Fueling cardiac myocyte proliferation
Regeneration is a biological process that is activated in response to various injuries, demonstrating the ability to restore lost components to their functional state. Therefore, it is not surprising that the concept of regeneration has fascinated humanity for centuries. In the ancient Greek myth of Prometheus, who faced punishment from Zeus for giving fire to mankind, Prometheus endured a punishment where an eagle would devour his liver every day. However, his liver would miraculously regenerate, resulting in an eternal cycle of torment and retribution for Prometheus.
From an evolutionary perspective, the ability to regenerate is widely distributed across phylogenetic lineages. For instance, hydra and planaria possess the ability to regenerate their entire bodies throughout their lives. On the other hand, lower vertebrates such as Xenopus, axolotl, and zebrafish can regrow lost limbs, tails, fins, or hearts. However, the ability of mammals to regenerate is typically limited to the early stages of development and is diminished as they mature[1]. Cell proliferation is a crucial process in regenerating tissue, and to support the energetically demanding process of proliferation, cellular metabolism undergoes significant changes to ensure a sufficient supply of essential nutrients. Likewise, the majority of proliferating cells utilize aerobic glycolysis for growth and proliferation.
In the heart, the ability of cardiac myocytes (CM) to proliferate decreases gradually after birth and becomes almost non-existent weeks after birth. Adult myocytes undergo terminal differentiation and lose their ability to proliferate. This aligns with the increase in oxygen availability that occurs after birth and a shift in the energy metabolism of CMs from carbohydrates like glucose and lactate to oxidative phosphorylation (OXPHOS)[2]. Although recent studies have highlighted several signaling pathways that can induce or facilitate the proliferation of adult CMs, the causal relationship between the progressive change in energy metabolism and the transition from quiescent to proliferative CMs remains uncertain. Therefore, investigation of the regulatory significance of different metabolic pathways and specific metabolic substrates in cardiac myocyte proliferation and heart regeneration is currently an active and promising field of research.
A recent article by Li et al. sheds light on the role of metabolism in cardiac myocyte proliferation and heart regeneration[3]. The authors reported that the expression pattern of the muscle-specific carnitine palmitoyl transferase 1b (CPT1B) is inversely correlated with CM proliferation, with Cpt1b being highly expressed in the adult heart while being negligible in the embryonic and neonatal heart. The CPT1B enzyme plays a crucial role in the adult heart by facilitating the oxidation of long-chain fatty acids (LCFAs) to produce ATP[4]. In order to study the impact of Cpt1b-mediated fatty acid oxidation (FAO) on CM proliferation, the authors genetically deleted this gene using two mouse models. The first model employed traditional
The role of CPT1β in facilitating the entry of long-chain fatty acids (LCFA) into the mitochondria suggests that removing this protein could potentially affect the tricarboxylic acid (TCA) cycle. Therefore, the authors performed a metabolic screen and discovered that alpha keto glutarate (α-KG) exhibited the most substantial upregulation among the metabolites in the Cpt1b knockout CMs. Alpha-ketoglutarate (α-KG) is an important intermediate in the tricarboxylic acid (TCA) cycle and plays a critical role in cellular energy metabolism. In addition, it serves as a regulator of gene expression by controlling α-ketoglutarate-dependent dioxygenases such as the JmjC family of histone lysine demethylase (KDM), the TET family of DNA
Several aspects of the present study raise further questions and open potential areas for further investigation.
1. The initial phenotypic observation indicates that the reduction in CPT1B leads to an increase in both the number of cardiac myocytes (CM) and the size of individual cardiac myocytes, i.e., hypertrophy. These two divergent phenotypes are likely caused by two distinct sets of cellular mechanisms. This prompts a critical inquiry about whether deletion of Cpt1b plays a dichotomous role in cellular metabolism based on the development stage of CM. Likewise, it will be interesting to identify the cell lineages of CMs that are more amenable to proliferation vs hypertrophic responses.
2. Previous studies of CPT1B deletion in mice showed an increase in heart weight: a fourfold increase in the body ratio as observed by Li et al., but the study also showed cardiac dysfunction[3]. This difference can be attributed to the non-cell autonomous effects leading to lipotoxicity[5] as proposed by Li et al.[3]. Furthermore, in another study by Ghosh et al.[6], skeletal muscle-specific deletion of CPT1B resulted in reduced α-KG, contrary to a substantial increase in α-KG as seen by Li et al.[3]. Therefore, to unequivocally rule out any deleterious effects in CM specific Cpt1b knockout mice, a long-term cardiac function and survival analysis is warranted.
3. Recent studies have documented the role of the KDM5 family of proteins in the maturation of cardiac myocytes[7]. It was shown that the levels of KDM5 gradually decrease during the maturation of CM and are negligible in the mature adult CM. In these circumstances, the present findings by Li et al. may be at odds with the role of KDM5 in adult CM proliferation[3]. A probable explanation for this paradox may be that Cpt1b deletion results in KDM5B re-expression in adult CMs and thereby its activity. However, the RNA levels of Kdm5b remained unaltered in this current study by Li et al.[3]. It is yet unknown if post-transcriptional changes can account for KDM5B protein expression in CM. Alternatively, it is also plausible that the elevated α-KG levels, as observed by Li et al., in the Cpt1b knockout CMs could promote residual KDM5B activity[3].
4. While the study by Li et al. provides some evidence of the potential role of KDM5B in regulating the immature gene program (mostly sarcomeric genes), the precise mechanism by which KDM5B induces the expression of cell cycle remains unknown[3]. Furthermore, the current study did not investigate the role of other KDM5 family of proteins that are also dependent on α-KG and their contribution, if any, in the CMs. Likewise, the context-dependent role of histone modifications should also be taken into consideration. For example, several studies have shown the induction of KDM5A and B in the context of heart failure, suggesting a pathogenic role for these proteins likely through the activation of the fetal gene program.
5. Given the many pleiotropic roles of α-KG, it is conceivable that the rise in α-KG levels could potentially result in the activation of TET enzymes[8-10]. TET protein has been shown to play a significant role in the proliferation of cardiac myocytes and the expression of[9] cardiac genes and thereby may contribute to CM proliferation and/or other phenotypes in conjunction with, or independent of KDM5s in the Cpt1b knockout CMs.
6. Finally, the study by Li et al. provides some evidence of cardiac repair mediated by Cpt1b in the context of Ischemic reperfusion[3]. Given that the process of optimal regenerating myocardium involves CM proliferation, electromechanical coupling, angiogenesis and resolution of fibrosis, and favorable immune system microenvironment, further studies will be required to establish the role of metabolic changes in the bona fide regeneration of the damaged and thereby cardiac regeneration.
Nevertheless, the current study by Li et al. highlights the importance of metabolism in regulating cardiac gene expression and underscores its significant role in cardiac myocyte proliferation[3]. The involvement of metabolites in regulating genes related to the heart offers innovative and promising opportunities for further “fueling cardiac regeneration”.
DECLARATIONS
Authors’ contributions
Conceived and wrote the paper: Deogharia M, Gurha P
Availability of data and materials
Not applicable.
Financial support and sponsorship
This work was supported by NIH grant (R56-HL165334-01) and John. Dunn foundation to Dr. Gurha P.
Conflicts of interest
Both authors declared that there are no conflicts of interest.
Ethical approval and consent to participate
Not applicable.
Consent for publication
Not applicable.
Copyright
© The Author(s) 2024.
REFERENCES
1. Bely AE, Nyberg KG. Evolution of animal regeneration: re-emergence of a field. Trends Ecol Evol 2010;25:161-70.
3. Li X, Wu F, Gunther S, et al. Inhibition of fatty acid oxidation enables heart regeneration in adult mice. Nature 2023;622:619-26.
4. Yamazaki N, Shinohara Y, Shima A, Terada H. High expression of a novel carnitine palmitoyltransferase I like protein in rat brown adipose tissue and heart: isolation and characterization of its cDNA clone. FEBS Lett 1995;363:41-5.
5. Haynie KR, Vandanmagsar B, Wicks SE, Zhang J, Mynatt RL. Inhibition of carnitine palymitoyltransferase1b induces cardiac hypertrophy and mortality in mice. Diabetes Obes Metab 2014;16:757-60.
6. Ghosh S, Wicks SE, Vandanmagsar B, et al. Extensive metabolic remodeling after limiting mitochondrial lipid burden is consistent with an improved metabolic health profile. J Biol Chem 2019;294:12313-27.
7. Deogharia M, Agrawal A, Shi M, et al. Histone demethylase KDM5 regulates cardiomyocyte maturation by promoting fatty acid oxidation, oxidative phosphorylation, and myofibrillar organization. bioRxiv 2023. Available from: https://www.biorxiv.org/content/biorxiv/early/2023/05/28/2023.04.11.535169.full.pdf [[Last accessed on 11 Dec 2023]
8. Dhat R, Mongad D, Raji S, et al. Epigenetic modifier alpha-ketoglutarate modulates aberrant gene body methylation and hydroxymethylation marks in diabetic heart. Epigenetics Chromatin 2023;16:12.
9. Fang S, Li J, Xiao Y, et al. Tet inactivation disrupts YY1 binding and long-range chromatin interactions during embryonic heart development. Nat Commun 2019;10:4297.
Cite This Article
How to Cite
Download Citation
Export Citation File:
Type of Import
Tips on Downloading Citation
Citation Manager File Format
Type of Import
Direct Import: When the Direct Import option is selected (the default state), a dialogue box will give you the option to Save or Open the downloaded citation data. Choosing Open will either launch your citation manager or give you a choice of applications with which to use the metadata. The Save option saves the file locally for later use.
Indirect Import: When the Indirect Import option is selected, the metadata is displayed and may be copied and pasted as needed.
About This Article
Copyright
Data & Comments
Data
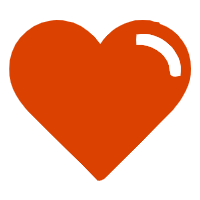
Comments
Comments must be written in English. Spam, offensive content, impersonation, and private information will not be permitted. If any comment is reported and identified as inappropriate content by OAE staff, the comment will be removed without notice. If you have any queries or need any help, please contact us at [email protected].