Defining a novel method to undertake sectoral risk management under climate change: a case study of intermodal transportation in the Peel Region, Ontario, Canada
Abstract
All levels of government must prepare for an increase in adverse weather events related to climate change. Developing resilient transportation infrastructure is critical to minimizing disruptions, economic loss, and human health impacts. A challenge for national and regional governments, however, is understanding how to prioritize investments given risk levels and limited resources. This study proposes a framework, using the Region of Peel, Canada as a case study to identify and prioritize key risks in a critical economic sector for the region: intermodal goods movement. The framework integrates projected changes in weather patterns, estimating the damage to infrastructure, interruption of economic activity, and adverse impacts on the workforce, accounting also for impacts on communities, for sound policy formulation. The framework will underpin a data collection plan to inform future policy and investment in strengthening adaptation and resilience to the most likely hazards affecting goods movement. The framework was designed with a view to being easily adapted to other sectors and regions.
Keywords
INTRODUCTION
Climate change is increasing the frequency and severity of adverse weather events, which have cascading impacts on a region’s economy. Regarding the region under consideration-Peel-studies using RCP (Representative Concentration Pathways) have yielded projections that demonstrate a likelihood of significant deterioration of operating parameters even under optimistic scenarios[1-3]. The RCPs represent potential future climate scenarios under varying emission levels and thereby enable an assessment of likely trends in weather factors[2]. Forecasts predict significantly higher temperatures and a likelihood of more intense rainstorm events during the summer. During winter, forecasts suggest an unpredictable pattern of snow events and large variations in temperature ranges[2,3]. This means that the intermodal transportation and storage system is particularly vulnerable to flooding, heatwaves, and the resulting erosion of land, degrading operational conditions and infrastructural capacity[3-7]. Identifying the business case supporting the significant financial commitment needed by cities and regions can justify the required investment to strengthen and enhance infrastructure to prepare for the consequences of climate change[7-9]. There is a distinction to be made between the need for a national approach to adaptation planning and approaches at local levels which may be more granular to ensure resilience of livelihoods[8-10].
A common critique of national economy-wide initiatives dealing with climate change impacts is that they are too expensive. However, recent studies have demonstrated that failing to invest in adaptation and resilience measures could lead to even greater economic costs, with projected losses of up to 5.18% of the world’s GDP (Gross Domestic Product) per capita by 2050 under “business as usual” approaches[10,11]. Furthermore, the Institute of Sustainable Finance estimates significant damage to Canada’s economy in the absence of adaptation-focused investment, with a projected loss of capital output of $2.773 trillion under a 2-degree Celsius warming scenario[12]. The Swiss Re Institute also identified an annual reduction of 6.9% in the Canadian economy by 2050 without impactful worldwide measures to deal with climate change[13]. In this context, the Region of Peel provides an ideal case study to delineate a process to assess the aggregate value at risk due to climate change-related events at a local level using the mechanism of measuring the impact on a critical economic system for the entire eastern Canadian economy[2,4,13,14]. This will require drawing on the changing projections of weather hazards and the consequent impact on component parts of the sector in terms of the personnel, property, and value affected[15,16]. Previously developed models for the U.S. Department of Transportation and urban planning state that the identification of value at risk can be drawn from first identifying IDF (Intensity-Duration-Frequency) curves of key weather events based on assessing the result of various RCPs and hence the likely variations in physical conditions. The emphasis in this framework has been on both the physical and the human aspects of operations that ensure timely movement of goods[1,6,15,17]. This points to the need to use or develop a data set that integrates the projected changes in weather, current design parameters of infrastructure, and census of employees[18,19].
The framework first identifies critical hazards that can have the most significant impact on the intermodal transportation system in the Region of Peel. This is essential since the adaptation plans for many regions could be economically unfeasible given the sheer number of potential hazards[16,17]. Next, the framework delineates the impact of these hazards by defining the value of physical infrastructure, workforce, and aggregate economic impact. This enables estimation and planning of the costs of business and regional economic activity that may arise from the most likely hazards affecting critical points in the system. The framework's primary goal is to develop a strategy for adaptation that enhances the entire system's resilience, reducing recovery times and long-term damage[6,15,20,21]. The key aim of this paper is to outline a conceptual grounding through a review of key literature that validates the proposed evaluation mechanism that enables guidelines for policy and investment decisions in strengthening adaptation and hazard resilience.
LITERATURE REVIEW
On February 27th, 2022, the second working group of the Intergovernmental Panel on Climate Change (IPCC) released a report highlighting key risks to economic and social infrastructure posed by climate change. The report conveys significantly increasing negative consequences on economic activity and livelihoods across the world, even in regions not naturally prone to hazardous weather conditions[2]. Transportation infrastructure will be particularly impacted, resulting in risks to economic activities based even on an assumption of a middle-of-the-road SSP (Shared Socioeconomic Pathway) scenario[2,22-24]. The SSPs provide an assessment of potential social and economic conditions resulting from varying climate change mitigation efforts[24]. Rapid degradation of operational capacity and frequent disruptions to goods movement are just a few of the adverse consequences of even moderate changes in climate change as emissions are controlled at current levels[16,18].
An overview of projected climate-related changes in Canada reveals increasing intensities of higher temperatures and precipitation compared to the 1950-2010 period, underscoring the need for socioeconomic vulnerability assessments[1,3,4,12]. Various commercial and public sectors are unprepared to deal with the impacts of climate change on the ability of the Canadian economy to cope and maintain high standards of living[24-26]. Climatic projections resulting from scientific models utilized by the IPCC and other government and non-governmental climate monitoring organizations, as well as commercial organizations such as insurance companies, have identified that climate-related adverse events are increasing in frequency and intensity, which has a consequent impact on the economy in two ways[27-29]. The first is that the frequency and intensity of adverse weather events directly affect commercial and residential activities in inhabited regions[3,23]. The second is the vulnerability of natural resources, especially those on which the viability of economies and livelihoods of a local populace depend, given the high likelihood of compound events, such as drought coupled with heatwaves[23,27,28]. These events result in rapid degradation and significantly slowed post-event recovery. Hence, it is critical to assess the area under study and develop a plan to look at the probability and likelihood of various risks to identify the most feasible modifications and the corresponding investment needed to implement measures to strengthen critical infrastructure components[29-31]. This process of modifying and strengthening key components of regional economies falls under the required adaptation planning that needs to be undertaken to ensure socioeconomic welfare and financial stability in climate change scenarios[2,12].
There is already considerable awareness of the increasing risks of flooding to domestic and commercial infrastructure resulting from increased precipitation, mainly due to the increasingly expensive insurance premiums paid by homeowners and commercial entities[20,32-34]. In addition to premiums, national and local budgets for infrastructure maintenance are expected to become inadequate under worsening climate change scenarios[26,34]. Roads are expected to be particularly vulnerable to the effects of climate change, with large increases in maintenance and rebuilding costs[27]. The impact on railways is an additional area of concern due to delays and expected damage to loading and guidance infrastructure especially those part of lines close to large water bodies[34-36]. Additional areas of concern relate to other increasingly intense hazards, such as prolonged heatwaves, which affect the viability of physical infrastructure and the well-being of employees[16].
One way to adapt infrastructure to counter the impact of climate change is to develop, for both the short-term and long-term, robustness (the ability to withstand shocks), redundancy (the ability to use alternate pieces of infrastructure), resourcefulness (the ability to deploy significant resources for alleviation of adverse impact), and rapidity (the ability to counter adverse impacts in a timely fashion)[37-39]. Therefore, infrastructure design must account for the possibility of failure and the consequences of that failure, pointing to the need for updated design parameters for handling capacity, weather stresses, and significant uncertainty[38,39].
Another way to identify the level of vulnerability to climate change impacts is to assess the extent to which operations related to the infrastructure will be affected and whether the system can adjust to novel or intensified stresses[18], meaning that the desired outcome is not the resilience of every infrastructure component, but that the system as a whole is resilient enough to withstand potential internal failures so as to prevent significant cascading consequences[34,40]. When a disruptive event occurs, a lack of redundancy and robustness in regional transportation systems, for example, could cause prolonged blockages of container movement, affecting supply chain activities across regions and sectors[18,19,39]. Transportation systems are susceptible to increasingly intense weather events and the greater prevalence of gradual degradation through increased erosion[6,41-43]. The most used road and rail paths are not the only key parts of an intermodal transportation system. There are also critical stationary assets that need to be assessed in terms of the ability to withstand significant weather events during operational activities[21,23,44].
Fichtinger et al. (2015) highlighted that the level of resiliency within complex transportation networks is important to monitor to mitigate risks[45]. Previous approaches have looked at key physical infrastructure components vulnerable to unforeseen changes in climactic range, which impact their operational viability[22]. The framework proposed in this article identifies issues in a value chain that could lead to economic risks[46], integrating findings related to the occurrence and consequences of climate change on transportation systems. The primary outcome highlights the need to understand the current condition of infrastructure and the consequences of expected changes in its capacity to handle adverse conditions[44].
METHODOLOGY
To identify the impact of climate change on the movement of goods and supply chains, and conduct climate hazard assessment, three key steps are followed. The first is developing an inventory of the intermodal transportation system entities in the region. Then, it is important to identify risk estimates based on the likelihood of various climate hazards. The third step is to estimate the potential economic consequences of the subsequent climate hazard risks[33,44]. Steps two and three follow other government-funded studies in the United States that sought to develop a comprehensive assessment process of the likely risks and mitigation efforts needed to safeguard key transportation assets[7,16]. An initial draft framework was presented and vetted at a workshop involving key stakeholders in the Region of Peel, who provided invaluable feedback in developing the details of the individual assessment steps and knowledge gaps that need to be filled for its implementation. The threat to the movement of goods and services in Peel Region due to climate change depends on the degree to which normal expected weather patterns are changing, resulting in more frequent and intense flooding and storms[1,3]; higher annual temperatures characterized by more frequent and longer heatwaves[4,45]; and increasing erosion[23,31,46].
Case study in the Region of Peel
The Region of Peel is located at a key intersection point in the Greater Toronto Area and the Great Lakes Region [Figure 1]. Peel’s goods movement sector comprises a network of roads and rail as part of the transportation pathways along with many storage and transfer sites; it facilitates the movement of $1.8 billion in goods[5] every day and contributes almost $50 billion to the region’s economy, accounting for about 40%[5]. The region is critical to normal operations and the distribution of goods, services, and people not only in Canada but internationally, given it is also host to Canada’s largest airport and the CN (Canadian National Railway) Brampton Intermodal Terminal which handles 60% of CN’s intermodal traffic. The logistics of the cross-border supply chain that affects so much economic activity in the Northeast and Midwestern United States as well as Eastern Canada runs through the region[14,32]. Previous assessments of vulnerability to the impacts of climate change in Canada’s road and rail system combined with the volume of goods movement in this region necessitate the development of a framework for prioritizing and investing in resilient infrastructure beyond current design parameters[27,35,47]. Much of the current planning for disasters and adverse events affecting citywide and regional transportation systems relies on parameters derived from historical data rather than on predictive RCPs/SSPs. This planning also largely disregards the varied consequences of climate change on the economy and social welfare[2,21,29,35,48]. The results of previous assessments of the vulnerability of Canada's road and rail system to the impacts of climate change and the volume of goods movement in this region therefore necessitate the development of a framework for prioritizing and investing in resilient infrastructure beyond current design parameters[27,35,47].
Projection of changes in climate in the Peel Region
Average annual temperatures in the Region of Peel are expected to increase by 2 °C by the 2050s and
Another area of concern is the increasing prevalence of “compound extreme events”, which refers to occurrences of multiple hazards in force at the same time, such as drought and heatwaves, storms, and flooding. Compound extreme events severely complicate the ability of local and national governments to undertake emergency measures[16,27,49]. Zscheischler et al. highlight the importance of developing frameworks for risk assessment due to more frequent extreme weather patterns and that the assessment process must integrate greater margins for projected factors and thus the consequent measurable cumulative events, such as flooding, water deficiencies, and erosion[30,50]. Systems thinking is an impactful approach to designing frameworks to address the multi-faceted and far-reaching consequences of extreme compound events[7,22].
There can now be a risk prioritization process based on an outlined layout of the region, its economic sectors or the sector in question, and weather patterns. The intermodal transportation system depends on timely movement through specific paths to key installations in the region. This means that there are expected vulnerabilities at key choke points within the system: Pearson airport, CN Brampton Yard, and corporate container storage points[3,5,52]. The specific paths to these key locations are also of key interest. The likely physical damage can be expected to come from rapid destruction related to precipitation and slower, but equally significant, consequences related to erosion (scouring, soil displacement, weakening of built components) leading to movement stoppage and capacity impairment[5,6,16,48,50]. The ranking of the risks to be considered will be identified based on the potential economic damage and, hence, the reinforcement investment needed. This is where the framework is utilized to outline key data requirements.
RISK ASSESSMENT FRAMEWORK
The framework presented in Figure 2 represents the key processes required for an economic vulnerability assessment of the intermodal transportation system. The framework identifies the most likely hazards and their consequent impacts on the most-used modes of transportation with enough flexibility built in to develop in-depth consideration of other modes where needed[44]. Considering the importance of the region as a critical conduit for goods, the framework identifies risks from three perspectives:
- Infrastructure: the vulnerability of pathways and installations to damage;
- People: injury and mortality in the labor force;
- Economy: impact on supply chain and goods movement economic activities.
The data collection process will thus have to be multi-faceted to develop a comprehensive picture of the value-at-risk for the sector in the region under consideration. The next sections will outline the key sources in segments that will be part of the data collection process. This will be outlined in stages as they will be brought together to summarize the vulnerabilities to prioritize investment decisions. The stages are as follows:
The first stage is climate Hazard Risk Identification: directed towards sources that identify current and projected weather patterns. This includes assessing the various hazards likely to affect the system under consideration. While climate change is expected to create changes in the way various hazards interact with components of economic sectors, the consequences are different for various stakeholders. This also means that the corresponding reinforcement and allowances for recovery and resilience will need to change based on the results of this and consequent steps. A process is outlined to generate a vulnerability assessment with an emphasis on identifying points of concern in built infrastructure and pathways. This should include assessing the physical conditions as well as welfare of the people who are part of the system. It should be noted that a significant amount of the data is already collated by regional and federal government agencies and trade associations[4,52,53]. The data garnered will be used to develop a combined risk map providing measurements for the frequency and severity of likely events[46]. This will mirror supply chain vulnerability assessments. The resulting decisions resulting from this process concern the identification of points of weakness that result in the most economic damage and, accordingly, the needed reinforcements.
Application of framework - processes and key outcomes
The application of the framework uses Peel Region to show how to define a recurring risk assessment process. There is enough commonality throughout transportation systems around the world that the framework can be used in other regions without significant modification. Regarding other economic sectors, some aspects can be modified to account for variations in processes and priorities in deliverables. It should be noted that studies examining the impact of climate change on economic sectors in general involve identifying what is “normal” in operating conditions and how much the respective sector is vulnerable to variations in temperature, precipitation, and other physical aspects of weather patterns[15,17,28]. Thus, this framework is adaptable to developing a risk map for disparate economic sectors with modifications as necessary. The first key step is assessing and defining what hazards should be of concern based on the location, function, and reach of the system under study. This then enables defining consequences for key players in the affected sectors. The outcome at the end of this process for policymakers, government officials, industry actors, and local communities is to be able to assess and define the points of key vulnerabilities and monitoring. The data collection process will require institutional involvement across the board, from climate monitoring departments to local industry, government, and community groups, to garner economic data.
Stage 1: climate hazard risk identification
Chang et al. (2021) developed a framework to implement transportation asset management based on the most likely hazards that will impact specific points in the goods movement system, which supports budget prioritization and allocations to reinforce these vulnerable nodes to prevent cascading impacts on the rest of the transportation system[44]. Other government-funded studies emphasize that it is not likely that all climate change-related weather hazards will affect all points in the system equally, which therefore means that a targeted approach is needed to develop resilience in transportation systems[16,17,33]. The general outline of the proposed steps in the hazard prioritization process is outlined in Figure 3.
The critical step after identifying specific hazards is to identify their likelihood of occurrence. The IPCC has identified that various levels of greenhouse gas emissions are likely to create specific outcomes for specific hazards depending on the geographic site and situation of the area[17,23,28]. This means Peel Region can expect the likelihood of each hazard to vary compared to selected baseline averages, for example, the 1981-2010 baseline in a 2016 study commissioned to examine the consequences of climate change[4]. The next step is to identify the severity of impacts based on three factors:
- Duration: This is the number of hours or days that each hazard is expected to prevail. Studies show that a change in the expected frequency, especially related to flooding from intense storms affecting key transportation system components (road, rail, and installations), will impact spatial reach and damage potential[1,53,54].
- Spatial reach: This is defined by the geographic extent of the projected damage. The aim is to identify the level of disruption expected during each projected event. This should be limited to the extent of disruption to operational activity and hence direct damage. Some supply chain impact could be included but the scope needs to be defined to include immediate damage[27,44,55].
- Damage potential: The damage potential to the facility or pathway should be quantified in terms of repair and replacement cost per projected incident. This should also include, if applicable, the cost of redesigning a facility that is able to withstand a higher level of hazard occurrence. The economic damage caused by disruption could be a difficult metric to ascertain with high accuracy, but estimates can be made based on surveying companies and cargoes[7,29,31,33].
These measures provide an aggregate potential impact on the intermodal transportation system which enables the planners to prioritize hazards. The IPCC in 2012 and then in 2021 highlighted the increased prevalence of what it defined as compound extreme events, which complicate planning and preparation for the transportation systems. Hence assigning costs and budget allocations for disaster management becomes a difficult exercise[16,27,28,30]. This makes the outcome of adverse events even more uncertain and affects the specifications of how to design for an economic scenario in which significant climate changes have occurred.
Stage 2: vulnerability assessment to define impacts
Previous studies undertaken on goods transportation systems have identified specific points of vulnerability that need to be related to the identified priority hazards. The British Columbia Ministry of Transportation and Infrastructure has identified the need for preparedness at the provincial level to protect critical local infrastructure which supports several sectors and towns due to the critical flow of goods[56]. Recently published reports have identified the interdependencies of the system in which each sub-section is connected, which should be kept in mind as the assessment is developed[26]. Each component identified as part of the vulnerability assessment section of the framework will have varying degrees of impact because of the prevalence of each hazard. This is a feature of non-linear systems and developing remedial actions will require identifying critical points for reinforcement or developing services that maintain the required handling capacity[6,29,57,58]. Below are the grouping and key points to identify under each vulnerability assessment.
Infrastructure vulnerability
This is the most critical part of the framework as failures in any essential node of the physical infrastructure will result in cascading impacts across the system. The following points have been highlighted as the primary metrics to assess.
Condition and carrying capacity
The assessment of the intermodal infrastructure system must be carried out on both the stationary facilities and pathways. The proposed mechanisms are based on previous research and consequent recommendations by the United States Department of Transportation to prepare infrastructure for the effects of climate change[7,22].
Assessing road and rail pathways
The process of assessing road and rail pathways starts with comprehensively mapping the flow of goods and services; such maps are partially available from local databases and can be complemented by additional federal and provincial government data[1]. Rail mapping should be easier due to the specified paths taken, whereas road mapping would require the identification of high-density paths for cargo movement[36,59]. The next step is to overlay key pathways with projected flood, temperature, and erosion mapping in terms of intensity and duration developed by government and international agencies with data that can then be projected to identify the consequences of climate change[51].
Assessing stationary facilities
The process of assessing stationary facilities draws on previous frameworks to analyze and update the status of key infrastructure with respect to expected climatic changes[7,52]. Stationary points include key facilities such as the CN Brampton Intermodal Terminal, which makes transportation of goods through the region economically efficient but also increases the risk should there be an adverse event, due to a lack of redundancy[4-6]. The facilities include not just government-owned and maintained facilities but also many privately owned facilities such as warehouses that are critical to the intermodal transportation infrastructure[5,60]. Another key stationary facility is Pearson Airport, encompassing its runways, roads, and buildings that house passengers, employees, vehicles, aircraft, and equipment[1,52]. The steps to be undertaken to assess the condition and requirements of stationary facilities are outlined in Figure 4.
This section draws on studies identifying the most at-risk points in an intermodal transportation system, which integrate the volume of goods transferred at risk and the consequent impacts on other components of the system. The importance of the Peel Region as a critical node for the flow of goods through the Eastern Canadian economy is well established[5,14,61]. An analysis requires identifying the sub-components of the system responsible for the highest volume of goods and services, disruptions to which would be deleterious for consumers and businesses[4,27,31]. The interdependencies between infrastructure, people, and the economy are reflected in the importance of these nodes. Any disruption has the potential for significant downstream economic impact.
This and the following sections identify the primary use of the framework outlined at the beginning of the methodology section. The cost of adapting cities and other built locations to the consequences of climate change is getting increasingly expensive as the world slips into complacence or unwillingness to take drastic steps[23,26,33]. The prioritization of investment into a more resilient economy can take place when the most vulnerable points are identified and the most likely value of cascading failure estimated[37,44]. This is a key goal of this framework through the process outlined here. This identification of the most consequential vulnerable points can allow governments and private organizations to come together to develop modus operandi and robust physical infrastructure for minimal disruption of regional and national economies[1,23,31].
Infrastructure impact points
As outlined previously, the framework will enable the identification of key impact points relating to the corresponding component at this stage of the assessment. For infrastructure, the key outcomes of the vulnerability assessment must be the following.
Damages and repair costs
As outlined in studies of the vulnerability of infrastructure to climate change, one key driver of such costs is that of repair, whether continuous or as needed. Without planning for the increased capacity needed, the cost of repair and replacement will be substantial. Ness et al. for Canada estimate a cost of CAD (in Canadian Dollars) $3.4 billion by mid-century[27].
Reduced service life
Connected to the damages and repair costs is the estimation of the reduction in service life due to increased pressures on facilities and pathways. Much planning is undertaken at a timescale that does not integrate the impacts of climate change[26,27]. It is also critical to understand how the consequences of climate change will reduce the life of many current components of the intermodal transportation infrastructure, whether due to erosion or heat that degrades structural integrity[1,26].
Travel disruptions
Travel disruptions are expected to be the most difficult to measure, considering the challenges of identifying exactly how many people would be affected by failure points in a system with consequent impacts on the movement of traffic. This also applies to the inability to undertake or complete scheduled work that is halted due to weather events as the climate becomes more unpredictable[7,27].
Labor force impact points
Goods movement in the Region of Peel accounts for four out of every nine jobs and CAD $29 billion in labor income, according to previous studies carried out by the Region of Peel[5]. This means that the transportation of goods and services is critical to the economic welfare of the region and any disruptions will impact livelihoods[1,5,32]. The welfare of employees who face loss of income due to adverse events has been an issue made clearer by the COVID-19 crisis, whereby shut-downs have been undertaken out of necessity. This can also be expected in the intermodal transportation sector, where a significant proportion of the employees are expected to hold precarious jobs, rendering them economically and physically vulnerable to the consequences of adverse events affecting operations[60,62]. The labor force of the intermodal system can be divided into three categories: transportation workers, facility (private and public) workers, and maintenance crews. A process for understanding the vulnerabilities of each group and their interconnected functions requires a data collection process and classification such as that outlined in Table 1.
Labor vulnerability and classification
Data | Purpose for collection |
Transportation workers - rail (non-maintenance) | Identify workforce involved in direct transportation of goods |
Transportation workers - rail (maintenance and emergency crews) | Identify at-risk workforce in case of adverse events |
Truck operators | Identify road intermodal transportation-focused workforce |
Goods handling facility (CN Brampton Yard, Pearson) | Identify workforce related to various publicly run road/rail paths and facilities |
Warehouse staff and frontline workers | Identify workforce in warehousing - directly involved in logistics |
These data can be collated from regional and national government and industry agencies that collect and use these data for planning projects and income taxation. These data points describe the demographic composition and access to services needed to tackle immediate needs and the long-term impacts of climate change. This could range from health impacts during heatwaves to injuries on the job dealing with sudden storms[6,21,49]. It is important to identify the likelihood of loss of life and limb to develop a planning mechanism for the welfare of the affected employees and dependents. This needs to be extended to not just the personnel working directly in the intermodal transportation system but also the various privately owned facilities for storage and transfer[5,6].
Economic impact points
The economy of the region and the importance of the intermodal transportation system draw from the flow of goods and services from the United States to and from the eastern port of Nova Scotia, Canada and through multiple intermediary points[14]. The CN Brampton Intermodal Terminal handles 60% of intermodal traffic[5]. The importance of the region to the economic welfare of surrounding towns and cities, and hence consequences of any disruption, is well established[61-64]. This means that disruptions to goods movement will affect sectors that rely on the timely delivery of commodities. A proposed mechanism to assess the economic vulnerability is presented in Table 2. The data from various sources such as national transportation planning, industry reports, and surveys of local entities can provide the necessary components for the assessment.
Economic vulnerability assessment
Step # | Activity to be undertaken |
1 | Layout of rail and road maps for high-density cargo transfer |
2 | Identify key goods transfer points in the region |
3 | Identify critical warehouses in region |
4 | Classify commodities transferred as for end use or for storage for later transfer |
5 | Integrate flood maps and assess changes in flood prevalence under varying climate change scenarios |
6 | Assess frequency and severity of high-temperature days |
7 | Identify preparedness for consistent high temperatures - commodities & workforce |
8 | Map potential risks in terms of loss of goods and increased costs of maintenance |
9 | Estimate potential delays due to adverse events by the value of goods affected |
Presentation of framework application to stakeholders
A seminar was undertaken as part of the framework development process to evaluate the viability of the conceptual foundations of the framework, the availability of key data needed, and community buy-in. Critical personnel who would be instrumental in implementing the data collection plan, setting up the results, and identifying urgent follow-up steps were part of the seminar. The first point raised was the need to develop an updated flood map that took into consideration both significantly changed projected weather patterns and the increasing levels of development[1]. Another important point that was raised was the issue of overlapping jurisdictions and hence decision-making authority which is also stated later as an avenue to develop further research[5,14]. Another intriguing insight provided from the seminar was the need for a coordinated assessment with transportation companies and logistics hubs as to key priorities, which ensures that investment is not duplicated and, in some cases, enhances the efficiency of resulting reinforcing mechanisms based on the priorities of the various stakeholders[32,44].
Limitations
There were some limitations to the development of this framework. One limitation included a lack of a comprehensive source of data, which presented challenges to preliminary quantification of some elements or the identification of certain qualitative outcomes. In addition, there was no department with direct responsibility for coordination, given the governance structure of the region, which could have facilitated data access as well as the issue of communicating the urgency of the situation to key decision makers. A further limitation to this framework may be the high-level perspective it takes; there may be more granular considerations, i.e., for individual businesses or neighborhoods. However, the framework provides a basis of interpretation for these and give both decision makers and individual businesses a model for risk identification.
CONCLUSIONS
The consequences of climate change necessitate developing a systemic risk assessment plan. The development of the framework considered both the physical consequences on individual components and the consequent impact on various economic sectors beyond the transportation system being examined. This requires comprehensive data collection and an information framework with clear outcomes to be able to identify weak points and long-term effects. The first phase of the framework, the hazard prioritization process, draws on the standard intensity-duration-frequency mechanism to identify, list, and prioritize hazards by potential monetary impact. The next phase of the framework addresses the key impact points where the most likely hazards will impact operations in the stationary (loading, storage, routing facilities) and pathway components (road and rail) of the intermodal transportation system. This leads to an estimation of at-risk value identified at key points in the sector[42,44], allowing for the identification of the investments needed to reinforce current and future infrastructure. Another important outcome of the framework is an estimation of the economic consequences of disruptions to movement in the intermodal transportation system. These include the value of goods at risk, especially perishables that need to be delivered within a specified time to prevent wastage[64]. Following this, estimations may be made regarding income loss for businesses and workers connected to the goods transportation network. The latter will include the health consequences for workers at various points in the system, which may stem from injuries or the long-term effects of weather changes[49].
The information-gathering process and the resulting decision making as to the prioritization of investments will require multi-level stakeholder involvement. This means that only government led measures, even if well-coordinated, will not be successful in disaster risk management under revised projections related to climate change[20,65]. It will also need the expanded involvement of the private sector in informing the awareness and response programs to sudden weather events and gradual degradation of key assets. The private sector involvement will range from logistical companies and health organizations to financial institutions, including banks and insurers[39,66,67]. It is important to recognize the concerns regarding the workforce capacity and investment needed to develop responsive mechanisms to deal with economic consequences of climate change. The key outcome is the development of a multi-pronged approach to risk management that ties in with national and regional adaptation planning[67]. The plans will tie into government policies and commercial investment for economic growth, resilience planning, and risk management.
Potential research and policy implications
There are certain avenues that can be further examined for modification of the framework and the potential extension of the conclusions from the study. The research does not directly examine the potential jurisdictional conflicts that arise from the need to lead and coordinate critical remedial actions. As mentioned partially in the limitations, the region and the sector under consideration fall under a patchwork of government entities responsible for various aspects of sector operations and for emergency response[1,4,13]. Any cascading failure will impact the economy of the entire eastern Canadian region, and thus, it is in the vested interest of all agencies, governments, and companies to develop a comprehensive plan to monitor and respond to any adverse event[26,33]. The availability and planning of a support structure for the workforce in the sector can be developed to compensate for vulnerabilities to economic loss and physical harm[6,15]. For example, research in other sectors has identified the vulnerability of lower-income residents[25,49]. The infrastructure ready to support personnel from this sector and others in the geographic vicinity should be further examined.
Regarding future policymaking, the framework points to certain key measures that can be taken by the respective jurisdictional authorities by themselves and in collaboration with private sector players. As outlined, a comprehensive assessment will enable the identification of specific hazards that may affect the sector. This, in turn, will empower specialist teams to develop infrastructure standards that take into consideration higher physical stress parameters and longer timelines[6,42]. Other policy measures include developing a flexible and larger budget for undertaking emergency repair and long-term renovation of vulnerable and critical infrastructure points. This can be coupled with the development of local or alternative supply chain sources, reducing the need to import critical goods from across distances[13,55].
DECLARATIONS
Acknowledgments
The authors acknowledge the help of Mark Pajot, Jeremy Schembri, Meaghan Eastwood,and Sharon Lam, TRCA (Toronto and Region Conservation Authority), Peel Region Administration, and key stakeholders in developing and validating the components of the proposed framework.
Authors’ contributions
First author, contributed by developing the core of the literature review and structuring the key components of the proposed model: Rahim MA
Co-author, contributed by identifying further the conceptualization of the model, managing partners/stakeholders for the project, and providing writing reviews: Wilson J
Co-author, contributed by refining the model outline/components, developing literature review further, and editing/proofreading throughout the article: Johnson R
Availability of data and materials
Not applicable.
Financial support and sponsorship
The framework was part of a funded collaboration with TRCA providing financing of research and study into the economic implications of climate change on a key economic sector with consequent impact on residents of the region. A stakeholder feedback session enabled the refining of key aspects and the identification of critical foundations for the future implementation of risk assessment and management. This session validated key assumptions and identified potential shortfalls.
Conflicts of interest
All authors declared that there are no conflicts of interest. Jeffrey W is an Editorial Board member of the journal Carbon Footprints.
Ethical approval and consent to participate
Not applicable.
Consent for publication
Not applicable.
Copyright
© The Author(s) 2024.
REFERENCES
1. Woudsma C, Towns W. Chapter 6: Ontario. In: Palko K, Lemmen DS, editors. Climate risks and adaptation practices for the Canadian transportation sector. Ottawa, ON: Government of Canada; 2016. pp. 139-79. Available from: https://natural-resources.canada.ca/sites/www.nrcan.gc.ca/files/earthsciences/pdf/assess/2016/Chapter-6e.pdf [Last accessed on 26 Mar 2024].
2. IPCC. Summary for policymakers. Climate change 2022: impacts, adaptation and vulnerability. IPCC; 2022. Available from: https://www.ipcc.ch/report/ar6/wg2/chapter/summary-for-policymakers/ [Last accessed on 26 Mar 2024].
3. Bush E, Lemmen DS. Canada’s changing climate report. Ottawa, ON: Government of Canada; 2019. Available from: https://changingclimate.ca/site/assets/uploads/sites/2/2020/06/CCCR_FULLREPORT-EN-FINAL.pdf [Last accessed on 26 Mar 2024].
4. Auld H, Switzman H, Comer N, Eng S, Hazen S, Milner G. Climate trends and future projections in the Region of Peel. Region of Peel; 2016. Available from: https://climateconnections.ca/app/uploads/2017/07/Climate-Trends-and-Future-Projections-in-the-Region-of-Peel.pdf [Last accessed on 26 Mar 2024].
5. Region of Peel. Peel Region goods movement strategic plan 2017-2021. 2017. Available from: https://www.peelregion.ca/transportation/goods-movement/_media/pdf/goods-movement-strategic-plan-2017-2021.pdf [Last accessed on 26 Mar 2024].
6. Region of Peel. Status of regional infrastructure assets. Available from: https://www.peelregion.ca/strategicplan/20-year-outcomes/status-of-regional-infrastructure-assets.asp [Last accessed on 28 Mar 2024].
7. Baesch J, Batac T, Bottomley G, et al. Impacts of climate change and variability on transportation systems and infrastructure: the gulf coast study, phase 2 task 3.2: engineering assessments of climate change impacts and adaptation measures. The USDOT Center for Climate Change and Environmental Forecasting; 2014. Available from: https://trid.trb.org/view/1328423 [Last accessed on 26 Mar 2024].
8. Borie M, Ziervogel G, Taylor FE, Millington JD, Sitas R, Pelling M. Mapping (for) resilience across city scales: an opportunity to open-up conversations for more inclusive resilience policy? Environ Sci Policy 2019;99:1-9.
9. Smith R. Canada’s economy won’t prosper without climate change investments. Canadian institute for climate choices - blog. Canadian institute for climate choices 2021. Available from: https://climatechoices.ca/canadas-economy-wont-prosper-without-climate-change-investments/ [Last accessed on 26 Mar 2024].
10. Kahn ME, Mohaddes K, Ng RN, Pesaran MH, Raissi M, Yang J. Long-term macroeconomic effects of climate change: a cross-country analysis. Energy Econ 2021;104:105624.
12. Willcott N, Cleary S. The physical costs of climate change: a Canadian perspective. Institute for sustainable finance. 2022. Available from: https://smith.queensu.ca/centres/isf/pdfs/ISF-Report-PhysicalCostsOfClimateChange.pdf [Last accessed on 26 Mar 2024].
13. Oxfam Canada. Canada’s economy could shrink by 6.9 per cent per year by 2050 without more ambitious climate action - Oxfam. Oxfam Canada. 2021. Available from: https://www.oxfam.ca/news/canadas-economy-could-shrink-by-6-9-per-cent-per-year-by-2050-without-more-ambitious-climate-action-oxfam/ [Last accessed on 26 Mar 2024].
14. Anderson WP, Maoh HF, Gingerich K. Cross-border freight movements in the Great Lakes and St. Lawrence Region, with insights from passive GPS data. Can Geogr 2019;63:69-83.
15. Joakim EP, Mortsch L, Oulahen G. Using vulnerability and resilience concepts to advance climate change adaptation. Environ Hazards 2015;14:137-55.
16. Meyer M, Flood M, Keller J, editors. Strategic issues facing transportation, volume 2: climate change, extreme weather events, and the highway system: practitioner’s guide and research report. Washington, DC: The National Academies Press; 2014.
17. IPCC. Managing the risks of extreme events and disasters to advance climate change adaptation: special report of the intergovernmental panel on climate change. Cambridge, UK: IPCC; 2012.
18. Rowan E, Snow C, Choate A, et al. Indicator approach for assessing climate change vulnerability in transportation infrastructure. Transp Res Rec 2014;2459:18-28.
19. Morlok EK, Chang DJ. Measuring capacity flexibility of a transportation system. Transport Res A Pol 2004;38:405-20.
20. Starita S, Scaparra MP, O’hanley JR. A dynamic model for road protection against flooding. J Oper Res Soc 2017;68:74-88.
21. Wallace B. A framework for adapting to climate change risk in coastal cities. Environ Hazards 2017;16:149-64.
22. Meyer MD, Weigel B. Climate change and transportation engineering: preparing for a sustainable future. J Transp Eng 2011;137:393-403.
23. Hawchar L, Naughton O, Nolan P, Stewart MG, Ryan PC. A GIS-based framework for high-level climate change risk assessment of critical infrastructure. Clim Risk Manag 2020;29:100235.
24. Riahi K, van Vuuren DP, Kriegler E, et al. The shared socioeconomic pathways and their energy, land use, and greenhouse gas emissions implications: an overview. Global Environ Chang 2017;42:153-68.
25. Schnitter R. Health of Canadians in a changing climate: advancing our knowledge for action/edited by Peter Berry, Rebekka Schnitter. Ottawa, ON: Government of Canada; 2022. Available from: https://publications.gc.ca/site/eng/9.906520/publication.html [Last accessed on 26 Mar 2024].
26. Lawrence J, Blackett P, Cradock-henry NA. Cascading climate change impacts and implications. Clim Risk Manag 2020;29:100234.
27. Ness R, Clark DG, Bourque J, Coffman D, Beugin D. Under water: the costs of climate change for Canada’s infrastructure. Ottawa, ON: Canadian Institute for Climate Choices; 2021. Available from: https://climatechoices.ca/wp-content/uploads/2021/09/Infrastructure-English-FINAL-Sep29.pdf [Last accessed on 26 Mar 2024].
28. IPCC. Summary for policymakers. In: Climate change 2021: the physical science basis. Contribution of working group I to the sixth assessment report of the intergovernmental panel on climate change. Cambridge University Press; 2021. Available from: https://www.ipcc.ch/report/ar6/wg1/ [Last accessed on 26 Mar 2024].
29. Botzen WJW, Van Den Bergh JCJM. Managing natural disaster risks in a changing climate. Environ Hazards 2009;8:209-25.
30. Zscheischler J, Westra S, van den Hurk BJJM, et al. Future climate risk from compound events. Nature Clim Change 2018;8:469-77.
31. Beheshtian A, Geddes RR, Donaghy KP. Modeling the impacts of climatic extremes on interregional freight-transportation system. Transp Res Rec 2018;2672:33-43.
32. CPCS. GTHA strategic goods movement network final report. 2018. Available from: https://assets.metrolinx.com/image/upload/v1663240133/Documents/Metrolinx/Strategic_Goods_Movement_Network_2018.pdf [Last accessed on 26 Mar 2024].
33. Molarius R, Könönen V, Leviäkangas P, et al. The extreme weather risk indicators (EWRI) for the European transport system. Nat Hazards 2014;72:189-210.
34. Kim Y, Eisenberg DA, Bondank EN, Chester MV, Mascaro G, Underwood BS. Fail-safe and safe-to-fail adaptation: decision-making for urban flooding under climate change. Clim Change 2017;145:397-412.
35. Feltmate DB, Moudrak M. Climate change and the preparedness of 16 major Canadian cities to limit flood risk. Waterloo, ON: Intact Centre on Climate Adaptation, University of Waterloo; 2021. Available from: https://www.intactcentreclimateadaptation.ca/wp-content/uploads/2021/02/16-Cities-Flood-Preparedness.pdf [Last accessed on 26 Mar 2024].
36. Chinowsky P, Helman J, Gulati S, Neumann J, Martinich J. Impacts of climate change on operation of the US rail network. Transport Policy 2019;75:183-91.
37. Delgado D, Aktas CB. Resilience of rail infrastructure in the U.S. Northeast Corridor. Procedia Eng 2016;145:356-63.
38. Kumar N, Poonia V, Gupta B, Goyal MK. A novel framework for risk assessment and resilience of critical infrastructure towards climate change. Technol Forecast Soc Change 2021;165:120532.
39. Mcdaniels T, Chang S, Cole D, Mikawoz J, Longstaff H. Fostering resilience to extreme events within infrastructure systems: characterizing decision contexts for mitigation and adaptation. Global Environ Chang 2008;18:310-8.
40. Mahmoud H. Barriers to gauging built environment climate vulnerability. Nat Clim Chang 2020;10:482-5.
41. Hall JW, Harvey H, Manning LJ. Adaptation thresholds and pathways for tidal flood risk management in London. Clim Risk Manag 2019;24:42-58.
42. Stone B Jr, Mallen E, Rajput M, et al. Compound climate and infrastructure events: how electrical grid failure alters heat wave risk. Environ Sci Technol 2021;55:6957-64.
43. Camp J, Abkowitz M, Hornberger G, Benneyworth L, Banks JC. Climate change and freight-transportation infrastructure: current challenges for adaptation. J Infrastruct Syst 2013;19:363-70.
44. Chang CM, Ortega O, Weidner J. Integrating the risk of climate change into transportation asset management to support bridge network-level decision-making. J Infrastruct Syst 2021;27:04020044.
45. Fichtinger J, Ries JM, Grosse EH, Baker P. Assessing the environmental impact of integrated inventory and warehouse management. Int J Prod Econ 2015;170:717-29.
46. Giannakis M, Papadopoulos T. Supply chain sustainability: a risk management approach. Int J Prod Econ 2016;171:455-70.
47. Climatedata.ca. Brampton, ON. ClimatedataCa 2021. Available from: https://climatedata.ca/explore/location/ [Last accessed on 26 Mar 2024].
48. Kwiatkowski KP, Stipanovic Oslakovic I, Ter Maat H, Hartmann A, Chinowsky P, Dewulf GPMR. Modeling cost impacts and adaptation of freeze-thaw climate change on a porous asphalt road network. J Infrastruct Syst 2020;26:04020022.
49. Paterson JA, Ford JD, Ford LB, et al. Adaptation to climate change in the Ontario public health sector. BMC Public Health 2012;12:452.
50. Byun K, Chiu CM, Hamlet AF. Effects of 21st century climate change on seasonal flow regimes and hydrologic extremes over the Midwest and Great Lakes region of the US. Sci Total Environ 2019;650:1261-77.
51. Rincón D, Khan U, Armenakis C. Flood risk mapping using GIS and multi-criteria analysis: a greater toronto area case study. Geosciences 2018;8:275.
52. PIEVC. Climate change vulnerability assessment for selected stormwater infrastructure at Toronto pearson international airport. Toronto pearson infrastructure climate vulnerability assessment. 2014. Available from: https://pievc.ca/wp-content/uploads/2021/01/gtaa_infrastructure_vulnerability_assessment_-_final-1.pdf [Last accessed on 26 Mar 2024].
53. Climate trends and projections 2018. Available from: https://www.canada.ca/en/environment-climate-change/services/climate-change/canadian-centre-climate-services/basics/trends-projections.html [Last accessed on 26 Mar 2024].
54. Evans B, Chen AS, Djordjević S, Webber J, Gómez AG, Stevens J. Investigating the effects of pluvial flooding and climate change on traffic flows in barcelona and bristol. Sustainability 2020;12:2330.
55. Becker A, Ng AK, Mcevoy D, Mullett J. Implications of climate change for shipping: ports and supply chains. WIREs Clim Change 2018;9:e508.
56.
57. Garschagen M, Doshi D, Moure M, James H, Shekhar H. The consideration of future risk trends in national adaptation planning: conceptual gaps and empirical lessons. Clim Risk Manag 2021;34:100357.
59. Jaroszweski D, Chapman L, Petts J. Climate change and road freight safety: a multidisciplinary exploration. Clim Change 2013;120:785-99.
60. Singh G. Logistics sprawl: spatial patterns and characteristics of new warehousing establishments in the Greater Toronto and Hamilton Area. M.A.S. University of Toronto (Canada). 2018. Available from: https://tspace.library.utoronto.ca/bitstream/1807/89515/1/Singh_Gagandeep_201806_MAS_thesis.pdf [Last accessed on 26 Mar 2024].
61. Turnquist MA, Rawls C. Multimodal network analysis and vulnerability assessment of U.S.-Canadian trade in Lake Erie Corridor. Transp Res Rec 2010;2168:9-16.
62. Han WJ, Hart J. Job precarity and economic prospects during the COVID-19 public health crisis. Soc Sci Q 2021;102:2394-411.
63. Stewart RD. Multimodal freight transportation within the Great Lakes-Saint Lawrence Basin. Transportation research board. 2012. Available from: https://nap.nationalacademies.org/catalog/22742/multimodal-freight-transportation-within-the-great-lakes-saint-lawrence-basin [Last accessed on 26 Mar 2024].
64. Zeuli K, Nijhuis A, Macfarlane R, Ridsdale T. The impact of climate Change on the food system in Toronto. Int J Environ Res Public Health 2018;15:2344.
65. Field CB, Barros V, Stocker TF, Dahe Q. Managing the risks of extreme events and disasters to advance climate change adaptation: special report of the intergovernmental panel on climate change. Cambridge: Cambridge University Press; 2012.
66. Pauw WP, Klein RJ, T, Vellinga P, Biermann F. Private finance for adaptation: do private realities meet public ambitions? Clim Change 2016;134:489-503.
Cite This Article
Export citation file: BibTeX | EndNote | RIS
OAE Style
Rahim MA, Wilson J, Johnson R. Defining a novel method to undertake sectoral risk management under climate change: a case study of intermodal transportation in the Peel Region, Ontario, Canada. Carbon Footprints 2024;3:8. http://dx.doi.org/10.20517/cf.2023.40
AMA Style
Rahim MA, Wilson J, Johnson R. Defining a novel method to undertake sectoral risk management under climate change: a case study of intermodal transportation in the Peel Region, Ontario, Canada. Carbon Footprints. 2024; 3(2): 8. http://dx.doi.org/10.20517/cf.2023.40
Chicago/Turabian Style
Muhammed Ahsanur Rahim, Jeffrey Wilson, Ryan Johnson. 2024. "Defining a novel method to undertake sectoral risk management under climate change: a case study of intermodal transportation in the Peel Region, Ontario, Canada" Carbon Footprints. 3, no.2: 8. http://dx.doi.org/10.20517/cf.2023.40
ACS Style
Rahim, MA.; Wilson J.; Johnson R. Defining a novel method to undertake sectoral risk management under climate change: a case study of intermodal transportation in the Peel Region, Ontario, Canada. Carbon. Footprints. 2024, 3, 8. http://dx.doi.org/10.20517/cf.2023.40
About This Article
Copyright
Data & Comments
Data
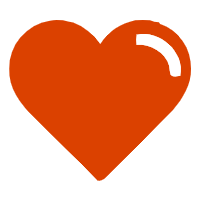
Comments
Comments must be written in English. Spam, offensive content, impersonation, and private information will not be permitted. If any comment is reported and identified as inappropriate content by OAE staff, the comment will be removed without notice. If you have any queries or need any help, please contact us at support@oaepublish.com.