K+ promoted fabrication of nanoneedle low-silicon ZSM-48 mesocrystal
Abstract
Owing to their distinct structural properties, low-dimensional zeolites are rising stars in the field of catalysis. However, shortening their size while maintaining the acidity continues to be challenging. In addition, simplified synthesis methods to efficiently prepare low-dimensional zeolites with more skeleton types and extended frame components are also of great interest. Herein, a facile strategy is developed for fabricating ultrathin nanoneedle (ca. 6-8 nm in diameter of each needle) ZSM-48 mesocrystals with a low Si/Al ratio (ca. 27, close to the lowest synthesized so far). This is achieved by adding potassium ions in a ZSM-12 synthetic system. The promoting effect of appropriate K+ ions was confirmed by adjusting the gel composition and tracking the crystallization process. Moreover, a superior conversion, reusability and regeneration performance for xylose to furfural is achieved with more accessible acidity and a more suitable Lewis/Brønsted acid ratio, which further expands the development of ZSM-48 zeolite.
Keywords
INTRODUCTION
New emerging low-dimensional zeolites, nanoscale or even < 10 nm in at least one dimension, have been considered as important catalysts due to their unique structure features[1-6]. The suitable design of low-dimensional zeolites can expand the effective accessibility of active sites while maintaining their intrinsic activity. This facilitates meeting the needs of catalytic activity, selectivity and lifetime, especially for macromolecular reactions. Nanosheets (two-dimensional)[7-11], nanorods (one-dimensional)[12-15], nanocrystals[16,17], and even subcrystals[18] (zero-dimensional) are all excellent structures that have been successfully designed. However, it is still a challenge to shorten the scale of one dimension from tens of nm to less than 10 nm under the premise of maintaining its acid properties. Besides, although some ultrathin zeolites can be directly prepared with the assistance of some structure directing agents (SDAs), such as MFI[10,19-21], MWW[7,8,22], and FER[23], and so on. So far, low-dimensional zeolites with other frameworks are still hard to construct because of the complex preparation procedure of zeolites and high cost of SDAs. Preparing low-dimensional zeolites with more frameworks using simplified synthesis methods has attracted considerable attention.
ZSM-48 zeolite is a kind of high-silicon zeolite developed by Mobil Corporation in the early 1980s. It has a one-dimensional linear channel composed of a 10-membered ring (10MR) with a diameter of 5.3 × 5.6 Å[24]. This topological feature of ZSM-48 allows it to be used in various important catalytic reactions, such as pyrolysis of alkanes[25,26], hydroisomerization of long-chain alkanes[27,28], hydrocarbon production with methanol[29], the pyrolysis of low-density polyethylene (LDPE)[30] and dehydration and isomerization of carbohydrates[31,32]. However, at present, the conventional synthesis systems of ZSM-48 are mainly divided into hexamethylenediamine (HDA) and hexamethyldiammonium (HM2+). These two systems are not only prone to the existence of MFI[33] and EUO[34] impurities but also accompanied by the problem of a relatively high Si/Al ratio of final products[35]. The high Si/Al ratio of ZSM-48 (normally > 50) results in an inadequate acid density, thus limiting the reaction effect[26].
In order to solve the issues mentioned above, many approaches have been developed. Introducing crystal seed can reduce the Si/Al ratio of ZSM-48 to a certain extent[36-38]. However, zeolites with other topological structures sometimes need to be introduced as heterogeneous seeds, which increases the complexity of the synthesis process. Changing the morphology of the zeolite product to increase the external specific surface area, which can enhance the accessibility of acidic sites, is also a method to improve the catalytic performance. Constructing hollow[39], nest-like[40] or lamellar[41] structures all showed excellent results, but it requires a cumbersome post-treatment process[30,39] or elaborate and expensive SDA designs[35,42]. Therefore, it remains an urgent problem for ZSM-48 zeolite synthesis to seek a simple means that can both adjust the morphology of ZSM-48 to improve the accessibility of acidic sites and reduce the Si/Al ratio to increase the amounts of active sites.
On the other hand, adding ionic zeolite growth modifiers (ZGM), mainly alkali metal ions (such as Na+, K+, etc.), into the zeolite synthesis system can change the crystallization behavior of zeolite[23,43,44], making it a cheap and facile method to adjust the morphology of zeolite. However, this strategy of optimizing morphology has not been used in the ZSM-48 synthesis.
In this context, ZSM-48 mesocrystal with ultrathin nanoneedle morphology was prepared by adding potassium ions into the existing ZSM-12 growth system without redesigning a new SDA. Among the multistage porous ZSM-48 synthesized with template agents so far[35,40,41,45], the Si/Al ratio of this nanoneedle product is close to the lowest in the existing literature[46]. It also displays a highly catalytic performance and a relatively long lifetime for conversion of xylose to furfural reaction.
EXPERIMENTAL
Materials
To prepare organic SDA, 1,6-dibromohexane (97%, Aladdin), methanol [analytical reagent (AR), Sinopharm Chemical Reagent Co., Ltd] and 1-methylpyrrolidine (98%, Aladdin) were employed. KCl (AR, Sinopharm Chemical Reagent Co., Ltd), NaOH (AR, Sinopharm Chemical Reagent Co., Ltd),
Synthesis of organic structure directing agent
The SDA was prepared as follows: 0.25 mol of 1,6-dibromohexane was added into a three-necked flask with 150 mL of methanol, and then 0.51 mol of 1-methylpyrrolidine was dropwise added into the above solution under stirring. Subsequently, the obtained mixture was heated to 60 °C under reflux conditions. After 72 h, the SDA power was obtained by evaporation of the organic solvent followed by recrystallization with hot methanol. Its purity was confirmed through a 13C nuclear magnetic resonance (NMR) experiment.
Preparation and treatment of ultrathin nanoneedle ZSM-48
Nanoneedle ZSM-48 and other zeolite samples mentioned were prepared as the following molar ratio: 30 SiO2 : 0.25 Al2O3 : 7.5 NaOH : 1.5 SDA : 1,200 H2O : 0-9 KCl (or other modifiers). In detail, 1.30 g SDA was added to 39.49 g deionized water and stirred until the SDA completely dissolved. Keep stirring conditions, then add 0.63 g NaOH, 0.3 g Al2(SO4)3·18H2O and the specified amount of modifier successively. After all the solid completely dissolved, 9.46 g silica sol was dripped into the mixture. After aging for 3 h under stirring, the obtained sol solution was transferred into a 25 mL Teflon-lined autoclave and sealed. Then, it was heated to 160 oC for 36-48 h under static conditions. Finally, the product was recovered by centrifugation, washed with deionized water and dried overnight at 80 oC. In order to remove the SDA, the obtained sample is calcined at 550 oC in air for 6 h. The H-type zeolite was obtained by ion exchange with
Catalytic performance evaluations
The conversion of xylose to furfural reactions was conducted in a 25 mL autoclave at 170 oC under rotation. For a typical run, 0.08 g xylose was added into 4.0 g deionized water. After stirring for 5 min, 0.48 g zeolite catalyst was added into the above mixture. Subsequently, the mixture was sealed and reacted at 170 oC for
In the reuse test of the catalyst, the reaction mixture is centrifuged for 5 min at 1,000 rpm to collect the underlying solid catalyst. After drying at 80 oC, the catalyst was added to the xylose solution again for the next cycle reaction. In the catalyst regeneration test, a Muffle furnace was used to calcinate the waste catalyst in an air atmosphere at 550 oC for 6 h. After cooling to room temperature, the calcined catalyst is used again for the reaction.
Characterization methods
The framework of the obtained product was confirmed by an X-ray diffraction (XRD) experiment on a Bruker D2 instrument with Cu Kα radiation.
Scanning (SEM) and transmission electron microscope (TEM) experiments were performed on a Hitachi
The 13C, 28Si and 27Al magic angle spinning NMR spectra (MAS NMR) of the zeolite samples before calcination were collected on a Bruker AV500 spectrometer.
The framework vibration of nanoneedle zeolite is obtained by Fourier transform infrared (FT-IR) spectroscopy on a PerkinElmer spectrometer.
After the sample was activated at 300 oC under vacuum for 7 h, a Nitrogen adsorption experiment was conducted on an autosorb iQ2 at 77 K.
The thermogravimetric analysis (TGA) curve was collected on a SDT Q600 thermal analysis instrument. The analysis was carried out in an air atmosphere with a flow rate of 100 mL/min; the temperature was raised to 900 oC at a heating rate of 10 oC/min.
FT-IR spectra of adsorbed pyridine (Py-IR) were attained using a Bruker Invenio S. The sample needs to be pre-dehydrated at 450 oC in vacuum for 2 h, exposed to pyridine vapor, then pumped to remove the physical adsorption of pyridine, and measured at 150 and 450 oC.
NH3-temperature programmed desorption (TPD) experiment was conducted on a Micromeritics AutoChem II 2920 instrument. In a typical run, a 100 mg sample was weighed and placed in the reaction tube. The sample was then heated at 10 oC/min from room temperature to 550 oC for drying pre-treatment. He flow (50 mL/min) was purged for 1 h, cooled to 100 oC, and 10% NH3/He mixture (50 mL/min) was injected for 1 h to saturation. Switching He flow (50 mL/min) to purge for 1 h to remove the weak physical adsorption of NH3 on the surface, followed by desorption at a warming rate of 10 oC/min at 650 oC in He atmosphere. Thermal conductivity detector (TCD) was used to detect the removed gas.
RESULTS AND DISCUSSION
Morphology and characteristics of the nanoneedle product
Firstly, all the samples obtained after adjusting the synthesis conditions are shown in Table 1. KCl/0.2/48 is considered typical among all the samples. SEM images exhibit that the KCl/0.2/48 products are aggregated particles with a size of less than 1 μm, and almost no impurity or amorphous substance can be observed
All samples obtained by adjusting synthesis conditions
Modifier | Modifier/Si | Crystallization time (h) | Sample name | Structure |
KCl | 0 | 48 | KCl/0/48 | ZSM-12 |
KCl | 0.1 | 48 | KCl/0.1/48 | ZSM-12 |
KCl | 0.2 | 48 | KCl/0.2/48 | ZSM-48 |
KCl | 0.3 | 48 | KCl/0.3/48 | ZSM-48 + quartz |
NaCl | 0.2 | 48 | NaCl/0.2/48 | ZSM-12 |
LiCl | 0.2 | 48 | LiCl/0.2/48 | ZSM-12 + impurity |
KNO3 | 0.2 | 48 | KNO3/0.2/48 | ZSM-48 |
KCl | 0.2 | 6 | KCl/0.2/6 | Amorphous |
KCl | 0.2 | 18 | KCl/0.2/18 | Amorphous |
KCl | 0.2 | 36 | KCl/0.2/36 | ZSM-48 + amorphous |
KCl | 0.2 | 40 | KCl/0.2/40 | ZSM-48 + amorphous |
KCl | 0.2 | 44 | KCl/0.2/44 | ZSM-48 + amorphous |
KCl | 0.2 | 46 | KCl/0.2/46 | ZSM-48 + amorphous |
/ | / | / | Commercial ZSM-48 | ZSM-48 |
Moreover, the rough surface of the aggregated particles is observed from a high-resolution SEM (HRSEM) image [Figure 1A], and the particles are composed of several very thin nanoneedles. A TEM is further employed to examine the morphology and inner structure of KCl/0.2/48. It is clear that the tail ends of the particles are nanoneedles [Figure 1B and Supplementary Figure 1C], especially the width of each nanoneedle is shortened to only ca. 6-8 nm (Figure 1B inset). Furthermore, the obvious lattice fringes can be identified from a high-resolution TEM (HRTEM) image [Figure 1C], implying the crystalline features of the as-synthesized zeolite products. In addition, after slicing KCl/0.2/48, the TEM images show that the inner of the particles also consists of some thin nanoneedles. The ordered lattice fringes and evident mesopores can be discerned from its HRTEM image [Supplementary Figure 2]. The framework of the obtained product is confirmed by XRD, which shows typical diffraction peaks of ZSM-48 zeolite. However, the peaks were relatively weakened and widened [Figure 1D] than normal commercial ZSM-48 [Supplementary Figure 3A]. It may be because the ultrathin morphology of KCl/0.2/48 is much thinner than commercial ZSM-48
Figure 1. (A) SEM image; (B and C) TEM image, inset: the needle diameter distribution; (D) XRD pattern image; (E) N2 adsorption isotherm of KCl/0.2/48 sample; and (F) pore size distribution in mesoporous segment. SEM: Scanning electron microscope; TEM: transmission electron microscope; XRD: X-ray diffraction.
The textural property of KCl/0.2/48 is characterized by N2 adsorption-desorption experiment. An obvious hysteresis loop at the middle relative pressure can be observed from the isotherm with the steep climb at the high relative pressure [Figure 1E], which implies the existence of mesopores, even macropores, consistent with the observation of SEM/TEM images. Moreover, the external specific surface areas are about 130 m2/g, which can be ascribed to the very thin width of the nanoneedle. As shown in the pore size distribution of KCl/0.2/48 [Figure 1F], there are mesoporous pores between nanoneedles at about 4 nm and intercrystalline mesoporous accumulation above 10 nm. Ar adsorption-desorption experiment was also used to test the microporosity of the sample. It showed similar adsorption isotherm and mesoporous distribution, and the micropore distribution is close to that of ZSM-48 [Supplementary Figure 5].
MAS NMR is used to study the chemical environment of the components in KCl/0.2/48. Two resonances at chemical shifts of ca. -100 and -113 ppm can be detected in the 29Si MAS NMR spectrum, corresponding to the Q3 [Si(OSi)3OH or Si(OSi)3(OAl)] and Q4 [Si(OSi)4], respectively [Figure 2A]. Only a signal at a chemical shift of ca. 55 ppm in the 27Al MAS NMR spectrum suggests that all Al atoms are located in the zeolite framework as the tetra-coordinated form [Figure 2B]. In addition, the 13C MAS NMR spectrum shows that the SDA is intact in the final product without decomposition during the hydrothermal treatment [Figure 2C]. And ca. 10.4 wt% weight loss could be detected from 200 to 750 oC in the TGA result, which corresponds to the decomposition of the template during calcination [Figure 2D]. According to the inductively coupled plasma (ICP) emission spectrometer analysis, the Si/Al ratio for KCl/0.2/48 is only ca. 27, which is comparable to the lowest value (ca. 23) reported among direct synthesis. Accordingly, it can be deduced that there is around 1 SDA molecule in each ZSM-48 zeolite unit cell.
Crystallization mechanism involving potassium ion
The decisive role of potassium ions
Generally, such a zeolite with extremely thin nanoneedles is difficult to synthesize, which means that complex bifunctional SDAs should be utilized for the preparation. However, it is hard to employ these SDAs widely due to the complicated procedure for template synthesis and related high cost. Herein, we use a simple diquaternary ammonium salt as SDA combined with K+ to facilely obtain the nanoneedle ZSM-48 mesocrystal. The high-angle annular dark field scanning transmission electron microscopy (HADDAF-STEM) images indicate that Si, Al, O and K elements are uniformly distributed in KCl/0.2/48 particles
Moreover, we adjusted the ratio of KCl and Si to obtain sample KCl/0-0.3/48 [Figure 3A]. If KCl/SiO2 is tailored to 0.1, the intensities of peaks in XRD of KCl/0.1/48 are slightly weaker, but only the ZSM-12 zeolite framework can be obtained. However, the SEM images show both the nanoneedle ZSM-48 zeolite and ZSM-12 products in KCl/0.1/48, which were aggregated by relatively larger nanoparticles, may be generated [Supplementary Figure 8] in this situation. When KCl/SiO2 = 0.2, the KCl/0.2/48 product of pure phase nanoneedle ZSM-48 can be obtained. This can be judged from the change in the relative distance between the two XRD characteristic peaks [Figure 3B]. If the amount of KCl is increased (KCl/SiO2 = 0.3), some impurities of quartz gradually appear in KCl/0.3/48 product.
Figure 3. (A and B) XRD pattern of samples with different KCl contents; (C) the K/Si in the solid zeolite products calculated by ICP and compared with that in the initial synthetic gel, inset: diagram of correlation between product and KCl quantities. XRD: X-ray diffraction; ICP: inductively coupled plasma.
We also calculate the K/Si in the solid zeolite products by ICP and compare it with that in the initial synthetic gel. Dividing the K/Si of the final zeolite products by the K/Si of the initial synthetic gel, it is surprising to find that the nanoneedle sample (KCl/0.2/48) reaches the lowest ratio among all samples
The Si/Al ratio and K content of different samples
K/Si for synthesis | Si/Ala | Final zeolite/synthesis | K/Sia |
0 | 27.47 | / | / |
0.1 | 23.99 | 0.055 | 0.005 |
0.2 | 27.01 | 0.030 | 0.006 |
0.3 | 21.67 | 0.067 | 0.020 |
To further demonstrate the important role of potassium ions, the KCl is replaced by NaCl or LiCl. Only ZSM-12 zeolite with aggregated nanoparticles is collected [Figure 4A and B] in NaCl/0.2/48 and LiCl/0.2/48. These results imply that K+ ions are critical for fabricating nanoneedle ZSM-48 zeolite. To further exclude the effects of anions, the KCl is replaced by KNO3 in the synthesis system. The XRD pattern of KNO3/0.2/48 shows that ZSM-48 zeolite is obtained with relatively lower crystallinity, and SEM images indicate that the as-prepared sample displays the nanoneedle assembled morphology [Figure 4C]. The texture information is also similar to KCl/0.2/48 [Figure 4D]. As evidently discerned in its TEM images, the width of each nanoneedle is less than 8 nm [Figure 4E and F].
Time-resolved investigation on ZSM-48 crystallization
It has been proven that K+ promotes the formation of nanoneedle ZSM-48 zeolite. Then, we further monitor the crystallization process of a typical sample to clear the exact effect of K+. Sample KCl/0.2/X is obtained by interrupting crystallization at X hour. At about 36 h, the weak XRD characteristic diffraction peaks of ZSM-48 appear [Figure 5A], and the five-membered bands of FT-IR [Figure 5B] also indicate that the skeleton structure of zeolite begins to form. After 44 h, the XRD peak intensity of the product has not changed significantly, indicating the final crystallinity of the product has been reached.
Figure 5. (A) XRD patterns; (B) FT-IR spectrums; (C) TEM images; and (D) N2 adsorption isotherms of nanoneedle ZSM-48 zeolite at different crystallization times. XRD: X-ray diffraction; FT-IR: Fourier transform infrared; TEM: transmission electron microscope.
With the extension of crystallization time from 36 to 44 h, TEM images show that the nanoneedle zeolite particles obviously increased with the continuous consumption of amorphous nanoparticles around [Figure 5C]. In particular, a large number of hollows are found in these amorphous nanoparticles
Catalytic effect of xylose dehydration reaction
Because of its adjustable acidity, zeolite is widely used in synthesizing high value-added chemicals from biomass, which is of great significance for green chemistry and sustainable development. Therefore, the catalytic performance of nanoneedle KCl/0.2/48 is evaluated through xylose to furfural reaction, with commercial ZSM-48 and KCl/0/48 as benchmarks. The effect of this reaction is closely related to the acid properties of the catalysts, so we have characterized the acidity of all three catalysts.
Firstly, the results of Py-IR at 150 oC [Figure 6A] and 450 oC [Figure 6B] indicate that KCl/0.2/48 has both Lewis acid and Brønsted acid sites. Moreover, the two ZSM-48 samples have a higher L/B ratio than KCl/0/48 (ZSM-12) at both 150 and 450 oC, which may be related to the topological structure [Table 3]. The acid amount and strength of catalysts are measured by the NH3-TPD experiment [Figure 6C]. Two distinctive desorption peaks at about 160 and 355 oC can be attributed to the weak and strong acid sites, respectively. Due to their lower Si/Al ratio, KCl/0.2/48 (0.299 mmol·g-1) and KCl/0/48 (0.321 mmol·g-1) have a higher total acid content than commercial ZSM-48 (0.246 mmol·g-1) [Table 3]. Moreover, the amounts of weak acid sites are larger for KCl/0.2/48 (0.209 mmol·g-1) than commercial ZSM-48 (0.184 mmol·g-1) and KCl/0/48 (0.174 mmol·g-1).
Figure 6. Py-IR spectrum at (A) 150 oC and (B) 450 oC; (C) NH3-TPD of different catalysts. Py-IR: FT-IR spectra of adsorbed pyridine; TPD: temperature programmed desorption.
Acidic properties of different catalysts
Samples | Max.temp. (°C) | Weak acid (mmol·g-1) | Max.temp. (°C) | Strong acid (mmol·g-1) | Total acid (mmol·g-1) | L/Ba |
Commercial ZSM-48 | 138.8 | 0.184 | 335.4 | 0.062 | 0.246 | 0.27 |
KCl/0.2/48 | 154.0 | 0.209 | 353.5 | 0.090 | 0.299 | 0.24 |
KCl/0/48 | 160.6 | 0.174 | 364.4 | 0.147 | 0.321 | 0.10 |
Once the nanoneedle KCl/0.2/48 is employed as the catalyst in the xylose conversion reaction [Figure 7A], the conversion of xylose greatly increases to around 95.8% [Figure 7B]. The selectivity of furfural is about 38.9% on KCl/0.2/48, while that on commercial ZSM-48 is only 13.7%. Consequently, the yield of furfural on KCl/0.2/48 (37.2%) is evidently higher than commercial ZSM-48 zeolite, which shows very low conversion (44.4%) and yield (6.1%) in this reaction. Obviously, the nanoneedle KCl/0.2/48 exhibits superiority for the conversion of xylose to furfural. This superiority is attributed not only to its more active sites[51] but also to its larger external specific surface area [Supplementary Table 2], which is more conducive to the reaction on the outer surface. We also compare the KCl/0.2/48 sample with the KCl/0/48 sample aggregated by larger rods (ca. 40 nm in diameter) and find that the morphology of ultrathin nanoneedle KCl/0.2/48 (ca. 6-8 nm) still has an advantage in the reaction, although KCl/0/48 (ZSM-12) has a larger 12-membered ring channel. This may be due to not only its larger external specific surface area but also its higher L/B ratio[52,53]. Other research has shown that more Lewis acid sites will catalyze the isomerization of xylose to xylulose [Figure 7A]. The activation energy of xylulose dehydration at the Brønsted acid site
Figure 7. (A) The reaction path; (B) conversion and furfural yield; and (C) reuse and regeneration test of xylose to furfural reaction on three catalysts.
The reusability and regeneration of the catalyst were also tested. After the reaction, the spent catalyst is centrifuged from the reaction liquid and added to the reaction system again. Because cokes covered the acidic sites, the catalytic effect of the three catalysts decreased to different degrees [Figure 7C]. However, KCl/0.2/48 samples showed the slowest rate of inactivation and still had a furfural yield larger than 10% after three cycles. In comparison, the commercial ZSM-48 and KCl/0/48 samples were reduced to less than 10% furfural yield after just one cycle. Moreover, KCl/0.2/48 also showed the best catalytic effect among the three catalysts after the catalyst was regenerated by removing coke through calcination. This exhibits that the nanoneedle morphology of KCl/0.2/48 has an advantage for diffusion, which may delay the coke formation, so that the catalyst has a longer lifetime.
CONCLUSIONS
A nanoneedle ZSM-48 mesocrystal is harvested, and the width of each nanoneedle is about 6-8 nm. Meanwhile, by systematically studying morphology evolution, topological structure change, Si/Al composition, and crystallization process details, the promoting effect of K+ has been confirmed. Furthermore, such an ultrathin ZSM-48 zeolite possesses a lower Si/Al ratio, larger external specific surface area, and more acid sites than commercial ZSM-48. It exhibits excellent catalytic performance and a long lifetime for the conversion of xylose because of more accessible acidity and a more suitable Lewis/Brønsted acid ratio. This work opens a facile and efficient avenue to fabricate low-dimensional zeolite catalysts with various frameworks, promoting the development of these materials and exhibiting great potential for improving their catalytic performance.
DECLARATIONS
Authors’ contributions
Conceptualization, investigation, data curation, formal analysis, writing - original draft, visualization: Yan K
Formal analysis, validation, data curation, writing - review and editing: Zhao Y
Formal analysis, validation, data curation: Zhao C
Investigation, methodology, validation: Li H, Ye Z, Yang X
Resources, formal analysis, writing - review and editing: Zhang Y
Resources, conceptualization, formal analysis, visualization, writing - review and editing: Zhang H
Resources, formal analysis, writing - review and editing, supervision, project administration: Tang Y
Availability of data and materials
The detailed materials and methods in the experiment were listed in the Supplementary Materials. Other raw data that support the findings of this study are available from the corresponding author upon reasonable request.
Financial support and sponsorship
This work was supported by the National Key R&D Program of China (No. 2023YFA1507602) and the National Natural Science Foundation of China (Nos. 22088101, 22175040, 22072028).
Conflicts of interest
Tang Y is an Editorial Board member of the journal Chemical Synthesis, while the other authors have declared that they have no conflicts of interest.
Ethical approval and consent to participate
Not applicable.
Consent for publication
Not applicable.
Copyright
© The Author(s) 2024.
Supplementary Materials
REFERENCES
1. Přech J, Pizarro P, Serrano DP, Čejka J. From 3D to 2D zeolite catalytic materials. Chem Soc Rev 2018;47:8263-306.
2. Xu L, Ma T, Shen Y, et al. Rational manipulation of stacking arrangements in three-dimensional zeolites built from two-dimensional zeolitic nanosheets. Angew Chem Int Ed Engl 2020;59:19934-9.
3. Jiao M, Huang J, Xu H, et al. ECNU-36: a quasi-pure polymorph CH beta silicate composed of hierarchical nanosheet crystals for effective VOCs adsorption. Angew Chem Int Ed Engl 2020;59:17291-6.
4. Lei C, Dong Z, Martínez C, et al. A cationic oligomer as an organic template for direct synthesis of aluminosilicate ITH zeolite. Angew Chem Int Ed Engl 2020;59:15649-55.
5. Guefrachi Y, Sharma G, Xu D, et al. Steam-induced coarsening of single-unit-cell MFI zeolite nanosheets and its effect on external surface Brønsted acid catalysis. Angew Chem Int Ed Engl 2020;59:9579-85.
6. Yan K, Ye Z, Kong L, et al. Seed-induced synthesis of disc-cluster zeolite L mesocrystals with ultrashort c-axis: morphology control, decoupled mechanism, and enhanced adsorption. Acta Phys Chim Sin 2024;40:2308019.
7. Luo HY, Michaelis VK, Hodges S, Griffin RG, Román-Leshkov Y. One-pot synthesis of MWW zeolite nanosheets using a rationally designed organic structure-directing agent. Chem Sci 2015;6:6320-4.
8. Chen JQ, Li YZ, Hao QQ, et al. Controlled direct synthesis of single- to multiple-layer MWW zeolite. Natl Sci Rev 2021;8:nwaa236.
9. Shen X, Mao W, Ma Y, et al. A hierarchical MFI zeolite with a two-dimensional square mesostructure. Angew Chem Int Ed Engl 2018;57:724-8.
10. Zhang Y, Shen X, Gong Z, Han L, Sun H, Che S. Single-crystalline MFI zeolite with sheet-like mesopores layered along the a axis. Chemistry 2019;25:738-42.
11. Tai W, Dai W, Wu G, Li L. A simple strategy for synthesis of b-axis-oriented MFI zeolite macro-nanosheets. Chem Synth 2023;3:38.
12. Li B, Sun B, Qian X, et al. In-situ crystallization route to nanorod-aggregated functional ZSM-5 microspheres. J Am Chem Soc 2013;135:1181-4.
13. Tao H, Yang H, Zhang Y, et al. Space-confined synthesis of nanorod oriented-assembled hierarchical MFI zeolite microspheres. J Mater Chem A 2013;1:13821-7.
14. Ren L, Guo Q, Zhang H, et al. Organotemplate-free and one-pot fabrication of nano-rod assembled plate-like micro-sized mordenite crystals. J Mater Chem 2012;22:6564-7.
15. Ye Z, Kong L, Zhao Y, et al. Alkalinity-controlled zeolite nucleation and growth: ultrafast synthesis of total-morphology zeolite L mesocrystals and adsorption evaluation. Chem Synth 2022;2:20.
16. Awala H, Gilson JP, Retoux R, et al. Template-free nanosized faujasite-type zeolites. Nat Mater 2015;14:447-51.
17. Yang XY, Tian G, Chen LH, et al. Well-organized zeolite nanocrystal aggregates with interconnected hierarchically micro-meso-macropore systems showing enhanced catalytic performance. Chemistry 2011;17:14987-95.
18. Sheng Z, Li H, Du K, et al. Observing a zeolite nucleus (subcrystal) with a uniform framework structure and its oriented attachment without single-molecule addition. Angew Chem Int Ed Engl 2021;60:13444-51.
19. Park W, Yu D, Na K, et al. Hierarchically structure-directing effect of multi-ammonium surfactants for the generation of MFI zeolite nanosheets. Chem Mater 2011;23:5131-7.
20. Xu D, Ma Y, Jing Z, et al. π-π interaction of aromatic groups in amphiphilic molecules directing for single-crystalline mesostructured zeolite nanosheets. Nat Commun 2014;5:4262.
21. Zhang Q, Li J, Wang X, et al. Silanol-engineered nonclassical growth of zeolite nanosheets from oriented attachment of amorphous protozeolite nanoparticles. J Am Chem Soc 2023;145:21231-41.
22. Zhang C, Lin F, Kong L, et al. c-Axis-penetrated mesoporous MWW zeolite nanosheets: preparation by H2O2-induced micro-explosion and their enhanced properties. Inorg Chem Front 2022;9:4030-40.
23. Lee Y, Park MB, Kim PS, et al. Synthesis and catalytic behavior of ferrierite zeolite nanoneedles. ACS Catal 2013;3:617-21.
24. Schlenker J, Rohrbaugh W, Chu P, Valyocsik E, Kokotailo G. The framework topology of ZSM-48: a high silica zeolite. Zeolites 1985;5:355-8.
25. Bhattacharya D, Tambe S, Sivasanker S. The influence of reaction temperature on the cracking mechanism of n-hexane over H-ZSM-48. Appl Catal A Gen 1997;154:139-53.
26. Zhao G, Teng J, Zhang Y, et al. Synthesis of ZSM-48 zeolites and their catalytic performance in C4-olefin cracking reactions. Appl Catal A Gen 2006;299:167-74.
27. Mériaudeau P, Tuan VA, Nghiem VT, Sapaly G, Naccache C. Comparative evaluation of the catalytic properties of SAPO-31 and ZSM-48 for the hydroisomerization of N-Octane: effect of the acidity. J Catal 1999;185:435-44.
28. Meng J, Bai D, Zeyaodong P, Li C, Chen X, Liang C. Hydroisomerization of n-hexadecane over Pt/ZSM-48 catalysts: effects of metal-acid balance and crystal morphology. Micropor Mesopor Mat 2022;330:111637.
29. Zhang J, Huang Z, Xu L, et al. Verifying the olefin formation mechanism of the methanol-to-hydrocarbons reaction over H-ZSM-48. Catal Sci Technol 2019;9:2132-43.
30. Azhari NJ, Mardiana S, Kadja GT. ZSM-48 zeolites with controllable mesopore formation: synthesis, characterization, and catalytic performance. Chem Eng J Advances 2023;16:100533.
31. Kadja GTM, Azhari NJ, Mardiana S, Khalil M, Subagjo, Mahyuddin MH. Accelerated, mesoporogen-free synthesis of hierarchical nanorod ZSM-48 assisted by hydroxyl radicals. Ind Eng Chem Res 2021;60:17786-91.
32. Saenluang K, Srisuwanno W, Salakhum S, Rodaum C, Dugkhuntod P, Wattanakit C. Nanoporous Sn-substituted ZSM-48 nanostructures for glucose isomerization. ACS Appl Nano Mater 2021;4:11661-73.
33. Xue Y, Li S, Li J, et al. Enhancing propene selectivity in methanol and/or butene conversion by regulating channel systems over ZSM-5/ZSM-48 composite zeolites. Micropor Mesopor Mat 2021;312:110803.
34. Giordano G, Nagy J, Derouane E. Zeolite synthesis in presence of hexamethonium ions. J Mol Catal A Chem 2009;305:34-9.
35. Astafan A, Benghalem MA, Michelin L, et al. Synthesis of hierarchical ZSM-48 nano-zeolites. New J Chem 2018;42:4457-64.
36. Meng J, Li C, Chen X, Song C, Liang C. Seed-assisted synthesis of ZSM-48 zeolite with low SiO2/Al2O3 ratio for n-hexadecane hydroisomerization. Micropor Mesopor Mat 2020;309:110565.
37. Shang S, Ren L, Liu Q, et al. Ultrafast synthesis and regulating Al status of mesoporous ZSM-48 zeolite via a pretreated-seed-solution-assisted strategy. Cryst Growth Des 2023;23:5008-18.
38. Liu W, Zhang X, Yu Q, et al. Unconventional seed-assisted strategy for Al-rich hierarchical ZSM-48 zeolite. J Colloid Interface Sci 2024;653:1715-24.
39. Liu W, Li J, Yu Q, et al. Construction of a one-dimensional Al-rich ZSM-48 zeolite with a hollow structure. ACS Appl Mater Interfaces 2022;14:52025-34.
40. Wang R, Peng Z, Wu P, et al. Direct synthesis of nanorod stacked “nest-like” hierarchical ZSM-48 hollow spheres using a triazine-based bolaform organic structure-directing agent. Inorg Chem Front 2022;9:2016-22.
41. Zhang Y, Ma Y, Che S. Synthesis of lamellar mesostructured ZSM-48 nanosheets. Chem Mater 2018;30:1839-43.
42. Zhang K, Li C, Liu Z, Wang M, Yan X, Xi H. Tailoring hierarchical zeolites with designed cationic surfactants and their high catalytic performance. Chem Asian J 2017;12:2711-9.
43. Ye Z, Zhao Y, Zhang H, et al. Mesocrystal morphology regulation by “alkali metals ion switch”: re-examining zeolite nonclassical crystallization in seed-induced process. J Colloid Interface Sci 2022;608:1366-76.
44. Lin F, Ye Z, Kong L, et al. Facile morphology and porosity regulation of zeolite ZSM-5 mesocrystals with synergistically enhanced catalytic activity and shape selectivity. Nanomaterials 2022;12:1601.
45. Liu W, Li J, Liu Z, et al. Direct preparation of *MRE zeolites with ultralarge mesoporosity: strategy and working mechanism. ACS Appl Mater Interfaces 2021;13:31756-65.
46. Zhai M, Ding H, Zeng S, et al. Aluminous ZSM-48 zeolite synthesis using a hydroisomerization intermediate mimicking allyltrimethylammonium chloride as a structure-directing agent. Ind Eng Chem Res 2020;59:11139-48.
47. Fan W, Li R, Ma J, Fan B, Dou T, Cao J. Crystallization mechanism study on ZSM-48 in the system Na2O-Al2O3-SiO2-H2N(CH2)6NH2. Micropor Mat 1997;8:131-40.
48. Sadrara M, Khorrami MK, Darian JT, Garmarudi AB. Fabrication of highly mesoporous ZSM-48 zeolite by anionic surfactant-organosilane system for catalytic conversion of methanol to gasoline. Solid State Sci 2022;128:106888.
49. Zhao Y, Ye Z, Wang L, et al. Engineering fractal MTW zeolite mesocrystal: particle-based dendritic growth via twinning-plane induced crystallization. Cryst Growth Des 2018;18:1101-8.
50. Fan W, Li R, Fan B, Ma J, Cao J. Effects of introduction of different alkali metal halides on crystallization and characteristics of ZSM-48 in a solid reaction mixture system effects of alkali metal chlorides. Appl Catal A Gen 1996;143:299-308.
51. Kim SB, You SJ, Kim YT, et al. Dehydration of D-xylose into furfural over H-zeolites. Korean J Chem Eng 2011;28:710-6.
52. Shao Y, Sun K, Zhang L, et al. Balanced distribution of Brønsted acidic sites and Lewis acidic sites for highly selective conversion of xylose into levulinic acid/ester over Zr-beta catalysts. Green Chem 2019;21:6634-45.
53. Valadares DS, Clemente MCH, de Freitas EF, Martins GAV, Dias JA, Dias SCL. Niobium on BEA dealuminated zeolite for high selectivity dehydration reactions of ethanol and xylose into diethyl ether and furfural. Nanomaterials 2020;10:1269.
Cite This Article
How to Cite
Download Citation
Export Citation File:
Type of Import
Tips on Downloading Citation
Citation Manager File Format
Type of Import
Direct Import: When the Direct Import option is selected (the default state), a dialogue box will give you the option to Save or Open the downloaded citation data. Choosing Open will either launch your citation manager or give you a choice of applications with which to use the metadata. The Save option saves the file locally for later use.
Indirect Import: When the Indirect Import option is selected, the metadata is displayed and may be copied and pasted as needed.
About This Article
Special Issue
Copyright
Author Biographies
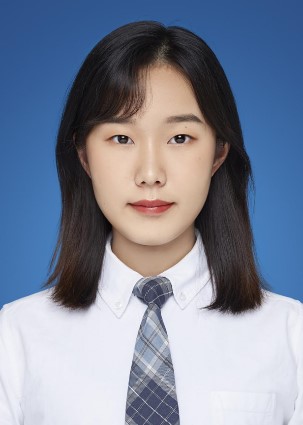
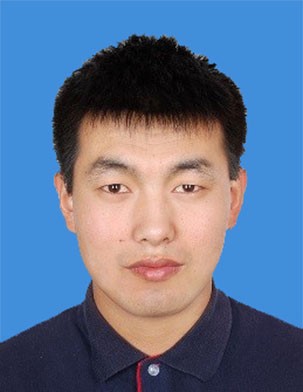
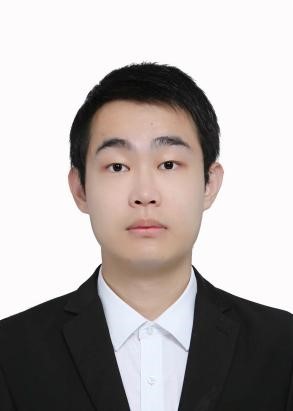
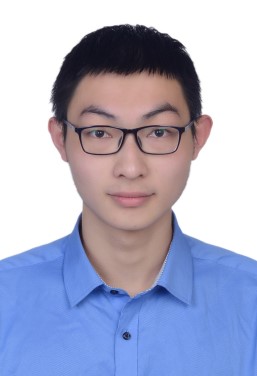
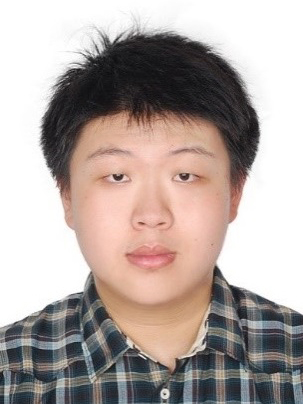
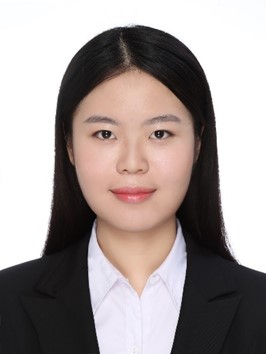
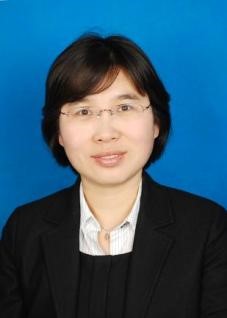
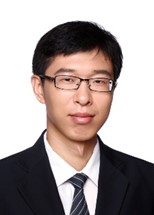
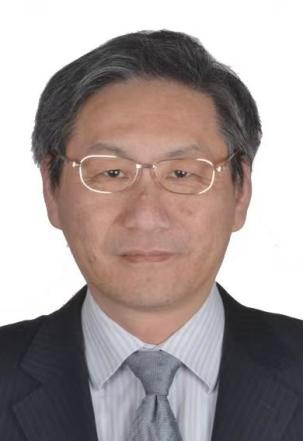
Comments
Comments must be written in English. Spam, offensive content, impersonation, and private information will not be permitted. If any comment is reported and identified as inappropriate content by OAE staff, the comment will be removed without notice. If you have any queries or need any help, please contact us at [email protected].