Prediction of embodied GHG emissions of building materials in Shanghai
Abstract
The construction sector stands as a fundamental component of urban development, providing indispensable spaces and infrastructure critical to societal demands. However, the consumption of considerable building materials engenders a significant environmental footprint, mainly from the exploitation, manufacturing, and processing. Characterizing the dynamic changes in a city’s future building development and material demand is crucial for achieving the goal of carbon neutrality. As an international metropolis, Shanghai faces challenges, including limited land and resources, high population density, and rapid building renewal rates. Understanding building renewal patterns and developing potential urban mineral resources will help alleviate resource shortages, achieve sustainable use of building materials, and meet carbon reduction goals. Taking Shanghai as a case study, this research applies material flow analysis alongside the carbon emission factor method to forecast the consumption of building materials and the associated embodied carbon emissions within the city. The study meticulously quantifies the evolving patterns of building material consumption and embodied carbon emissions from 2023 to 2060. The results indicate that Shanghai's civil building stock area will undergo incremental growth, attaining a pinnacle in 2030 before embarking downward. In contrast, the scope of new construction and demolition within the urban fabric will follow an ascending trend throughout the same period. Regarding material consumption, gravel, cement, and bricks are identified as the predominant components, accounting for 58%, 18%, and 15% of the total material inflow, respectively. Embodied carbon emissions from Shanghai’s civil buildings are anticipated to rise steadily, mirroring the pattern of material consumption. Notably, emissions from residential buildings are expected to experience a slight decline from 2023 to 2028, ultimately reaching 5.43 Million metric tons (Mt) in 2060. The insights garnered from this research are instrumental in deepening the comprehension of urban development dynamics and the intricacies of resource management.
Keywords
INTRODUCTION
As global warming and extreme weather events become more frequent, the Intergovernmental Panel on Climate Change (IPCC) has underscored the urgent need for accelerated efforts to reduce the consumption of building materials[1]. The construction industry, one of the three significant sources of energy consumption in China and a primary source of carbon emissions, plays a crucial role in this endeavor. The production of building materials, a critical stage in the carbon emission of buildings, can account for 1/4 of the total national energy consumption[2]. China, as the largest global producer of bulk materials, confronts formidable challenges in mitigating greenhouse gas emissions arising from their production[3]. Due to the increase in carbon emissions caused by the production of primary materials and the depletion of related natural resources, improving the efficiency of materials and prolonging the demand for service life has become a global necessity. As one of the most important sources of urban mining, the building sector is responsible for 39% of greenhouse gas (GHG) emissions globally and 25% of total solid waste generated worldwide. As the economy expands and urbanization progresses, the number of residential buildings continues to rise, significantly impacting carbon emissions. Understanding the entire building process - including extraction, manufacturing, transportation, construction, maintenance, and disposal - is crucial for mitigating CO2 emissions. Buildings utilize various materials that consume energy and emit CO2 throughout their life cycle, collectively referred to as embodied energy and embodied carbon. In 2018, the annual embodied carbon of building materials in China reached 1,757 Mt. Notably, cement, steel, brick, lime, and linoleum constituted 34.0%, 20.2%, 24.7%, 13.4%, and 3.4% of the total embodied carbon, respectively[4]. Evaluating the embodied carbon of building materials is a key strategy for reducing the carbon footprint. Choosing sustainable materials can reduce embodied CO2 emissions by approximately 30% over a building's lifespan. Additional strategies, such as substituting low-carbon materials, optimizing material use, and prioritizing locally sourced materials, can further aid in carbon reduction. Implementing a cascaded strategy has been shown to potentially decrease the average embodied carbon intensity by 28.8%[5]. Therefore, to better manage the materials and improve the material efficiency, it is essential to construct a systematic flow framework diagram of building materials[6].
The material flow analysis model, grounded in the principle of mass conservation, enables the forecasting of material stocks and flows throughout 20 to 100 years. Estimations can be made in two different ways, which have been characterized as bottom-up and top-down approaches. The bottom-up approach divides dwelling vintages into different categories. For each category and vintage class, a typical representative is chosen to analyze the material composition of different building elements. The top-down approach uses information about the annual consumption of materials used for new dwelling construction, divided by the annually produced applicable floor area[7]. Recently, this method has gained widespread application for estimating material stocks in residential construction and infrastructure projects[8,9], shifting gradually from static material flow models to dynamic models, moving away from previous singular approaches of top-down or bottom-up methods to combining both approaches. For example, Zhang et al. conducted a bottom-up stock analysis of cement in Shenzhen, China[10]. Using dynamical materials flow analysis, Cheng et al. studied the historical evolution of steel stock in residential buildings in the Yangtze River Delta, China[7]. Through a bottom-up approach, Liu et al. also explored steel stocks and their distribution in Chongqing, China[11]. Muller et al. utilized the dynamic material flow analysis model by combining the bottom-up and top-down approaches to analyze concrete diffusion in the Dutch dwelling stock from 1900 to 2100[12]. Long et al. established a 4D GIS-MFA-LCA model by combining the bottom-up and top-down approaches to address these gaps for a detailed characterization of the Macao urban building stocks and flows’ quantity, quality, and temporal-spatial distribution[13].
In the examination of embodied carbon emissions in buildings, Wang et al. and Wiik et al. conducted assessments of the carbon footprint associated with building facade materials and explored potential reduction strategies, including innovative facade design approaches[14,15]. Bai et al. classified and sorted the calculation parameters of infrastructure material stock and embodied carbon emissions. It estimated the trends and magnitude of 31 provinces for 20 years (1997-2016) and analyzed the spatial-temporal characteristics[16]. Shirazi and Ashuri undertook a comparative analysis of embodied carbon emissions arising from retrofit interventions in single-family homes in Atlanta. Their study juxtaposed the embodied emissions associated with various retrofit measures to enhance operational energy efficiency across different building categories[17]. Yan et al. have observed that concrete and steel constitute approximately 94%-95% of the total embodied carbon emissions from building materials[18]. By utilizing recycled materials, it is possible to reduce the use of virgin materials in subsequent stages of construction, thereby substantially mitigating embodied carbon emissions[19].
Previous studies have primarily focused on overall building energy use and emissions without delving into the specific functional attributes that drive variations in carbon footprints across different types of buildings. Research about building materials and their embodied carbon emissions has predominantly concentrated on accounting for carbon emissions. However, the embodied carbon emission forecasting field remains underdeveloped, with remarkably scant research at a city level, which is currently in an incipient, exploratory phase[20,21]. Most studies have focused on one or a few metal resources, lacking a more comprehensive analysis at a long-term scale, and previous studies have paid more attention to the carbon emissions from existing buildings, frequently neglecting the embodied carbon emissions of newly built buildings in the future for forecasting. To address this research gap, this study selected the economic and population development patterns and the variation in per capita building area under the SSP2 scenario, combined top-down and bottom-up methods, categorized the functional attributes of civil building types at the city scale, and made long-term dynamic predictions regarding the trends in implied carbon emissions from non-metallic and metallic building materials across different building types.
Predicting future carbon emissions related to building materials helps formulate effective emission reduction strategies, thereby mitigating the pace of climate change. It assists governments in setting more reasonable carbon emission targets and devising feasible emission reduction policies and measures. Additionally, predicting resource demand and carbon emissions facilitates the adoption of more environmentally friendly and low-carbon materials and technologies in the construction industry. It focuses resources on developing alternative materials or improving the production processes of existing materials to reduce carbon emissions, providing crucial data support and a scientific basis for the sustainable development of the entire construction industry. This study also provides a long-term dynamic prediction of the implicit carbon emission trends of non-metallic and metallic building materials across different building types, which will help to identify effective pathways to improve the resource efficiency of building materials and provide basic data to support future research on the carbon reduction potential of building materials.
As a cosmopolitan city, Shanghai faces multiple challenges, including limited land and resources, high population density, and a rapid rate of building renewal[22]. Understanding how the existing stock of building materials can be utilized could help reduce the need for new building raw materials, thereby reducing carbon emissions through urban mineral resource development, efficient resource management, and utilization. In addition, Shanghai represents economic and social development, and its urban building regeneration and changes will serve as a reference and model for other similar cities. This study establishes a dynamic prediction model for Shanghai's building material consumption and embodied carbon emissions, utilizing population and per capita floor space forecasts derived from the SSP2 baseline scenario of the IMAGE model[23]. To project the future trajectory of construction material consumption and the associated embodied carbon emissions in Shanghai, material flow analysis is employed to assess the anticipated demand for these materials from 2023 to 2060[24,25]. Furthermore, it offers strategic recommendations for diminishing the embodied carbon emissions of Shanghai’s construction sector. The emission reduction strategies delineated herein may serve as a valuable reference for urban centers worldwide seeking to curtail carbon emissions associated with construction materials. By providing insights into the primary drivers of carbon emissions in the context of high population density and rapid urbanization, these strategies enable cities to better understand and address their unique challenges. Drawing on Shanghai’s experience, other urban centers can adopt and adapt effective carbon reduction practices, thereby formulating more targeted and impactful policies and measures to promote sustainable development. This study delineates the dynamic changes in building materials in Shanghai from 2023 to 2060, providing valuable insights that can enhance the efficiency of resource utilization in construction materials. By understanding these trends, urban planners and policymakers can develop strategies to optimize material use, reduce waste, and lower carbon emissions, thereby promoting more sustainable development practices. This comprehensive analysis also supports the adoption of innovative materials and technologies, fostering an environment that encourages continuous improvement and adaptation to future challenges in urban construction.
METHODS
Research framework
This study delineates residential and non-residential buildings in Shanghai as the system boundary and concentrates on the embodied carbon emissions attributable to the consumption of building materials during the construction phase of a building's life cycle. A predictive model for the consumption of building materials and the associated embodied carbon emissions in Shanghai is developed, employing material flow analysis and the carbon emission factor method. The model forecasts the trends in construction material consumption and embodied carbon emissions for 2023-2060 while also suggesting strategies for emission reduction. The SSP2 baseline scenario, which assumes medium growth in Shanghai’s population and GDP, is selected for the study. The forecast data on population and per capita floor space from this scenario enable the model to represent the future trends in Shanghai's construction sector objectively. The model is structured into three components: the forecast of civil building stock area, estimating building material inflow and consumption, and projecting embodied carbon emissions from construction activities. The study’s approach to quantifying the material metabolism of construction materials is informed by previous research at both the national level and within Shanghai[26]. It examines seven fundamental construction materials: cement, iron and steel, timber, lime, bricks, gravel, and glass. The analysis focuses on civil buildings, segmented into six distinct categories - residential buildings, educational buildings, office buildings, commercial buildings, healthcare buildings, and others (as shown in Figure 1). These categories are delineated following the classification standards for residential and non-residential buildings as outlined in historical documentation such as 1949-2019 Glorious Seventy Years and the “Shanghai Municipal Statistical Yearbook”[27]. This study introduces a new classification framework for building functional attributes, which provides a more accurate basis for carbon emission predictions compared to traditional classification methods. We developed a dynamic prediction model that accounts for long-term changes in building functions and usage patterns, offering a more reliable long-term forecast of carbon emissions.
Projecting building floor area stock
The IMAGE model is used to support a variety of international assessments, including IPCC assessments, the United Nations Environment Programme’s Global Environment Outlook, and the Organization for Economic Cooperation and Development's Environment Outlook[28], and different shared socio-economic pathways (SSPs) are modeled in IMAGE, reflecting the likely future development trends of global
The building area stock is calculated by using the population to multiply the per capita floor area. Per capita floor area projections for China during 2023-2060 are sourced from the Image model. We used China's per capita floor area trend from 2023 to 2060 and Shanghai’s data from 2000 to 2021 to forecast Shanghai's per capita floor area from 2023 to 2060. Population projections for Shanghai during 2023-2060 are derived from Chen et al.[30]. Their estimation considers the demographic characteristics (including sex, age, and seven educational attainment categories) under various Shared Socio-economic Pathways (SSPs) and national policies regarding fertility and population ceiling. We use the SSP2 projection, which depicts a “middle-of-the-road” future where demographic characteristics broadly follow their historical patterns. Due to its representation of a moderate path that is neither overly optimistic nor overly pessimistic, this scenario allows for balanced and realistic projections. SSP2 assumes that social, economic, and technological trends will continue without significant shifts, making it a valuable baseline for comparison with other extreme scenarios. However, it has limitations in capturing the potential impacts of significant positive or negative deviations from historical trends and might lead to complacency in addressing urgent climate policy interventions.
where
Building material inflow
Materials flow analysis is valuable for resource, waste, and environmental management. It is widely utilized for calculating material flows and stocks in the building sector, employing bottom-up and top-down methods to estimate building material stocks[18].
The annual new construction area in the model equals the sum of the difference between the building stock area and the demolition area (Equation 2).
The demolition area of the house in the model is calculated using the life distribution function to establish the obsolescence curve (Equation 3). Based on the existing literature and the actual situation of building demolition in China[31,32], this study determines the average life span of urban buildings in Shanghai to be 40 years. For the life span of the building, a normal distribution function is chosen to fit the curve of the building's life span (Equation 4).
The annual consumption of new building materials in the construction industry is obtained by multiplying the area of new building construction each year and the building material intensity (Equation 5).
Where
Embodied GHG emissions calculation
The Carbon Emission Factor (CEF) method is currently the most widely used method[33], derived from the 2006 IPCC Guidelines for National Greenhouse Gases. It can be used to quantify GHG emissions per unit of activity. The basic idea of the method is to construct activity data and emission factors for each emission source and use the two products as the source's carbon emission estimate[34]. This study uses the carbon emission factor method to account for the embodied carbon emissions from building materials in Shanghai, combined with the data information published in the Construction Industry Statistical Yearbook. The embodied carbon emissions from the consumption of building materials can be obtained by summing up the products of the inflows of various primary building materials and their corresponding carbon emission factors, as shown in Equation 6.
Whererefers to the total embodied carbon emissions of material n in building type m, and is a value that is calculated over the emissions of material n for all construction sectors; refers to the embodied carbon emission factor for material n in the construction sector m;refers to the inflow of material n in the construction industry m.
Data sources
The sources of data required for this study are shown in Table 1. Historical data on building area are derived from the “Shanghai Statistical Yearbook 1996-2022”[20], and population and per capita floor space projections required for floor space projections are derived from the IMAGE model’s SSP2 scenario[35], shown in Figure 2. Building material intensity is based on the primary material consumption standards for construction projects “Building Construction Manual”[36] and literature data[37,38], shown in Figure 3. The carbon emission factors for the production phase of building materials are taken from the building emission calculation standard GBT51366-2019[39], and the corresponding carbon emission factors are shown in Figure 4.
Figure 2. (A) the population of Shanghai from 2000 to 2060; (B) the building floor area per capita from 2000 to 2060.
Data source
Data | Data source |
Historical data on floor space | Shanghai statistical yearbook 1996-2022[20] |
Population projection data | SSP2 scenarios for the IMAGE model[33] |
Projected data on floor space per capita | SSP2 scenarios for the IMAGE model[33] |
Building material intensity | Building construction manual[29] and literature data[30,31] |
Carbon emission factors for building materials | Standard for building carbon emission calculation GBT51366-2019[37] |
RESULTS
Analysis of the stock and flow building floor area
Between 2023 and 2030, the expanse of Shanghai's civil building stock is projected to increase gradually, as depicted in Figure 5, culminating in a peak area of 99.245 million m2 in 2030. Subsequently, a year-on-year decline is anticipated, with the area expected to diminish to 82.962 million m2 by 2060. The extent of the residential building stock is intrinsically linked to demographic factors, such as population and per capita floor area, economic conditions, and urban development policies. With the per capita floor space in Shanghai expected to sustain its growth trajectory and the city's population projected to reach its zenith around 2030, it is anticipated that these demographic trends, along with economic and policy factors, will significantly influence the peak of the civil building stock area in 2030.
From 2023 to 2060, the new area of civil buildings in Shanghai shows a general growth trend. From 2023 to 2051, the area of new civil construction will increase, reaching a peak of 31,765,100 m2 in 2051. This growth can be attributed to economic expansion, urban development policies, and population increases driving the demand for new buildings. However, after 2051, the new floor space begins to decline annually, eventually dropping to 20,217,100 m2 by 2060. This decline may result from demographic stabilization, advancements in construction technology, and a shift toward sustainability efforts, leading to more efficient use of existing buildings and reduced need for new construction [Figure 6].
The peak construction for new residential and non-residential buildings occurs in 2051 and 2049, reaching 22.72 and 9.48 million m2, respectively. By 2060, these figures are projected to decline to
Building material consumption
Expanding new construction areas is a predominant factor influencing the consumption of building materials for civil structures within Shanghai. Over the period spanning from 2023 to 2060, there is a substantial increase in the consumption of these materials, escalating from 6.04 to 19.49 Mt, representing an increase of more than threefold. The trend towards increased consumption is vividly illustrated by the peak in 2051, where material usage reaches 29.58 Mt, as depicted in Figure 8. Several factors contribute to this sustained growth in material consumption: economic progress and the implementation of urban renewal initiatives have amplified the demand for contemporary and resilient buildings, thereby increasing material requirements. Additionally, cutting-edge technologies and the adoption of stringent construction standards have necessitated increasingly sophisticated materials, further increasing consumption levels. The trajectory of material consumption for residential structures is markedly upward, culminating in a peak of 22.24 Mt in 2051. This ongoing rise in material utilization for residential construction is attributed to persistent housing demand driven by demographic growth and rising living standards, which, in turn, stimulate construction activities.
Figure 8. Building materials consumption for residential and public buildings in Shanghai from 2023 to 2060.
Conversely, the consumption pattern for non-residential structures exhibits more moderate growth, initially experiencing a minor annual reduction from 0.97 to 0.87 Mt during 2023-2026. However, from 2027 onwards, a yearly increase in material consumption is observed, with a pronounced surge between 2027 and 2050 coinciding with the revitalization of non-residential construction projects. After 2051, the pace of urban renewal construction reaches a plateau, and the consumption of materials gradually decreases, descending to 1.06 Mt by 2060. Examining the categories of construction materials shown in Figure 9, gravel, cement, and bricks emerge as the leading constituents of total material inflow, representing 58%, 18%, and 15% of the total, respectively.
Buildings embodied GHG emissions
The predictive analysis indicates a correlation between the trend of embodied carbon emissions from civil building materials in Shanghai and the annual consumption of these materials. An initial marginal decline in carbon emissions is observed from 2023 to 2025. However, a steady increase ensues from 2029, with emissions escalating from 1.24 Mt in 2029 to 5.43 Mt by 2060. Notably, the peak of embodied carbon emissions from residential buildings is projected for 2051 at 8.87 Mt, followed by a decrease to 5.43 Mt by 2060 (as shown in Figure 10). The material flow analysis reveals that the building stock and demolition activities substantially affect new construction, with the timing of a building's carbon emission peak closely linked to its lifespan. Specifically, a shorter lifespan precipitates an earlier peak in emissions from the production of building materials. Embodied carbon emissions per unit of civil floor area are anticipated to peak in 2051 at 9.66 kgCO2/m2, subsequently declining to 6.55 kgCO2/m2 by 2060. Residential buildings exhibit higher embodied carbon emissions than non-residential buildings when comparing building types. The peak emissions for residential buildings are forecasted for 2051 at 6.09 Mt, with a gradual reduction to 5.05 Mt by 2060. The per unit area emissions for residential buildings are expected to peak in 2055 at
Figure 10. Embodied carbon emission projections for residential and public buildings in Shanghai from 2023 to 2060.
Conversely, the peak for non-residential buildings is anticipated in 2049 at 2.91 Mt, with a decline to
Figure 11. Prediction of embodied carbon emissions of different building materials for civil buildings in Shanghai from 2023 to 2060.
Within the spectrum of carbon emissions attributed to various building materials, cement, steel, and bricks represent the most significant contributors, accounting for 43%, 31%, and 13% of emissions due to the high materials and energy consumption during the production phase, respectively. Lime follows these materials, while emissions associated with gravel and glass are comparatively minimal, each constituting less than 5% of the total. The predominant emissions from cement and bricks primarily result from their extensive annual consumption. Conversely, emissions from iron and steel are primarily driven by their substantial carbon emission factors. Consequently, cement and steel emerge as the construction sector's primary sources of embodied carbon emissions. Consequently, strategies to reduce emissions should prioritize these specific materials, concentrating on the formulation and execution of effective abatement measures designed to lessen their environmental footprint[40].
DISCUSSION AND SUGGESTIONS
The variation of Shanghai's civil building stock is intimately linked to demographic variables, including population size and per capita floor area. As a significant factor influencing the dynamics of construction and demolition, the building stock offers a lever for the government to modulate per capita floor area expansion through macro-control policies. Such interventions provide critical leeway for the management of urban infrastructure and the facilitation of waste disposal processes. Anticipated trends suggest that Shanghai's per capita floor space will continue to expand, with the city's population projected to crest in the vicinity of 2030. This demographic shift will influence the civil building stock area, which is expected to reach its zenith in 2030 before entering a phase of gradual decline. Between 2023 and 2060, the new construction and demolition cycle within Shanghai’s civil building sector is forecasted to follow an overall uptrend, primarily driven by the city’s swift urbanization in recent years. This has precipitated a surge in new buildings and will eventually necessitate large-scale decommissioning, intensifying the demand for new construction. Future trends associated with construction and demolition activities within Shanghai's civil building sector are contingent upon the longevity of existing structures and the magnitude of new developments. Municipal authorities should enhance the precision of urban master planning and augment the utilization efficiency of the urban building stock. Addressing the aging buildings in the city's core, accelerating building renewal initiatives - like “green home” retrofits and the redevelopment of “two old villages” - is underway in Shanghai. These measures aim to protract the service life of buildings, alleviate the immediate demand for new construction, and consequently curtail the carbon emissions attributed to building materials at their origin.
Shanghai is projected to maintain a robust demand for construction materials in the foreseeable future. A strategic extension of the lifespan of buildings could mitigate the consumption of virgin resources and alleviate the tension between resource supply and demand. Analyzing the inflow of specific construction materials, gravel, cement, and bricks emerge as the predominant materials, succeeded by steel and lime, whereas wood and glass exhibit lower demand. The potential for more efficient use of building materials could be enhanced through consumer-driven practices, such as reducing housing vacancies, encouraging shared workspaces, and telecommuting. Proactive early warning systems are recommended to amplify the use of recycled construction materials before reaching the anticipated peak in construction waste generation, thereby diminishing material consumption. Considering the anticipated increase in material consumption, exploring more sustainable and efficient building materials and technologies is crucial.
The trajectory of embodied carbon emissions from building materials, both residential and non-residential, in Shanghai shows a direct correlation with the annual consumption of materials. The apex of embodied carbon emissions from civil buildings is expected in 2051, at 8.87 Mt, which surpasses the national target to peak carbon emissions by 2030. The elevated emissions from cement are primarily attributed to its substantial annual consumption, while steel's significant emissions stem from its high carbon emission factors. For materials such as cement and bricks, extensively consumed in residential construction, the emphasis should be on curtailing usage or sourcing alternative materials with a lower carbon footprint[41], such as recycled concrete, to minimize carbon emissions during production and utilization. Adopting lightweight structural designs presents a viable method to curtail the consumption of cement and bricks. Concurrently, alternative materials, such as the increased incorporation of wood, should be explored. For materials like steel, characterized by high annual consumption and carbon emission factors, particularly in non-residential construction, using low-carbon energy sources during manufacturing emerges as a pragmatic solution. Strengthening the recycling and reuse of construction materials and establishing comprehensive waste management systems are crucial steps. Encouraging the application of innovative technologies further enhances the efficiency of building materials, contributing to energy and resource conservation throughout the construction process. These combined measures align with sustainability objectives and support the goal of reducing carbon emissions in the built environment.
CONCLUSION
With the development of the economy and improving living standards in Shanghai, building material consumption has become a significant source of GHG emissions. This study utilized the materials flow analysis combined with the bottom-up and top-down methods to predict the newly built building floor area from 2023 to 2060 and summarized the metabolic pattern of building materials in Shanghai. Finally, we calculated the embodied carbon emissions of buildings by combining the consumption of building materials with their carbon emission factors. The results of this study will help cities take effective measures toward achieving a circular economy and highlight the role of such information and urban planning in urban building resources and carbon reduction.
Between 2023 and 2030, the expanse of Shanghai's civil building stock is projected to experience a gradual increase, culminating in a peak area of 99.245 million m2 in 2030. Subsequently, a year-on-year decline is anticipated, with the area forecasted to diminish to 82.962 million m2 by 2060.
From 2023 to 2060, there has been a substantial increase in the consumption of building materials, escalating from 6.04 to 19.49 Mt, which constitutes an increase of over threefold. Regarding the categories of building materials, gravel, cement, and bricks are the top three in total inflow, succeeded by steel and lime. The area designated for new construction will principally dictate the material consumption of civil buildings in Shanghai, with the projection indicating that the demand for building materials will surge, potentially tripling by 2060. Analyzing material consumption by building type, residential structures are expected to exceed non-residential ones within 2023 to 2060.
Upon analysis of the trajectory for embodied carbon emissions, it is projected that Shanghai’s civil buildings will demonstrate a rising trend in total emissions, with an increase from 1.75 Mt in 2023 to 5.43 Mt by 2060. The per unit area embodied carbon emissions of civil buildings stood at 1.78 kgCO2/m2 in 2023, with a projected incremental rise to a peak of 9.66 kgCO2/m2 in 2051, followed by a gradual decrease to
To achieve more efficient resource management and utilization and reduce carbon emissions associated with building materials, it is essential to understand the changes in urban building resource stocks and flows and to analyze their driving factors. Simultaneously, achieving carbon neutrality in the construction industry requires considering carbon reduction at each stage from a lifecycle perspective. This study examined the embedded carbon emissions related to building materials, considering only the carbon emissions during the production phase of the building lifecycle. In the future, we will incorporate carbon emissions accounting at buildings’ operational, demolition, and recycling stages and study the emission reduction pathways necessary for achieving carbon neutrality in the construction industry. Additionally, we will focus on the dynamic spatial variations in the stock and flow of building materials, utilizing tools such as spatio-temporal sequence analysis to characterize the spatial patterns of material flows[42]. This will provide a scientific basis for formulating effective carbon reduction strategies, thereby promoting the sustainable development of the construction industry.
DECLARATIONS
Author contributions
Writing - original draft, visualization, software, methodology, investigation, formal analysis, data curation: Long Y
Writing - review & editing, methodology, investigation, formal analysis: Cui H
Writing - review & editing, supervision, resources, investigation: Lu Z
Writing - review & editing, methodology, investigation: Huang B, Wang X
Conceptualization, writing - review & editing: Dou Y
Availability of data and materials
The data and code used in this study are available upon a reasonable request.
Financial support and sponsorship
This work was financially supported by the National Natural Science Foundation of China (52370197), the Programme of Special Appointment [Eastern Scholar] of the Shanghai Institute of Higher Learning [TP2020049], and MEXT/JSPS KAKENHI Grant Number JP21K14276.
Conflicts of interest
All authors declared that there are no conflicts of interest.
Ethical approval and consent to participate
Not applicable.
Consent for publication
Not applicable.
Copyright
© The Author(s) 2024.
REFERENCES
1. Bongaarts J. Global Warming of 1.5 °C. Switzerland: IPCC; 2019. pp. 251-2. Available from: https://ipcc.ch/sr15/ [Last accessed on 23 Jul 2024].
2. Heisel F, Mcgranahan J, Ferdinando J, Dogan T. High-resolution combined building stock and building energy modeling to evaluate whole-life carbon emissions and saving potentials at the building and urban scale. Resour Conserv Recy 2022;177:106000.
3. Song L, van Ewijk S, Masanet E, et al. China’s bulk material loops can be closed but deep decarbonization requires demand reduction. Nat Clim Chang 2023;13:1136-43.
4. Chen W, Yang S, Zhang X, Jordan ND, Huang J. Embodied energy and carbon emissions of building materials in China. Build Environ 2022;207:108434.
5. Zhang X, Li Y, Chen H, Yan X, Liu K. Characteristics of embodied carbon emissions for high-rise building construction: a statistical study on 403 residential buildings in China. Resour Conserv Recy 2023;198:107200.
6. Long Y, Li Z, Song Q, Cai K, Tan Q, Yang G. The dynamic stock-flow and driving force analysis of the building metal and non-metal resources at a city scale: an empirical study in Macao. Circ Econ 2022;1:100004.
7. Cheng C, Wen B. Historical evolution analysis of building steel stock in China: a case study of Yangtze River delta region. China Mining Magzine 2019;28:18-22. (in Chinese). Available from: https://kns.cnki.net/kcms2/article/abstract?v=yqeyU9EK6jQoNfNJSHBAnupB6VcfeqPx2FrI-hPfoJGZSLtQ8DMN2Ah1qsqSs59cHK_zSb6vYUgYzf_mAvOMQ7lmOTVxSVBrxL_qIXqQaWVwupJ2XWv8AAxdgESpdHLK_zAOYHjBrmuWWgwaEDpS8g==&uniplatform=NZKPT&language=CHS [Last accessed on 23 Jul 2024].
8. Li Y. Study on evolution of construction stock and its driving factors in Beijing. Beijing Uni Technol 2019. (in Chinese).
9. Liang H, Bian X, Dong L, Shen W, Chen SS, Wang Q. Mapping the evolution of building material stocks in three eastern coastal urban agglomerations of China. Resour Conserv Recy 2023;188:106651.
10. Zhang C, Hu M, Sprecher B, et al. Recycling potential in building energy renovation: a prospective study of the Dutch residential building stock up to 2050. J Clean Prod 2021;301:126835.
11. Liu Q, Liu L, Liu J, Li S, Bai H, Liu G. Estimation and driving force of steel stocks in Chongqing. Resour Sci 2018;40:15-24. (in Chinese). Available from: https://kns.cnki.net/kcms2/article/abstract?v=yqeyU9EK6jQ6fdkl6UER-tcT2unLTogEvvbwUGISgCocPFU_t39RV6lIhNLaay8NtwsD3umEQfeU4WidCY3ZtHDhOMk35rIRd0shtuBkMCEU0TB22zbenQZWXOcUQuyBT1pr8P9f0cb4tEZ-FZppuQ==&uniplatform=NZKPT&language=CHS [Last accessed on 23 Jul 2024].
12. Müller DB. Stock dynamics for forecasting material flows - case study for housing in the Netherlands. Ecol Econ 2006;59:142-56.
13. Long Y, Song Q, Huang B, Zeng X, Wu H. Characterizing temporal and spatial characteristics of urban building material metabolism and embodied carbon emissions through a 4D GIS-MFA-LCA model. Resour Conserv Recy 2024;206:107642.
14. Wang J, Wei J, Liu Z, Huang C, Du X. Life cycle assessment of building demolition waste based on building information modeling. Resour Conserv Recy 2022;178:106095.
15. Wiik MK, Fufa SM, Kristjansdottir T, Andresen I. Lessons learnt from embodied GHG emission calculations in zero emission buildings (ZEBs) from the Norwegian ZEB research centre. Energy Build 2018;165:25-34.
16. Bai J, Qu J, Maraseni TN, Wu J, Xu L, Fan Y. Spatial and temporal variations of embodied carbon emissions in China’s infrastructure. Sustainability 2019;11:749.
17. Shirazi A, Ashuri B. Embodied life cycle assessment (LCA) comparison of residential building retrofit measures in Atlanta. Build Environ 2020;171:106644.
18. Yan H, Shen Q, Fan LC, Wang Y, Zhang L. Greenhouse gas emissions in building construction: a case study of one Peking in Hong Kong. Build Environ 2010;45:949-55.
19. Wang X, Huang B, Wang Y, Liu J, Long Y, Daigo I. The impact of allocation methods on carbon benefits - a case study of construction waste recycling. Resour Conserv Recy 2023;199:107269.
20. Zhang N, Luo Z, Liu Y, Feng W, Zhou N, Yang L. Towards low-carbon cities through building-stock-level carbon emission analysis: a calculating and mapping method. Sustain Cities Soc 2022;78:103633.
21. Akbarnezhad A, Xiao J. Estimation and minimization of embodied carbon of buildings: a review. Buildings 2017;7:5.
22. Heilig GK. World urbanization prospects: the 2011 revision. Available from: https://www.un.org/en/development/desa/population/publications/pdf/urbanization/WUP2011_Report.pdf [Last accessed on 23 Jul 2024].
23. Hertwich E, Lifset R, Pauliuk S, et al. Resource efficiency and climate change. International Resource Panel (IRP) 2022. Available from: https://www.resourcepanel.org/sites/default/files/documents/document/media/resource_efficiency_and_climate_change_presentation_slides_main_report_implications_for_business_leaders.pdf [Last accessed on 25 Jul 2024].
24. Zhang YX, Luo HL, Wang C. Progress and trends of global carbon neutrality pledges. Adv Clim Chang Res 2021;17:88-97. (in Chinese).
25. Zhang Y. Situation and start-up thought of carbon peaking and carbon neutralization. Admin Reform 2021:77-85. (in Chinese).
26. Tian Y, Zuo S, Ju J, Dai S, Ren Y, Dou P. Local carbon emission zone construction in the highly urbanized regions: application of residential and transport CO2 emissions in Shanghai, China. Build Environ 2024;247:111007.
27. Shanghai Municipal Statistics Bureau. Shanghai statistical yearbook 1996-2022. Shanghai: China Statistical Press; 2022. (in Chinese). Available from: https://www.stats.gov.cn/sj/ndsj/ [Last accessed on 23 Jul 2024].
28. Riahi K, van Vuuren DP, Kriegler E, et al. The shared socioeconomic pathways and their energy, land use, and greenhouse gas emissions implications: an overview. Global Environ Chang 2017;42:153-68.
29. Zhong X, Hu M, Deetman S, et al. Global greenhouse gas emissions from residential and commercial building materials and mitigation strategies to 2060. Nat Commun 2021;12:6126.
30. Chen Y, Guo F, Wang J, et al. Provincial and gridded population projection for China under shared socioeconomic pathways from 2010 to 2100. Sci Data 2020;7:83.
31. Sarkar S, Chamberlain JF, Miller SA. A comparison of two methods to conduct material flow analysis on waste tires in a small island developing state. J Ind Ecol 2011;15:300-14.
32. Hashimoto S, Tanikawa H, Moriguchi Y. Where will large amounts of materials accumulated within the economy go? Waste Manag 2007;27:1725-38.
33. Liu M, Meng J, Liu B. Research progress on domestic and international carbon emission accounting methods. Trop Geogr 2014;34:248-58. (in Chinese).
34. Hao Q, Huang M, Bao G. Overview and comparative study of carbon emission accounting methods. Chin J Environ Manag ;2011:51-5. (in Chinese).
35. Riahi K, van Vuuren DP, Kriegler E, et al. The shared socioeconomic pathways and their energy, land use, and greenhouse gas emissions implications: an overview. Glob Environ Chang 2017;42:153-68.
36. Building construction manual. Beijing: China Building Industry Press; 2007. (in Chinese). Available from: https://thinker.cnki.net/bookstore/book/bookdetail?bookcode=9787801778963001&type=book [Last accessed on 23 Jul 2024].
37. National bureau of statistics of the People’s Republic of China, 1996-2022. (in Chinese). Available from: https://www.stats.gov.cn/sj/ndsj/ [Last accessed on 23 Jul 2024].
38. Ma L, Jiang Q, Zhao C. Research on the environmental coordination evaluation index system of green building materials based on LCA. China Build Mater Sci Technol 2010:90-5. (in Chinese). Available from: https://kns.cnki.net/kcms2/article/abstract?v=yqeyU9EK6jRYwjU3hiNKxnomYQveluYa_82ZN-t2nZWe-uVgIO2PueJeslDxsOSULHFFPp1NASXYEC1mXpkeGCqe1epldXpdDoBcJHhHWM5jMcAo1FlZyZnW99-BNyCc&uniplatform=NZKPT&language=CHS [Last accessed on 23 Jul 2024].
39. Standard for building carbon emission calculation GB/T 51366-2019S 2019. (in Chinese). Available from: https://baijiahao.baidu.com/s?id=1773260438801045556&wfr=spider&for=pc [Last accessed on 23 Jul 2024].
40. Xu X, Huang B, Liu L, et al. Modernizing cement manufacturing in China leads to substantial environmental gains. Commun Earth Environ 2022;3:276.
41. Watari T, Cao Z, Hata S, Nansai K. Efficient use of cement and concrete to reduce reliance on supply-side technologies for net-zero emissions. Nat Commun 2022;13:4158.
Cite This Article

How to Cite
Download Citation
Export Citation File:
Type of Import
Tips on Downloading Citation
Citation Manager File Format
Type of Import
Direct Import: When the Direct Import option is selected (the default state), a dialogue box will give you the option to Save or Open the downloaded citation data. Choosing Open will either launch your citation manager or give you a choice of applications with which to use the metadata. The Save option saves the file locally for later use.
Indirect Import: When the Indirect Import option is selected, the metadata is displayed and may be copied and pasted as needed.
About This Article
Special Issue
Copyright
Data & Comments
Data
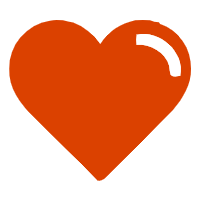
Comments
Comments must be written in English. Spam, offensive content, impersonation, and private information will not be permitted. If any comment is reported and identified as inappropriate content by OAE staff, the comment will be removed without notice. If you have any queries or need any help, please contact us at [email protected].