Acyl transfer-enabled catalytic asymmetric Michael addition of α-hydroxy-1-indanones to nitroolefins
Abstract
We report herein an enantioselective acyl transfer protocol via electrophile activation. The reaction cascade sequence encompasses dinuclear zinc-catalyzed asymmetric Michael addition, intramolecular cyclization, and retro-Claisen reaction, which leads to a step- and atom-economic approach to a variety of protected cyclic tertiary α-hydroxyketones in good yields with excellent enantioselectivities (24 examples, 56%-82% yield, 1.5-13 dr and 79%-96% ee). Besides, the large-scale synthesis and further transformation of the products demonstrate the effectiveness of this method for organic synthesis.
Keywords
INTRODUCTION
Acyl transfer presents an omnipresent and efficient chemoselective ligation process in biological systems[1], which has attracted extensive attention in the chemical community in recent years[2]. In order to circumvent intrinsic reactivity or selectivity issues associated with mono-activated reactants, acyl transfer strategy utilizes the acyl group as a transient activating group to produce bis-activated reactants [Scheme 1A]. Such a chemical event involves a reaction cascade of X-Y bond formation, intramolecular cyclization, and the retro-Claisen reaction, where the challenging mono-activated function could be implemented formally. In this context, several catalytic asymmetric acyl transfer methods have been developed based on nucleophile activation [Scheme 1B]. For example, Mondal, Song et al. developed the asymmetric cascade Michael/acyl transfer reactions of α-nitroketones and 1,3-diketones using bifunctional organocatalysts[3-11]. Rodriguez and Luo reported the secondary or primary amine-catalyzed acyl transfer reactions[12-16]. Very recently, Yi et al. have established an elegant iridium-catalyzed asymmetric cascade allylation/acyl transfer reaction for the synthesis of enantiomerically enriched 3-hydroxymethyl pentenal units[17]. Besides, using acyl transfer, Zhou, Yang et al. prepared the medium-sized-ring lactams from cyclobutanone β-ketoamides[18-21]. Despite these achievements via nucleophile activation, acyl transfer via electrophile activation remains far less developed [Scheme 1C]. As far as we know, there was only one example from the Pan group, where nitroenone was used as an electrophilic acyl transfer reagent in catalytic asymmetric Friedel-Crafts and Michael reactions[22]. Therefore, the development of new catalytic asymmetric acyl transfer methods via electrophile activation is highly desired.
Our group has a long-standing interest in developing facile protocols for synthesizing biologically important molecules. Recently, we have discovered that α-hydroxy-1-indanones could serve as a valid synthon in cyclization reactions with activated Michael acceptors via chiral dinuclear zinc catalysis[23,24]. Along this line, we envisioned that dinuclear zinc-catalyzed asymmetric acyl transfer reaction between α-hydroxy-1-indanones 1 and nitroenones 2 was feasible via less-explored electrophile activation mechanism, generating thereby the protected cyclic tertiary α-hydroxyketones 3 in an enantioselective, step- and atom-economic manner. As illustrated in Scheme 2, the reaction cascade was triggered by dinuclear zinc-catalyzed Michael reaction[25,26], which led to the intermediate Int-1. The subsequent intramolecular cyclization/retro-Claisen reaction resulted in the acyl transfer product 3. However, therein lie several synthetic organic chemistry challenges to this reaction proposal, which include: the enantioselective formation of the tetrasubstituted stereocenter, the side-formations of potential interruption product hemiketal Int-2 and dehydration product dihydrofuran 4. Herein, we introduce a highly enantioselective acyl transfer protocol via under-exploited electrophile activation by making use of dinuclear zinc-catalyzed Michael/cyclization/retro-Claisen reaction cascade, which led to a step- and atom-economic access to a variety of protected cyclic tertiary α-hydroxyketones in good yields with excellent enantioselectivities.
EXPERIMENTAL
Under a nitrogen atmosphere, a solution of diethylzinc (20 μL, 1.0 M in hexane, 0.02 mmol) was added dropwise to a solution of L4 (0.01 mmol, 9.6 mg) in MeCN (2 mL). After the mixture was stirred for 30 min at 30 oC, 1a (0.2 mmol, 29.6 mg) and 2a (0.2 mmol, 50.6 mg) were added. The reaction mixture was stirred for 48 h at the same temperature. The reaction was quenched with HCl solution (1 M, 2 mL), and the organic layer was extracted with CH2Cl2 (3 × 5 mL). The combined organic layer was washed with brine and dried over Na2SO4. The solvent was removed under reduced pressure by using a rotary evaporator. The residue was purified by flash chromatography with petroleum ether/ethyl acetate (4:1) to afford the desired chiral product 3a.
RESULTS AND DISCUSSION
The model reaction between α-hydroxy-1-indanone 1a and 2-nitro-1,3-diphenylprop-2-en-1-one 2a was initially performed in the presence of 10 mol % of dinuclear zinc catalyst in situ generated from 10 mol % of ligand L1 and 20 mol % of ZnEt2 in tetrahydrofuran (THF) at 30 oC oC Figure 1. The desired product 3a was obtained in 56% yield with 1.5:1 diastereoselectivity and 39% ee value [Table 1, entry 1]. The screening of different chiral ligands including ProPhenol ligands (L2-L5) and AzePhenol ligands (L6-L8) indicated that L4 bearing 4-CF3C6H4 groups was the best ligand [Table 1, entries 2-8]. Subsequently, the examination of solvent effect demonstrated that MeCN could give a high ee value of 84% [Table 1, entries 9-13]. Unfortunately, raising or lowering the reaction temperature failed to optimize the efficiency and stereoselectivity. [Table 1, entries 14-17]. Finally, we turned our attention to investigating the catalyst loading, and found that by utilizing 5 mol % of ligand L1 and 10 mol % of ZnEt2, the yield and stereoselectivity could be improved to a high level (82% yield, 88% ee, 3:1 dr) [Table 1, entries 18-20].
Condition ptimizationa
Entry | L | solvent | x | T (oC) | yieldb (%) | drc | eed (%) |
1 | L1 | THF | 10 | 30 | 56 | 1.5:1 | 39 |
2 | L2 | THF | 10 | 30 | 54 | 3.5:1 | 35 |
3 | L3 | THF | 10 | 30 | 61 | 3.3:1 | 69 |
4 | L4 | THF | 10 | 30 | 65 | 3.5:1 | 77 |
5 | L5 | THF | 10 | 30 | 55 | 3.1:1 | 37 |
6 | L6 | THF | 10 | 30 | 45 | 1.1:1 | 37 |
7 | L7 | THF | 10 | 30 | 39 | 1.2:1 | 23 |
8 | L8 | THF | 10 | 30 | 49 | 1:1 | 37 |
9 | L4 | PhMe | 10 | 30 | 56 | 2.8:1 | 60 |
10 | L4 | MeCN | 10 | 30 | 71 | 3:1 | 84 |
11 | L4 | CH2Cl2 | 10 | 30 | 65 | 2.7:1 | 75 |
12 | L4 | CHCl3 | 10 | 30 | 70 | 1.7:1 | 59 |
13 | L4 | PhCF3 | 10 | 30 | 60 | 2.1:1 | 63 |
14 | L4 | MeCN | 10 | 0 | 46 | 1.5:1 | 40 |
15 | L4 | MeCN | 10 | 10 | 69 | 3:1 | 80 |
16 | L4 | MeCN | 10 | 40 | 71 | 3:1 | 84 |
17 | L4 | MeCN | 10 | 50 | 69 | 3:1 | 82 |
18 | L4 | MeCN | 3 | 30 | 54 | 3:1 | 85 |
19 | L4 | MeCN | 5 | 30 | 82 | 3:1 | 88 |
20 | L4 | MeCN | 15 | 30 | 74 | 3:1 | 80 |
With the best conditions in hand, the scope of both nitroenones and α-hydroxy-1-indanones for the projected reaction was examined [Scheme 3]. Firstly, the Ar1 group of nitroenones was studied. A range of ortho-, meta-, para-substituted phenyl substrates 2 had been successfully employed to afford the corresponding products 3b-3f in 56%-74% yields, 1.5:1-8.3:1 diastereoselectivities and 82%-94% enantioselectivities. In addition, 1-naphthyl group was also well tolerated, giving the desired product 3g in 54% yield, 4.5:1 dr and 82% ee value. Next, the scope of Ar2 group appended to the double bond of nitroenones was investigated. As illustrated in Scheme 3, both electron-rich and electron-deficient aryl groups could be tolerated, delivering the corresponding products 3h-3k in 54%-79% yields, 2.8:1-9:1 diastereoselectivities and 79%-91% enantioselectivities. Furthermore, incorporating a heteroaromatic group (Ar2 = 2-furyl) or a sterically bulky group (Ar2 = 1-naphthyl) did not affect the efficiency of the reaction (3l and 3m). Currently, only nitroenones bearing different aromatic substituents have been examined. Subsequently, we investigated the substrate generality of α-hydroxy-1-indanones by reacting them with 1-(2-methoxyphenyl)-2-nitro-3-phenylprop-2-en-1-one 2f. Different substituents (from electron-donating to electron-withdrawing) at the C-4 to 6 positions of α-hydroxy-1-indanones 1 participated in the cascade reactions to give the desired products 3n-3x in 62%-81% yields, 4.3:1-13:1 diastereoselectivities and 86%-96% enantioselectivities. Notably, the absolute configuration of the major isomer of product 3f was determined by the X-ray crystallographic analysis and that of other products was assigned by analogy[27].
Scheme 3. Reaction scope. Reaction conditions: Unless otherwise noted, all reactions were conducted with 5 mol% of L4, 10 mol % of ZnEt2, 0.20 mmol 1 and 0.20 mmol 2 in 2 mL MeCN. Isolated yields. The diastereomeric ratio parameter of 3 was detected by 1H NMR of the crude reaction mixture. The enantiomeric excess (ee) value was determined by HPLC analysis.
To showcase the synthetic utility of this protocol, a gram-scale synthesis of 3f was carried out by using 5 mmol of 1a and 5 mmol of 2f. Under the standard condition, the reaction proceeded smoothly to give product 3f in 69% yield (1.48 g) with 10:1 dr and 93% ee [Scheme 4A]. Further reduction of nitro group with the NiCl2/NaBH4 system and hydrolysis of the ester group took place in one-pot and afforded the indeno[1,2-b]pyrrol-(3H)-ol derivative 5 [Scheme 4B].
As previously described, a plausible reaction mechanism for this acyl transfer-enabled catalytic asymmetric Michael addition was illustrated in Scheme 5. Firstly, α-hydroxy-1-indanone 1a and nitroenone 2a were coordinated to the two zinc atoms in the chiral pocket of the dinuclear zinc complex in a less hindered manner. Then, the enantioselective Michael addition led to the intermediate B. Next, an intramolecular hemiketalization proceeded in the same chiral pocket to give intermediate C, which underwent the retro-Claisen reaction to afford the complex D. Finally, the catalytic cycle was restarted after a proton exchange of intermediate D with another α-hydroxy-1-indanone 1a, followed by the release of product 3a.
DFT calculations were performed on the enantioselectivity-determining step to elucidate the origins of selectivity. Model catalyst IN1 [Figure 2] was used for the calculation. The ligand exchange of substrate 1a and ethane leads to IN2, which is exergonic by 21.5 kcal/mol, followed by the Michael addition step. Our calculations show that the pathway leading to the major RR-enantiomer (via transition state TS3-RR) has a free energy barrier of 13.8 kcal/mol, which is 2.3 kcal/mol more favorable than that leading to the minor SS-enantiomer (via transition state TS3-SS) and agrees with experiment. Detailed analyses indicate that there is favorable C-H…π interactions between the C-H bond adjacent to C1 in 1a and the phenyl ring connecting to C2 in 2a (2.69 Å, Figure 2) in TS3-RR. On the other hand, however, unfavorable steric repulsion was found between hydrogen atoms of the two substrates in TS3-SS (the closest H-H distance is 2.04 Å, Figure 2). The steric repulsion between the two substrates in TS3-SS would retard the C1-C2 bond formation, as manifested by the much longer C1-C2 distance in TS3-SS (2.29 Å) than in TS3-RR (2.16 Å). Thus, our computations reveal that both the C-H…π interaction in TS3-RR and the steric effect in TS3-SS account for the observed enantioselectivity.
Figure 2. The calculated free energy profile and geometries of the key transition states for the enantioselectivity-determining Michael addition step (calculations were performed at the
CONCLUSION
In conclusion, we have disclosed a novel acyl transfer-enabled catalytic asymmetric Michael reaction of α-hydroxy-1-indanones with nitroolefins via an underexplored electrophilic mode of activation. The chemical event underwent a reaction cascade of dinuclear zinc-catalyzed asymmetric Michael addition, intramolecular cyclization, and the retro-Claisen reaction. Good yields and stereoselectivities of the desired products were obtained with a wide substrate scope under mild conditions. In the activation mode, the dinuclear zinc complex acted as a bifunctional catalyst, wherein one zinc atom worked as a Lewis acid and the another functioned as a Brønsted base. Further applications of this catalytic asymmetric acyl transfer via electrophile activation for the synthesis of polyfunctional heterocycles are ongoing in our laboratory.
DECLARATIONS
Authors’ contributions
Designing the experiments, writing the manuscript, and being responsible for the whole work: Jia SK, Wang MC, Mei GJ
Performing the experiments: Xu ZH, Hua YZ
Synthesizing the substrates: Chang ZR
DFT calculations: Li N, Xu LP
Availability of data and materials
Supplementary materials are available online for this paper.
Financial support and sponsorship
Financial support from the Natural Science Foundation of Henan Province (222300420084, 222300420292), China Postdoctoral Science Foundation (2022M712862), application research plan of Key Scientific Research Projects in Colleges and Universities of Henan Province (22A150056), and Zhengzhou University (JC22261005) are gratefully acknowledged.
Conflicts of interest
All authors declared that there are no conflicts of interest.
Ethical approval and consent to participate
Not applicable.
Consent for publication
Not applicable.
Copyright
© The Author(s) 2023.
Supplementary Materials
REFERENCES
1. Burke HM, McSweeney L, Scanlan EM. Exploring chemoselective S-to-N acyl transfer reactions in synthesis and chemical biology. Nat Commun 2017;8:15655.
2. Rodriguez J, Quintard A. Acyl transfer strategies as transient activations for enantioselective synthesis. Synthesis 2019;51:1923-34.
3. Gao Y, Ren Q, Siau WY, Wang J, Asymmetric organocatalytic cascade Michael/ hemiketalization/retro-Henry reaction of β, γ-unsaturated ketoesters with α-nitroketones. Chem Commun 2011;47:5819-21.
4. Lu RJ, Yan YY, Wang JJ, Du QS, Nie SZ, Yan M. Organocatalytic asymmetric conjugate addition and cascade acyl transfer reaction of α-nitroketones. J Org Chem 2011;76:6230-9.
5. Liu Y, Wang Y, Song H, Zhou Z, Tang C. Asymmetric organocatalytic cascade Michael/hemiketalization/retro-aldol reaction of 2-[(E)-2-nitrovinyl]phenols with 2,4-dioxo-4-arylbutanoates: A convenient access to chiral α-keto esters. Adv Synth Catal 2013;355:2544-9.
6. Zhou J, Jia LN, Wang QL, et al. Organocatalytic asymmetric cascade Michael/hemiketalization/retro-aldol reaction of 3-acetyl-oxindole with β,γ-unsaturated ketoesters catalyzed by bifunctional amino-squaramides. Tetrahedron 2014;70:8665-71.
7. Maity R, Gharui C, Sil AK, Pan SC. Organocatalytic asymmetric Michael/hemiketalization/ retro-aldol reaction of α-nitroketones with unsaturated pyrazolones: synthesis of 3-acyloxy pyrazoles. Org Lett 2017;19:662-5.
8. Gharui C, Behera D, Pan SC. Organocatalytic asymmetric domino Michael/acyl transfer reaction between α-nitroketones and in situ-generated ortho-quinone methides: Route to 2-(1-arylethyl)phenols. Adv Synth Catal 2018;360:4502-8.
9. Mondal K, Pan, SC. Organocatalytic asymmetric domino Michael/acyl transfer reaction between gamma/delta-hydroxyenones and alpha-nitroketones. J Org Chem 2018;83:5301-12.
10. Song YX, Du DM. Bifunctional squaramide-catalysed asymmetric Michael/hemiketalization/retro-aldol reaction of unsaturated thiazolones with α‐nitroketones: Synthesis of chiral 4-acyloxythiazole derivatives. Adv Synth Catal 2019;361:5042-9.
11. Biswas RG, Ray SK, Unhale RA, Singh VK. Organocatalytic asymmetric cascade Michael-acyl transfer reaction between 2-fluoro-1,3-diketones and unsaturated thiazolones: Access to fluorinated 4-acyloxy thiazoles. Org Lett 2021;23:6504-9.
12. Roudier M, Constantieux T, Quintard A, Rodriguez J. Enantioselective cascade formal reductive insertion of allylic alcohols into the C(O)-C bond of 1,3-diketones: ready access to synthetically valuable 3-alkylpentanol units. Org Lett 2014;16:2802-5.
13. Quintard A, Rodriguez J. Organo- and Iron(0) Catalysis for an enantioselective michael addition-hemiketalization-fragmentation sequence to protected ω-hydroxy-nitroketones. Adv Synth Catal 2016;358:3362-7.
14. Zhu Y, Zhang L, Luo S. Asymmetric retro-Claisen reaction by chiral primary amine catalysis. J Am Chem Soc 2016;138:3978-81.
15. Han Y, Zhang L, Luo S. Asymmetric retro-Claisen reaction by synergistic chiral primary amine/palladium catalysis. Org Lett 2019;21:7258-61.
16. Han Y, Zhang L, Luo S. Highly stereoselective construction of β, β-diaryl-α-branched ketones by the chiral primary amine-catalyzed asymmetric retro-Claisen reaction. Org Lett 2022;24:1752-6.
17. Yi ZY, Xiao L, Chang X, Dong XQ, Wang CJ. Iridium-catalyzed asymmetric cascade allylation/retro-Claisen reaction. J Am Chem Soc 2022;144:20025-34.
18. Zhou Y, Wei YL, Rodriguez J, Coquerel Y. Enantioselective organocatalytic four-atom ring expansion of cyclobutanones: synthesis of benzazocinones. Angew Chem Int Ed Engl 2019;58:456-60.
19. Yang WL, Li W, Yang ZT, Deng WP. Organocatalytic regiodivergent ring expansion of cyclobutanones for the enantioselective synthesis of azepino[1,2-a]indoles and cyclohepta[b]indoles. Org Lett 2020;22:4026-32.
20. Yang WL, Wang YL, Li W, et al. Diastereo- and enantioselective synthesis of eight-membered heterocycles via an allylation/ring expansion sequence enabled by multiple catalysis. ACS Catal 2021;11:12557-64.
21. Zhang MM, Chen P, Xiong W, Hui XS, Lu LQ, Xiao WJ. A dipolar cyclization/fragmentation strategy for the catalytic asymmetric synthesis of chiral eight-membered lactams. CCS Chem 2022;4:2620-9.
22. Parida C, Maity R, Chandra Sahoo S, Chandra Pan S. α-Nitro-β, β-unsaturated ketones: An electrophilic acyl transfer reagent in catalytic asymmetric friedel-crafts and Michael reactions. Org Lett 2019;21:6700-4.
23. Yang WP, Jia SK, Liu TT, Hua YZ, Wang MC. Dinuclear zinc-catalyzed asymmetric [3 + 2] cyclization reaction for direct assembly of chiral α-amino-γ-butyrolactones bearing three stereocenters. Org Chem Front 2021;8:6998-7003.
24. Han JJ, Zhang C, Mei GJ, Hua YZ, Jia SK, Wang MC. Zinc-catalyzed asymmetric [3 + 2] annulations for the construction of chiral spiro[1-indanone-γ-butyrolactones] via a C-N bond cleavage process. Org Chem Front 2022;9:5819-24.
25. Pellissier H. Recent developments in enantioselective zinc-catalyzed transformations. Coord Chem Rev 2021;439:213926.
26. Trost BM, Hung CJ, Mata G. Dinuclear metal-prophenol catalysts: Development and synthetic applications. Angew Chem Int Ed Engl 2020;59:4240-61.
27. Cambridge Crystallographic Data Centre. CCDC 2097558 for the major isomer of 3f. Available from: https://www.ccdc.cam.ac.uk/ [Last accessed on 24 Mar 2023]
Cite This Article
Export citation file: BibTeX | RIS
OAE Style
Xu ZH, Li N, Chang ZR, Hua YZ, Xu LP, Jia SK, Wang MC, Mei GJ. Acyl transfer-enabled catalytic asymmetric Michael addition of α-hydroxy-1-indanones to nitroolefins. Chem Synth 2023;3:17. http://dx.doi.org/10.20517/cs.2022.35
AMA Style
Xu ZH, Li N, Chang ZR, Hua YZ, Xu LP, Jia SK, Wang MC, Mei GJ. Acyl transfer-enabled catalytic asymmetric Michael addition of α-hydroxy-1-indanones to nitroolefins. Chemical Synthesis. 2023; 3(2): 17. http://dx.doi.org/10.20517/cs.2022.35
Chicago/Turabian Style
Xu, Zhi-Hua, Na Li, Zhe-Ran Chang, Yuan-Zhao Hua, Li-Ping Xu, Shi-Kun Jia, Min-Can Wang, Guang-Jian Mei. 2023. "Acyl transfer-enabled catalytic asymmetric Michael addition of α-hydroxy-1-indanones to nitroolefins" Chemical Synthesis. 3, no.2: 17. http://dx.doi.org/10.20517/cs.2022.35
ACS Style
Xu, Z.H.; Li N.; Chang Z.R.; Hua Y.Z.; Xu L.P.; Jia S.K.; Wang M.C.; Mei G.J. Acyl transfer-enabled catalytic asymmetric Michael addition of α-hydroxy-1-indanones to nitroolefins. Chem. Synth. 2023, 3, 17. http://dx.doi.org/10.20517/cs.2022.35
About This Article
Special Issue
Copyright
Author Biographies
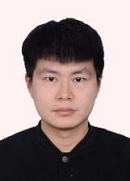
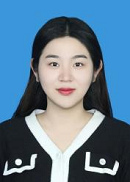
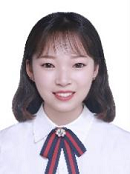
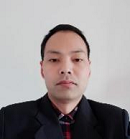
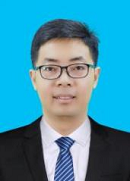
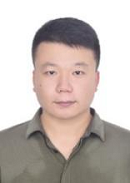
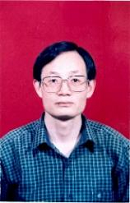
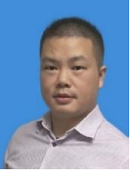
Data & Comments
Data
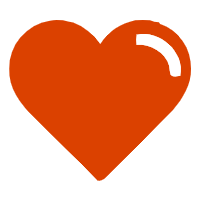

Comments
Comments must be written in English. Spam, offensive content, impersonation, and private information will not be permitted. If any comment is reported and identified as inappropriate content by OAE staff, the comment will be removed without notice. If you have any queries or need any help, please contact us at support@oaepublish.com.