Measures for response assessment in HCC treatment
Abstract
The evolving treatment landscape of hepatocellular carcinoma (HCC) includes curative treatments such as ablation, resection, and transplantation, along with palliative interventions such as locoregional and systemic therapies. Evaluating the response to therapy is critical to planning the next intervention or follow-up needed, as well as for comparing the outcomes across the treatment options. Response to therapy can be measured using serum markers, through pathology, using imaging surrogates, and clinical response. This review provides a brief overview of these measures of treatment response and their relevance to HCC management.
Keywords
INTRODUCTION
Hepatocellular carcinoma (HCC), the most prevalent primary liver cancer, often arises within the context of liver cirrhosis[1]. In 2020, primary liver cancer ranked as the sixth most frequently occurring cancer globally and the second leading cause of cancer-related mortality worldwide[2]. The primary curative treatment options for HCC are surgical resection and liver transplantation. However, the presentation of cirrhosis or multifocal tumors in many patients precludes these curative treatments. Ablation provides a minimally invasive curative treatment option in the setting of limited tumor size and number. Unfortunately, not all patients are good candidates due to the tumor size or location close to critical structures. In such instances, locoregional transarterial therapies (LRT) such as transarterial chemoembolization (TACE) and transarterial radioembolization (TARE) have been demonstrated to improve survival in the non-curative setting and can serve as a bridge to liver transplantation by reducing the tumor burden to within Milan criteria[3]. More recently, systemic treatment options have demonstrated efficacy in HCC including tyrosine kinase inhibitors, anti-vascular agents, and immunotherapies[4]. Finally, combining systemic therapy with locoregional therapies has been demonstrated to improve survival over either treatment alone.
The evaluation of HCC’s response to treatment can be measured using serum markers, pathology after resection, imaging surrogates, and clinical response. Each of these measures provides a distinct insight into the treatment response, and together, they provide complimentary assessments for monitoring response to therapy. In this review, we examine these measures of treatment response and their utility for the management of patients with HCC.
SERUM BIOMARKER RESPONSE ASSESSMENT
Serum levels of alpha-fetoprotein (AFP) are useful in following response to treatment in those patients whose HCC tumors express AFP[5]. A decrease of 20% in AFP during the initial weeks after treatment or more than 50% in the first month after treatment is indicative of a positive response and prolonged survival[6]. Similarly, changes in AFP-L3 levels after localized treatments or surgery for HCC have been linked to increased overall survival[7]. Most clinicians will continue to periodically monitor serum AFP levels after therapy for evidence of early tumor recurrence or the development of a new disease. However, the lack of AFP expression in many HCC tumors limits the effectiveness of AFP for measuring treatment response. Similarly, the genetic heterogeneity of HCC and variability in the expression of AFP and other serum biomarkers also limit their use as a prognostic indicator, as they often do not correlate with outcomes or responses to treatment[8]. For example, sorafenib, the first approved antiangiogenic agent for advanced HCC, has proven effective regardless of the patient’s initial biomarker levels, such as AFP, vascular endothelial growth factor (VEGF), or angiopoietin 2 (ANG-2)[9,10]. Similarly, serum levels of des-γ-carboxy prothrombin (DCP) have been related to positive outcomes and are seen as a prognostic indicator[11]. DCP levels below 40 mAU/mL have been related to longer survival and fewer recurrences in hepatitis B virus-affected patients following radiofrequency ablation[12]. Serum VEGF levels are known to be significantly elevated after tumor embolization due to tumor hypoxia and necrosis resulting in stimulation of tumor neoangiogenesis; however, a phase III trial to investigate TACE in combination with sorafenib failed to demonstrate an improvement in survival[13]. Serum liver function tests (LFTs) such as total bilirubin and liver transaminases reflect overall liver health and significantly influence prognosis in patients undergoing LRT. Elevated transaminases and total bilirubin predict worse outcomes post-LRT due to impaired liver recovery capabilities[14]. Conversely, patients with healthier liver indicators (e.g., normal serum liver function tests) typically experience better post-treatment survival, as their livers can effectively handle the therapy’s demands and support recovery[15]. Acute kidney injury (AKI) significantly affects the prognosis and survival of patients after LRT. The International Club of Ascites has updated AKI criteria to include a serum creatinine increase of ≥ 0.3 mg/dL within 48 h or a ≥ 50% rise from baseline, with a three-stage system[16]. Higher AKI stages correlate with poorer outcomes in HCC patients undergoing TACE[17]. Immunotherapy has revolutionized cancer treatment by targeting how cancer cells evade immune detection, particularly through proteins like programmed death-ligand 1 (PD-L1), programmed cell death-ligand 2 (PD-L2), and Cytotoxic T-lymphocyte associated protein 4 (CTLA-4)[18]. Monoclonal antibodies are used to block these proteins and activate the body’s immune response against cancer. Clinical trials of immunotherapy in HCC have demonstrated that tumors with high levels of PD-L1, increased VEGF receptor 2 expression, and a greater presence of T-regulatory (Tregs) cells have a greater response to immunotherapy and longer survival[19]. Recent clinical research has extensively explored the efficacy of combining tyrosine kinase inhibitors (TKIs) and immune checkpoint inhibitors (ICIs) in treating various cancers. These treatments synergistically inhibit tumor angiogenesis via TKIs and enhance immune responses against cancer cells through ICIs targeting CTLA-4, programmed cell death protein 1 (PD-1), and PD-L1. Notable clinical trials include a phase 1b study combining sorafenib with nivolumab for advanced HCC, which showed a significant increase in partial response rates compared to sorafenib alone[20]. Similarly, the KEYNOTE-524 trial of lenvatinib and pembrolizumab reported improved survival rates in HCC, indicating a synergistic effect[21]. Additionally, early studies of regorafenib with atezolizumab for metastatic colorectal cancer (mCRC) demonstrated the potential to overcome resistance to prior treatments[22]. The rationale for these combinations is their complementary effects on the tumor microenvironment - TKIs reduce the tumor’s nutrient supply, while ICIs counteract immune suppression. Eligibility for such therapies typically depends on specific tumor characteristics like PD-L1 expression or mutational burden, with contraindications including autoimmune conditions or prior adverse drug reactions. Predictive biomarkers such as PD-L1 and VEGF levels are crucial for selecting suitable patients, allowing for a more personalized treatment approach. These findings underscore the potential of TKI and ICI combinations to enhance cancer treatment outcomes, emphasizing the importance of continued research to optimize these strategies[23].
PATHOLOGIC RESPONSE ASSESSMENT
After resection, pathological responses to treatment are often separated into three categories based on the degree of tumor necrosis. Complete pathologic response (CPR) or complete pathologic necrosis (CPN) are used when more than 90% of the tumor is necrotic. Partial response (PR) is used when necrosis is between 50% and 90%. Treatment failure (TF) is used for any tumors with necrosis less than 50%[24]. Examining pathologic responses to transarterial therapies such as TACE after resection or transplantation reveals a wide range of pathologic responses. For example, different authors report complete necrosis in approximately 18%-70% of cases, partial response in 17%-25%, and treatment failure in 15%-30% of patients[25-27]. Higher rates of necrosis after TACE are associated with reduced recurrence rates of HCC post-transplantation[28]. Pathologists today do much more than just histological assessments. Their role now includes the characterization of the immune microenvironment of the tumor and the complex field of functional genomics, where they analyze genetic markers within tumors. This comprehensive profiling helps to understand the tumor’s biological activity and how the tumor might respond to different therapies. Such insights are invaluable in developing new treatments, as they can pinpoint specific genetic targets for novel drugs. In the future, these genetic profiles may allow for the customization of treatment plans to the individual’s unique genetic makeup of their cancer, improving both the efficacy of treatments and patient outcomes[29].
IMAGING RESPONSE ASSESSMENT
Commonly used imaging methods for response assessment include computed tomography (CT) and magnetic resonance imaging (MRI), and less commonly, contrast-enhanced ultrasound (CE-US) and fluorodeoxyglucose-positron emission tomography (FDG-PET). Treatment effectiveness is typically gauged by the radiologic assessment of objective response (OR), which is typically based on comparing measurements of tumor size and enhancement pre- and post-therapy[30]. Imaging OR is used as a surrogate biomarker for treatment response and patient survival in clinical trials. For example, patients with incomplete imaging responses to locoregional therapies experience higher rates of HCC recurrence post-liver transplant[28]. Although the value of OR is recognized in patients undergoing locoregional treatments like TACE or ablation, its significance in the context of systemic therapy or immunotherapy has yet to be fully validated[31].
Measuring objective response in clinical trials
Various scoring systems for measuring objective response have been developed that use recognized criteria for changes in tumor size or enhancement after treatment. These response criteria have been developed by organizations such as the World Health Organization (WHO), the European Association for the Study of the Liver (EASL), and the National Cancer Institute (NCI) [Figure 1][32]. Over the past two decades, these scoring systems and criteria have evolved to include tumor size, tumor number, and tumor enhancement [Table 1]. The original Response Evaluation Criteria in Solid Tumors (RECIST) criteria compares pre-treatment and post-treatment tumor diameter. The modified RECIST (mRECIST) and EASL criteria combine size criteria with the enhancement of the residual tumor, excluding areas of non-enhancement that are taken to reflect necrosis caused by treatment. Post-treatment, complete necrosis is the goal, though partial necrosis is often seen.
Figure 1. Comparing imaging response assessment and pathological response assessment. Create with BioRender. RECIST: Response Evaluation Criteria in Solid Tumors; WHO: World Health Organization; mRECIST: modified RECIST; EASL: European Association for the Study of the Liver; LI-RADS: liver imaging reporting and data system; CR: complete response; PR: partial response; SD: stable disease; PD: progressive disease; CPN: complete pathologic necrosis.
Types of RECIST criteria in image response
Name | Year of introduction | Imaging modality | Assessing factors |
RECIST 1.0 | 2000 | CT/MRI | Tumor size measured on CT or MRI |
RECIST 1.1 | 2009 | CT/MRI | Number of target lesions, measurement of lymph nodes, identification of new lesions |
mRECIST | 2010 | CT/MRI | Enhancement patterns on contrast-enhanced scans, diameter changes |
iRECIST | 2017 | CT/MRI | Tumor enlargement, appearance of new lesions, subsequent tumor shrinkage |
pRECIST | 2009 | FDG-PET | Changes in tumor metabolism as indicated by FDG uptake on PET scans |
mRECIST is the most used and extensively validated scoring system for evaluating responses to locoregional therapies in HCC. It categorizes treatment outcomes into four groups: complete response (CR), PR, stable disease (SD), and progressive disease (PD). CR means no enhancing “viable” tissue in the target lesions. PR is a decrease of at least 30% in the sum of the viable target lesions’ diameters. SD is when cases do not meet the criteria for PR or PD. PD is defined by a 20% increase in the sum of the diameters. Survival outcomes are poorer for HCC patients categorized as SD or PD after treatment[33] [Figure 2]. mRECIST is less effective in assessing infiltrative lesions and hypo-enhancing tumors, as well as in the context of systemic therapies. Additionally, changes in the liver tissue surrounding the treatment area, which might include altered blood flow, inflammation, necrosis, or fibrosis, can confound the response assessment. While guidelines for the ideal timing of post-treatment assessments are controversial, it is common to start evaluating one month after treatment for TACE and ablation, and 3 months after TARE to enable sufficient response to radiation. Afterward, multi-phase magnetic resonance (MR) or CT scans every three to four months are commonly used for maintaining surveillance[34]. The EASL guidelines include tumor enhancement. The guidelines, enhanced by the quantitative EASL (qEASL) criteria, adopt a three-dimensional analysis of tumor enhancement, significantly improving the accuracy of treatment response assessment[35]. This methodology is based on the differential analysis of pre- and post-contrast MRI, identifying viable tumor tissue through specific enhancement thresholds. Demonstrating a high correlation with histopathological findings in HCC, the qEASL criteria surpass RECIST, mRECIST, and traditional EASL benchmarks in predicting patient survival[33]. In the evaluation of hypovascular HCC tumors, tumor response criteria that include measures of enhancement such as mRECIST or EASL have lower utility for defining response, and advanced imaging techniques like dual-energy CT, contrast-enhanced ultrasond (CEUS), or MR perfusion studies are emerging as valuable tools[36]. RECIST primarily assesses tumor response through unidimensional measurements of tumor size, which may not fully capture the actual tumor burden or volumetric changes, as tumors can vary in shape and density. The criteria also rely on specific percentage thresholds to define progressive disease and partial response, potentially leading to misestimations of treatment effects when small yet clinically significant changes occur[37]. Additionally, RECIST does not consider tumor heterogeneity, which can result in variations in how different parts of the same tumor or different tumors within the same patient respond to treatment. This oversight may provide an oversimplified view of treatment efficacy. Moreover, RECIST fails to adequately track the dynamic changes in tumor growth or regression over time, with the potential to overlook rapid fluctuations depending on when imaging studies are performed, possibly leading to incorrect disease categorization. Furthermore, RECIST is not suitable for evaluating conditions like diffuse liver infiltration by cancer or certain leukemias, where there are no distinct, measurable lesions, necessitating alternative assessment methods[38].
Figure 2. Comparing various imaging assessment criteria at different levels with each other. RECIST: Response Evaluation Criteria in Solid Tumors; mRECIST: modified RECIST; EASL: European Association for the Study of the Liver; LI-RADS: liver imaging reporting and data system; CR: complete response; PR: partial response; SD: stable disease; PD: progressive disease.
Although overall survival (OS) remains the gold standard for assessing treatment efficacy, many treatments have been approved based on surrogate endpoints such as time to progression (TTP), progression-free survival (PFS), and objective response rate (ORR). PFS is recognized as a composite endpoint, incorporating two distinct variables: mortality and the presence of radiological progression, which is typically determined by established criteria such as qEASL or mRECIST. Similarly, TTP measures the duration from the point of trial enrollment to the occurrence of radiological progression, again defined under standard benchmarks like qEASL or mRECIST. These surrogate endpoints are predictive of OS and have become increasingly relevant in oncology, especially with the advent of effective post-progression therapies[39]. In the USA, the accelerated approval process for cancer treatments relies heavily on these surrogate endpoints. Despite their utility in speeding up therapy approvals, surrogate endpoints face challenges such as interpretation bias, often managed by centralized radiology reviews and adjudication[40].
Immunotherapy is showing promising outcomes for HCC and a well-tolerated safety profile[41,42]. Additionally, Tregs cells are identified as a critical component in the immunosuppressive microenvironment of HCC, especially prominent in the setting of chronic liver diseases, both viral and autoimmune. Tregs, characterized by CD4+ CD25+ Foxp3+ markers, play a significant role in the progression and invasiveness of tumors. They exert their influence via mechanisms such as cell-to-cell contact and the secretion of inhibitory molecules. Notably, these cells also express checkpoint molecules like CTLA-4 and PD-1, making them targets for ICI therapies. However, the efficacy and safety of these therapies can be complicated by the diverse roles Tregs play in different types of liver diseases, potentially leading to immune-related adverse events (irAEs). This complex interaction underscores the need for careful evaluation of Tregs in tumor biopsies, which could serve as prognostic markers for ICI outcomes[43]. The introduction of immunotherapy, both as a monotherapy and in synergy with antiangiogenic agents, presents challenges in the radiologic assessment of treatment response and disease progression, as inflammation induced by the therapy can cause a temporary growth in tumors and lymph nodes, mimicking tumor progression[44]. These challenges have led to the development of immune-specific response criteria to better evaluate treatment efficacy, including the immune-related RECIST (iRECIST) and the immune-modified RECIST (imRECIST) criteria. The criteria for immune complete response (iCR), immune partial response (iPR), and immune stable disease (iSD) continue to align with the definitions outlined in RECIST 1.1; however, the determination of progressive disease requires the verification of increased lesion size or number on serial imaging performed 4-8 weeks later to differentiate progression from pseudoprogression[45].
The PET response criteria in solid tumors (pRECIST) were proposed to address the limitations of traditional anatomical imaging measures like RECIST in evaluating the efficacy of cancer therapies. These criteria utilize PET imaging, most commonly with the radiotracer 18F-FDG, to assess the metabolic changes in tumors in response to treatment. pRECIST aims to capture the continuous and dynamic changes that occur in tumor metabolism after treatment, potentially allowing for earlier and potentially more accurate assessment of treatment response compared to size-based criteria alone. These criteria include quantitative measurements such as the standardized uptake value (SUV), which are used to assess changes in the metabolic activity of tumors. The pRECIST approach recognizes that tumors may show metabolic changes, such as a decrease in 18F-FDG uptake, that precede or do not correspond with changes in tumor size. Conversely, tumors and lymph nodes may remain stable in size after treatment, but demonstrate persistent or even increased metabolic activity at PET-CT, suggesting residual tumor. These metabolic changes may provide a more sensitive indicator of tumor response to therapy, especially with treatments that are cytostatic rather than cytotoxic, and therefore may not immediately lead to a reduction in tumor size but still represent a positive therapeutic response[46,47] [Table 2].
Comparative criteria for HCC response evaluation to systemic therapies
Criteria | Standard RECIST 1.1 | mRECIST for HCC | EASL guidelines | iRECIST | Metabolic RECIST (pRECIST) |
Measurement of lesion | Unidimensional, largest diameter | Unidimensional, enhancing tumor | Bidimensional, enhancing tumor | Unidimensional, largest diameter | PET-based assessment of metabolic activity |
Number of targets | 5 (max 2 per organ) | 5 (max 2 per organ), with arterial phase enhancement | All measurable lesions | 5 (max 2 per organ) | Not specifically limited; based on metabolic activity |
Target lesions | Measurable (> 10 mm), lymph node ≥ 15 mm in short axis | Measurable (> 10 mm), lymph node ≥ 20 mm in short axis at porta hepatis | Measurable lesions | Measurable (> 10 mm), lymph node ≥ 15 mm in short axis | Lesions with increased metabolic activity as per PET |
Nontarget lesions | Nonmeasurable or small lesions, ascites | Infiltrative HCC, malignant portal vein thrombosis, lesions with atypical enhancement | Nonmeasurable lesions | Nonmeasurable or small lesions, ascites | Lesions without significant metabolic activity or below PET detection threshold |
Complete response | Disappearance of all target lesions; lymph nodes < 10 mm | Disappearance of any intratumoral arterial enhancement in all target lesion(s) | Disappearance of any intratumoral enhancement | Disappearance of all target lesions; lymph nodes < 10 mm | No metabolic evidence of disease on PET scan |
PR | ≥ 30% decrease in the sum of diameters of target lesions | ≥ 30% decrease in the sum of diameters of enhancing target lesion(s) | ≥ 50% decrease in total tumor diameters of all measurable lesions | ≥ 30% decrease in the sum of diameters of target lesion(s) | Significant reduction in metabolic activity according to PET |
SD | Neither PR nor PD | Neither PR nor PD | Neither PR nor PD | Neither PR nor PD | No significant change in metabolic activity |
PD | ≥ 20% increase in the sum of diameters and ≥ 5-mm absolute increase | ≥ 20% increase in the sum of diameters of enhancing lesion(s) and/or new lesion(s) | ≥ 25% increase in size of ≥ 1 measurable lesion(s) and/or new lesion(s) | uPD: ≥ 20% increase in sum with absolute increase; cPD: further increase ≥ 5 mm | Increase in metabolic activity or new sites of disease on PET |
Imaging measures of response in clinical practice
The liver imaging reporting and data system (LI-RADS) from the American College of Radiology provides criteria for interpreting and reporting liver lesions after locoregional treatments and surgical resection. The LI-RADS Treatment Response (LR-TR) algorithm facilitates lesion categorization into three groups: LR-TR non-viable, LR-TR equivocal, and LR-TR viable. The non-viable category is for lesions showing no signs of tumor enhancement or only thin peripheral enhancement post-treatment. The viable category is designated for nodular or central enhancement that suggests a remaining tumor. The equivocal category is for atypical enhancements that do not quite match the expected patterns for complete response or definite residual tumor[48]. The LI-RADS criteria have demonstrated high positive and negative predictive values for determining the viability of HCC after TACE[49]. While these criteria typically assess individual lesions, they can also be adapted for broader patient-level response assessments, akin to mRECIST.
Imaging response assessment by treatment
After ablation, treatment success is defined by the creation of a non-enhancing ablation zone that encompasses the entire tumor and ideally includes a safety margin of at least 5 mm of the non-tumorous liver parenchyma. The formation of tiny gas bubbles from tissue boiling is observable immediately after ablation on CT. These typically resolve by the one-month follow-up imaging. It is important to distinguish these transient gas bubbles from persistent intra-tumoral gas indicative of a hepatic abscess or a hepatic infarct[50]. The main feature observed immediately within the ablation site post-treatment is a central area of coagulative necrosis, which appears as hyperdensity on CT and as a hyperintense signal on T1-weighted MRI[51]. These imaging findings generally resolve in follow-up scans. Subtraction imaging may assist in differentiating between hemorrhage and tumor enhancement on MRI. A uniform, thin enhancement of the treated zone, indicative of transient hyperemia, is a common response to thermal injury; however, this may initially obscure small areas of residual tumor. This enhancement typically resolves within a few months[52]. Persistent arterial enhancement with washout in delayed phase imaging during follow-up necessitates further evaluation for additional targeted treatment[53]. Independent of the treatment approach, nodular or thick arterial enhancement at the margin of a treated HCC suggests residual tumor and requires further intervention[54]. Arterioportal shunts resulting from thermal injury to small vessels may also be observed post-ablation but are expected to resolve upon follow-up[55].
After TACE with Lipiodol, unenhanced CT can assess the distribution of Lipiodol, with complete retention indicating lesion necrosis and partial retention suggesting a spectrum of responses. Lack of immediate post-therapy Lipiodol uptake in a portion of the tumor predicts incomplete therapy, likely due to vascular supply to the tumor outside of the treatment vessel[56]. Nevertheless, multiphasic CT imaging remains useful for evaluating areas without Lipiodol uptake by their patterns of contrast uptake and washout. Over time, necrotic tumor tissue continues to retain Lipiodol, albeit decreasing in size, making MRI preferable for evaluating residual or recurrent tumors[57].
After TARE, unique radiation effects are observed including diffuse heterogeneous enhancement within the treated liver segment, which could be misinterpreted as tumor progression. Reduced enhancement and size of the treated lesions correlate with pathologic necrosis, while peripheral nodular enhancement suggests viable tumor presence. The effect of TARE on tumor necrosis and shrinkage is not immediate, with median response times reported around 30 to 120 days. Interpreting initial post-procedural imaging post-TARE should be done with caution as it may be difficult to distinguish between normal post-treatment appearances and residual or recurrent tumors[58]. In addition, after TARE, tumor size and arterial enhancement may temporarily increase, a pseudo-progression phenomenon that complicates assessment. Serial imaging is thus recommended to monitor changes[59].
Like locoregional therapy outcomes, the presence of thick and nodular areas of arterial enhancement within HCC tumors post-systemic therapy indicates the persistence of untreated tumor tissue[60]. Conversely, the reduction in the tumor’s blood supply, as shown by decreased enhancement on imaging, is a key marker of a favorable response to treatment[61]. CT imaging has revealed that sorafenib can reduce the enhancement of HCC without necessarily impacting the size of the tumor[15]. MRI studies post-sorafenib treatment have predominantly identified T1 and T2 hyperintensity and non-enhancing necrosis as the main response patterns in the HCC[62]. Instances of increased tumor size attributed to necrosis have been reported, suggesting that the measurement of necrosis could serve as a more accurate metric for assessing treatment efficacy than changes in tumor size for HCC patients treated with systemic therapies[63].
Functional and metabolic imaging
Diffusion-weighted imaging (DWI) has become a valuable tool in the treatment response assessment in HCC. DWI tracks changes in apparent diffusion coefficient (ADC) values, which help distinguish viable from necrotic tumors[64]. Restricted diffusion serves as a marker for viable tumors after therapy. DWI is often used alongside contrast-enhanced T1-weighted enhanced imaging to monitor tumor response to both systemic and locoregional therapies. Correlations between ADC values and the extent of tumor necrosis confirmed by pathology confirm DWI’s potential to predict complete tumor necrosis following therapy. However, the performance of DWI by itself compared to contrast-enhanced imaging is variable, with some studies indicating lower sensitivity for DWI in identifying local HCC recurrence[65]. Merging DWI with morphologic evaluation criteria, such as EASL and mRECIST, provides an imaging strategy that assesses tumor response volumetrically and functionally. Additionally, perfusion studies like dynamic contrast enhanced (DCE)-MRI and CEUS offer semi-quantitative and alternative means to confirm the technical success of HCC therapies. These quantitative methods are validated even in traditionally challenging scenarios such as post-TARE or radiation. The integration of volumetric and functional imaging using DWI provides a more comprehensive understanding of treatment response and tumor viability in the HCC[66].
CLINICAL RESPONSE ASSESSMENT
The most important treatment outcome for both clinicians and patients is the clinical outcome, which includes measures of treatment toxicity, patient’s quality of life, and OS. Common Terminology Criteria for Adverse Events (CTCAE) framework facilitates the evaluation of adverse events such as hepatic dysfunction, fatigue, abdominal discomfort, and enzymatic fluctuations[67]. CTCAE toxicity grading allows the objective comparison between the toxicity profiles of different treatments, potentially prompting modifications in clinical management, including lengthening the time between treatments or the contemplation of alternate therapeutic options. Post-treatment toxicity can sometimes correlate with treatment response. For example, the onset of a hand-foot-skin reaction of grade 2 or higher within the initial two months following sorafenib has been associated with improved outcomes in patients with intermediate-stage HCC undergoing combined TACE and sorafenib therapy[68]. Severe post-treatment hepatic toxicity, however, often indicates that more severe underlying liver dysfunction was present than may have been appreciated pre-therapy[69]. The diligent surveillance, documentation, and management of toxicities, as dictated by CTCAE standards, are essential components in the therapeutic strategy for HCC patients undergoing TACE, ensuring that treatment decisions are informed by a holistic perspective on the patient’s health and prospects for recovery[70].
Quality of life (QoL) measures are infrequently the main objective in clinical studies[71]. However, considering the non-curative nature of many locoregional therapies, maintaining a satisfactory QoL is arguably as crucial as extending survival. The inclusion of Quality-Adjusted Life Years (QALY) as an outcome measure in evaluating response to locoregional therapies provides direct insight into effects on patient well-being and resource allocation in healthcare. Despite its importance, a paucity of prospective research thoroughly examines QoL outcomes in patients undergoing locoregional therapies[72]. The assessment and management of toxicity, guided by the CTCAE, offers a universal framework for classifying and grading the adverse effects of cancer therapies, which is indispensable for uniform toxicity reporting and management[73]. In the setting of clinical trials involving locoregional therapies for HCC, the OS represents the most definitive outcome measure, directly correlating with the goal of any cancer therapy, which is to extend the patient’s lifespan[74]. Unlike other surrogate markers of treatment efficacy such as tumor size reduction or biomarker levels, OS provides an unambiguous metric that accounts for all causes of death, not just cancer-specific mortality[75]. This makes it a gold standard for evaluating the success of locoregional therapies in clinical trials and practice. The outcomes of locoregional therapies can vary widely based on tumor characteristics, liver function, and patient comorbidities. Measuring OS allows clinicians to compare the real-world effectiveness of therapies. This is particularly important in HCC, where the liver’s compromised function due to underlying cirrhosis or hepatitis can influence patient outcomes independent of tumor response[76]. In the end, extending the quantity of life must also be balanced with quality of life, to ensure that treatment benefits are not outweighed by adverse effects. Therefore, OS remains a critical measure for clinicians to evaluate and refine TACE as a treatment for HCC, guiding decision making to optimize patient outcomes[77].
Comparing surrogate measures of response with clinical outcomes
The pathological response to TACE is recognized as a potential indicator of local disease control. Since the Child-Pugh score at the first TACE procedure remains the most robust predictor of overall survival in HCC, the pathological response cannot fully mirror the patient’s clinical response to treatment. This underscores the importance of evaluating treatment outcomes not only in terms of pathological changes but also in how these changes translate into tangible clinical benefits for patients[78]. Criteria for HCC response, which include measures of enhancement, are more reliable than tumor size alone in their ability to predict survival. Patients in the CR category in mRECIST have improved survival. While a link between pathological response and clinical outcomes is proposed, a thorough assessment should integrate radiological findings with clinical evaluations to form a complete prognosis[79].
ASSESSMENT CRITERIA BASED ON THE TYPE OF TREATMENT
The treatment of liver tumors, whether by surgical or non-surgical methods, demands distinct evaluation criteria due to the different mechanisms by which these treatments impact the disease.
Surgical treatments
Resection is the removal of the tumor along with a margin of healthy tissue. It is typically reserved for patients with sufficient liver function and without widespread liver involvement. The primary assessment criteria include complete resection with negative margins (R0 resection), post-surgical liver function, and the absence of postoperative complications like bile leaks or liver failure. Liver Transplantation is considered for patients with early-stage HCC who meet specific criteria such as the Milan Criteria (single tumor ≤ 5 cm or up to 3 tumors each ≤ 3 cm). The success is evaluated based on survival rates (1-year,
Non-surgical treatments
Ablative Techniques (e.g., Radiofrequency Ablation, Microwave Ablation) are used primarily for small tumors and in patients who are not suitable for surgery. The efficacy is assessed using imaging criteria such as the absence of viable tumor tissue on follow-up imaging and the size reduction of the ablated area over time. TACE is suitable for patients with multifocal HCC who do not have vascular invasion or extrahepatic spread, assessing response to TACE discussed above. Systemic Therapies (e.g., Sorafenib for HCC) are used for advanced liver cancers with extensive liver involvement or metastatic disease. The effectiveness is usually evaluated using mRECIST criteria based on changes in tumor size and number, as systemic treatments generally aim for disease stabilization rather than complete tumor eradication. These diverse treatment strategies and their corresponding assessment criteria reflect the complexity and heterogeneity of liver tumors, emphasizing the need for a tailored approach based on the individual patient’s disease stage and overall health [Figure 3].
Figure 3. Optimal assessment method for different HCC treatments at different disease stages. HCC: Hepatocellular carcinoma; LI-RADS: liver imaging reporting and data system; mRECIST: modified RECIST; EASL: European Association for the Study of the Liver; OS: overall survival; TTR: time to recurrence; QoL: quality of life.
FUTURE DIRECTIONS
HCC management is moving toward an integrative approach that combines imaging results with pathological insights and other biological markers to fine-tune the monitoring of TACE responses and enhance patient management strategies[80] [Figure 4]. Innovations such as hyperpolarized MRI, novel PET imaging radiotracers, and radiomic imaging assessment are at the forefront of these advancements. For instance, the observation of blurred tumor boundaries and peripheral enhancement often indicates peripheral micro invasion surrounding the tumor, which is closely associated with prognosis following surgery. Traditionally, microvascular invasion (MVI) can only be confirmed through postoperative pathological analysis. However, the advent of sophisticated computer algorithms has propelled numerous studies in recent years to employ radiomics and deep learning techniques for the preoperative prediction of MVI. This approach demonstrates a significant shift toward utilizing cutting-edge technologies to enhance diagnostic accuracy in assessing outcomes of treatment response[81,82]. In the context of TACE and TARE, radiomics features have been linked to key outcomes such as overall survival, progression-free survival, and specific response rates. This utility reflects the capacity of radiomics to capture subtle changes in tumor texture and heterogeneity post-treatment, providing a more accurate assessment of efficacy to help guide subsequent management[83,84]. Advances in technology have enabled the integration of radiomics and radiogenomics with clinical data to enhance decision making in cancer treatment, particularly in identifying biomarkers for immunotherapy. Functional and molecular imaging techniques provide comprehensive qualitative and quantitative assessments of tumors and immune cells, which are crucial for developing reliable biomarkers despite the challenges posed by tumor heterogeneity. Furthermore, artificial intelligence, including machine learning and deep learning, has revolutionized the extraction of image-based features, facilitating the characterization of tumor phenotypes and the prediction of treatment responses. Studies exemplify how radiomic profiles correlated with biological markers can predict responses to immunotherapy, underscoring the potential of imaging data in managing complex cases where traditional biomarkers fall short. Emerging approaches like radiogenomics are beginning to link imaging features with genomic data, offering promising insights into patient-specific treatment outcomes, particularly in non-small cell lung cancer and glioblastomas[85].
CONCLUSION
In summary, the ultimate measure of treatment response centers on patient-derived clinical outcome measures such as overall survival. Pathological analysis, traditionally held as the gold standard for measuring treatment response, has a poor correlation with clinical outcome measures. Advances in the molecular characterization of tumors have begun to improve our understanding of tumor biology and the predictive value of pathologic characterization. Imaging measures of response provide a non-invasive surrogate to clinical response, providing a snapshot of immediate tumor response and bearing a better correlation to clinical response assessment. By synthesizing advances in molecular pathology with advances in molecular and functional imaging, radiomics, and artificial intelligence, future measures of treatment response will better correlate with clinical measures of patient outcomes.
DECLARATIONS
Authors’ contributions
Conceptualized the review, conducted the literature review, and contributed to the writing and editing of the manuscript: Yazdanpanah F, Hunt S
Assisted in the literature review: Al-Daoud O, Moradpour M
Availability of data and materials
Not applicable
Financial support and sponsorship
None.
Conflicts of interest
All authors declared that there are no conflicts of interest.
Ethical approval and consent to participate
Not applicable.
Consent for publication
Not applicable.
Copyright
© The Author(s) 2024.
REFERENCES
1. Yang JD, Hainaut P, Gores GJ, Amadou A, Plymoth A, Roberts LR. A global view of hepatocellular carcinoma: trends, risk, prevention and management. Nat Rev Gastroenterol Hepatol 2019;16:589-604.
2. Sung H, Ferlay J, Siegel RL, et al. Global cancer statistics 2020: GLOBOCAN estimates of incidence and mortality worldwide for 36 cancers in 185 countries. CA Cancer J Clin 2021;71:209-49.
3. Huang A, Yang XR, Chung WY, Dennison AR, Zhou J. Targeted therapy for hepatocellular carcinoma. Signal Transduct Target Ther 2020;5:146.
4. Fan Y, Xue H, Zheng H. Systemic therapy for hepatocellular carcinoma: current updates and outlook. J Hepatocell Carcinoma 2022;9:233-63.
5. Galle PR, Foerster F, Kudo M, et al. Biology and significance of alpha-fetoprotein in hepatocellular carcinoma. Liver Int 2019;39:2214-29.
6. Liu L, Zhao Y, Jia J, et al. The prognostic value of alpha-fetoprotein response for advanced-stage hepatocellular carcinoma treated with sorafenib combined with transarterial chemoembolization. Sci Rep 2016;6:19851.
7. Piñero F, Dirchwolf M, Pessôa MG. Biomarkers in hepatocellular carcinoma: diagnosis, prognosis and treatment response assessment. Cells 2020;9:1370.
8. Johnson P, Zhou Q, Dao DY, Lo YMD. Circulating biomarkers in the diagnosis and management of hepatocellular carcinoma. Nat Rev Gastroenterol Hepatol 2022;19:670-81.
9. Llovet JM, Montal R, Sia D, Finn RS. Molecular therapies and precision medicine for hepatocellular carcinoma. Nat Rev Clin Oncol 2018;15:599-616.
10. Casadei-Gardini A, Orsi G, Caputo F, Ercolani G. Developments in predictive biomarkers for hepatocellular carcinoma therapy. Expert Rev Anticancer Ther 2020;20:63-74.
11. Feng H, Li B, Li Z, Wei Q, Ren L. PIVKA-II serves as a potential biomarker that complements AFP for the diagnosis of hepatocellular carcinoma. BMC Cancer 2021;21:401.
12. Tang SC, Wu YY, Lin ZW, et al. Prognostic implications of preoperative, postoperative, and dynamic changes of alpha-fetoprotein and des-gamma (γ)-carboxy prothrombin expression pattern for hepatocellular carcinoma after hepatic resection: a multicenter observational study. Front Oncol 2024;14:1425292.
13. Meyer T, Fox R, Ma YT, et al. Sorafenib in combination with transarterial chemoembolisation in patients with unresectable hepatocellular carcinoma (TACE 2): a randomised placebo-controlled, double-blind, phase 3 trial. Lancet Gastroenterol Hepatol 2017;2:565-75.
14. Chang Y, Jeong SW, Young Jang J, Jae Kim Y. Recent updates of transarterial chemoembolilzation in hepatocellular carcinoma. Int J Mol Sci 2020;21:8165.
15. Kudo M, Ueshima K, Ikeda M, et al. Final results of TACTICS: a randomized, prospective trial comparing transarterial chemoembolization plus sorafenib to transarterial chemoembolization alone in patients with unresectable hepatocellular carcinoma. Liver Cancer 2022;11:354-67.
16. Sohn W, Ham CB, Kim NH, et al. Effect of acute kidney injury on the patients with hepatocellular carcinoma undergoing transarterial chemoembolization. PLoS One 2020;15:e0243780.
17. Hayashi M, Abe K, Fujita M, Okai K, Takahashi A, Ohira H. Acute kidney injury after platinum-based transcatheter arterial chemoembolization and transarterial infusion chemotherapy in patients with hepatocellular carcinoma. Jpn J Clin Oncol 2020;50:36-43.
18. Zhang H, Dai Z, Wu W, et al. Regulatory mechanisms of immune checkpoints PD-L1 and CTLA-4 in cancer. J Exp Clin Cancer Res 2021;40:184.
19. Kudo M. Scientific rationale for combined immunotherapy with PD-1/PD-L1 antibodies and VEGF inhibitors in advanced hepatocellular carcinoma. Cancers 2020;12:1089.
20. Chen SC, Huang YH, Chen MH, et al. Anti-PD-1 combined sorafenib versus anti-PD-1 alone in the treatment of advanced hepatocellular cell carcinoma: a propensity score-matching study. BMC Cancer 2022;22:55.
21. Finn RS, Ikeda M, Zhu AX, et al. Phase Ib study of lenvatinib plus pembrolizumab in patients with unresectable hepatocellular carcinoma. J Clin Oncol 2020;38:2960-70.
22. Eng C, Kim TW, Bendell J, et al; IMblaze370 Investigators. Atezolizumab with or without cobimetinib versus regorafenib in previously treated metastatic colorectal cancer (IMblaze370): a multicentre, open-label, phase 3, randomised, controlled trial. Lancet Oncol 2019;20:849-61.
23. Shen KY, Zhu Y, Xie SZ, Qin LX. Immunosuppressive tumor microenvironment and immunotherapy of hepatocellular carcinoma: current status and prospectives. J Hematol Oncol 2024;17:25.
24. Cheng AL, Qin S, Ikeda M, et al. Updated efficacy and safety data from IMbrave150: atezolizumab plus bevacizumab vs. sorafenib for unresectable hepatocellular carcinoma. J Hepatol 2022;76:862-73.
25. Sarwar A, Bonder A, Hassan L, et al. Factors associated with complete pathologic necrosis of hepatocellular carcinoma on explant evaluation after locoregional therapy: a national analysis using the UNOS database. AJR Am J Roentgenol 2023;220:727-35.
26. Frenette CT, Osorio RC, Stark J, et al. Conventional TACE and drug-eluting bead TACE as locoregional therapy before orthotopic liver transplantation: comparison of explant pathologic response. Transplantation 2014;98:781-7.
27. Shim JH, Lee HC, Kim SO, et al. Which response criteria best help predict survival of patients with hepatocellular carcinoma following chemoembolization? A validation study of old and new models. Radiology 2012;262:708-18.
28. Wu ZJ, Xie YF, Chang X, et al. Type of necrosis influences prognosis in hepatocellular carcinoma after the first transarterial chemoembolization. Med Sci Monit 2021;27:e929884.
29. Malone ER, Oliva M, Sabatini PJB, Stockley TL, Siu LL. Molecular profiling for precision cancer therapies. Genome Med 2020;12:8.
30. Kulik L, Heimbach JK, Zaiem F, et al. Therapies for patients with hepatocellular carcinoma awaiting liver transplantation: a systematic review and meta-analysis. Hepatology 2018;67:381-400.
31. Lencioni R, Montal R, Torres F, et al. Objective response by mRECIST as a predictor and potential surrogate end-point of overall survival in advanced HCC. J Hepatol 2017;66:1166-72.
32. Mendiratta-Lala M, Masch WR, Shampain K, et al. MRI assessment of hepatocellular carcinoma after local-regional therapy: a comprehensive review. Radiol Imaging Cancer 2020;2:e190024.
33. Zhao Y, Duran R, Bai W, et al. Which criteria applied in multi-phasic CT can predict early tumor response in patients with hepatocellular carcinoma treated using conventional TACE: RECIST, mRECIST, EASL or qEASL? Cardiovasc Intervent Radiol 2018;41:433-42.
34. Choi MH, Park GE, Oh SN, et al. Reproducibility of mRECIST in measurement and response assessment for hepatocellular carcinoma treated by transarterial chemoembolization. Acad Radiol 2018;25:1363-73.
35. Chapiro J, Lin M, Duran R, Schernthaner RE, Geschwind JF. Assessing tumor response after loco-regional liver cancer therapies: the role of 3D MRI. Expert Rev Anticancer Ther 2015;15:199-205.
36. Hennedige T, Venkatesh SK. Imaging of hepatocellular carcinoma: diagnosis, staging and treatment monitoring. Cancer Imaging 2013;12:530-47.
37. Fournier L, de Geus-Oei LF, Regge D, et al. Twenty years on: RECIST as a biomarker of response in solid tumours an EORTC imaging group - ESOI joint paper. Front Oncol 2021;11:800547.
38. Seyal AR, Parekh K, Velichko YS, Salem R, Yaghmai V. Tumor growth kinetics versus RECIST to assess response to locoregional therapy in breast cancer liver metastases. Acad Radiol 2014;21:950-7.
39. Llovet JM, Montal R, Villanueva A. Randomized trials and endpoints in advanced HCC: role of PFS as a surrogate of survival. J Hepatol 2019;70:1262-77.
40. Zhao F. Surrogate end points and their validation in oncology clinical trials. J Clin Oncol 2016;34:1436-7.
41. Yau T, Park JW, Finn RS, et al. Nivolumab versus sorafenib in advanced hepatocellular carcinoma (CheckMate 459): a randomised, multicentre, open-label, phase 3 trial. Lancet Oncol 2022;23:77-90.
42. Finn RS, Qin S, Ikeda M, et al; IMbrave150 Investigators. Atezolizumab plus bevacizumab in unresectable hepatocellular carcinoma. N Engl J Med 2020;382:1894-905.
43. Granito A, Muratori L, Lalanne C, et al. Hepatocellular carcinoma in viral and autoimmune liver diseases: role of CD4+ CD25+ Foxp3+ regulatory T cells in the immune microenvironment. World J Gastroenterol 2021;27:2994-3009.
44. Cannella R, Lewis S, da Fonseca L, Ronot M, Rimola J. Immunotherapy-based treatments of hepatocellular carcinoma: AJR expert panel narrative review. AJR Am J Roentgenol 2022;219:533-46.
45. Seymour L, Bogaerts J, Perrone A, et al; RECIST working group. iRECIST: guidelines for response criteria for use in trials testing immunotherapeutics. Lancet Oncol 2017;18:e143-52.
46. Wahl RL, Jacene H, Kasamon Y, Lodge MA. From RECIST to PERCIST: evolving considerations for PET response criteria in solid tumors. J Nucl Med 2009;50 Suppl 1:122S-50S.
47. Storto G, Nicolai E, Salvatore M. [18F]FDG-PET-CT for early monitoring of tumor response: when and why. Q J Nucl Med Mol Imaging 2009;53:167-80.
48. Kielar A, Fowler KJ, Lewis S, et al. Locoregional therapies for hepatocellular carcinoma and the new LI-RADS treatment response algorithm. Abdom Radiol 2018;43:218-30.
49. Cunha G, Chernyak V, Fowler KJ, Sirlin CB. Up-to-date role of CT/MRI LI-RADS in hepatocellular carcinoma. J Hepatocell Carcinoma 2021;8:513-27.
50. Minami Y, Nishida N, Kudo M. Therapeutic response assessment of RFA for HCC: contrast-enhanced US, CT and MRI. World J Gastroenterol 2014;20:4160-6.
51. Granata V, Grassi R, Fusco R, et al. Diagnostic evaluation and ablation treatments assessment in hepatocellular carcinoma. Infect Agent Cancer 2021;16:53.
52. Maas M, Beets-Tan R, Gaubert JY, et al. Follow-up after radiological intervention in oncology: ECIO-ESOI evidence and consensus-based recommendations for clinical practice. Insights Imaging 2020;11:83.
53. Yaghmai V, Besa C, Kim E, Gatlin JL, Siddiqui NA, Taouli B. Imaging assessment of hepatocellular carcinoma response to locoregional and systemic therapy. AJR Am J Roentgenol 2013;201:80-96.
54. Lune P, Abdel Aal AK, Klimkowski S, Zarzour JG, Gunn AJ. Hepatocellular carcinoma: diagnosis, treatment algorithms, and imaging appearance after transarterial chemoembolization. J Clin Transl Hepatol 2018;6:175-88.
55. Vogl TJ, Emara EH, Elhawash E, et al. Feasibility of diffusion-weighted magnetic resonance imaging in evaluation of early therapeutic response after CT-guided microwave ablation of inoperable lung neoplasms. Eur Radiol 2022;32:3288-96.
56. Zhang W, Xu AH, Wang W, Wu YH, Sun QL, Shu C. Radiological appearance of hepatocellular carcinoma predicts the response to trans-arterial chemoembolization in patients undergoing liver transplantation. BMC Cancer 2019;19:1041.
57. Kloeckner R, Otto G, Biesterfeld S, Oberholzer K, Dueber C, Pitton MB. MDCT versus MRI assessment of tumor response after transarterial chemoembolization for the treatment of hepatocellular carcinoma. Cardiovasc Intervent Radiol 2010;33:532-40.
58. Sacco R, Mismas V, Marceglia S, et al. Transarterial radioembolization for hepatocellular carcinoma: an update and perspectives. World J Gastroenterol 2015;21:6518-25.
59. Ma Y, Wang Q, Dong Q, Zhan L, Zhang J. How to differentiate pseudoprogression from true progression in cancer patients treated with immunotherapy. Am J Cancer Res 2019;9:1546-53.
60. Chou R, Cuevas C, Fu R, et al. Imaging techniques for the diagnosis of hepatocellular carcinoma: a systematic review and meta-analysis. Ann Intern Med 2015;162:697-711.
61. Bruix J, da Fonseca LG, Reig M. Insights into the success and failure of systemic therapy for hepatocellular carcinoma. Nat Rev Gastroenterol Hepatol 2019;16:617-30.
62. Tsujita Y, Sofue K, Ueshima E, et al. Evaluation and prediction of treatment response for hepatocellular carcinoma. Magn Reson Med Sci 2023;22:209-20.
63. Hwang YJ, Lee Y, Park H, Lee Y, Lee K, Kim H. Prognostic significance of viable tumor size measurement in hepatocellular carcinomas after preoperative locoregional treatment. J Pathol Transl Med 2021;55:338-48.
64. Afaq A, Andreou A, Koh DM. Diffusion-weighted magnetic resonance imaging for tumour response assessment: why, when and how? Cancer Imaging 2010;10 Spec no A:S179-88.
65. Gluskin JS, Chegai F, Monti S, Squillaci E, Mannelli L. Hepatocellular carcinoma and diffusion-weighted MRI: detection and evaluation of treatment response. J Cancer 2016;7:1565-70.
66. Stefanini B, Ielasi L, Chen R, et al. TKIs in combination with immunotherapy for hepatocellular carcinoma. Expert Rev Anticancer Ther 2023;23:279-91.
67. Liu W, Xie Z, Shen K, et al. Analysis of the safety and effectiveness of TACE combined with targeted immunotherapy in the treatment of intermediate and advanced hepatocellular carcinoma. Med Oncol 2023;40:251.
68. Wang E, Xia D, Bai W, et al. Tumor hypervascularity and hand-foot-skin reaction predict better outcomes in combination treatment of TACE and sorafenib for intermediate hepatocellular carcinoma. BMC Cancer 2019;19:409.
69. Andrade RJ, Robles-Díaz M. Diagnostic and prognostic assessment of suspected drug-induced liver injury in clinical practice. Liver Int 2020;40:6-17.
70. Gaba RC, Lokken RP, Hickey RM, et al; Society of Interventional Radiology Standards of Practice Committee. Quality improvement guidelines for transarterial chemoembolization and embolization of hepatic malignancy. J Vasc Interv Radiol 2017;28:1210-23.e3.
71. Kirchner T, Marquardt S, Werncke T, et al. Comparison of health-related quality of life after transarterial chemoembolization and transarterial radioembolization in patients with unresectable hepatocellular carcinoma. Abdom Radiol 2019;44:1554-61.
72. Eltawil KM, Berry R, Abdolell M, Molinari M. Quality of life and survival analysis of patients undergoing transarterial chemoembolization for primary hepatic malignancies: a prospective cohort study. HPB 2012;14:341-50.
73. Guo T, Wu P, Liu P, et al. Identifying the best anticancer agent combination in tace for HCC patients: a network meta-analysis. J Cancer 2018;9:2640-9.
74. Celsa C, Cabibbo G, Enea M, et al. Are radiological endpoints surrogate outcomes of overall survival in hepatocellular carcinoma treated with transarterial chemoembolization? Liver Int 2021;41:1105-16.
75. Cammarota A, Zanuso V, Pressiani T, Personeni N, Rimassa L. Assessment and monitoring of response to systemic treatment in advanced hepatocellular carcinoma: current insights. J Hepatocell Carcinoma 2022;9:1011-27.
76. Cardarelli-Leite L, Hadjivassiliou A, Klass D, et al. Current locoregional therapies and treatment strategies in hepatocellular carcinoma. Curr Oncol 2020;27:S144-51.
77. Chen Z, Xie H, Hu M, et al. Recent progress in treatment of hepatocellular carcinoma. Am J Cancer Res 2020;10:2993-3036.
78. Yang K, Sung PS, You YK, et al. Pathologic complete response to chemoembolization improves survival outcomes after curative surgery for hepatocellular carcinoma: predictive factors of response. HPB 2019;21:1718-26.
79. Choi JY, Lee JM, Sirlin CB. CT and MR imaging diagnosis and staging of hepatocellular carcinoma: part II. Extracellular agents, hepatobiliary agents, and ancillary imaging features. Radiology 2014;273:30-50.
80. Deutsch JS, Cimino-Mathews A, Thompson E, et al. Association between pathologic response and survival after neoadjuvant therapy in lung cancer. Nat Med 2024;30:218-28.
81. Sun BY, Gu PY, Guan RY, et al. Deep-learning-based analysis of preoperative MRI predicts microvascular invasion and outcome in hepatocellular carcinoma. World J Surg Oncol 2022;20:189.
82. Liu SC, Lai J, Huang JY, et al. Predicting microvascular invasion in hepatocellular carcinoma: a deep learning model validated across hospitals. Cancer Imaging 2021;21:56.
83. Zhang Y, Shu Z, Ye Q, et al. Preoperative prediction of microvascular invasion in hepatocellular carcinoma via multi-parametric MRI radiomics. Front Oncol 2021;11:633596.
84. Stark S, Wang C, Savic LJ, et al. Automated feature quantification of Lipiodol as imaging biomarker to predict therapeutic efficacy of conventional transarterial chemoembolization of liver cancer. Sci Rep 2020;10:18026.
Cite This Article

How to Cite
Download Citation
Export Citation File:
Type of Import
Tips on Downloading Citation
Citation Manager File Format
Type of Import
Direct Import: When the Direct Import option is selected (the default state), a dialogue box will give you the option to Save or Open the downloaded citation data. Choosing Open will either launch your citation manager or give you a choice of applications with which to use the metadata. The Save option saves the file locally for later use.
Indirect Import: When the Indirect Import option is selected, the metadata is displayed and may be copied and pasted as needed.
About This Article
Copyright
Data & Comments
Data
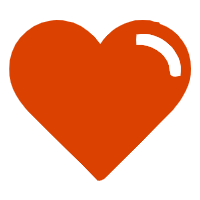
Comments
Comments must be written in English. Spam, offensive content, impersonation, and private information will not be permitted. If any comment is reported and identified as inappropriate content by OAE staff, the comment will be removed without notice. If you have any queries or need any help, please contact us at [email protected].