g, 4.96-167 ng/g, and 3.40-97.5 ng/g, respectively. The residual OCPs in soil were dominated by DDTs and HCHs, accounting for 38.7% and 16.1% of ∑24OCPs. Source analysis shows that the HCHs come from historical application and possible recent pesticide use, and DDTs are mainly from early application residues that formed in aerobic environments created by agriculture ploughing, which aerates the soil. The risk assessment showed that the soil in the Hexi Corridor may have a potential risk of residual OCPs, and the carcinogenic risk (CR) was 1.90 × 10-7-6.12 × 10-7 for adults and 5.6 × 10-7-1.8 × 10-6 for children, but the hazardous index (HI) was 0.0093 to 0.2817 for adults and 0.032 to 0.932 for children. Only a few samples showed values higher than the acceptable range for children. Therefore, in this study area, there is a low health risk to local residents. Nevertheless, our results provide a strong rationale for ongoing risk assessment and management and, hopefully, eradication of OCPs in the environment.
Keywords
INTRODUCTION
Organochlorine pesticides (OCPs) are mostly derivatives of chlorine and contain one or more benzene rings. These pesticides are semi-volatile and have high toxicity, environmental persistence, bioaccumulation, and long-range migration[1]. They are also considered to be typical persistent organic pollutants (POPs)[2-4]. OCPs accumulate through the enrichment and amplification effects that happen in food chains, and eventually enter the human body, endangering human health. As a big cultivated country, China has been a major producer and consumer of OCPs, but it has gradually banned OCP usage since 1983[5-7]. However, studies have found that the residual concentrations of OCPs are still at a high level in areas where OCPs were once widely used. Soil is an important reservoir of OCPs. The half-life of OCPs in soil lasts for several years to several decades, and they can be adsorbed into soil organic matter and enter food chains, resulting in biological accumulation and enrichment[8]. Simultaneously, the contaminated soil is also part of the global cycle of OCPs. From soil, they can be transferred to the atmosphere and bodies of water through volatile diffusion, forming secondary pollution[9,10]. Due to their stable chemical structure, higher toxicity, and resistance to degradation, the environmental issues of OCPs in different regions and countries have become a global concern[7,8,11-14].
At present, the studies on OCP contamination have been mainly concentrated in the economically developed provinces and regions of China (e.g., the southeastern coast of China)[15,16], and the plateau areas such as the Tibetan Plateau of China[17,18]. However, there are few studies that have examined the northwest of China[19,20]. The Hexi Corridor, in western Gansu Province, is an important grain-producing area located in a key part of the Silk Road Economic Belt[21]; it provides more than 2/3 of the commercial grain and cash crops for Gansu Province. The area is remote from the sea and deep in the hinterland of Eurasia. OCPs were widely applied in this area before they were banned[22]. Geographically, the Hexi Corridor is a typical semiarid area with a unique valley and basin terrain and complex meteorological conditions. Data show that before the prohibition of OCPs, the proportion of HCHs and DDTs in the region accounted for more than 50% of the total pesticide use[23]. In summary, the Hexi Corridor is unique from other areas that have been studied regarding OCPs because of its unique climate, topography, and historical application of OCPs and because the source and input of OCPs in this middle-high latitude area are expected to be different from the developed regions and plateau areas. Therefore, the data on pollution levels and geographical spread of OCPs obtained through this study can offer valuable insights to policymakers, allowing them to implement suitable measures for mitigating potential ecological and health hazards.
MATERIAL AND METHODS
Study area and sample collection
The study region (92°13′-108°46′ E and 32°31′-42°57′ N) was represented by five main cities. Fifty soil samples were collected in November 2018 from Jiuquan (A1-A10), Jiayuguan (B1-B10),
Chemicals and reagents
OCPs mixed standards were purchased from AccuStandard and included 24 individual compounds: α-HCH, β-HCH, δ-HCH, γ-HCH, p,p'-DDD, p,p'-DDT, p,p'-DDE, o,p'-DDT, dicofol (DIC), HCB, heptachlor (HE), heptachlor epoxide (HPE), cis-chlordane (cis-CD), trans-chlordane (trans-CD), α-endosulfan (α-ED), β-endosulfan (β-ED), endosulfan sulfate (EDS), aldrin (AD), dieldrin endrin (DE), endrin aldehyde (EDA), endrin ketone (EDK), mirex (ME), and methoxychlor (MEC). The 100-200 mesh silica gel was purchased from Qingdao Haiyang Chemical Co. (Shandong, China). It was activated by heating it at 130 °C for 16 h, then deactivated by adding purified water (3.3%), and stored in a desiccator. To use, anhydrous Na2SO4 was baked at 600 °C for 6 hours. All solvents used were of HPLC grade (Fisher Chemical Company, USA) and all glassware was thoroughly cleaned with detergent, K2Cr2O7-H2SO4 solution, running water, deionized water, and acetone. It was then baked at 400 °C for 3.5 h before reusing.
Sample pretreatment and instrumental analysis
Our team followed the procedure detailed in our previous report to evaluate the target OCPs[25]. First, 10 g soil samples were weighed for each group and mixed with 10 g of anhydrous Na2SO4. Next, we spiked this mixture with a surrogate (4-Chloro-m-xylene with a concentration of 2.5 × 10-4μg/g) and used a low-temperature ultrasonic extractor to extract it with a 200-mL combination of hexane/acetone (1:1 v/v). The resulting extracts were concentrated, and their solvent was exchanged to hexane. We utilized a silica gel column of dimensions 25 cm × 1 cm I.D. containing 6 g of deactivated silica gel topped with 2 cm of anhydrous sodium sulfate. To prepare the column, we first eluted it with 40 mL of hexane before injecting the extract. Then, we proceeded by eluting the column with 80 mL of n-hexane, followed by 35 mL of
Quality assurance
Table 1 presents the limits of detection (LOD), analysts' recoveries, and relative standard deviations (RSD) obtained by testing natural soil spiked with individual OCP standards (n = 5) to assess the recovery efficiency of the procedure. To ensure proper procedural performance and monitor matrix effects, different blank and triplicate samples were evaluated for each of the five samples, with additional surrogates added to each sample. Soil samples spiked with surrogates showed recoveries ranging from 63.4% to 92.0%, while the OCP variabilities in triplicate samples were no more than 10%. The results were presented on a dry weight basis, and were not adjusted for recoveries.
Statistics results and the detected limit, recovery and RSD of the method of OCPs in soil (n = 50, ng/g)
Analysts | LOD | Recovery | RSD | Min | Max | Mean | SD | DF | RV |
α- HCH | 0.016 | 94.15 | 6.72 | 0.121 | 14.4 | 1.71 | 2.64 | 100 | 2.5 |
β- HCH | 0.019 | 84.39 | 8.79 | 0.303 | 80.1 | 6.20 | 12.38 | 100 | 1.0 |
γ- HCH | 0.027 | 89.58 | 5.36 | 0.791 | 35.0 | 4.33 | 5.25 | 100 | 0.5 |
δ-HCH | 0.015 | 86.21 | 8.13 | 0.164 | 16.5 | 3.79 | 2.92 | 100 | - |
ΣHCHs | - | - | - | 3.40 | 97.5 | 16.1 | 13.5 | 100 | 10 |
p,p′-DDD | 0.007 | 91.43 | 6.66 | 1.09 | 37.6 | 5.98 | 5.43 | 100 | - |
p,p′-DDE | 0.005 | 82.37 | 7.32 | 1.54 | 40.7 | 8.83 | 6.76 | 100 | - |
p,p′-DDT | 0.010 | 89.89 | 7.98 | 1.35 | 93.6 | 18.3 | 15.7 | 100 | - |
o,p′-DDT | 0.012 | 81.32 | 5.32 | 0.262 | 14.6 | 2.62 | 2.64 | 100 | - |
ΣDDTs | - | - | - | 4.96 | 167 | 35.8 | 47.5 | 100 | 2.5 |
HCB | 0.005 | 92.35 | 4.74 | 0.391 | 83.9 | 4.28 | 12.0 | 100 | 2.5 |
Nonachlor | 0.035 | 71.47 | 9.80 | 1.03 | 35.0 | 6.27 | 8.24 | 100 | - |
Heptachlor (HE) | 0.025 | 84.36 | 4.38 | 0.113 | 4.92 | 1.05 | 1.12 | 100 | - |
Heptachlor epoxide (HPE) | 0.015 | 94.32 | 4.58 | 0.293 | 21.1 | 3.30 | 3.38 | 100 | - |
trans-Nonachlor | 0.020 | 68.87 | 7.82 | 0.181 | 8.19 | 1.46 | 1.68 | 100 | - |
cis-Chlordane (cis-CD) | 0.020 | 78.34 | 6.68 | nd | 6.41 | 1.43 | 1.33 | 98.0 | 2.5 |
trans-Chlordane (trans-CD) | 0.015 | 78.80 | 5.54 | nd | 8.35 | 1.97 | 1.50 | 98.0 | 2.5 |
ΣCHLs | - | - | - | 0.270 | 8.16 | 2.58 | 1.01 | 100 | |
Endrin aldehyde (EDA) | 0.030 | 81.34 | 6.25 | 0.924 | 35.2 | 4.18 | 5.77 | 100 | - |
Endrin ketone (EDK) | 0.032 | 78.18 | 8.17 | 0.351 | 11.7 | 2.33 | 2.07 | 100 | - |
α-endosulfan (α-ED) | 0.016 | 69.27 | 6.15 | 0.362 | 9.83 | 2.01 | 2.29 | 100 | - |
β-endosulfan (β-ED) | 0.018 | 89.54 | 6.42 | nd | 19.63 | 2.74 | 3.16 | 96.0 | - |
Endosulfan sulfate (EDS) | 0.025 | 85.25 | 7.47 | nd | 1.60 | 0.34 | 0.31 | 98.0 | - |
Endrin (ED) | 0.030 | 75.54 | 9.88 | nd | 9.48 | 2.25 | 1.80 | 92.0 | 1.0 |
Aldrin (AD) | 0.046 | 78.84 | 5.35 | 0.293 | 14.9 | 2.35 | 2.40 | 100 | - |
Diedrin endrin (DE) | 0.036 | 72.45 | 9.78 | 0.222 | 17.2 | 2.03 | 2.47 | 100 | - |
Methoxychlor (MEC) | 0.025 | 78.84 | 6.88 | nd | 17.59 | 4.86 | 3.73 | 96.0 | - |
Σ24OCPs | - | - | - | 23.1 | 393 | 94.7 | 45.3 | 100 | - |
RESULTS AND DISCUSSION
Pollution characteristics and residual levels of OCPs
In this paper, we collected 50 soil samples from five cities located in the Hexi Corridor and determined the concentrations for 24 individual OCPs. The statistical results of OCPs in the soils are shown in Table 1, and the residual contents of OCPs in the soil of five cities are shown in [Supplementary Table 1]. From Table 1, except for a few sampling locations for individual OCPs, such as EDS, EDA, EDK, ME, and MEC, other individual OCPs were detected in the soil at all sampling locations, which indicated that the OCP contamination in the Hexi Corridor was diverse and ubiquitous. Based on historical reports, the usage of DDTs and HCHs was 15,589 tons and 64,991 tons, respectively, from the 1950s to the 1980s in Gansu Province[26]. As may be expected, the entire study location, as a main grain and vegetable production location, has relatively widespread OCP residues, mostly likely due to historical usage as well as recent input in the study areas. The contents of ∑24OCPs ranged from 23.1-393 ng/g (average: 94.7 ng/g), the ∑DDTs contents ranged from 4.96-167 ng/g, and the ∑HCHs contents were in a range of 3.40-97.5 ng/g. The sum of AD, DE, EDA, and EDK (∑Drins) was in a range of 4.04-64.3 ng/g, accounting for 12.6% of ∑24OCPs. The total concentration of cis-CD and trans-CD (ΣCHLs) ranged from 0.740-24.1 ng/g. The order of the dominant individual OCPs in the Hexi Corridor was as follows: DDTs (average: 35.8 ng/g) > HCHs (average: 16.0 ng/g) > ∑Drins (average: 11.6 ng/g) > Nonachlor (average: 6.27 ng/g) > Methoxychlor (average: 4.86 ng/g) > CHLs (average: 4.32 ng/g) > HCB (average: 4.28 ng/g) > Endrin aldehyde
There are many studies on OCP contamination in soil from China and worldwide [Table 2]. The ∑OCPs levels in urban soils vary considerably, ranging from 7.6 to 37,331 ng/g with a median value of 53.7 ng/g. The average content of OCPs is 2,861 ng/g, which is several orders of magnitude higher than the values (58.9 ng/g) reported for agricultural soils in China[12]. Compared with other regions and countries in the world, the content of ∑HCHs in this study was lower than that of Kalashah Kaku, Pakistan[28], Spain[29], and Tianjin[30] in China. ∑HCHs were comparable to the results from Urumqi[31], Taiyuan[32], and Fujian Province[8] in China. But ∑HCHs were higher than those reported for Hong Kong[33], Beijing[34], Guangzhou[22], and Shanghai[25]; seven cities in India[35]; Punjab Province of Pakistan[36]; Canada; and the UK[37]. The content of ∑DDTs in soil was lower than that in Romanian soil[38,39], Germany[40], Beijing[34] and Guangzhou[22] in China; but higher than that reported for the Pearl River Delta[41], Urumqi[31] and Hong Kong[33] in China as well as that reported for India[42], Nepal[43], Italian[13,14], and Poland[44]. In general, compared with other areas in China and worldwide, the contents of HCHs and DDTs in the soil in the Hexi Corridor fell in the upper middle level. The study area is located in the temperate continental climate, which is characterized by arid and semiarid areas with less wind and rain, and fewer microorganisms in soil, resulting in a slower degradation of HCHs and DDTs.
Distribution of DDTs and HCHs (ng/g) in soils of different regions
Location | Sample time | ∑HCH (ng/g) | ∑DDT (ng/g) | References |
Kalashah Kaku, Pakistan | 2008 | 25-122 | - | [28] |
Spain | 2003-2004 | 200 | - | [29] |
Tianjin | 2008 | 77.67 | - | [30] |
Urumqi | - | nd-30.86 ng/g | nd-40.03 | [31] |
Taiyuan | 2006 | 5.24-97.81 | - | [32] |
Fujian Province | - | 0.97-247 | - | [8] |
Hong Kong | - | 2.5-11 | nd-5.7 | [33] |
Beijing | 2007 | 0.64-32.32ng/g | 1.42-5,910.80 | [34] |
Guangzhou | - | nd-17.79 ng/g | 3.58-831 | [22] |
Shanghai | 2007 | nd-10.38 ng/g | - | [25] |
seven cities in India | - | 0.01-60 | - | [35] |
Punjab Province of Pakistan | 2014 | 1.7-20 | - | [36] |
UK | - | < 0.01-0.07 | - | [37] |
rural soil of Romania | 2009 | - | 226.9 ± 157.2 | [38-39] |
urban soil of Romania | 2009 | - | 113.1 ± 151.8 | [38-39] |
Germany | 1999 | - | 23.7-173 | [40] |
the Pearl River Delta | 2006 | - | 0.27-414 | [41] |
Hexi Corridor | 2019 | 3.40-97.48 | 4.96-166.97 | This study |
Spatial distribution of OCPs
Figure 2 and [Supplementary Table 1] show the distribution characteristics and spatial distribution of OCPs in the soils from the study area. OCPs are normally associated with agricultural application contamination[25], which may explain the differences in OCP levels obtained among the five cities [Supplementary Table 1]. From [Supplementary Table 1], Jiuquan is known to have a long and intensive agricultural and pesticide production history, and therefore the highest average level with a mean of 179 ng/g
Figure 2. Spatial distribution of (A) for ∑24OCPs; (B) for ∑HCHs; and (C) for ∑DDTs in soil from Hexi Corridor in Northwest China.
The contents of p,p'- DDT and p,p'-DDD were the highest among detected individual OCPs, which were 1.35-93.6 ng/g and 1.54-40.7 ng/g with a mean of 18.3 ng/g and 8.33 ng/g, respectively. As can be seen from Figure 2, the highest contents of DDTs appear at sampling locations A3, A4, and A7 (Jiuquan City). The order of content of ∑24OCPs in the soils of five cities from highest to lowest in Hexi was Jiuquan > Jinchang > Jiayuguan > Wuwei > Zhangye. The order of ∑HCHs residual content was Jiuquan > Wuwei > Jinchang > Jiayuguan > Zhangye. The order of ∑DDTs residual content was Jiuquan > Jinchang > Jiayuguan > Wuwei > Zhangye. Most of the sampling locations in Jiuquan were near cultivated land and farmland. Previous research data indicates that before the prohibition of OCPs, the proportion of HCHs and DDTs in the region accounted for more than 50% of the total pesticide use[23]. Therefore, there were high residual amounts of HCHs and DDTs. In addition, Jiuquan is a city where industry and agriculture jointly develop, while Zhangye is an agricultural city. Therefore, the sources of OCPs in Jiuquan soil are more extensive than those in Zhangye. Thus, the residual OCPs in the soil of Jiuquan are higher compared to other cities.
The composition of OCPs
Composition characteristics of HCHs
Figure 3A displays the HCH composition present in the soil of the Hexi Corridor. All four HCH isomers (α, β, γ, and δ) were detected with a 100% detection rate, and the total residual concentration of HCHs ranged from 3.40 ng/g to 97.5 ng/g, with an average of 16.0 ng/g. The residual concentration of individual isomers was as follows from highest to lowest: δ-HCH (6.20 ng/g) > β-HCH (4.33 ng/g) > γ-HCH (3.79 ng/g) >
Composition patterns of the DDTs
The compositions of four isomers of DDTs are presented in Figure 3B. The detection frequencies of individual isomers of DDT in the soil was 100%, and the total contents of DDTs in the soil were 4.96-167 ng/g with a mean of 35.8 ng/g. The amounts of p,p'- DDT, p,p' - DDD, and p,p'- DDE accounted for 48.67%, 26.56%, and 17.38%, respectively, while o,p'- DDT accounted for only 7.40% of the ∑DDTs [Figure 3B]. The lowest content of DDT was o,p′-DDT, with a mean of 2.62 ng/g. Judging from Figure 3B, the DDT contamination in the study area was mainly DDT and DDD, indicating that the soil DDTs in the Hexi Corridor were mainly derived from early pesticide residues. This may be due to the fact that the study area is arid and semiarid, and the annual average temperature is low, so DDT had the highest contribution to the residual amount of OCPs.
Composition patterns of the other OCPs
The high levels of HCB originate from industrial and agricultural applications, as well as from the incomplete burning of waste, fossil fuels, and biomass[45]. Additionally, HCB is capable of getting released into the surroundings when different production procedures linked to the byproducts of chlorinated solvents, aromatics, and pesticides occur. The historical production of HCB was 3,522 tons/year until it was banned in 2004 in China[16]. HCB was found to be prevalent in all locations that were studied, with an average of 4.28 ng/g (ranging from 0.389-83.9 ng/g) [Table 1]. The higher levels of HCB were distributed in Jiuquan and Wuwei. The soil samples indicated the presence of the highest concentration of HCB at sites A8, A3, and A4 in Jiuquan and at E3, E4, E6, and E7 in Wuwei. ED comprised 3.1-9.7% of Σ24OCPs in the collected samples [Figure 2]. The higher levels of cis- and trans-ED were found in Jiuquan in the range of 0.79-7.23 ng/g (mean 2.85 ng/g). The contents of cis- and trans-ED detected in Jiayuguan, Jinchang, and Wuwei were similar--0.36-2.27 ng/g, 0.41-2.57 ng/g, and 0.17-3.15 ng/g, respectively [Supplementary Table 1]. The highest concentrations of EDs were detected at A3, A1, and A7 in Jiuquan [Supplementary Figure 1]. Industrial ED is still applied in cotton and other crops in China. As such, it is found in more than 90% of agricultural soils in China and is the most chemically rich OCP. This is consistent with the fact that ED is currently being utilized in China and that extensive cotton cultivation exists around the study area in the upwind areas, causing it to accumulate in soils over time. The historical production of chlordane was 363 tons/year[16]. Chlordane was not widely applied in agricultural practice and was banned in China in 2009. Technical chlordane is dominated by TC (13%), CC (11%), HEPT (5%), and trans-nonachlor (5%)[15]. Chlordane chemicals were highly detected OCPs and accounted for 4.31%-11.7% of ∑24OCPs [Figure 2]. The relative abundance of chlordane was found in all study sites and showed a stable concentration irrespective of sampling sites [Supplementary Figure 1 and Supplementary Table 2]. The CC and TC concentrations were in the range of 1.52-1.62 ng/g and 1.50-1.69 ng/g, respectively [Table 1]. Higher levels of CC and TC were detected at A2, A3, and A4 in Jiuquan, and at E3, E4, and E7 in Wuwei [Supplementary Figure 1]. Despite being banned in China for years, the higher detection of Chlordane in many areas suggests that its presence in soil is a result of secondary distribution and specific sources.
The relationship between total organic carbon and OCPs
TOC in soil has the ability to adsorb OCPs due to its hydrophobicity properties. Furthermore, TOC provides a sufficient amount of carbon to reduce the levels of OCPs through bio-degradation[46,47]. Accordingly, TOC can affect the behavior and risk of OCPs in soil. In the present study, TOC in soil was in a range of 4.43-28.4 g/kg with a mean of 11.9 g/kg. To get insights into the role of TOC, the contents of Σ24OCPs, ΣHCHs, and ΣDDTs were plotted with TOC concentration using a scatter plot [Supplementary Figure 2]. The results showed that the OCPs were positively correlated with TOC concentrations in soil (Σ24OCPs with R2 = 0.609, ΣDDTs with R2 = 0.671, and ΣHCHs with R2 = 0.409 at P < 0.05 [Supplementary Figure 2]. This suggests TOC in soil plays a crucial role in adsorbing OCPs[25,48]. In an equilibrated soil-air system, there is a proportional relationship between the residual OCP concentrations and the soil organic matter (SOM)[49]. However, if the system is imbalanced or if other complex soil conditions exist, deficient correlations may result[50]. In a previous report, we also noticed a weak correlation between TOC and OCPs[25]. Nevertheless, our current study has found a stable linear correlation between OCPs and TOC, which agrees with prior investigations in Central Europe[17] and the Central Tibetan Plateau[51].
Source apportionment of OCPs in soil
The proportion of parent compound to its metabolites can offer valuable insights into the origin of contamination[52]. The technical HCH compound typically contains around 60%-70% α-HCH, 5%-12% β-HCH, 10%-12% γ-HCH, and 6%-10% δ-HCH (UNEP). In addition, lindane (> 99% β-HCH) was also used, despite the ban on technical HCH[53]. β-HCH is the most easily absorbed by SOM and less likely to evaporate compared to other isomers. Meanwhile, α-HCH and γ-HCH can transform into β-HCH in soil, and the molecules' chlorine atoms in β-HCH resist degradation from microorganisms in soil, causing its accumulation. This study employed the ratios of α-HCH/γ-HCH and β-HCH/(α-HCH+γ-HCH) to trace the source of HCHs. Ratios of α-HCH/γ-HCH greater than 3 but less than 7 indicate technical HCH contamination, whereas a ratio less than 3 suggests new lindane input[54]. For the β-HCH/(α-HCH + γ-HCH) ratio, values over 0.5 denote historical contamination, while lower values indicate pollution from recent pesticide use and atmospheric deposition. Results reveal the presence of HCH contamination in all sampling sites, with low ratios of α-HCH/γ-HCH, indicating early-stage lindane input. The β-HCH/(α-HCH+γ-HCH) ratios are in a range of 0.12-3.57, with 70% of sampling locations > 0.5 [Figure 4A], signifying historical application, mainly in the Zhangye region, indicating contamination of the Hexi corridor with HCHs from past sources and possible new contamination.
Studies indicate that DDT is a commonly used insecticide, with p,p'-DDE and p,p'-DDD being the primary products resulting from the dechlorination of p,p'-DDT[55]. The ratio of p,p'-DDT to (p,p'-DDE + p,p'-DDD) is utilized to determine whether the input of DDT in the environment is increasing or decreasing. A low ratio of p,p'-DDT/(p,p'-DDE + p,p'-DDD) suggests historical DDT use, while a value > 2.0 denotes recent application[56]. As observed in Figure 4B, the majority of values of p,p'-DDT/(p,p'-DDE + p,p'-DDD) were < 2.0 (ranging from 0.204 to 3.70), indicating that DDT in the soil of the sample points primarily originated from the past and had been bio-converted to DDD and DDE. Additionally, a DDD/DDE ratio of less than 1 was observed in 24% of sampling sites, suggesting that DDT had undergone aerobic bio-degradation, influenced by the environment's oxygen content and soil porosity. Notably, the technical endosulfan accounted for 70% and 30% of α and β-ED, correspondingly, with a ratio of almost 2.33[25]. A ratio of
Pollution and risk assessment
Pollution assessment
The soil quality in this study was assessed according to the national standards of China (GB15618-1995), which classifies soil into three levels based on the residual concentrations of ΣDDTs and ΣHCHs. The first level is less than 50 ng/g, the second level ranges from 50-500 ng/g, and the third level ranges from 500-1,000 ng/g. Out of the 43 soil samples collected, the ΣHCHs content was below the first-level guideline. Additionally, out of the 50 total soil samples, only two collected from A3 and A7 were in the second-level category for ΣDDTs, while none of the samples exceeded the third level. Compared to the soil protection guidelines of the Netherlands [Table 1], the levels of ΣHCHs in all collected soil samples were higher than the target value for uncontaminated soil, but the concentrations of β-HCH and γ-HCH were lower than the target value for unpolluted soil. Similarly, the levels of ΣDDTs were higher than the target value of uncontaminated soil. The target values of HCB, endrin, and CHLs in the soil protection guideline were higher than corresponding OCP levels in some of the collected soil samples. Overall, the HCH pollution of the soil in the Hexi Corridor may be categorized as existing, while the level of DDTs was considered low pollution. HCB, endrin, and CHLs were classified as low contamination for half of the soil samples, with very few samples considered non-contaminated. Although no remediation strategies were deemed necessary for soil OCP pollution, further research is necessary on the adverse ecological and health effects of OCPs' endocrine-disruptive properties, which may lead to biological magnifications in the food chain, including human beings.
Ecotoxicological risk assessment for OCPs
The study evaluates the risk of OCP pollution to soil biota in the Hexi Corridor in Northwestern China. To accomplish this, the Sediment Quality Guidelines (SQG) were used to assess the potential ecological risk of OCPs in the soil[60]. These guidelines utilize two sets of values: the Effect Range Low (ERL) and the Effect Range Median (ERM). The values of ERL, ERM, Threshold Effect Level (TEL), and Probable Effect Level (PEL) for different OCP compounds were analyzed, and the findings were listed in Table 3. The results indicated that some elements did not exceed ERL, but a considerable number of soil samples had a higher concentration of p,p'-DDT, p,p'-DDE, HCH, and endrin as compared to ERL. 50% of soil samples for
Bio-toxicity risk assessment of OCPs in surface soil of Hexi Corridor
OCPs | ERL | ERM | Range | Sample number ratio /% | ||
< ERL | ERM-ERL | > ERM | ||||
p,p'-DDE | 2.20 | 27.00 | 1.09-37.58 | 16% | 82% | 2% |
p,p'-DDD | 2.00 | 20.00 | 1.54-40.71 | 6% | 88% | 6% |
p,p'-DDT | 1.00 | 7.00 | 1.35-93.58 | 0 | 18% | 82% |
∑DDT | 1.58 | 46.1 | 4.96-166.97 | 0 | 84% | 16% |
∑HCH | 0.02 | 8.00 | 3.40-97.48 | 0 | 26% | 74% |
Endrin | 1.58 | 46.10 | 0.36-9.83 | 60% | 40% | 0 |
Health risk assessment
Exposure risk assessment of OCPs in soils was conducted by the USEPA method[61]. The non-carcinogenic and carcinogenic risks for children and adults under three exposure pathways (ingestion, dermal contact, and inhalation) were estimated and established upon Equations (1)-(5). The applied equations of OCP exposure in different exposure pathways were presented as follows:
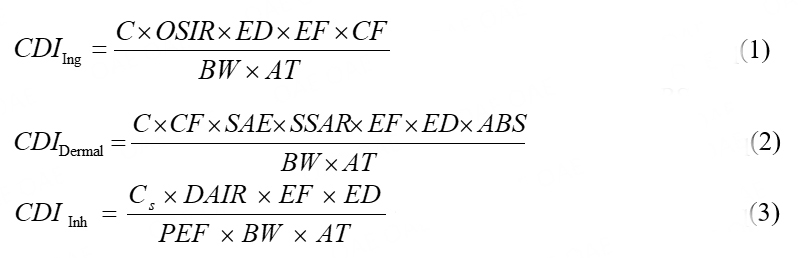
where C is the concentration of OCPs, CDIIng is ingestion exposure, OSIR is daily soil intake, ED is exposure years, EF is the rate of exposure, CF is conversion factor, BW is average weight, AT is average contact time, SAE is skin area touching the soil, SSAR is soil-skin adhesion coefficient, ABS is absorption factor, DAIR is daily air volume, PEF is formation coefficient of soil dust, CDIDermal refers to dermal contact exposure, and CDIInh is inhalation exposure. The exposure risk is divided into the carcinogenic risk (CR) and the non-carcinogenic effect (HI).
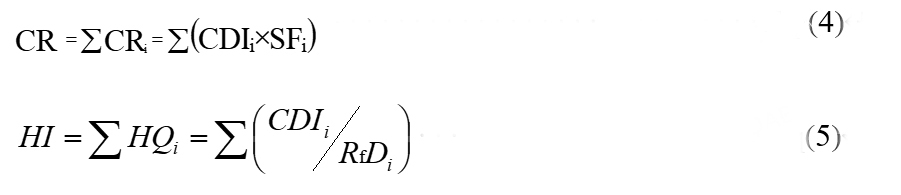
where CR is the composite index of carcinogenic risk, CRi represents the three exposure routes, HI is the composite non-carcinogenic risk index, HQi expresses the non-carcinogenic risk of an exposure path, CDIi is the soil exposure amount under a certain exposure path, RfDi is the reference dose of a non-carcinogen for an exposure route. The related parameter used in the Eq(1)-Eq(5) is presented in Supplementary Table 2 and Supplementary Table 3.
The CR (HCHs, DDTs, HCB, aldrin, and chlordane) and the HI (HCHs, DDTs, HCB, aldrin, CDs, and EDs) were evaluated in the soil of the Hexi Corridor [Supplementary Table 4]. Generally, CR < 1.0 × 10-6 is an acceptable risk level, while CR ranging from 1.0 × 10-6 to 1.0 × 10-4 indicates a medium cancer risk to humans. CR above 1.0 × 10-4 is considered to be a relatively high cancer risk[14]. Meanwhile, HI > 1 indicates a higher health damage risk, while < 1 indicates a low health risk to humans. Based on the calculated results, the total CR fluctuated in a range of 1.86 × 10-7 to 5.62 × 10−6 for children and 1.21 × 10-7 to 1.91 × 10-6 for adults. Moreover, for the three exposure pathways, the CR through ingestion was higher or equal to the risk through dermal contact, but two or three orders of magnitude higher than the risk through inhalation. The order of the means of carcinogenic risk of OCPs in soil was Jiuquan (1.80 × 10-6 for children, 6.12 × 10-7 for adults) > Jinchang (7.60 × 10-7 for children, 2.58 × 10-7 for adults) > Jiayuguan (7.26 × 10-7 for children, 2.46 × 10-7 for adults) > Wuwei (7.31 × 10-7 for children, 2.48×10−7 for adults) > Zhangye (5.60 × 10-7 for children, 1.90 × 10-7 for adults) [Supplementary Table 4]. According to the CR index [Supplementary Table 4], the CR index of Jiuquan is higher than that for other regions, and the carcinogenic risk of OCPs in the soil was higher for children than for adults. HI of OCPs for children and adults ranged from 0.032 to 0.932 (average: 0.458) and 0.093 to 0.281 (average: 0.155), respectively, and all of these were < 1, indicating a low health risk. For HI, HCHs contributed the most, accounting for more than 50% on average, followed by DDT, and CHL [Figure 5]. In summary, fortunately, the levels of OCPs in very few of the collected soil samples have certain carcinogenic and other health risks to humans; however, these should receive further research and management attention. According to the risk index of different exposure routes, the main exposure pathway was ingestion, followed by dermal contact and inhalation. These findings align with prior research indicating that children are a demographic that is more susceptible to the adverse health effects of these pollutants[8,19].
CONCLUSIONS
The results exhibited the content of ∑24OCPs ranged from 23.1 to 393 ng/g, with a mean of 94.7 ng/g. The order of the dominant OCPs in the Hexi Corridor soil was as follows: ∑DDTs > ∑HCHs > Heptachlor > HCB > Chlordane > Endosulfan. HCHs and DDTs were much higher than other OCPs, accounting for 38.7% and 16.1% of ∑24OCPs, respectively. The order of content of ∑24OCPs (from high to low) in the Hexi Corridor was Jiuquan > Jinchang > Jiayuguan > Wuwei > Zhangye. Source analysis showed that there was recent pesticide use in these areas, and the higher levels of OCPs and unused OCPs suggest that a certain amount of OCPs were, in fact, recently input into the study areas. The results indicated that there was a certain ecological risk of OCP contamination in the soil of the Hexi Corridor, which may cause harm to the ecological environment. The results showed that the main health risk of OCPs in the different areas of the Hexi Corridor was ingestion, followed by dermal contact and oral inhalation, and OCPs in the soil in Jiuquan, in particular, pose carcinogenic and other health risks to humans, demanding vigilant attention.
The present results provide contamination information and a strong rationale for taking measures to control these pollutants in the contaminated sites in order to further guarantee the health of the residents living around the study areas. Future studies should focus on these unique high-contamination sites in the middle latitudes and the input and output sources of the pollutants in the cities in this area.
DECLARATIONS
Acknowledgments
This research was jointly supported by the National Natural Science Foundation of China (21966020).
Authors’ contributions
Writing - original Manuscript Preparation: He R
Experiment, data analysis: Yuan L
Writing - manuscript preparation, reviewing and editing, funding acquisition: Jiang Y
Software, validation analysis: Jia Z
Soil sampling and treatment: Ding W
Instrumental analysis, reviewing and editing: Yang Z
Availability of data and materials
Data published as supplementary information in the journal.
Financial support and sponsorship
This work was funded by the National Nature Science Foundation of China (21966020).
Conflicts of interest
All authors declared that there are no conflicts of interest.
Ethical approval and consent to participate
Not applicable.
Consent for publication
Not applicable.
Copyright
© The Author(s) 2023.
Supplementary Materials
REFERENCES
1. Buccina J. The development of a global treaty on persistent organic pollutants. persistent organic pollutants; 2003: 123-201.
2. UNEP. Dralt revised guidance on the global monitoring plan for persistent organic pollutants, UNEP/POPS/COP5/INF/27. Available from: https://www.informea.org/zh-hans/draft-revised-guidance-global-monitoring-plan-persistent-organic-pollutants [Last accessed on 28 Aug 2023].
3. UNEP. Final act of the conference of plenipotentiaries on the Stockholm Convention on persistent organic pollutant. Available from: https://www.scirp.org/reference/ReferencesPapers.aspx?ReferenceID=1726464 [Last accessed on 28 Aug 2023].
4. UNEP. Report of the Conference of the Parties of the Stockholm Convention on Persistent Organic Pollutants on the work of its fourth meeting. Available from: http://chm.pops.int/portals/0/repository/cop4/unep-pops-cop.4-38.english.pdf [Last accessed on 28 Aug 2023].
5. Zhang J, Wang X, Gong P, Wang C, Sun D. Seasonal variation and source analysis of persistent organic pollutants in the atmosphere over the western Tibetan Plateau. Environ Sci Pollut Res Int 2018;25:24052-63.
6. Lv M, Luan X, Guo X, et al. A national-scale characterization of organochlorine pesticides (OCPs) in intertidal sediment of China: occurrence, fate and influential factors. Environ Pollut 2020;257:113634.
7. Yu H, Liu Y, Shu X, Ma L, Pan Y. Assessment of the spatial distribution of organochlorine pesticides (OCPs) and polychlorinated biphenyls (PCBs) in urban soil of China. Chemosphere 2020;243:125392.
8. Zhang Y, Zhang H, Shi XM. Risk assessment of reuse of site contaminated by organic chemicals after soil remediation. Bull Soil Water Conserv ;30:203-7.
9. Luo Y, Yang R, Li Y, et al. Accumulation and fate processes of organochlorine pesticides (OCPs) in soil profiles in Mt. Shergyla, Tibetan Plateau: a comparison on different forest types. Chemosphere 2019;231:571-8.
10. Ding Y, Huang H, Chen W, et al. Background levels of OCPs, PCBs, and PAHs in soils from the eastern Pamirs, China, an alpine region influenced by westerly atmospheric transport. J Environ Sci 2022;115:453-64.
11. Ali U, Syed JH, Malik RN, et al. Organochlorine pesticides (OCPs) in South Asian region: a review. Sci Total Environ 2014;476-477:705-17.
12. Niu L, Xu C, Zhu S, Liu W. Residue patterns of currently, historically and never-used organochlorine pesticides in agricultural soils across China and associated health risks. Environ Pollut 2016;219:315-22.
13. Qu C, Albanese S, Li J, et al. Organochlorine pesticides in the soils from Benevento provincial territory, southern Italy: spatial distribution, air-soil exchange, and implications for environmental health. Sci Total Environ 2019;674:159-70.
14. Qu C, Albanese S, Lima A, et al. The occurrence of OCPs, PCBs, and PAHs in the soil, air, and bulk deposition of the Naples metropolitan area, southern Italy: implications for sources and environmental processes. Environ Int 2019;124:89-97.
15. Zhang A, Fang L, Wang J, et al. Residues of currently and never used organochlorine pesticides in agricultural soils from Zhejiang Province, China. J Agric Food Chem 2012;60:2982-8.
16. Fang Y, Nie Z, Die Q, et al. Organochlorine pesticides in soil, air, and vegetation at and around a contaminated site in southwestern China: Concentration, transmission, and risk evaluation. Chemosphere 2017;178:340-9.
17. Yuan GL, Qin JX, Li J, Lang XX, Wang GH. Persistent organic pollutants in soil near the Changwengluozha glacier of the Central Tibetan Plateau, China: their sorption to clays and implication. Sci Total Environ 2014;472:309-15.
18. Gai N, Pan J, Tang H, et al. Organochlorine pesticides and polychlorinated biphenyls in surface soils from Ruoergai high altitude prairie, east edge of Qinghai-Tibet Plateau. Sci Total Environ 2014;478:90-7.
19. Huang T, Guo Q, Tian H, et al. Assessing spatial distribution, sources, and human health risk of organochlorine pesticide residues in the soils of arid and semiarid areas of northwest China. Environ Sci Pollut Res Int 2014;21:6124-35.
20. Mao X, Yu Z, Ding Z, et al. Sources and potential health risk of gas phase PAHs in Hexi Corridor, Northwest China. Environ Sci Pollut Res Int 2016;23:2603-12.
21. Jia Z, Yuan L, Jiang Y, He R, Ding W. Status, distribution, source, and risk of polychlorinated biphenyl levels in soils of five cities from the Hexi Corridor, Northwest China. Environ Monit Assess 2023;195:282.
22. Gao F, Jia J, Wang X. Occurrence and ordination of dichlorodiphenyltrichloroethane and hexachlorocyclohexane in agricultural soils from Guangzhou, China. Arch Environ Contam Toxicol 2008;54:155-66.
23. Gao H, Dong JY, Wu JN. Transfer and fate of HCHs in Lanzhou region. Chin Environ Sci 2008; 28: 407-411. (In Chinese). Available from: https://kns.cnki.net/kcms2/article/abstract?v=3Nyamzb2at545_H2f2X6QtEzk7va_3J-lzhCN-y4N_3N3-LqAl7p4RqYgFO3RtX_-uaYtO4wVE85qLNO9yKlYPwFC9NeLS-a-RswpmIRzlGaoaaurGuPSBObVqj7aDVp&uniplatform=NZKPT&language=CHS [Last accessed on 28 Aug 2023].
24. Jiang Y, Yuan L, Liang X, Nan Z, Deng X, Ma F. Status, Sources and Potential Risk of Polycyclic Aromatic Hydrocarbons in Soils from Hexi Corridor in Northwest China. Bull Environ Contam Toxicol 2022;108:563-70.
25. Jiang YF, Wang XT, Jia Y, et al. Occurrence, distribution and possible sources of organochlorine pesticides in agricultural soil of Shanghai, China. J Hazard Mater 2009;170:989-97.
26. Li XY. Study on the inventories and numerical simulation of fate and transport of OCPs in Gansu Province. Master’s dissertation, Lanzhou University, Lanzhou 2011. Available from: https://kns.cnki.net/kcms2/article/abstract?v=3Nyamzb2at6lWhwjrTpupNNBrm63FjXN72X6hO2dcKzm34Otd0X1Bj4CeRB7t7BiiphfkVouMZ5RwbKaT1wt-CN9e5zRoALvCH2FiDSzZk6tldQpHHFABAamrCkulquE&uniplatform=NZKPT&language=CHS [Last accessed on 28 Aug 2023].
27. Tang D, Liu X, He H, Cui Z, Gan H, Xia Z. Distribution, sources and ecological risks of organochlorine compounds (DDTs, HCHs and PCBs) in surface sediments from the Pearl River Estuary, China. Mar Pollut Bull 2020;152:110942.
28. Syed JH, Malik RN. Occurrence and source identification of organochlorine pesticides in the surrounding surface soils of the Ittehad Chemical Industries Kalashah Kaku, Pakistan. Environ Earth Sci 2011;62:1311-21.
29. Pereira R, Monterroso Martínez MC, Martínez Cortízas A, Macías F. Analysis of composition, distribution and origin of hexachlorocyclohexane residues in agricultural soils from NW Spain. Sci Total Environ 2010;408:5583-91.
30. Lv J, Shi R, Cai Y, et al. Assessment of 20 organochlorine pesticides (OCPs) pollution in suburban soil in Tianjin, China. Bull Environ Contam Toxicol 2010;85:137-41.
31. Chen M, Chen L, Huang P. Residues and possible sources of organochlorine pesticides in surface soil of Urumqi. Chin Environ Sci 2013; 34: 1838-1846. (In Chinese). Available from: https://kns.cnki.net/kcms2/article/abstract?v=3Nyamzb2at6C0RMSPjzPBamnOG8jmy0VDoCKRUyO6vMQOv5JW376HrKYvxLv-U_PyrOi5qfP9fOhKQvUmwcEfMDdD0jqLuxc0Uc8tli6kELLGvo65tHVlWWTOfko0Q6K&uniplatform=NZKPT&language=CHS [Last accessed on 28 Aug 2023].
32. Fu S, Cheng HX, Liu YH, Xu XB. Levels and distribution of organochlorine pesticides in various media in a mega-city, China. Chemosphere 2009;75:588-94.
33. Zhang HB, Luo YM, Zhao QG, Wong MH, Zhang GL. Residues of organochlorine pesticides in Hong Kong soils. Chemosphere 2006;63:633-41.
34. Sun K, Zhao Y, Gao B, Liu X, Zhang Z, Xing B. Organochlorine pesticides and polybrominated diphenyl ethers in irrigated soils of Beijing, China: levels, inventory and fate. Chemosphere 2009;77:1199-205.
35. Chakraborty P, Zhang G, Li J, Sivakumar A, Jones KC. Occurrence and sources of selected organochlorine pesticides in the soil of seven major Indian cities: assessment of air-soil exchange. Environ Pollut 2015;204:74-80.
36. Bajwa A, Ali U, Mahmood A, et al. Organochlorine pesticides (OCPs) in the Indus River catchment area, Pakistan: status, soil-air exchange and black carbon mediated distribution. Chemosphere 2016;152:292-300.
37. Meijer SN, Halsall CJ, Harner T, et al. Organochlorine pesticide residues in archived UK soil. Environ Sci Technol 2001;35:1989-95.
38. Ene A, Bogdevich O, Sion A. Levels and distribution of organochlorine pesticides (OCPs) and polycyclic aromatic hydrocarbons (PAHs) in topsoils from SE Romania. Sci Total Environ 2012;439:76-86.
39. Yadav IC, Devi NL, Li J, Zhang G, Shakya PR. Occurrence, profile and spatial distribution of organochlorines pesticides in soil of Nepal: Implication for source apportionment and health risk assessment. Sci Total Environ 2016;573:1598-606.
40. Manz M, Wenzel KD, Dietze U, Schüürmann G. Persistent organic pollutants in agricultural soils of central Germany. Sci Total Environ 2001;277:187-98.
41. Yu HY, Li FB, Yu WM, et al. Assessment of organochlorine pesticide contamination in relation to soil properties in the Pearl River Delta, China. Sci Total Environ 2013;447:160-8.
42. Mishra K, Sharma RC, Kumar S. Contamination levels and spatial distribution of organochlorine pesticides in soils from India. Ecotoxicol Environ Saf 2012;76:215-25.
43. Pokhrel B, Gong P, Wang X, Chen M, Wang C, Gao S. Distribution, sources, and air-soil exchange of OCPs, PCBs and PAHs in urban soils of Nepal. Chemosphere 2018;200:532-41.
44. Falandysz J, Ichihashi H, Szymczyk K, Yamasaki S, Mizera T. Metallic elements and metal poisoning among white-tailed sea eagles from the Baltic south coast. Mar Pollut Bull 2001;42:1190-3.
46. Meijer SN, Ockenden WA, Steinnes E, Corrigan BP, Jones KC. Spatial and temporal trends of POPs in Norwegian and UK background air: implications for global cycling. Environ Sci Technol 2003;37:454-61.
47. Nam JJ, Gustafsson O, Kurt-Karakus P, Breivik K, Steinnes E, Jones KC. Relationships between organic matter, black carbon and persistent organic pollutants in European background soils: Implications for sources and environmental fate. Environ Pollut 2008;156:809-17.
48. Zhang A, Chen Z, Ahrens L, Liu W, Li YF. Concentrations of DDTs and enantiomeric fractions of chiral DDTs in agricultural soils from Zhejiang Province, China, and correlations with total organic carbon and pH. J Agric Food Chem 2012;60:8294-301.
49. Kannan K, Battula S, Loganathan BG, et al. Trace organic contaminants, including toxaphene and trifluralin, in cotton field soils from Georgia and South Carolina, USA. Arch Environ Contam Toxicol 2003;45:30-6.
50. Borisover MD, Graber ER. Specific interactions of organic compounds with soil organic carbon. Chemosphere 1997;34:1761-76.
51. Degrendele C, Audy O, Hofman J, et al. Diurnal Variations of Air-Soil Exchange of Semivolatile Organic Compounds (PAHs, PCBs, OCPs, and PBDEs) in a Central European Receptor Area. Environ Sci Technol 2016;50:4278-88.
52. Qiu X, Zhu T. Using the o,p'-DDT/p,p'-DDT ratio to identify DDT sources in China. Chemosphere 2010;81:1033-8.
53. Qiu X, Zhu T, Yao B, Hu J, Hu S. Contribution of dicofol to the current DDT pollution in China. Environ Sci Technol 2005;39:4385-90.
54. Willett KL, Ulrich EM, Hites RA. Differential Toxicity and Environmental Fates of Hexachlorocyclohexane Isomers. Environ Sci Technol 1998;32:2197-207.
55. Doong RA, Peng CK, Sun YC, Liao PL. Composition and distribution of organochlorine pesticide residues in surface sediments from the Wu-Shi River Estuary, Taiwan. Mar Pollut Bull 2002;45:246-53.
56. Lee KT, Tanabe S, Koh CH. Distribution of organochlorine pesticides in sediments from Kyeonggi Bay and nearby areas, Korea. Environ Pollut 2001;114:207-13.
57. Sovoccol GW, Lewis RG, Harless RL, Wilson NK, Zehr RD. Analysis of technical chlordane by gas chromatography/mass spectrometry. Anal Chem 1977;49:734-40.
58. Eitzer BD, Mattina MI, Iannucci-berger W. Compositional and chiral profiles of weathered chlordane residues in soil. Environ Toxicol Chem 2001;20:2198-204.
59. Bidleman TF, Jantunen LM, Helm PA, Brorström-Lundén E, Juntto S. Chlordane enantiomers and temporal trends of chlordane isomers in arctic air. Environ Sci Technol 2002;36:539-44.
60. Long ER, Macdonald DD, Smith SL, Calder FD. Incidence of adverse biological effects within ranges of chemical concentrations in marine and estuarine sediments. Environmental Management 1995;19:81-97.
Cite This Article

How to Cite
Download Citation
Export Citation File:
Type of Import
Tips on Downloading Citation
Citation Manager File Format
Type of Import
Direct Import: When the Direct Import option is selected (the default state), a dialogue box will give you the option to Save or Open the downloaded citation data. Choosing Open will either launch your citation manager or give you a choice of applications with which to use the metadata. The Save option saves the file locally for later use.
Indirect Import: When the Indirect Import option is selected, the metadata is displayed and may be copied and pasted as needed.
Comments
Comments must be written in English. Spam, offensive content, impersonation, and private information will not be permitted. If any comment is reported and identified as inappropriate content by OAE staff, the comment will be removed without notice. If you have any queries or need any help, please contact us at [email protected].