Catalyst-free decarboxylative alkylation: access to quaternary center
Abstract
The formation of C(sp3)−C(sp3) bonds has received continuous attention in organic synthesis, and the focus on versatile alkyl precursors remains constant. In our work, prevalent amines and carboxylic acids successfully serve as alkyl sources to construct C(sp3)−C(sp3) bonds via decarboxylative deamination. The catalyst-free decarboxylative alkylation reaction provides alternative access to the quaternary center. Primary mechanistic experiments suggest that it undergoes a polar mechanism.
Keywords
INTRODUCTION
The generation of new organic molecules relies on the formation of new bonds. Primary amines, as general and useful feedstocks, are generally used in constructing C−N bonds in organo-metallic chemistry and biochemistry [Scheme 1A][1,2]. If primary aliphatic amino groups are converted into leaving groups, they will be considered versatile alkyl sources, expanding the synthetic value of amines. Alkyl ammonium salts and alkyl pyridinium salts, prepared from primary amines, have been treated as versatile alkyl precursors. Especially, there is significant progress in deaminative functionalization of pyridinium salts through polar and radical processes. Commonly, alkyl radicals are accessible via metal reduction, photoredox catalysis, electron donor-acceptor complex, Lewis base catalysis, and electrochemical reduction[3-6]. The generated carbon radical could participate in subsequent transformation, establishing secondary and tertiary centers.
Scheme 1. (A) Amidation reaction between amines and carboxylic acids; (B) esterification reaction between carboxylic acids and amine-derived pyridinium salts; (C) this work: decarboxylative alkylation reaction. TPT: 2,4,6-triphenylpyrylium; DMSO: Dimethyl sulfoxide; RT: room temperature.
In 1979, Katritzky found that alkylpyridinium salts reacted with diverse nucleophiles to form C−hetero bonds[7]. Recently, McGrath et al. disclosed esterification reactions between carboxylic acids and amine-derived Katritzky salts. In this work, second and tertiary alkyl carboxylic acids participated in the formation of C−O bonds [Scheme 1B][8]. To the best of our knowledge, decarboxylative deamination has rarely been studied. In consideration of the significance of quaternary centers[9-11], we report nucleophilic substitution reactions of alkyl pyridinium salts with alkyl carboxylic acids to form C(sp3)−C(sp3) bonds containing these specialized centers [Scheme 1C].
It is well known that alkyl carboxylic acids could undergo radical decarboxylation via single electron oxidation to generate alkyl radicals[12-14]. When treated with base, the nucleophilic C(sp3) intermediates can be generated by ionic decarboxylation[15-20]. Based on the previous work, a plausible mechanistic pathway is proposed in Scheme 2. In the presence of a base, alkyl carboxylate is generated. After decarboxylation, carbon anion II attacks Katritzky salts I, giving the final target products. The key step is ionic decarboxylation, which could give relatively stable tertiary carbon anion II under mild conditions.
EXPERIMENTAL
To a mixture of pyridinium salt 1 (0.1 mmol), 2,2,2-triphenylacetic acid 2a (0.1 mmol), KHCO3 (0.1 mmol) was added Dimethyl sulfoxide (DMSO) (0.5 mL) under N2 atmosphere. Next, the reaction mixture was replaced Magnetic Stirrer at room temperature for 6 h. When the reaction finished, the mixture was purified by flash column chromatography on silica gel (petroleum ether: ethyl acetate = 50/1).
RESULTS AND DISCUSSION
To verify our proposal presented in Scheme 2, 1-benzyl-2,4,6-triphenylpyridin-1-ium tetrafluoroborate and 2,2,2-triphenylacetic acid were selected as the model substrate [Table 1]. In the presence of KHCO3, the reaction proceeded smoothly in MeCN, giving the desired product in 43% yield, without esterification product (entry 1). The screening of solvents showed that DMSO resulted in the best yield (entries 1-5). These results suggested that aprotic solvent was beneficial to decarboxylation for the carboxylate. When inducing the amount of DMSO (0.5 mL), a similar yield was obtained (84% isolated yield, entry 6). Inorganic base performed better than organic base (entries 6-9). When using 20% equiv of KHCO3, a lower yield was given (21%, entry 10). Exposure to air adversely affects the polar reaction (entry 11). The carbon anion intermediate may be oxidized by O2, delivering triphenylmethanol, detected by gas chromatography mass spectrum (GCMS). Hence,
The optimization of reactiona
![]() | |||
Entry | Solvent | Base | Yield (%)b |
1 | CH3CN | KHCO3 | 43 |
2 | Toluene | KHCO3 | 0 |
3 | CHCl3 | KHCO3 | 29 |
4 | Acetone | KHCO3 | 79 |
5 | DMSO | KHCO3 | 95 |
6c | DMSO | KHCO3 | 91 (84) |
7c | DMSO | K2HPO4 | 82 |
8c | DMSO | KH2PO4 | 13 |
9c | DMSO | 2,4,6-Collidine | 18 |
10c,d | DMSO | KHCO3 | 21 |
11c,e | DMSO | KHCO3 | 20 |
12c | DMSO | - | 17 |
13c,f | DMSO | KHCO3 | 92 |
With the optimal reaction conditions in hand, we evaluated the scope of the reaction, as summarized in Scheme 3. Generally, the reaction occurred in good to excellent yield with benzylic pyridinium salts. Benzylic amines with donating groups on the phenyl ring at para-position performed well in this decarboxylative alkylation (3b-3c, 70%-80%). Moreover, strong electron-deficient (CN, CF3, OCF3) benzylic pyridinium salts were tolerated and isolated in acceptable yields (3e-3g, 51%-55%), while (4-chlorophenyl)methanamine gave a better result (3d, 80%). The substituent groups at ortho-position and meta-position were both compatible (3h-3l). Under the reaction conditions, substrates could bear bis-substituted methyl, methoxy groups, and halogen (3m-3q). Heteroaromatic rings, given their importance in numerous pharmaceuticals, were next examined. To our delight, the pyridinium salts, such as those containing functionalized thiophenes, pyridines, pyrimidines, and pyrazines, were successfully treated as alkyl sources to construct quaternary centers (3r-3u). Generally, secondary pyridinium salts show larger steric hindrance than primary pyridinium salts. As expected, 1x resulted in a low yield, even extended to
Scheme 3. The scope of pyridinium salts. aReaction conditions: 1a (0.1 mmol, 1.0 equiv), 2a (0.1 mmol, 1.0 equiv), KHCO3 (0.1 mmol,
To clarify the practice of this method, a gram-scale reaction between 1-(but-3-en-2-yl)-2,4,6-triphenylpyridin-1-ium tetrafluoroborate (1w) and 2,2,2-triphenylacetic acid (2a) was performed [Scheme 4]. The desired coupling product (3w), a kind of terminal olefine with a quaternary carbon center, was afforded as a white solid in 61% yield.
When monitoring the reaction, it was shown that most of the reactants transferred into 3a in a short time (59% in 0.5 h), and the reaction rate decreased as time increased due to a decrease in reactant concentration [Scheme 5A]. To further clarify the possible reaction mechanism, radical inhibiting experiments were first conducted [Scheme 5B]. Under the standard condition, three kinds of radical inhibitors were added. It is clear that reductive inhibitors, such as 2,6-di-tert-butyl-4-methylphenol (BHT) and N-tert-Butyl-α-phenylnitrone (PBN), make no difference. When adding 2,2,6,6-tetramethylpiperidinyloxy (TEMPO), the reaction was inhibited, probably due to its strong oxidation. These results suggest that the present decarboxylative alkylation may be a polar reaction. We assume that pyridinium salts might act as electrophiles, and 2,2,2-triphenylacetic acid undergoes ionic decarboxylation to give nucleophilic alkyl carbon anion intermediates. In the presence of D2O, we found that the prepared potassium 2,2,2-triphenylacetate directly proceeded with decarboxylation and gave D-triphenylmethane in 98% yield [Scheme 5C]. Next, anhydrous DMSO was used as the solvent. The desired decarboxylative alkylation product was afforded in a compatible yield (88%), accompanying a trace amount of triphenylmethane. As shown in Scheme 2, in the presence of KHCO3, tertiary carboxylate was formed. When finishing decarboxylation, the generated carbon anion (II) acted as a nucleophile. Followed by the cleavage of C−N bonds, the desired product 3 was delivered.
CONCLUSIONS
In conclusion, a catalyst-free decarboxylative alkylation has been developed. Versatile pyridinium salts, prepared from primary amines, acted as an effective electrophile and reacted with tertiary carboxylic acids to afford quaternary centers under mild conditions without excess reagents. Radical-inhibiting and deuteration-labeled experiments suggest that the reaction may proceed via a polar mechanism.
DECLARATIONS
Authors’ contributions
Designing the experiments, writing the manuscript, and being responsible for the whole work: Xu W
Synthesizing the substrates and performing the experiments: Cai L, Li F, Wang S
Availability of data and materials
Detailed experimental procedures and spectroscopic data are published as Supplementary Materials in the journal, and the data supporting the findings of this study are available within its Supplementary Materials.
Financial support and sponsorship
This work is supported by the Natural Science Foundation of Jiangxi Province (20212BAB213036 and 20224BAB203001) and the start-up fund of Nanchang University.
Conflicts of interest
Xu W and Cai L declare a competing interest, stating that one patent has been registered (202311376023.8).
Ethical approval and consent to participate
Not applicable.
Consent for publication
Not applicable.
Copyright
© The Author(s) 2024.
Supplementary Materials
REFERENCES
1. Ouyang K, Hao W, Zhang WX, Xi Z. Transition-metal-catalyzed cleavage of C−N single bonds. Chem Rev 2015;115:12045-90.
2. Ruiz-Castillo P, Buchwald SL. Applications of palladium-catalyzed C−N cross-coupling reactions. Chem Rev 2016;116:12564-649.
3. Pang Y, Moser D, Cornella J. Pyrylium salts: selective reagents for the activation of primary amino groups in organic synthesis. Synthesis 2020;52:489-503.
4. Rössler SL, Jelier BJ, Magnier E, Dagousset G, Carreira EM, Togni A. Pyridinium salts as redox-active functional group transfer reagents. Angew Chem Int Ed Engl 2020;59:9264-80.
5. Correia JTM, Fernandes VA, Matsuo BT, Delgado JAC, de Souza WC, Paixão MW. Photoinduced deaminative strategies: Katritzky salts as alkyl radical precursors. Chem Commun 2020;56:503-14.
6. Li YN, Xiao F, Guo Y, Zeng YF. Recent developments in deaminative functionalization of alkyl amines. Eur J Org Chem 2021;2021:1215-28.
7. Katritzky AR. Conversions of primary amino groups into other functionality mediated by pyrylium cations. Tetrahedron 1980;36:679-99.
8. McGrath A, Zhang R, Shafiq K, Cernak T. Repurposing amine and carboxylic acid building blocks with an automatable esterification reaction. Chem Commun 2023;59:1026-9.
9. Feng J, Holmes M, Krische MJ. Acyclic quaternary carbon stereocenters via enantioselective transition metal catalysis. Chem Rev 2017;117:12564-80.
10. Li M, Liu T, Li J, He H, Dai H, Xie J. Visible-light-mediated deoxyalkynylation of activated tertiary alcohols. J Org Chem 2021;86:12386-93.
11. Green SA, Huffman TR, McCourt RO, van der Puyl V, Shenvi RA. Hydroalkylation of olefins to form quaternary carbons. J Am Chem Soc 2019;141:7709-14.
12. Xiao P, Pannecoucke X, Bouillon JP, Couve-Bonnaire S. Wonderful fusion of organofluorine chemistry and decarboxylation strategy. Chem Soc Rev 2021;50:6094-151.
13. Kitcatt DM, Nicolle S, Lee AL. Direct decarboxylative Giese reactions. Chem Soc Rev 2022;51:1415-53.
14. Li X, Yuan X, Hu J, Li Y, Bao H. Radical decarboxylative carbon-nitrogen bond formation. Molecules 2023;28:4249.
15. Kong D, Moon PJ, Bsharat O, Lundgren RJ. Direct catalytic decarboxylative amination of aryl acetic acids. Angew Chem Int Ed Engl 2020;59:1313-9.
16. Moon PJ, Lundgren RJ. Metal-catalyzed ionic decarboxylative cross-coupling reactions of C(sp3) acids: reaction development, mechanisms, and application. ACS Catal 2020;10:1742-53.
17. Destro G, Horkka K, Loreau O, et al. Transition-metal-free carbon isotope exchange of phenyl acetic acids. Angew Chem Int Ed Engl 2020;59:13490-5.
18. Kong D, Moon PJ, Lui EKJ, Bsharat O, Lundgren RJ. Direct reversible decarboxylation from stable organic acids in dimethylformamide solution. Science 2020;369:557-61.
19. Xing WL, Wang JX, Fu MC, Fu Y. Efficient decarboxylative/defluorinative alkylation for the synthesis of gem-difluoroalkenes through an SN2’-type route. Chin J Chem 2022;40:323-8.
Cite This Article
How to Cite
Download Citation
Export Citation File:
Type of Import
Tips on Downloading Citation
Citation Manager File Format
Type of Import
Direct Import: When the Direct Import option is selected (the default state), a dialogue box will give you the option to Save or Open the downloaded citation data. Choosing Open will either launch your citation manager or give you a choice of applications with which to use the metadata. The Save option saves the file locally for later use.
Indirect Import: When the Indirect Import option is selected, the metadata is displayed and may be copied and pasted as needed.
About This Article
Copyright
Author Biographies
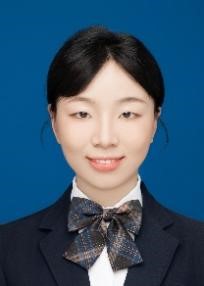
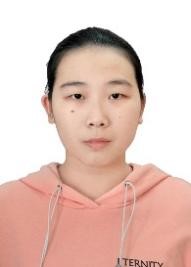
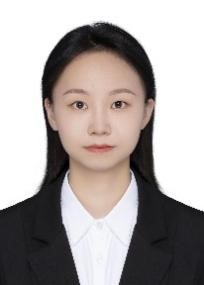
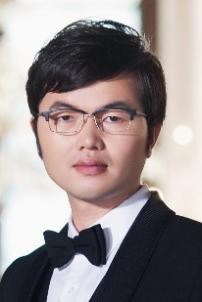
Data & Comments
Data
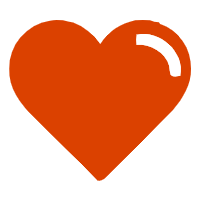
Comments
Comments must be written in English. Spam, offensive content, impersonation, and private information will not be permitted. If any comment is reported and identified as inappropriate content by OAE staff, the comment will be removed without notice. If you have any queries or need any help, please contact us at [email protected].