Engineered extracellular vesicles as “supply vehicles” to alleviate type 1 diabetes
Abstract
Recent findings have indicated that the deficiency of inhibitory programmed cell death ligand 1 (PD-L1) and galectin-9 (Gal-9) in pancreatic β-cells is associated with the progression of type 1 diabetes (T1D). This suggests that exogenous PD-L1 and Gal-9 may have promising potential as therapeutics for the treatment of T1D. In light of these reports, a recent work investigated the potential of artificial extracellular vesicles (aEVs) with the presentation of PD-L1 and Gal-9 ligands (PD-L1–Gal-9 aEVs) as a treatment for T1D, with the findings published in Diabetes. Notably, the PD-L1–Gal-9 aEVs demonstrated the capacity to induce apoptosis of T cells and the formation of regulatory T (Treg) cells, thereby maintaining immune tolerance. Furthermore, the in vivo administration of PD-L1–Gal-9 aEVs resulted in a reduction in T cell infiltration in the pancreas, an increase in β-cell integrity protection, a significant decrease in blood glucose levels, and a delay in the progression of T1D. In conclusion, this study proposed an innovative approach to the treatment of T1D progression through the use of immunosuppressive EVs. This highlight provides a comprehensive analysis and discussion of the pivotal findings of this study.
Keywords
MAIN TEXT
Type 1 diabetes (T1D) is an autoimmune disease that is characterized by elevated blood glucose levels. This results from T lymphocyte-caused damage to the β-cells in the pancreas and subsequent insulin secretion failure[1,2]. T1D accounts for 5%-10% of all reported diabetic cases, with a globally increasing incidence[3]. It is postulated that T1D is a highly heterogeneous disease, influenced by a variety of factors, including age, genetic alterations, and environmental influences. Research suggests that pancreatic β-cells and their interactions with immune cells play a pivotal role in the development and pathogenic progression of T1D[4]. Insulin therapy is considered the primary treatment option for T1D, as it has a crucial role in the regulation of glucose intracellular transportation[5-7]. However, insulin therapy is confronted with significant adverse effects, including hypoglycemia and insulin resistance. Considering the interactions between pancreatic β-cells and immune cells, a variety of immunosuppressive therapies have been proposed as potential alternatives for the treatment of T1D[8]. In 2022, the US Food and Drug Administration (FDA) approved teplizumab, a CD3-binding monoclonal antibody, for the prevention of T1D. Nevertheless, teplizumab therapy may result in severe side effects and is currently expensive[9]. Therefore, it is necessary to develop treatment methodologies with enhanced therapeutic effects for the prevention and treatment of T1D.
Recently, nanotechnology-based biomaterials have been employed extensively as therapeutic agents or innovative drug delivery platforms in treating a variety of illnesses, including T1D[10-13]. Among the various types of nanomaterials, extracellular vesicles (EVs), which are nano-sized phospholipid bilayer membrane-enclosed particles secreted by various cell types, have attracted considerable interest for their potential as a next-generation nanomedicine with applications in numerous biomedical fields[14-18]. In particular, it has been demonstrated that EVs derived from various cell resources, including mesenchymal stem cells (MSCs), adipocytes, and endothelial progenitor cells (EPCs), achieved remarkable therapeutic efficacy in the treatment of T1D[19]. For instance, MSC-derived exosomes have been demonstrated to stimulate the regeneration of pancreatic islet cells and insulin secretion by regulating a group of genes[20]. In addition, microvesicles derived from EPCs were shown to facilitate the neoangiogenesis process of the transplanted islet via the delivery of miRNAs[21].
It is noteworthy that EVs can be utilized as drug delivery platforms for various biomolecules, such as nucleic acids, proteins, and chemotherapeutic drugs[22-25]. For example, Wang et al. proposed an inhalable vaccine for the prevention of SARS-CoV-2[26]. The vaccine is developed using lung-derived exosomes with conjugation to the recombinant domains binding to SARS-CoV-2 receptors (RBD). The exosome vaccine was observed to improve the retention rate and time of RBD within the lung, and also inducing systemic mucosal immunity and T cell immunity in mice and hamsters[26,27]. In another report, decoy exosomes modified with GC-enriched tetrahedral DNA nanostructures have been shown to mitigate doxorubicin (DOX)-induced hepatotoxicity by scavenging DOX and polarizing macrophages into the M2 phenotype[28].
Given their remarkable capacity to carry cargo, EVs could also be employed as “supply vehicles” of therapeutic biomolecules in the treatment of T1D. A deficiency in immune checkpoint molecules with pro-inhibitory effects, including programmed cell death ligand 1 (PD-L1) and galectin-9 (Gal-9), has been observed in pancreatic β-cells and has been linked to the progression of T1D[29]. Accordingly, Yang et al. postulated that the delivery of exogenous PD-L1 and Gal-9 to β-cells might result in an immunosuppressive therapeutic effect, thereby alleviating T1D[30]. By leveraging genetic engineering technology, the authors generated Raw264.7 cells overexpressing PD-L1 and Gal-9 molecules on their cell membranes. The overexpression of Gal-9 resulted in the transformation of the Raw264.7 cells to an M2 macrophage phenotype. In addition, genetically engineered Raw264.7 cells were demonstrated to generate artificial extracellular vesicles (aEVs) with high expression levels of PD-L1 and Gal-9 (called PD-L1–Gal-9 aEVs). The developed EV-based nanoplatforms displayed the binding ability to the PD-1 and T-cell immunoglobulin mucin 3 (TIM-3) receptors on the T lymphocytes. The binding is attributed to the interactions between PD-L1 and PD-1, as well as Gal-9 and TIM-3.
The authors showed that the PD-L1–Gal-9 aEVs induced a significant inhibition of the AKT signaling pathway and the induction of T cell apoptosis. Treatment with PD-L1–Gal-9 aEVs resulted in notable expansion of the regulatory T (Treg) cell population. Furthermore, this study provided in vivo evidence of the therapeutic effects of the developed EV-based nanoplatforms. In vivo imaging results demonstrated the distribution of the developed EV-based nanoplatforms in the pancreas following intravenous administration. Moreover, the treatment with PD-L1–Gal-9 aEVs was observed to markedly attenuate T1D progression in a diabetic mouse model, accompanied by elevated levels of C-peptide and IL-10 expression. Additionally, PD-L1–Gal-9 aEVs demonstrated capacity in safeguarding pancreatic β-cells from destruction, impeding the T cell infiltration, and enhancing the population of Treg cells within the pancreatic tissue.
The study collectively presented an aEV-based platform for the delivery of therapeutic factors to pancreatic tissue, with the objective of improving T1D outcomes. The destruction of β-cells in the pancreas tissue by autoreactive T cells represents a critical cause of T1D. The authors demonstrated that the administration of immunosuppressive PD-L1 and Gal-9 represents an efficacious approach to inhibit the infiltration of T cells and alleviate T1D. This study suggested that aEVs could serve as a delivery system for macromolecules, such as proteins, for the treatment of T1D. In light of the benefits associated with aEVs, including high yield, reduced expense, and fewer side effects, aEVs are emerging as a promising therapeutic nanoplatform for the treatment of T1D.
DECLARATIONS
Authors’ contributions
Writing, reviewing, and editing the draft: Wang F, Li Z
Availability of data and materials
Not applicable.
Financial support and sponsorship
None.
Conflicts of interest
All authors declared that there are no conflicts of interest.
Ethical approval and consent to participate
Not applicable.
Consent for publication
Not applicable.
Copyright
© The Author(s) 2024.
REFERENCES
1. Roep BO, Thomaidou S, van Tienhoven R, Zaldumbide A. Type 1 diabetes mellitus as a disease of the β-cell (do not blame the immune system?). Nat Rev Endocrinol. 2021;17:150-61.
2. Zhang J, Lin C, Jin S, et al. The pharmacology and therapeutic role of cannabidiol in diabetes. Exploration. 2023;3:20230047.
3. Mobasseri M, Shirmohammadi M, Amiri T, Vahed N, Hosseini Fard H, Ghojazadeh M. Prevalence and incidence of type 1 diabetes in the world: a systematic review and meta-analysis. Health Promot Perspect. 2020;10:98-115.
4. Li Y, Sun F, Yue TT, et al. Revisiting the antigen-presenting function of β cells in T1D pathogenesis. Front Immunol. 2021;12:690783.
6. Primavera R, Kevadiya BD, Swaminathan G, et al. Emerging nano- and micro-technologies used in the treatment of type-1 diabetes. Nanomaterials. 2020;10:789.
7. Liu J, Zhou Y, Lyu Q, Yao X, Wang W. Targeted protein delivery based on stimuli-triggered nanomedicine. Exploration. 2024;4:20230025.
8. Lernmark A, Larsson HE. Immune therapy in type 1 diabetes mellitus. Nat Rev Endocrinol. 2013;9:92-103.
9. Goldman JD, Choi H. Teplizumab: the first treatment to delay the progression of type 1 diabetes. Clin Diabetes. 2023;41:474-6.
10. Veiseh O, Tang BC, Whitehead KA, Anderson DG, Langer R. Managing diabetes with nanomedicine: challenges and opportunities. Nat Rev Drug Discov. 2015;14:45-57.
11. Jing Z, Li Y, Ma Y, Zhang X, Liang X, Zhang X. Leverage biomaterials to modulate immunity for type 1 diabetes. Front Immunol. 2022;13:997287.
12. Feng C, Tan P, Nie G, Zhu M. Biomimetic and bioinspired nano-platforms for cancer vaccine development. Exploration. 2023;3:20210263.
13. Dai H, Fan Q, Wang C. Recent applications of immunomodulatory biomaterials for disease immunotherapy. Exploration. 2022;2:20210157.
14. Zhang K, Cheng K. Stem cell-derived exosome versus stem cell therapy. Nat Rev Bioeng. 2023;Online ahead of print.
15. Cheng K, Kalluri R. Guidelines for clinical translation and commercialization of extracellular vesicles and exosomes based therapeutics. Extracellular Vesicle. 2023;2:100029.
16. Li L, Wang F, Zhu D, Hu S, Cheng K, Li Z. Engineering exosomes and exosome-like nanovesicles for improving tissue targeting and retention. Fundamental Research. 2024;Online ahead of print.
17. Zhu D, Cheng K. Cardiac cell therapy for heart repair: should the cells be left out? Cells. 2021;10:641.
18. Ding Y, Wang Y, Hu Q. Recent advances in overcoming barriers to cell-based delivery systems for cancer immunotherapy. Exploration. 2022;2:20210106.
19. Soltani S, Mansouri K, Emami Aleagha MS, et al. Extracellular vesicle therapy for type 1 diabetes. Front Immunol. 2022;13:865782.
20. Sabry D, Marzouk S, Zakaria R, Ibrahim HA, Samir M. The effect of exosomes derived from mesenchymal stem cells in the treatment of induced type 1 diabetes mellitus in rats. Biotechnol Lett. 2020;42:1597-610.
21. Cantaluppi V, Biancone L, Figliolini F, et al. Microvesicles derived from endothelial progenitor cells enhance neoangiogenesis of human pancreatic islets. Cell Transplant. 2012;21:1305-20.
22. Liu M, Hu S, Yan N, Popowski KD, Cheng K. Inhalable extracellular vesicle delivery of IL-12 mRNA to treat lung cancer and promote systemic immunity. Nat Nanotechnol. 2024;19:565-75.
23. Liu H, Zhang X, Zhang M, et al. Mesenchymal stem cell derived exosomes repair uterine injury by targeting transforming growth factor-β signaling. ACS Nano. 2024;18:3509-19.
24. Popowski KD, López de Juan Abad B, George A, et al. Inhalable exosomes outperform liposomes as mRNA and protein drug carriers to the lung. Extracell Vesicle. 2022;1:100002.
25. Zhu D, Liu S, Huang K, et al. Intrapericardial exosome therapy dampens cardiac injury via activating Foxo3. Circ Res. 2022;131:e135-50.
26. Wang Z, Popowski KD, Zhu D, et al. Exosomes decorated with a recombinant SARS-CoV-2 receptor-binding domain as an inhalable COVID-19 vaccine. Nat Biomed Eng. 2022;6:791-805.
27. Popowski KD, Dinh PC, George A, Lutz H, Cheng K. Exosome therapeutics for COVID-19 and respiratory viruses. View. 2021;2:20200186.
28. Fan M, Li H, Shen D, et al. Decoy exosomes offer protection against chemotherapy-induced toxicity. Adv Sci. 2022;9:e2203505.
29. Ding JT, Yang KP, Lin KL, Cao YK, Zou F. Mechanisms and therapeutic strategies of immune checkpoint molecules and regulators in type 1 diabetes. Front Endocrinol. 2022;13:1090842.
Cite This Article

How to Cite
Download Citation
Export Citation File:
Type of Import
Tips on Downloading Citation
Citation Manager File Format
Type of Import
Direct Import: When the Direct Import option is selected (the default state), a dialogue box will give you the option to Save or Open the downloaded citation data. Choosing Open will either launch your citation manager or give you a choice of applications with which to use the metadata. The Save option saves the file locally for later use.
Indirect Import: When the Indirect Import option is selected, the metadata is displayed and may be copied and pasted as needed.
About This Article
Copyright
Data & Comments
Data
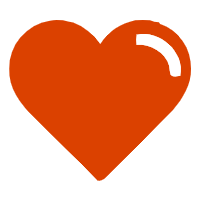
Comments
Comments must be written in English. Spam, offensive content, impersonation, and private information will not be permitted. If any comment is reported and identified as inappropriate content by OAE staff, the comment will be removed without notice. If you have any queries or need any help, please contact us at [email protected].