Circulating culprit or therapeutic bullseye: lipoprotein(a) in cardiovascular risk assessment and novel therapeutic prospects
Abstract
Lipoprotein(a) [Lp(a)] has emerged as a significant player in the realm of cardiovascular disease (CVD), exerting a pivotal role in atherosclerotic cardiovascular disease (ASCVD), aortic valve stenosis (AVS), and overall cardiovascular (CV) and all-cause mortality. Since its discovery in 1963 by Kåre Berg, our understanding of Lp(a) has undergone significant evolution. This comprehensive review delves into the genetics, structure, assembly, and inter-population differences of Lp(a), shedding light on its intricate involvement in CVD. Genetically, Lp(a) is primarily influenced by variations in the LPA gene. The LPA gene encodes apo(a) and the variation in the kringle domains is the main determinant of plasma Lp(a) levels. Other genetic variants, such as SNPs in the LPA gene region, the pentanucleotide repeat polymorphism, and specific SNPs in the coding sequences of kringle domains, have also been associated with varying Lp(a) concentrations. Additionally, genes outside the LPA locus, including APOE, APOH, and CEPT gene regions, contribute to Lp(a) variability across different populations. Inter-population differences in Lp(a) levels are evident, with ethnicity and sex playing significant roles. Racial disparities in median Lp(a) concentration have been observed, with black individuals often displaying higher levels compared to their white counterparts. The review underscores Lp(a) as an independent, heritable CV risk factor in both primary and secondary settings. High Lp(a) levels are closely linked to the recurrence of myocardial infarction, AVS, and CV events. The necessity of measuring Lp(a) concentration at least once in life to assess an individual's absolute global CV risk is emphasized. Despite substantial progress, many questions remain unanswered about Lp(a), including its physiological role in the cardiovascular system and its involvement in inflammatory and thrombotic processes. Ongoing research holds promise for the development of therapeutic interventions, such as pharmacological agents and apheresis, to mitigate the cardiovascular risks associated with elevated Lp(a) levels. This review highlights the multifaceted nature of Lp(a) in the context of cardiovascular health, emphasizing the importance of continued research efforts to unravel its complexities and develop innovative strategies for managing its associated risks.
Keywords
INTRODUCTION
Lipoprotein(a) (Lp[a] is a low-density lipoprotein (LDL) cholesterol-like particle that was first described by Kåre Berg, MD, in 1963[1]. The current literature has established a link between Lp(a) concentration and increased risks of atherosclerotic cardiovascular disease (ASCVD) and calcific aortic valve stenosis (AVS). This relationship was also described in a recent consensus statement of the European Atherosclerotic Society (EAS) on Lp(a)[2]. As a risk factor, an elevated Lp(a) concentration is a co-leader - together with inflammatory, metabolic, and thrombotic risk factors - in determining residual cardiovascular risk, which is responsible for an increased risk of developing ASCVD, even in well-treated patients. The Lp(a) circulation level is mainly determined by genetic factors, and its circulating level tends to be stable despite a few environmental or medical factors that may influence it. Drugs for reducing Lp(a) are in various stages of clinical trials, while apheresis remains a viable alternative for lowering Lp(a) levels. This article aims to review the current literature on the biology, pathophysiology, and therapies available and under development for Lp(a).
GENETICS, STRUCTURE, AND ASSEMBLY
Lp(a) assembly occurs at the hepatocyte cell membrane surface or in the space of Disse[3]. Lp(a) is an LDL cholesterol-like particle with a core of triglycerides and cholesterol esters and a protein component made by a single copy of apolipoprotein B-100 (apoB) and a single copy of apolipoprotein(a) (apo[a])[4] The apo(a) copy is synthesized by the liver[5] and is bound to the Lp(a) structure via a disulfide linkage. The size of apo(a) mainly assesses the Lp(a) circulating level. Indeed, Lp(a) blood level is inversely correlated to apo(a) size: the smaller the size, the higher the Lp(a) blood level[6] A specific characteristic of the apo(a) structure is the presence of loop-like structures called kringles[7]. Kringles are also present in some coagulation factors, such as plasminogen (PLG); however, the linker domain among kringles is glycosylated in apo(a). Kringle domains are also present in proteins such as angiostatin, hepatocyte growth factor, prothrombin, urokinase, and tissue-type plasminogen activator. The common structure of apo(a) and angiogenic factors such as plasminogen and angiotensin has led to a possible anti-angiogenic role of apo(a). Regarding this association, contradictive results are available in the current literature[8].
The LPA gene is the major gene controlling Lp(a) levels and codes for two kringle domains (kringles IV [KIV] and kringles V [KV]). KIV expands into 10 kringle copies (KIV-1-KIV-10)[3]. Nine of them (KIV-1, KIV-3-KIV-10) are present in only one copy of the apo(a) structure; KIV-2 could result in 1-40 copies. Therefore, a low number of KIV-2 copies (< 23) results in a small apo(a) structure and low Lp(a) circulating levels[2]. Indeed, a small apo(a) copy secretion is more efficient than long ones because of the shorter retention period in the endoplasmic reticulum and the lower degree of presecretory degradation[9,10]. Hence, Lp(a) concentrations depend on the apo(a) synthesis rate rather than its metabolism[11]. Lp(a) circulating levels are ≈ 70% to ≥ 90% genetically determined[12], and they tend to be stable for a lifetime. However, some nongenetic factors such as diet, kidney and hepatic function, and inflammatory conditions may influence these levels. Indeed, a low carbohydrate/high-fat diet[13] or kidney impairment may decrease Lp(a) blood level[14], whereas hepatic impairment may increase them[15].
In addition to the KIV-2 size polymorphism, other sequence variations in the LPA gene have been described as responsible for different Lp(a) plasma concentrations. Mack et al. conducted a meta-analysis of five studies and found that SNPs significantly influenced Lp(a) circulating levels[16]. In patients with high Lp(a) levels, the authors identified variants such as rs10455872 and rs3798220 in the LPA gene region[16]. A repeated polymorphism of the pentanucleotide sequence TTTTA (5′pentanucleotide repeat polymorphism [5′PNRP]) has been identified in the promoter region of the LPA gene. Although alleles with eight repeats are the most frequent in all populations, alleles with 4-12 repeats have been described. A lower number of repeats has been described in European patients with higher Lp(a) concentrations[17]. This polymorphism has been identified as responsible for 3%-14% of the variability in Lp(a) levels; however, it has not been confirmed in Africans.
In the 5′-flanking region of the LPA locus, two other single-nucleotide polymorphisms (SNPs) have been identified as being responsible for Lp(a) concentration: rs1853021 (c.-49T>C, traditionally +93 C/T) and rs1800769 (c.-21G>A, traditionally +121 G/A)[18,19]. In vitro studies have shown that rs1853021 leads to lower Lp(a) blood levels[20]. Studies on the sub-Saharan African population have confirmed this finding, whereas it has not been established in Europeans. For rs1800769, Lp(a) alleles have been associated with higher transcriptional activity and Lp(a) levels[19,20,21].
In the coding sequences of KIV-6-KIV-10, there are nonsynonymous substitutions in KIV-6 (rs200561706; p.Ser1193Phe) and in KIV-8 (p.Gly1393Arg) in Africans[22], whereas rs41272110 (p.Thr1399Pro; Thr3888Pro) in KIV-8 is linked to lower Lp(a) concentrations in Europeans[23]. However, rs140720828 (p.Arg1508Trp) in KIV-9 has been responsible for higher Lp(a) levels in South Africans. In Europeans, rs3798220, a nonsynonymous SNP (p.Ile1891Met, also referred to as Ile4399Met) in the protease-like domain of LPA, and rs10455872 in KIV-7 have been associated with high Lp(a) concentrations[24].
Said et al. concluded that variant rs10455872 is the principal genetic determinant of Lp(a) circulating levels (explaining 24.4% of its variance). However, variant rs41267813 (A) in the LPA gene locus has a protective effect on Lp(a), increasing in carriers at least 1 G allele of rs10455872[25]. The sheer length of the KIV-2 sequence in the LPA gene (up to 50% of the LPA gene sequence) suggests that mutations may be present in this region; however, little is known about its sequence variation due to technical limitations[4]. Other genes are associated with Lp(a) levels, such as the APOE ε2 allele, which is responsible for 0.5% of Lp(a) concentration variations to lower-level[16] APOH (it codes β2-glycoprotein 1)[16] and CEPT gene regions[9]. The lowering Lp(a) concentration effect of the APOE ε2 allele has been proposed to be linked to both Lp(a) catabolism (it may lead to an increase in the number of receptors available for Lp[a]) and synthesis effects[16].
Hoekstra et al. identified variants outside the LPA gene locus, such as rs1065853 on chromosome 19 (located downstream of APOE) and rs247617 (located upstream of CEPT) or rs826128 on chromosome 2, that were responsible for lower Lp(a) concentrations. However, variant rs8178824 in APOH leads to an increase in Lp(a) concentrations[26]. The link between genetic factors and circulating levels of Lp(a) has led researchers to look for genetic therapies for lowering Lp(a) level, such as RNA-targeted therapies, in particular antisense oligonucleotides (ASOs) or small interfering RNAs (siRNAs), to regulate apo(a) mRNA production.
INTRA- AND INTER-POPULATION DIFFERENCES
As shown above, the Lp(a) concentration is principally influenced by genetic variants. Otherwise, Lp(a) circulating levels tend to remain stable over the years, although nongenetic factors may impact them. Ethnicity and sex are other possible factors influencing Lp(a) levels.
Results from the 460,506 patients of the UK Biobank with a median follow-up of 11.2 years confirmed racial differences in median Lp(a) concentration. Among the study group, 94.3% self-reported as white, 1.9% as South Asian, 1.6% as Black, and 0.3% as Chinese. Their median Lp(a) concentrations were 19, 31, 75, and
The ARIC study indicated similar results. Among 13,318 participants (3,467 Blacks and 9,851 whites), the median Lp(a) level was 12.8 mg/dL in Blacks and 4.3 mg/dL in whites. Otherwise, women showed higher Lp(a) levels than men (Blacks 13.7 vs. 11.9 mg/dL, P < 0.0001; whites 4.8 vs. 3.9 mg/dL, P < 0.0001)[27]. Among the Asian population, a higher median Lp(a) concentration was found in Indian patients than in Chinese patients[31].
Principally, differences in mean Lp(a) concentrations among ethnic groups may be explained by genetic variants in the LPA gene locus, mainly by apo(a) gene size polymorphism. Furthermore, some SNPs may contribute to Lp(a) heterogeneity across ethnicities due to their different expression among populations. Two SNPs (T3888P and G+1/inKIV-8A) are more frequent in Caucasians and lead to a decrease in Lp(a) levels, whereas SNP (G-21A) is more common in African-Americans and leads to an increase in Lp(a) levels.
Beyond the LPA gene, genetic variants in other gene loci may explain Lp(a) variability across ethnicities. In Hutterites, the Lp(a) concentration is influenced by eight genes on chromosome 6q26-q27. In Caucasians, three variants in the PLG gene (rs14224, rs783147, and r6935921) impact the Lp(a) concentration, whereas in the Spanish population, variants in the TFPI gene have the same rule.
LP(A) AND CARDIOVASCULAR DISEASES
Lp(a) has emerged as a significant player in the realm of cardiovascular disease (CVD) due to robust evidence from epidemiology and genetics that demonstrates a causal association of high Lp(a) levels with ASCVD, AVS, and cardiovascular (CV) and all-cause mortality in men and women[32]. Lp(a) is an independent heritable CV risk factor in both primary and secondary settings; based on the available data, high Lp(a) levels are closely related to myocardial infarction (MI) and AVS. Furthermore, data from the Copenhagen general population study of individuals with preexisting CVD revealed that Lp(a) levels are associated with the recurrence of CV events[33] [Figure 1]. The Lp(a) concentration must be measured at least once to avoid any substantial underestimation of a patient’s absolute global CV risk. However, this special lipoprotein in the body is still not completely understood; neither its physiological role in the CV system nor all the pathological processes in which it is involved are known. Lp(a) also seems to play a key role in inflammation; however, its function in thrombosis remains controversial [Figure 2].
Figure 1. Risk of clinical outcomes according to Lipoprotein(a) levels. Absolute risk per 10,000 person-years according to Lp(a) concentrations. Based on data from 70,286 white individuals in the Copenhagen General Population Study with a median of 7.4 years of follow-up.
LP(A) AND ATHEROSCLEROSIS
The 2022 European Atherosclerosis Society (EAS) Lp(a) consensus statement strongly supports a causal association between elevated Lp(a) concentrations and ASCVD in different ethnicities, and this relationship is continuous, with no apparent threshold effect even at low levels of low-density lipoprotein cholesterol (LDL-C)[2]. The higher one’s Lp(a) concentration, the greater one’s risk of ASCVD. According to data from the 415,274 participants of European ancestry in the UK Biobank, individuals with Lp(a) levels of 30, 50, 75, 100, and 150 mg/dL had 1.22-, 1.40-, 1.65-, 1.95-, and 2.72-fold higher ASCVD risk, respectively, compared to those with a median Lp(a) concentration of 7 mg/d[2,32-34]. Knowledge of an elevated Lp(a) value influences the management of other risk factors[32]. This increased CV risk is consistent across all baseline risk categories determined by traditional risk factors. Hence, while awaiting new specific Lp(a)-lowering therapies, the EAS recommends personalized CV risk management through intensive and early control of other CV risk factors, particularly LDL-C. By analyzing data from the UK Biobank, it is possible to estimate how much intensification of LDL-C lowering therapies is needed to mitigate the tendency for atherosclerotic plaque progression and the increased risk of major CV events (MACEs) caused by a person’s Lp(a) level[2]. Data from the Multi-Ethnic Study of Atherosclerosis (MESA)[35] have recently highlighted a 60% increase in the risk of coronary heart disease (CHD) in individuals with elevated Lp(a) levels
Interesting emerging data have shown that the use of Lp(a) may allow better management of patients with familial hypercholesterolemia (FH) who are genetically prone to accelerated ASCVD and premature CV events. Elevated Lp(a) levels provide a partial explanation for clinical FH in patients for whom no FH-related genetic variant can be identified. In as many as 9% of cases, the clinical diagnosis of FH no longer applies when LDL-C levels are adjusted for Lp(a) effects. The inclusion of Lp(a) measurements reclassifies approximately 20% of patients into a higher ASCVD risk category[40]. In addition, the Copenhagen General Population Study on 69,644 patients produced insightful findings about Lp(a) levels and their association with LDL-C in clinical and genetic FH. Specifically, the analysis revealed levels of Lp(a) equivalent to
Another powerful link between Lp(a) and atherosclerosis comes from Mendelian randomization studies that provide robust evidence of a cause-and-effect relationship between elevated Lp(a) concentrations and ASCVD[2]. Intriguing genetic investigations that considered factors such as apo(a) isoform size or K-IV repeat numbers revealed that genetic variants associated with higher Lp(a) levels were more prevalent in ASCVD patients compared to control groups[41-43]. Conversely, rare genetic variants causing Lp(a) reduction or certain common splice site variants with Lp(a)-lowering effects have been found to offer protection against CV disease[44].
Lp(a) is the principal lipoprotein carrier for OxPL and its role adversely affects arterial wall. In fact, OxPLs, preferentially carried by Lp(a) in the plasma, are supposed to play a crucial role in the Lp(a) pro-inflammatory properties: several studies suggest that the OxPL component of Lp(a) particles promotes endothelial cell dysfunction, autotaxin and chemo-attractants delivery, transendothelial migration of monocytes and macrophage apoptosis.
Such cellular mechanisms could underlie atherosclerosis through increased plaque vulnerability, enhanced foam cell formation, increased endothelial cell permeability and vascular smooth muscle cell proliferation and migration[45].
Schnitzler et al., in an intriguing study, highlight another metabolic pathway associated with Lp(a) pathogenicity: 6-phophofructo-2-kinase/fructose-2,6-biphosphatase (PFKFB3) is considered the main glycolytic player of Lp(a)-induced endothelial inflammation. Increased endothelial PFKFB3 and ICAM (intercellular adhesion molecule)-1 expression has been detected in carotid endarterectomy patients with high Lp(a) levels and blocking PFKFB3 activity seems to reduce the inflammatory burden of OxPLs associated with Lp(a)[46,47].
Lp(a) promotes its atherogenic actions when transferred from circulation to the arterial wall: high Lp(a) levels result in an excessive amount of OxPLs in the arterial wall, leading to plaque instability and subsequent CV events mainly represented by myocardial infarction and ischemic stroke[48-51].
The presence of high levels of Lp(a) in the blood promotes its atherogenic actions when transferred to the arterial wall, increasing the vulnerability of atherosclerotic plaques, promoting vascular smooth muscle cell proliferation, and enhancing the adhesion of molecules, chemotactic factors, and plasma cytokines[45]. Lp(a) pro-atherosclerotic properties can be partially explained by oxidized phospholipids (OxPL). The molecule Lp(a) carries OxPL[15], and its function in the bloodstream adversely affects the inflammation of the arterial wall, atherosclerotic lesions, and endothelial function, which ultimately leads to CV events. High levels of Lp(a) cause an excessive amount of oxidized phospholipids (OxPLs) to form in the arterial wall. This results in plaque instability due to the development of foam cells, chemotaxis, and heightened inflammation, exacerbating the risks of cardiovascular events[48-51].
In order to reduce the impact of Lp(a) on the development and progression of atherosclerosis, potent therapies are emerging, which enable the direct targeting of Lp(a) levels.
LP(A) AND AORTIC VALVE STENOSIS
Both epidemiological and genetic data strongly support the association between elevated Lp(a) levels and the development of AVS. Increased Lp(a) levels are linked to both micro- and macrocalcification of the aortic valve[52]. This connection is particularly clear in relatively young and healthy individuals aged 45-54 years, and the risk increases threefold when Lp(a) exceeds the 80th percentile compared to lower levels (15.8% vs. 4.3%, respectively)[53,54]. The population-based Rotterdam study provided significant insight into the impacts of Lp(a) on aortic valve disease. Kaiser et al. analyzed cardiac computed tomography at baseline and after a median follow-up of 14 years for 922 individuals whose Lp(a) measurements were available[55]. For each 50 mg/dL increase in Lp(a) concentration, there was a 43% higher risk of baseline aortic valve calcification (AVC); a new-onset AVC was defined when an individual exhibited an Agatston score > 0 on the follow-up scan, even if there was no aortic valve calcification on the initial scan. Surprisingly, nearly 60% (415 out of 702) of individuals without AVC at baseline showed calcifications on the follow-up scan. An increase in Lp(a) concentration of 50 mg/dL was associated with a 30% elevated risk of new aortic valve calcification instances. Among 220 individuals undergoing baseline and follow-up scans, Agatston units were used to measure the progression of aortic valve calcification. Signs of calcification were evident in both scans, but there was no association between Lp(a) levels and the advancement of the disease[55]. Therefore, high Lp(a) levels should be involved in the initial phase of AVS; thus, Lp(a)-lowering interventions may be most effective in the early pre-calcific stages of aortic valve disease. Lp(a)-transported OxPL is considered a key factor contributing to the development of AVS because it induces the expression of calcification genes in vascular and valvular cells promoting AVS, increasing the incidence of aortic valve replacement and death[56].
Pro-osteogenic effects of Lp(a) and OxPL on valvular interstitial cells promote the progression of AVS through an increased calcification activity and a faster progression in calcium score and in hemodynamic progression assessed both by echocardiography and computed tomography, leading to an enhanced aortic valve replacement and death[56].
In addition, it is interesting to refer to a brilliant study by Bouchareb et al. in which they identified that autotaxin and lysophosphatidic acid are involved in the pathobiology of calcific aortic valve disease by promoting inflammation and mineralization of the aortic valve[57,58]. Therefore, those findings have a potentially relevant translational impact because autotaxin or lysophosphatidic receptors could represent novel therapeutic targets for the management of calcific aortic valve disease[59].
LP(A) AND THROMBOSIS
Extensive observational and genetic studies in adults have failed to establish a connection between genetically elevated Lp(a) levels and venous thromboembolism[2,59]. The hypothesis that Lp(a) might hinder plasmin’s fibrinolytic activity due to its structural similarity to plasminogen has been proposed[60]. However, the protease domain of apo(a), a component of Lp(a), lacks enzymatic functionality. Despite some in vitro evidence in support of this theory, it has been challenging to prove any antifibrinolytic effect of Lp(a) elevation in vivo in humans. Indeed, in recent phase 1 and phase 2 clinical trials, reducing elevated Lp(a) by over 80% with apo(a) antisense therapy did not lead to any changes in various fibrinolytic assays conducted ex vivo[61]. Furthermore, even Mendelian randomization studies do not support elevated Lp(a) as a causal risk factor for venous thromboembolism. Conversely, the thrombogenic role of Lp(a) in the arterial system is much more controversial. It has been proposed that high Lp(a) levels might promote plaque-associated thrombosis after plaque erosion and rupture; however, the most supported hypothesis is that the pathogenic action of Lp(a) is mainly expressed by inducing inflammation and plaque instability, while plaque rupture-associated thrombosis might be a consequence of the naturally occurring coagulation process rather than a direct effect of Lp(a)[32].
Nevertheless, some conflicting evidence on the pediatric population should be mentioned. Limited data suggest a correlation between Lp(a) and the occurrence of arterial ischemic stroke in children. This association appears to be more pronounced for recurrent strokes, with the risk more than doubling when Lp(a) levels exceed 30 mg/dL[62]. Children who experience strokes of unclear origin often have other complicating conditions, such as congenital heart disease, coagulation disorders, or chronic inflammatory conditions. Consequently, the pro-inflammatory and pro-atherogenic properties of Lp(a) might be more prominent in young adults, whereas in the elderly, multiple risk factors combined with elevated Lp(a) could increase the likelihood of cerebrovascular events[63].
LP(A) AND INFLAMMATION
Inflammation plays a central role in the initiation and progression of atherosclerotic plaque growth. Lp(a) triggers various inflammatory pathways in the walls of arterial blood vessels by encouraging the extravasation of monocyte cells and activating endothelial cells through the release of inflammatory markers, such as IL-6, IL-1β, and TNFα[64]. Additionally, Lp(a) upregulates various receptors and molecules related to chemotaxis, cell adhesion, transmigration, and scavenging (CCR7, CD62L, CD11b, CD11c, CD29, CD36, and SR-A). Experimental research has demonstrated that these effects can be attributed to adhesion molecules, such as intercellular adhesion molecule-1 (ICAM-1), transcription and translation upregulation, and increased activity of the enzyme 6-phosphofructo-2-kinase/ fructose-2,6-bisphosphatase (PFKFB)-3 induced by Lp(a)[37]. This pro-inflammatory state induced by Lp(a) in monocytes results in heightened adhesion of these cells to the endothelium and their subsequent migration across the endothelial barrier, leading to plaque instability and accelerated atherosclerosis.
In a prespecified post hoc secondary analysis of the Assessment of Clinical Effects of Cholesteryl Ester Transfer Protein Inhibition with Evacetrapib in Patients at a High Risk for Vascular Outcomes (ACCELERATE) trial[65], in patients with established vascular disease undergoing optimal medical therapy, increasing Lp(a) levels during treatment were significantly associated with hard CV events (CV death, MI, and stroke) in patients with high-sensitivity C-reactive protein (hs-CRP) levels of 2 mg/L or higher during treatment but not in those with lower levels. These recent data reveal for the first time that the Lp(a)-related risk of developing ASCVD appears to be substantially influenced by the presence of ongoing systemic inflammation. These findings, in addition to providing greater clarity on the mechanisms linking Lp(a) to ASCVD risk, could have practical implications for identifying individuals who might benefit most from emerging therapies designed to reduce Lp(a) levels.
LP(A) AS AN EMERGING THERAPEUTIC TARGET
Impact of current lipid-lowering therapies on Lp(a) levels
The effects of current lipid-lowering drugs on Lp(a) levels are variable and, in many instances, of limited clinical significance [Figure 3]. This complexity is compounded by the fact that the absolute reductions in Lp(a) required to achieve clinically meaningful reductions in CVD risk remain uncertain [Table 1].
Figure 3. Effect of lipid-lowering therapies on Lp(a) levels. CEPT: Cholesteryl ester transfer protein; Lp(a): lipoprotein(a); LLT: lipid-lowering therapy; PCSK9: proprotein convertase subtilisin/kexin type 9.
Efficacy in reducing Lp(a) and proven benefit in reducing cardiovascular events
Drug/class | Effects on Lp(a) concentration | Proven MACE reduction |
Statins | 8.5%-19.6% increase | Yes |
Ezetimibe | No effect | Yes |
Niacin | 20%-30% reduction | No |
CETP Inhibitors | 32%-56% reduction | No |
PCSK9i | 26% reduction | Yes |
Lp(a) apheresis | 78%-94% reduction | Yes (retrospective trials only) |
Inclisiran | 18.6%-25.6% reduction | No |
Bempedoic acid | No effect | Yes |
Pelacarsen | 77.8% reduction | Unknown |
Olpasiran | 100% reduction | Unknown |
Muvalaplin | 63%-65% reduction | Unknown |
Nonetheless, several Mendelian randomization studies have suggested that a decrease in Lp(a) concentration of 50-100 mg/dL could result in a clinically significant reduction in major adverse cardiovascular events (MACE) at a five-year follow-up. In this context, Madsen et al. demonstrated that, assuming a 39 mg/dL reduction in LDL cholesterol is associated with a 22% reduction in the risk of major vascular events across primary and secondary prevention trials, an absolute reduction in Lp(a) total mass of 55 mg/dL (116 nmol/L) would be necessary to achieve a similar effect[33].
STATINS
Statin therapy has demonstrated a significant elevation in Lp(a) levels, with mechanisms that remain unclear. These findings have been supported by a recent subject-level meta-analysis of 5,256 patients (1,371 receiving placebo and 3,885 on statins) from six randomized controlled trials (RCTs), revealing a mean percentage increase in Lp(a) levels from 8.5% to 19.6%[66]. However, another meta-analysis that included 39 trials and 24,448 patients indicated a nonsignificant 0.1% increase in Lp(a) within the statin-treated groups, with no differences observed among various statins or different statin intensities[66].
Nevertheless, any potential effect in terms of Lp(a) elevation does not appear to be associated with a concomitant reduction in CV outcomes in statin-treated patients[66,67]. Consequently, the recent consensus statement from the EAS[2] recommends against discontinuing statin therapy in individuals with elevated Lp(a) levels, specifying that any potential risks of statin therapy are outweighed by the benefits[68,69]
EZETIMIBE
Ezetimibe does not appear to affect the levels of Lp(a) in the blood. In fact, a meta-analysis of 10 randomized placebo-controlled trials involving 5,188 subjects found that the administration of Ezetimibe had no impact on modifying plasma Lp(a) concentrations[69].
NIACIN
In an RCT involving 25,673 patients, niacin exhibited a substantial dose-dependent decrease in Lp(a) levels ranging from 20%-30%. Nevertheless, its use is currently not recommended by guidelines due to the absence of any clinical advantage. Furthermore, niacin use is limited by adverse effects, including new-onset diabetes, flushing, and gastrointestinal distress[70,71].
CETP INHIBITORS
Cholesteryl ester transfer protein (CETP) inhibitors have shown the ability to reduce Lp(a) levels by approximately 30% in three studies. Within a limited cohort of 39 patients, anacetrapib treatment resulted in a notable Lp(a) reduction of 34.1%[72]. In a placebo-controlled trial with 393 participants, evacetrapib achieved a significant decrease of 32% in Lp(a) concentrations[73]. In an RCT of 120 dyslipidemic individuals receiving high-intensity statin therapy, obicetrapib elicited a substantial reduction in Lp(a) levels of up to 56%[74]
However, it is worth noting that CETP inhibitors have been predominantly evaluated in trials that did not select patients based on elevated Lp(a) levels. Furthermore, CETP inhibitors did not demonstrate any discernible advantages in terms of reducing MACEs. Consequently, CETP inhibitors are not currently recommended in clinical practice.
PCSK9 INHIBITORS
In two clinical trials in which they were investigated, namely FOURIER (Further Outcomes Research with PCSK9 Inhibition in Subjects with Elevated Risk) for evolocumab and ODYSSEY OUTCOMES (Evaluation of Cardiovascular Outcomes After an Acute Coronary Syndrome During Treatment With Alirocumab) for alirocumab, PCSK9 inhibitors demonstrated a reduction in both absolute Lp(a) levels (approximately 26%) and MACEs, potentially indicating their efficacy in lowering Lp(a)-related CV risk[75,76].
Additional findings emerged from the secondary analyses of these RCTs. For instance, within the ODYSSEY OUTCOMES trial, individuals with LDL-C levels approaching 70 mg/dL and Lp(a) levels surpassing the median value of 13.7 mg/dL derived substantial clinical advantages from alirocumab treatment. Conversely, those with LDL-C levels near 70 mg/dL and lipoprotein(a) levels below 13.7 mg/dL did not experience further reductions in MACEs with alirocumab[77]. In a secondary analysis derived from the FOURIER study, patients with higher baseline Lp(a) levels (exceeding 37 nmol/L-14.8 mg/dL) exhibited more significant reductions in Lp(a) and tended to experience greater CV benefits from PCSK9 inhibitor treatment[78]
These findings suggest that PCSK9 inhibitor therapy may confer clinical benefits regarding Lp(a)-related CV risk, although the magnitude of these benefits remains unclear. It is important to emphasize that these medications have not been specifically tested in patients with elevated Lp(a) levels. In fact, in the pivotal trials, median Lp(a) levels were relatively low (13.7 mg/dL and 37 nmol/L, respectively). Therefore, PCSK9 inhibitors are not currently recommended for the treatment of patients with elevated Lp(a) levels.
LIPOPROTEIN APHERESIS
Currently, lipoprotein apheresis stands as the sole FDA-approved intervention for lowering Lp(a) levels. According to a recent EAS document, this therapy may be considered in individuals exhibiting markedly elevated Lp(a) levels and experiencing progressive CVD despite the optimal management of conventional risk factors[71]
In a single-center study involving 14 patients, this intervention demonstrated a 63% reduction in Lp(a) levels and a 94% decrease in MACEs over an average treatment duration of 48 months[79]. Conversely, a multicenter investigation incorporating 170 patients afflicted by Lp(a) hyperlipoproteinemia, who were already receiving the maximum tolerated lipid-lowering medications, showed a substantial 70% reduction in Lp(a) levels, coupled with a proportionate reduction of 78% in MACEs[80,81].
However, all evidence regarding the efficacy of lipoprotein apheresis is derived solely from modest retrospective studies. Furthermore, this treatment approach may pose not only logistical challenges for patients but also carry the inherent risk of adverse events, including complications related to vascular access.
INCLISIRAN
Inclisiran is a novel drug for lipid lowering that uses small interfering RNA (siRNA) technology. It is designed to specifically inhibit the production of PCSK9 in the liver via RNA interference, promoting PCSK9 mRNA degradation[81] .
The impact of inclisiran on reducing cholesterol-containing molecules was evaluated in ORION trials. In two phase 3 trials, patients with established ASCVD (ORION-10 trial) and individuals with ASCVD or an equivalent risk (ORION-11 trial) who had elevated LDL cholesterol levels despite taking statins at the maximum dose, saw improved results after receiving inclisiran. After 510 days of treatment, inclisiran led to a 25.6% reduction in Lp(a) levels for ORION-10 and an 18.6% reduction for ORION-11[82].
Whether the reduction in LDL levels translates into clinical benefits in terms of MACEs remains to be determined, although some data appear promising[83]. The ORION-4 trial (NCT03705234) addresses this aspect. Similar uncertainties surrounding inclisiran, as with PCSK9 inhibitors, pertain to whether the mortality-reducing benefit is solely applicable to LDL reduction or whether inclisiran also diminishes Lp(a)-related mortality.
BEMPEDOIC ACID
Bempedoic acid is a novel drug for LDL-C lowering that acts by inhibiting ATP citrate lyase (ACL) in the liver, an enzyme that is featured upstream of HMG-CoA reductase in the cholesterol synthesis pathway, studied within the CLEAR program[84-88].
Bempedoic has the potential to manage forms of dyslipidemia associated with statin intolerance, as it induces a reduction in LDL-C levels without displaying the adverse side effects of muscular damage. Furthermore, bempedoic shows a decrease in the production of acute-phase proteins, particularly
However, in a phase 2 trial, bempedoic acid did not show a significant effect on reducing Lp(a) levels[84]. Likewise, combining bempedoic acid with evolocumab did not yield better results than evolocumab alone in altering the levels of Lp(a).
EMERGING THERAPIES ON LP(A) LEVELS
Traditional drug therapies typically involve the use of small compounds that inhibit enzyme activity or receptor function, as seen in HMG-CoA reductase inhibitors, or antibodies that bind to and deactivate proteins, as seen with PCSK9 inhibitors. These methods may not be suitable for addressing elevated Lp(a) levels due to the absence of enzyme activity or receptor functions in Lp(a); therefore, traditional lipid-lowering drugs can only indirectly contribute to the reduction of circulating Lp(a) levels.
A fundamentally innovative approach involves the utilization of RNA-targeted therapeutics, specifically antisense oligonucleotides (ASO) or small interfering RNAs (siRNA), to block the production of apo(a) mRNA within hepatocytes. Considering the structure and production of the molecule, two potential genes for reducing serum Lp(a) levels are APOB and LPA[89]. Initial research focused on mipomersen, an mRNA inhibitor targeting apo B100, which effectively reduced Lp(a) levels by approximately 75% in Lp(a)-transgenic mice[89]. Similar outcomes were observed in clinical trials involving human subjects, in which mipomersen yielded a significant decrease in Lp(a) levels ranging from 21%-39%[90]. However, these favorable effects come at the expense of a noteworthy risk of hepatic steatosis and liver enzyme abnormalities[91]. Consequently, mipomersen has received approval from the United States Food and Drug Administration (FDA) but not from the European Medicines Agency (EMA).
PELACARSEN
Pelacarsen (previously known as IONIS-APO[a]) is a second-generation antisense oligonucleotide (ASO) currently investigated in several clinical trials. A phase 1 trial conducted with volunteers employing IONIS-APO(a) demonstrated a noteworthy reduction in Lp(a) levels of 77.8%[90].
Subsequently, IONIS-APO(a)-LRx replaced this molecular entity. Although keeping an identical nucleic acid sequence, this compound is designed to target hepatocytes directly by incorporating triantennary
A phase 1/2a randomized, placebo-controlled trial enrolled 58 participants with elevated Lp(a). Subjects in the pelacarsen group exhibited notable Lp(a) reductions, spanning from 26%-92%[93]. In a phase 2 randomized, double-blind, placebo-controlled, dose-finding study involving 286 patients with established CVD and elevated Lp(a) levels of at least 60 mg/mL, pelacarsen was administered at various doses. The study observed significant reductions in Lp(a) concentrations, with mean percentage decreases ranging from 35% to 80%, depending on the dose administered. Notably, the drug exhibited a favorable safety profile, with no significant adverse effects reported[93].
Presently, a phase 3 randomized, double-blind, placebo-controlled multicenter trial named HORIZON (NCT04023552) is currently in progress. The objective of this study is to evaluate the effectiveness of pelacarsen in reducing CV risk in patients with established CVD and elevated levels of Lp(a). The trial involves patients with Lp(a) levels exceeding 70 mg/dL who are already receiving optimal therapy to lower their LDL cholesterol levels. Additionally, these patients have a history of myocardial infarction, ischemic stroke, or significant symptomatic peripheral artery disease. Participants were randomly assigned to receive either 80 mg of pelacarsen via subcutaneous injection monthly or a placebo. The study's primary outcomes are focused on assessing CV mortality, non-fatal myocardial infarctions, non-fatal strokes, and the need for urgent coronary revascularizations.
OLPASIRAN
In a similar fashion to pelacarsen, olpasiran is a hepatocyte-selective siRNA designed to target the LPA gene. A phase 1 clinical trial was conducted to assess the safety, tolerability, pharmacokinetics, and pharmacodynamics of single doses of olpasiran compared to placebo in patients with elevated Lp(a) levels. The patients were grouped into different cohorts based on their Lp(a) concentrations: ≥ 70 nmol/L
A phase 2 randomized, double-blind, placebo-controlled, dose-finding trial named OCEAN[a]-DOSE evaluated the effects of olpasiran in 281 patients with established CVD and elevated Lp(a) levels exceeding 150 nmol/L (60 mg/dL). The median Lp(a) concentration at baseline was 260.3 nmol per liter (104 mg/dL). Most of the patients had baseline LDL-C levels that were considered low (median: 67.5 mg/dL) and were already taking medication to lower their LDL-C levels, with 88% on statins and 23% on PCSK9 inhibitors. Despite this background therapy, olpasiran significantly reduced Lp(a) concentrations, with reductions ranging from -70.5% to -100.5% across different doses. The incidence of adverse events was generally similar between the olpasiran and placebo groups, with the most common adverse events related to olpasiran being injection site reactions[95].
A phase 3 randomized, double-blind, placebo-controlled multicenter study named OCEAN(a)-Outcomes Trial is currently ongoing (NCT05581303). This study plans to include 6,000 participants aged 18 to 85 with elevated Lp(a) levels of at least 200 nmol/L (80 mg/dL). The participants will have a history of myocardial infarction and/or coronary revascularization. The primary endpoints of the study are time to CHD death, myocardial infarction, or urgent coronary revascularization.
MUVALAPLIN
Muvalalin is the first oral medication specifically designed to reduce Lp(a) levels by interfering with the initial noncovalent interaction between apo(a) and apo B100. By preventing the formation of Lp(a) through the inhibition of this disulfide bond, Muvalalin mimics genetic variations of apo(a) that are unable to associate with apo B100, resulting in lowered Lp(a) levels[96].
In a recent phase 1 randomized, double-blind trial involving 114 healthy individuals with elevated Lp(a) levels (≥ 30 mg/dL), muvalaplin was administered in a single daily dose over 14 days, in ascending increments ranging from 30 mg to 800 mg. The results were promising, with an average reduction in Lp(a) levels between 63% and 65%. Remarkably, 93% of the participants exhibited Lp(a) levels lower than
CONCLUSIONS
This comprehensive review underscores the critical role of Lp(a) in the landscape of CV health. The understanding of this enigmatic lipoprotein has evolved significantly since its discovery by Kåre Berg in 1963. Lp(a) is now recognized as a formidable and heritable CV risk factor, with a pivotal role in ASCVD, AVS, and overall CV and all-cause mortality. Genetic determinants, particularly variations in the LPA gene and its coding sequences, have emerged as key factors influencing Lp(a) levels across different populations. Additionally, the interplay of ethnicity, sex, and hormonal changes further modulate Lp(a) concentrations.
Despite this substantial progress, many questions remain unanswered regarding the precise physiological functions and pathological implications of Lp(a). Notably, its involvement in inflammation and its controversial role in thrombosis warrant further investigation. The ongoing development of therapeutic interventions, including pharmacological agents and apheresis, offers hope for individuals with elevated Lp(a) levels. As research continues to unravel the complexities of Lp(a), it is imperative that clinicians and researchers remain vigilant in their pursuit of innovative strategies to mitigate the CV risks associated with this intriguing lipoprotein.
DECLARATIONS
Authors’ contributions
Made substantial contributions to the conception and design of the study and performed data analysis and interpretation: Cesaro A, Calabrò P, Acerbo V, Scherillo G, De Michele G, Fimiani F, Signore G, Panico D, Porcelli G, Scialla F
Performed data acquisition and provided administrative, technical, and material support: Raucci G, Rotolo FP, Tontodonato M, De Pasquale A, Vergara A, Lisi D, Mensorio MM
Availability of data and materials
Not applicable.
Financial support and sponsorship
None.
Conflicts of interest
All authors declared that there are no conflicts of interest.
Ethical approval and consent to participate
Not applicable.
Consent for publication
Not applicable.
Copyright
© The Author(s) 2024.
REFERENCES
1. Berg K. A new serum type system in man-the Lp system. Acta Pathol Microbiol Scand 1963;59:369-82.
2. Kronenberg F, Mora S, Stroes ESG, et al. Lipoprotein(a) in atherosclerotic cardiovascular disease and aortic stenosis: a European atherosclerosis society consensus statement. Eur Heart J 2022;43:3925-46.
3. Lau F, Giugliano RP. Lipoprotein(a) and its significance in cardiovascular disease: a review. JAMA Cardiol 2022;7:760-9.
4. Schmidt K, Noureen A, Kronenberg F, Utermann G. Structure, function, and genetics of lipoprotein(a). J Lipid Res 2016;57:1339-59.
5. Kraft HG, Menzel HJ, Hoppichler F, Vogel W, Utermann G. Changes of genetic apolipoprotein phenotypes caused by liver transplantation. Implications for apolipoprotein synthesis. J Clin Invest 1989;83:137-42.
6. Tsimikas S. A test in context: lipoprotein(a): diagnosis, prognosis, controversies, and emerging therapies. J Am Coll Cardiol 2017;69:692-711.
7. Kratzin H, Armstrong VW, Niehaus M, Hilschmann N, Seidel D. Structural relationship of an apolipoprotein(a) phenotype (570 kDa) to plasminogen: homologous kringle domains are linked by carbohydrate-rich regions. Biol Chem Hoppe Seyler 1987;368:1533-44.
8. Kalaivani V, Jaleel A. Apolipoprotein(a), an enigmatic anti-angiogenic glycoprotein in human plasma: a curse or cure? Pharmacol Res 2020;158:104858.
9. Kalaivani V, Krishna MS, Kumar AA, Satheesh G, Jaleel A. O-glycan structures in apo(a) subunit of human lipoprotein(a) suppresses the pro-angiogenic activity of galectin-1 on human umbilical vein endothelial cells. FASEB J 2023;37:e22813.
10. White AL, Guerra B, Lanford RE. Influence of allelic variation on apolipoprotein(a) folding in the endoplasmic reticulum. J Biol Chem 1997;272:5048-55.
11. Rader DJ, Cain W, Ikewaki K, et al. The inverse association of plasma lipoprotein(a) concentrations with apolipoprotein(a) isoform size is not due to differences in Lp(a) catabolism but to differences in production rate. J Clin Invest 1994;93:2758-63.
12. Reyes-Soffer G, Ginsberg HN, Berglund L, et al. Lipoprotein(a): a genetically determined, causal, and prevalent risk factor for atherosclerotic cardiovascular disease: a scientific statement from the american heart association. Arterioscler Thromb Vasc Biol 2022;42:e48-60.
13. Enkhmaa B, Petersen KS, Kris-Etherton PM, Berglund L. Diet and Lp(a): does dietary change modify residual cardiovascular risk conferred by Lp(a)? Nutrients 2020;12:2024.
14. Hopewell JC, Haynes R, Baigent C. The role of lipoprotein(a) in chronic kidney disease. J Lipid Res 2018;59:577-85.
16. Mack S, Coassin S, Rueedi R, et al. A genome-wide association meta-analysis on lipoprotein(a) concentrations adjusted for apolipoprotein(a) isoforms. J Lipid Res 2017;58:1834-44.
17. Ferreira H, Costa E, Vieira E, et al. Pentanucleotide repeat (TTTTA)n polymorphism in the 5′ control region of the apoliprotein (A) gene and atherothrombotic serum lipoprotein (A) concentration, in a pediatric population. Haematologica 2003;88:ELT07.
18. Ichinose A, Kuriyama M. Detection of polymorphisms in the 5′-flanking region of the gene for apolipoprotein(a). Biochem Biophys Res Commun 1995;209:372-8.
19. Ichinose A. Characterization of the apolipoprotein(a) gene. Biochem Biophys Res Commun 1995;209:365-71.
20. Suzuki K, Kuriyama M, Saito T, Ichinose A. Plasma lipoprotein(a) levels and expression of the apolipoprotein(a) gene are dependent on the nucleotide polymorphisms in its 5′-flanking region. J Clin Invest 1997;99:1361-6.
21. Puckey LH, Lawn RM, Knight BL. Polymorphisms in the apolipoprotein(a) gene and their relationship to allele size and plasma lipoprotein(a) concentration. Hum Mol Genet 1997;6:1099-107.
22. Ogorelkova M, Kraft HG, Ehnholm C, Utermann G. Single nucleotide polymorphisms in exons of the apo(a) kringles IV types 6 to 10 domain affect Lp(a) plasma concentrations and have different patterns in Africans and Caucasians. Hum Mol Genet 2001;10:815-24.
23. Prins J, Leus FR, Bouma BN, van Rijn HJ. The identification of polymorphisms in the coding region of the apolipoprotein (a) gene-association with earlier identified polymorphic sites and influence on the lipoprotein (a) concentration. Thromb Haemost 1999;82:1709-17.
24. Clarke R, Peden JF, Hopewell JC, et al. Genetic variants associated with Lp(a) lipoprotein level and coronary disease. N Engl J Med 2009;361:2518-28.
25. Said MA, Yeung MW, van de Vegte YJ, et al. Genome-wide association study and identification of a protective missense variant on lipoprotein(a) concentration: protective missense variant on lipoprotein(a) concentration-brief report. Arterioscler Thromb Vasc Biol 2021;41:1792-800.
26. Hoekstra M, Chen HY, Rong J, et al. Genome-wide association study highlights APOH as a novel locus for lipoprotein(a) levels-brief report. Arterioscler Thromb Vasc Biol 2021;41:458-64.
27. Virani SS, Brautbar A, Davis BC, et al. Associations between lipoprotein(a) levels and cardiovascular outcomes in black and white subjects: the atherosclerosis risk in communities (ARIC) study. Circulation 2012;125:241-9.
28. Patel AP, Wang M, Pirruccello JP, et al. Lp(a) (lipoprotein[a]) concentrations and incident atherosclerotic cardiovascular disease: new insights from a large national biobank. Arterioscler Thromb Vasc Biol 2021;41:465-74.
29. Derby CA, Crawford SL, Pasternak RC, Sowers M, Sternfeld B, Matthews KA. Lipid changes during the menopause transition in relation to age and weight: the study of women’s health across the nation. Am J Epidemiol 2009;169:1352-61.
30. Cesaro A, Schiavo A, Moscarella E, et al. Lipoprotein(a): a genetic marker for cardiovascular disease and target for emerging therapies. J Cardiovasc Med 2021;22:151-61.
31. Banerjee D, Wong EC, Shin J, Fortmann SP, Palaniappan L. Racial and ethnic variation in lipoprotein(a) levels among Asian Indian and Chinese patients. J Lipids 2011;2011:291954.
32. Kronenberg F, Mora S, Stroes ESG, et al. Frequent questions and responses on the 2022 lipoprotein(a) consensus statement of the European atherosclerosis society. Atherosclerosis 2023;374:107-20.
33. Madsen CM, Kamstrup PR, Langsted A, Varbo A, Nordestgaard BG. Lipoprotein(a)-lowering by 50 mg/dL (105 nmol/L) may be needed to reduce cardiovascular disease 20% in secondary prevention: a population-based study. Arterioscler Thromb Vasc Biol 2020;40:255-66.
34. Kamstrup PR, Tybjærg-Hansen A, Nordestgaard BG. Extreme lipoprotein(a) levels and improved cardiovascular risk prediction. J Am Coll Cardiol 2013;61:1146-56.
35. Rikhi R, Hammoud A, Ashburn N, et al. Relationship of low-density lipoprotein-cholesterol and lipoprotein(a) to cardiovascular risk: the multi-ethnic study of atherosclerosis (MESA). Atherosclerosis 2022;363:102-8.
36. Yao Y, Liu J, Wang B, et al. Baseline low-density-lipoprotein cholesterol modifies the risk of all-cause death associated with elevated lipoprotein(a) in coronary artery disease patients. Front Cardiovasc Med 2021;8:817442.
37. Di Fusco SA, Maggioni AP, Scicchitano P, Zuin M, D’Elia E, Colivicchi F. Lipoprotein(a), inflammation, and atherosclerosis. J Clin Med 2023;12:2529.
38. Verbeek R, Hoogeveen RM, Langsted A, et al. Cardiovascular disease risk associated with elevated lipoprotein(a) attenuates at low low-density lipoprotein cholesterol levels in a primary prevention setting. Eur Heart J 2018;39:2589-96.
39. Afshar M, Pilote L, Dufresne L, Engert JC, Thanassoulis G. Lipoprotein(a) interactions with low-density lipoprotein cholesterol and other cardiovascular risk factors in premature acute coronary syndrome (ACS). J Am Heart Assoc 2016;5:e003012.
40. Tromp TR, Ibrahim S, Nurmohamed NS, et al. Use of lipoprotein(a) to improve diagnosis and management in clinical familial hypercholesterolemia. Atherosclerosis 2023;365:27-33.
41. Kronenberg F, Mora S, Stroes ESG. Consensus and guidelines on lipoprotein(a) - seeing the forest through the trees. Curr Opin Lipidol 2022;33:342-52.
42. Kronenberg F. Human genetics and the causal role of lipoprotein(a) for various diseases. Cardiovasc Drugs Ther 2016;30:87-100.
43. Sandholzer C, Saha N, Kark JD, et al. Apo(a) isoforms predict risk for coronary heart disease. A study in six populations. Arterioscler Thromb 1992;12:1214-26.
44. Coassin S, Kronenberg F. Lipoprotein(a) beyond the kringle IV repeat polymorphism: the complexity of genetic variation in the LPA gene. Atherosclerosis 2022;349:17-35.
45. Lampsas S, Xenou M, Oikonomou E, et al. Lipoprotein(a) in Atherosclerotic diseases: from pathophysiology to diagnosis and treatment. Molecules 2023;28:969.
46. Schnitzler JG, Hoogeveen RM, Ali L, et al. Atherogenic lipoprotein(a) increases vascular glycolysis, thereby facilitating inflammation and leukocyte extravasation. Circ Res 2020;126:1346-59.
47. Bartrons R, Rodríguez-García A, Simon-Molas H, Castaño E, Manzano A, Navarro-Sabaté À. The potential utility of PFKFB3 as a therapeutic target. Expert Opin Ther Targets 2018;22:659-74.
48. Tsimikas S, Bergmark C, Beyer RW, et al. Temporal increases in plasma markers of oxidized low-density lipoprotein strongly reflect the presence of acute coronary syndromes. J Am Coll Cardiol 2003;41:360-70.
49. Bergmark C, Dewan A, Orsoni A, et al. A novel function of lipoprotein [a] as a preferential carrier of oxidized phospholipids in human plasma. J Lipid Res 2008;49:2230-9.
50. Tsimikas S, Tsironis LD, Tselepis AD. New insights into the role of lipoprotein(a)-associated lipoprotein-associated phospholipase A2 in atherosclerosis and cardiovascular disease. Arterioscler Thromb Vasc Biol 2007;27:2094-9.
51. Tsimikas S, Witztum JL. The role of oxidized phospholipids in mediating lipoprotein(a) atherogenicity. Curr Opin Lipidol 2008;19:369-77.
52. Després AA, Perrot N, Poulin A, et al. Lipoprotein(a), oxidized phospholipids, and aortic valve microcalcification assessed by 18F-sodium fluoride positron emission tomography and computed tomography. CJC Open 2019;1:131-40.
53. Kaiser Y, Singh SS, Zheng KH, et al. Lipoprotein(a) is robustly associated with aortic valve calcium. Heart 2021;107:1422-8.
54. Kaiser Y, Nurmohamed NS, Kroon J, et al. Lipoprotein(a) has no major impact on calcification activity in patients with mild to moderate aortic valve stenosis. Heart 2022;108:61-6.
55. Kaiser Y, van der Toorn JE, Singh SS, et al. Lipoprotein(a) is associated with the onset but not the progression of aortic valve calcification. Eur Heart J 2022;43:3960-7.
56. Zheng KH, Tsimikas S, Pawade T, et al. Lipoprotein(a) and oxidized phospholipids promote valve calcification in patients with aortic stenosis. J Am Coll Cardiol 2019;73:2150-62.
57. Bouchareb R, Mahmut A, Nsaibia MJ, et al. Autotaxin derived from lipoprotein(a) and valve interstitial cells promotes inflammation and mineralization of the aortic valve. Circulation 2015;132:677-90.
58. Federico L, Pamuklar Z, Smyth SS, Morris AJ. Therapeutic potential of autotaxin/lysophospholipase d inhibitors. Curr Drug Targets 2008;9:698-708.
59. Nordestgaard BG, Langsted A. Lipoprotein(a) as a cause of cardiovascular disease: insights from epidemiology, genetics, and biology. J Lipid Res 2016;57:1953-75.
60. Boffa MB. Beyond fibrinolysis: the confounding role of Lp(a) in thrombosis. Atherosclerosis 2022;349:72-81.
61. Boffa MB, Marar TT, Yeang C, et al. Potent reduction of plasma lipoprotein(a) with an antisense oligonucleotide in human subjects does not affect ex vivo fibrinolysis. J Lipid Res 2019;60:2082-9.
62. Sultan SM, Schupf N, Dowling MM, Deveber GA, Kirton A, Elkind MS. Review of lipid and lipoprotein(a) abnormalities in childhood arterial ischemic stroke. Int J Stroke 2014;9:79-87.
63. Tsimikas S. Elevated lipoprotein(a) and the risk of stroke in children, young adults, and the elderly. Eur Heart J 2021;42:2197-200.
64. Dzobo KE, Kraaijenhof JM, Stroes ESG, Nurmohamed NS, Kroon J. Lipoprotein(a): an underestimated inflammatory mastermind. Atherosclerosis 2022;349:101-9.
65. Puri R, Nissen SE, Arsenault BJ, et al. Effect of C-reactive protein on lipoprotein(a)-associated cardiovascular risk in optimally treated patients with high-risk vascular disease: a prespecified secondary analysis of the ACCELERATE trial. JAMA Cardiol 2020;5:1136-43.
66. Tsimikas S, Gordts PLSM, Nora C, Yeang C, Witztum JL. Statin therapy increases lipoprotein(a) levels. Eur Heart J 2020;41:2275-84.
67. de Boer LM, Oorthuys AOJ, Wiegman A, et al. Statin therapy and lipoprotein(a) levels: a systematic review and meta-analysis. Eur J Prev Cardiol 2022;29:779-92.
68. Yahya R, Berk K, Verhoeven A, et al. Statin treatment increases lipoprotein(a) levels in subjects with low molecular weight apolipoprotein(a) phenotype. Atherosclerosis 2019;289:201-5.
69. Sahebkar A, Simental-Mendía LE, Pirro M, et al. Impact of ezetimibe on plasma lipoprotein(a) concentrations as monotherapy or in combination with statins: a systematic review and meta-analysis of randomized controlled trials. Sci Rep 2018;8:17887.
70. Boden WE, Probstfield JL, Anderson T, et al. Niacin in patients with low HDL cholesterol levels receiving intensive statin therapy. N Engl J Med 2011;365:2255-67.
71. Landray MJ, Haynes R, Hopewell JC, et al. Effects of extended-release niacin with laropiprant in high-risk patients. N Engl J Med 2014;371:203-12.
72. Thomas T, Zhou H, Karmally W, et al. CETP (cholesteryl ester transfer protein) inhibition with anacetrapib decreases production of lipoprotein(a) in mildly hypercholesterolemic subjects. Arterioscler Thromb Vasc Biol 2017;37:1770-5.
73. Nicholls SJ, Ruotolo G, Brewer HB, et al. Evacetrapib alone or in combination with statins lowers lipoprotein(a) and total and small LDL particle concentrations in mildly hypercholesterolemic patients. J Clin Lipidol 2016;10:519-27.e4.
74. Nicholls SJ, Ditmarsch M, Kastelein JJ, et al. Lipid lowering effects of the CETP inhibitor obicetrapib in combination with high-intensity statins: a randomized phase 2 trial. Nat Med 2022;28:1672-8.
75. Sabatine MS, Giugliano RP, Keech AC, et al. Evolocumab and clinical outcomes in patients with cardiovascular disease. N Engl J Med 2017;376:1713-22.
76. Schwartz GG, Steg PG, Szarek M, et al. Alirocumab and cardiovascular outcomes after acute coronary syndrome. N Engl J Med 2018;379:2097-107.
77. Schwartz GG, Szarek M, Bittner VA, et al. Lipoprotein(a) and benefit of PCSK9 inhibition in patients with nominally controlled LDL cholesterol. J Am Coll Cardiol 2021;78:421-33.
78. O’Donoghue ML, Fazio S, Giugliano RP, et al. Lipoprotein(a), PCSK9 inhibition, and cardiovascular risk. Circulation 2019;139:1483-92.
79. Moriarty PM, Gray JV, Gorby LK. Lipoprotein apheresis for lipoprotein(a) and cardiovascular disease. J Clin Lipidol 2019;13:894-900.
80. Leebmann J, Roeseler E, Julius U, et al. Lipoprotein apheresis in patients with maximally tolerated lipid-lowering therapy, lipoprotein(a)-hyperlipoproteinemia, and progressive cardiovascular disease: prospective observational multicenter study. Circulation 2013;128:2567-76.
81. Cupido AJ, Kastelein JJP. Inclisiran for the treatment of hypercholesterolaemia: implications and unanswered questions from the ORION trials. Cardiovasc Res 2020;116:e136-9.
82. Ray KK, Wright RS, Kallend D, et al. Two phase 3 trials of inclisiran in patients with elevated LDL cholesterol. N Engl J Med 2020;382:1507-19.
83. Ray KK, Raal FJ, Kallend DG, et al. Inclisiran and cardiovascular events: a patient-level analysis of phase III trials. Eur Heart J 2023;44:129-38.
84. Thompson PD, Rubino J, Janik MJ, et al. Use of ETC-1002 to treat hypercholesterolemia in patients with statin intolerance. J Clin Lipidol 2015;9:295-304.
85. Ballantyne CM, Banach M, Mancini GBJ, et al. Efficacy and safety of bempedoic acid added to ezetimibe in statin-intolerant patients with hypercholesterolemia: a randomized, placebo-controlled study. Atherosclerosis 2018;277:195-203.
86. Ray KK, Bays HE, Catapano AL, et al. Safety and efficacy of bempedoic acid to reduce LDL cholesterol. N Engl J Med 2019;380:1022-32.
87. Goldberg AC, Leiter LA, Stroes ESG, et al. Effect of bempedoic acid vs placebo added to maximally tolerated statins on low-density lipoprotein cholesterol in patients at high risk for cardiovascular disease: the CLEAR wisdom randomized clinical trial. JAMA 2019;322:1780-8.
88. Laufs U, Banach M, Mancini GBJ, et al. Efficacy and safety of bempedoic acid in patients with hypercholesterolemia and statin intolerance. J Am Heart Assoc 2019;8:e011662.
89. Merki E, Graham MJ, Mullick AE, et al. Antisense oligonucleotide directed to human apolipoprotein B-100 reduces lipoprotein(a) levels and oxidized phospholipids on human apolipoprotein B-100 particles in lipoprotein(a) transgenic mice. Circulation 2008;118:743-53.
90. Santos RD, Raal FJ, Catapano AL, Witztum JL, Steinhagen-Thiessen E, Tsimikas S. Mipomersen, an antisense oligonucleotide to apolipoprotein B-100, reduces lipoprotein(a) in various populations with hypercholesterolemia: results of 4 phase III trials. Arterioscler Thromb Vasc Biol 2015;35:689-99.
91. Tsimikas S, Viney NJ, Hughes SG, et al. Antisense therapy targeting apolipoprotein(a): a randomised, double-blind, placebo-controlled phase 1 study. Lancet 2015;386:1472-83.
92. Yu RZ, Graham MJ, Post N, et al. Disposition and pharmacology of a GalNAc3-conjugated ASO targeting human lipoprotein(a) in mice. Mol Ther Nucleic Acids 2016;5:e317.
93. Tsimikas S, Karwatowska-Prokopczuk E, Gouni-Berthold I, et al. Lipoprotein(a) reduction in persons with cardiovascular disease. N Engl J Med 2020;382:244-55.
94. Koren MJ, Moriarty PM, Baum SJ, et al. Preclinical development and phase 1 trial of a novel siRNA targeting lipoprotein(a). Nat Med 2022;28:96-103.
95. O’Donoghue ML, Rosenson RS, Gencer B, et al. Small interfering RNA to reduce lipoprotein(a) in cardiovascular disease. N Engl J Med 2022;387:1855-64.
Cite This Article
Export citation file: BibTeX | EndNote | RIS
OAE Style
Cesaro A, Scherillo G, De Michele G, Acerbo V, Signore G, Panico D, Porcelli G, Scialla F, Raucci G, Rotolo FP, Tontodonato M, De Pasquale A, Vergara A, Lisi D, Mensorio MM, Fimiani F, Calabrò P. Circulating culprit or therapeutic bullseye: lipoprotein(a) in cardiovascular risk assessment and novel therapeutic prospects. J Cardiovasc Aging 2024;4:10. http://dx.doi.org/10.20517/jca.2023.35
AMA Style
Cesaro A, Scherillo G, De Michele G, Acerbo V, Signore G, Panico D, Porcelli G, Scialla F, Raucci G, Rotolo FP, Tontodonato M, De Pasquale A, Vergara A, Lisi D, Mensorio MM, Fimiani F, Calabrò P. Circulating culprit or therapeutic bullseye: lipoprotein(a) in cardiovascular risk assessment and novel therapeutic prospects. The Journal of Cardiovascular Aging. 2024; 4(1): 10. http://dx.doi.org/10.20517/jca.2023.35
Chicago/Turabian Style
Arturo Cesaro, Gianmaria Scherillo, Gianantonio De Michele, Vincenzo Acerbo, Giovanni Signore, Domenico Panico, Gennaro Porcelli, Francesco Scialla, Giuseppe Raucci, Francesco Paolo Rotolo, Marco Tontodonato, Antonio De Pasquale, Andrea Vergara, Danilo Lisi, Mario Massimo Mensorio, Fabio Fimiani, Paolo Calabrò. 2024. "Circulating culprit or therapeutic bullseye: lipoprotein(a) in cardiovascular risk assessment and novel therapeutic prospects" The Journal of Cardiovascular Aging. 4, no.1: 10. http://dx.doi.org/10.20517/jca.2023.35
ACS Style
Cesaro, A.; Scherillo G.; De Michele G.; Acerbo V.; Signore G.; Panico D.; Porcelli G.; Scialla F.; Raucci G.; Rotolo FP.; Tontodonato M.; De Pasquale A.; Vergara A.; Lisi D.; Mensorio MM.; Fimiani F.; Calabrò P. Circulating culprit or therapeutic bullseye: lipoprotein(a) in cardiovascular risk assessment and novel therapeutic prospects. J. Cardiovasc. Aging. 2024, 4, 10. http://dx.doi.org/10.20517/jca.2023.35
About This Article
Copyright
Data & Comments
Data
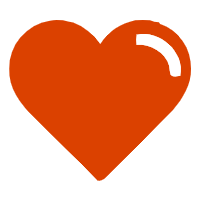
Comments
Comments must be written in English. Spam, offensive content, impersonation, and private information will not be permitted. If any comment is reported and identified as inappropriate content by OAE staff, the comment will be removed without notice. If you have any queries or need any help, please contact us at support@oaepublish.com.