Gene therapy for the heart: encapsulated viruses to the rescue
Abstract
This commentary provides an in-depth analysis and perspective on the pioneering research article titled ‘Extracellular Vesicle-Encapsulated Adeno-Associated Viruses for Therapeutic Gene Delivery to the Heart’. The original study explores the innovative use of extracellular vesicle-encapsulated AAVs (EV-AAV-6 and -9) as a superior gene-delivery approach for cardiomyocytes (CMs), which not only provides increased AAV neutralizing antibody (NAb) resistance but also has implications for increased gene delivery efficacy to ischemic hearts. This study examined the efficacy of EVs isolated from the conditioned medium of AAV-6 and -9 producing HEK293T cells in combinatorial in vitro and in vivo model systems in comparison to free AAVs in the presence of the NAbs. This commentary highlights the key findings, discusses potential implications, limitations, and suggests future directions for research in this evolving field.
Keywords
Heart disease is the leading cause of death in the United States. Cellular therapies have emerged as promising modalities for patients afflicted with heart disease[1,2]. Extracellular vesicles (EVs) - nano-sized (30-200 nm) cell membrane-derived spherical structures, released by various cell types, are known to shuttle bioactive cargoes to recipient cells. In recent times, EVs have been increasingly leveraged for therapeutic use in the treatment of several diseases[3,4,5]. Along these lines, an elegant study by Cambier et al. demonstrated the efficacy of cardiosphere-derived EVs carrying the Y-RNA (EV-YF1), in reducing the size of the myocardial infarct via modulation of interleukin (IL)-10 expression[6]. In addition, several other myocardial gene transfer strategies have also been developed utilizing the Adeno-Associated Vectors (AAVs), primarily owing to their attributes of non-pathogenicity and enhanced transgene expression[7-13]. A key limitation in the success of AAV strategies, however, has been the presence in the host of the AAV neutralizing antibodies (NAbs), which negates the efficacy of AAV gene therapy[14-17]. To circumvent this roadblock, recent years have seen the use of EV-entrapped AAVs as a promising gene therapy approach for counteracting the effects of AAV NAbs. While such approaches have been developed, one caveat with these has been the presence of free AAVs, which are also secreted along with the EV-AAVs from the conditioned media of the AAV-producing cells. The presence of these free AAVs, in turn, results in decreased EV-AAV dosing, lowering their NAb resistance while also inducing deleterious side effects of free AAVs. There is thus an urgent need to utilize highly purified and characterized EV-AAVs devoid of free AAVs for gene therapy approaches.
The study by Li et al. makes a pivotal contribution to the field of gene therapy by demonstrating the use of purified EV-AAVs as potential cardiac gene therapeutic carriers for enhancing long-term gene delivery to the heart. This study provides the functional benefits and mechanisms underlying the EV-AAV approach. Herein, the authors optimized the EV-AAV purification strategy, and characterized in-depth the EV-AAVs with validation in five different in vitro and in vivo model systems, establishing cardiotropism and higher uptake and therapeutic efficacy of this vector. Specifically, the authors tested the efficacy of delivering SERCA2A as a therapeutic target via EV-AAVs (AAV-6 and AAV-9) both in the human left ventricular CMs as well as induced human pluripotent stem cell (hiPSC)-derived CMs. This was also validated in vivo in murine hearts, wherein the therapeutic potential of EV-AAV-SERCA2A was compared with the AAV-SERCA2A delivery in a preimmunized mouse model of myocardial infarction. This study effectively demonstrated that EV-AAV-SERCA2A not only effectively transduced the therapeutic gene into the CMs, despite the presence of NAbs, but that such an approach was also effective in improving the cardiac function, and remodeling in mice with myocardial infarct. The present study emphasizes the potential clinical translation of such an approach for AAV NAb+ patients with heart failure, even in treated patients. This sets the stage for future refinement and development of therapeutic, adjunctive therapies for heart failure patients with AA NAbs[18]. This study also delineated the uptake of EV-AAVs into the acidic subcellular compartments such as late endosomes and lysosomes, which, in turn, could release the AAVs from EV-AAVs, and thus facilitate nuclear entry and gene expression.
While the authors have also looked at the non-cardiomyocyte cells, one of the limitations of this study is the possible off-target effects of such an approach. Despite the advantages of AAV/EV-AAV vectors in localized intramyocardial delivery, it is not fraught without off-target delivery to organs such as the liver. The use of engineered AAVs88 containing EV-AAVs, which possess enhanced cardiac or muscle delivery potency, could thus prove valuable as an effective approach for translational studies. Although the authors demonstrated that EV-AAVs expressed increased numbers of transgenes in CMs compared to non-cardiomyocytes (NMs), it would have behooved the authors to provide some discussion on the possible off-target effects of such an approach, specifically for clinical translational ramifications. Additionally, as rightly pointed out by the authors, since AAVR could likely be a common receptor for both AAVs and EV-AAVs, it would be necessary for EV-AAVs to undergo further purification to remove traces of AAV contaminants prior to moving this approach into preclinical and/or clinical applications. It would have been important for the authors to have also included the rationale for the choice of AAV-6 over AAV-9 in this study[18]. Furthermore, there is no mention of how the EVs from HEK cells can target CMs efficiently. One speculates if there is a specificity of the HEK EVs for CMs or that just a small proportion of the injected EVs actually reach the heart. It is essential to understand what proportion of EVs reach the heart. The authors’ thoughts on the biodistribution of these EVs, implications for targeted therapy, and the next steps critical for moving the product into the clinical area would benefit the readers.
Future studies should be aimed at examining the efficacy of various routes of delivery of these therapeutic EVs. Understanding the half-life of these therapeutic EVs and the stability of the gene expression in an organism would be the next step before embarking on human clinical trials. Combining the delivery of several other target genes would also be the next step as an effective delivery approach.
Overall, the research article marks a significant advancement in the field of gene therapy by introducing the integration of two powerful vector systems - EV & AAVs and holds promise for addressing longstanding challenges associated with traditional AAV delivery methods. Although, current research suggests that small EVs secreted from the AAV-producing HEK293T cells can effectively deliver intact AAVs in several organ systems such as the nervous system, inner ear, retina, and liver in mice[19-23], the production of pure EV-AAVs devoid of free AAVs is the need of the hour to minimize NAb effect. Additionally, while the previous studies targeted the non-cardiac tissues (retina and brain)[21-25], the use of CM-targeting strategy via EV-AAVs with improved delivery of AAVs in the cardiac muscle is an important outcome in this article, leading a step forward towards clinical translatio[26,27]. As summarized in Figure 1, EV-AAVs were identified as potent delivery vectors for targeted cardiac gene therapy by using five in vitro and in vivo methods. In conclusion, authors reported enriched isolation, and characterization of purified EV-AAVs with their validation as an effective therapeutic modality for improvement of myocardial infarction outcome with minimal Nab effects. Future studies aimed at testing the efficacy of this approach in humans by including other gene therapy targets will be of value. Overall, this is an innovative approach that has the potential to revolutionize gene delivery strategies and unlock new horizons for the treatment of various pathologies.
DECLARATIONS
Author’s contributions
Writing, reviewing, and editing the draft: Deshetty UM, Sil S, Buch S
Availability of data and materials
Not applicable.
Financial support and sponsorship
None.
Conflict of interest
Shilpa Buch is an Editorial Board Member of the journal Extracellular Vesicles and Circulating Nucleic Acids. The other authors declared that there are no conflicts of interest.
Ethical approval and consent to participate
Not applicable.
Consent for publication
Not applicable.
Copyright
© The Author(s) 2024.
REFERENCES
1. Kieserman JM, Myers VD, Dubey P, Cheung JY, Feldman AM. Current landscape of heart failure gene therapy. J Am Heart Assoc 2019;8:e012239.
2. Niwano K, Arai M, Koitabashi N, et al. Lentiviral vector-mediated SERCA2 gene transfer protects against heart failure and left ventricular remodeling after myocardial infarction in rats. Mol Ther 2008;16:1026-32.
3. Di Bella MA. Overview and update on extracellular vesicles: considerations on exosomes and their application in modern medicine. Biology 2022;11:804.
4. Sil S, Dagur RS, Liao K, et al. Strategies for the use of extracellular vesicles for the delivery of therapeutics. J Neuroimmune Pharmacol 2020;15:422-42.
5. Chivero ET, Dagur RS, Peeples ES, et al. Biogenesis, physiological functions and potential applications of extracellular vesicles in substance use disorders. Cell Mol Life Sci 2021;78:4849-65.
6. Cambier L, de Couto G, Ibrahim A, et al. Y RNA fragment in extracellular vesicles confers cardioprotection via modulation of IL-10 expression and secretion. EMBO Mol Med 2017;9:337-52.
7. Ginn SL, Amaya AK, Alexander IE, Edelstein M, Abedi MR. Gene therapy clinical trials worldwide to 2017: an update. J Gene Med 2018;20:e3015.
9. Valdmanis PN, Lisowski L, Kay MA. rAAV-mediated tumorigenesis: still unresolved after an AAV assault. Mol Ther 2012;20:2014-7.
10. Li H, Malani N, Hamilton SR, et al. Assessing the potential for AAV vector genotoxicity in a murine model. Blood 2011;117:3311-9.
11. Flotte TR, Berns KI. Adeno-associated virus: a ubiquitous commensal of mammals. Hum Gene Ther 2005;16:401-7.
12. Herzog RW, Yang EY, Couto LB, et al. Long-term correction of canine hemophilia B by gene transfer of blood coagulation factor IX mediated by adeno-associated viral vector. Nat Med 1999;5:56-63.
13. Wang Z, Zhu T, Qiao C, et al. Adeno-associated virus serotype 8 efficiently delivers genes to muscle and heart. Nat Biotechnol 2005;23:321-8.
14. Rapti K, Louis-Jeune V, Kohlbrenner E, et al. Neutralizing antibodies against AAV serotypes 1, 2, 6, and 9 in sera of commonly used animal models. Mol Ther 2012;20:73-83.
15. Naim C, Yerevanian A, Hajjar RJ. Gene therapy for heart failure: where do we stand? Curr Cardiol Rep 2013;15:333.
16. Louis Jeune V, Joergensen JA, Hajjar RJ, Weber T. Pre-existing anti-adeno-associated virus antibodies as a challenge in AAV gene therapy. Hum Gene Ther Methods 2013;24:59-67.
17. Greenberg B, Butler J, Felker GM, et al. Prevalence of AAV1 neutralizing antibodies and consequences for a clinical trial of gene transfer for advanced heart failure. Gene Ther 2016;23:313-9.
18. Li X, La Salvia S, Liang Y, et al. Extracellular vesicle-encapsulated adeno-associated viruses for therapeutic gene delivery to the heart. Circulation 2023;148:405-25.
19. MacLaren RE, Groppe M, Barnard AR, et al. Retinal gene therapy in patients with choroideremia: initial findings from a phase 1/2 clinical trial. Lancet 2014;383:1129-37.
20. György B, Sage C, Indzhykulian AA, et al. Rescue of hearing by gene delivery to inner-ear hair cells using exosome-associated AAV. Mol Ther 2017;25:379-91.
21. Hudry E, Martin C, Gandhi S, et al. Exosome-associated AAV vector as a robust and convenient neuroscience tool. Gene Ther 2016;23:380-92.
22. Wassmer SJ, Carvalho LS, György B, Vandenberghe LH, Maguire CA. Exosome-associated AAV2 vector mediates robust gene delivery into the murine retina upon intravitreal injection. Sci Rep 2017;7:45329.
23. György B, Fitzpatrick Z, Crommentuijn MH, Mu D, Maguire CA. Naturally enveloped AAV vectors for shielding neutralizing antibodies and robust gene delivery in vivo. Biomaterials 2014;35:7598-609.
24. Maguire CA, Balaj L, Sivaraman S, et al. Microvesicle-associated AAV vector as a novel gene delivery system. Mol Ther 2012;20:960-71.
25. Lener T, Gimona M, Aigner L, et al. Applying extracellular vesicles based therapeutics in clinical trials - an ISEV position paper. J Extracell Vesicles 2015;4:30087.
26. Bozoglu T, Lee S, Ziegler T, et al. Endothelial retargeting of AAV9 in vivo. Adv Sci 2022;9:e2103867.
Cite This Article

How to Cite
Download Citation
Export Citation File:
Type of Import
Tips on Downloading Citation
Citation Manager File Format
Type of Import
Direct Import: When the Direct Import option is selected (the default state), a dialogue box will give you the option to Save or Open the downloaded citation data. Choosing Open will either launch your citation manager or give you a choice of applications with which to use the metadata. The Save option saves the file locally for later use.
Indirect Import: When the Indirect Import option is selected, the metadata is displayed and may be copied and pasted as needed.
About This Article
Copyright
Data & Comments
Data
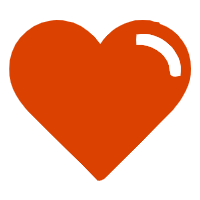
Comments
Comments must be written in English. Spam, offensive content, impersonation, and private information will not be permitted. If any comment is reported and identified as inappropriate content by OAE staff, the comment will be removed without notice. If you have any queries or need any help, please contact us at [email protected].