Angiogenesis: the Yin and Yang in intrahepatic cholangiocarcinoma
Abstract
The tumor microenvironment (TME) constitutes a complex structure comprising different cell types and soluble factors that surround the tumor and promote its progression. Primarily for its pivotal role in malignant growth, TME has become a potential therapeutic objective for developing new targeted therapy and a marker for assessing therapeutic response. In intrahepatic cholangiocarcinoma (iCCA), the second most common primary liver malignancy, TME has also gained a central role in understanding the mechanisms underlying tumor progression. In this review, we focused on the role of angiogenic factors and their pathway in iCCA and analyzed possible therapeutic and prognostic implications.
Keywords
INTRODUCTION
Cholangiocarcinoma (CCA) embraces a highly heterogeneous group of malignancies originating from different tracts of the biliary tree, which are classified in intrahepatic (iCCA), perihilar (pCCA) and distal (dCCA) CCA[1]. The iCCA can be further subclassified as mass-forming (MF), periductal-infiltrating (PI), and intraductal growing (IG). MF-iCCA subtype identifies a mass grown in the hepatic parenchyma; in the PI-iCCA subtype, the tumor originates in the duct wall and spreads longitudinally along the wall, while in the IG-iCCA subtype, it grows within the duct lumen[2]. From a histological point of view, it is possible to identify two macro subgroups in the conventional iCCA family according to the size of the affected ducts: small and large bile duct iCCA. The former type arises in small intrahepatic bile ducts and may derive from progenitor cells and cuboidal cholangiocytes. The latter originates in large ducts from columnar cholangiocytes or peribiliary glands[1]. Almost all pCCA and dCCA share the same histological derivation with large duct iCCA[3,4]. We will focus on iCCA not only due to its standing as the second most frequent primary liver cancer but also owing to its swiftly escalating global incidence[5,6]. Surgery is the main therapeutic option, with liver transplant offering a curative option for specific cases[7]. However, the diagnosis usually occurs at an advanced stage when tumors are unresectable or not transplantable. In these cases, classical chemotherapeutic agents are the first-line therapy but with limited benefit for survival[8,9].
Most CCA cases are sporadic and often related to heterogeneous risk factors, distributed differently according to the geographical area. Inflammatory damage of the bile duct [e.g., hepatobiliary flukes infections, hepatolithiasis, primary sclerosing cholangitis (PSC), and viral hepatitis B and C] are the most frequent causes. CCA development also recognizes relevant genetic alterations with different genetic profiles among the subtypes. Fibroblast Growth Factor Receptor 2 (FGFR2) alteration and Isocitrate Dehydrogenase 1,2 (IDH-1/2) and BRCA-1 associate protein (BAP1) mutation are more commonly found in small bile duct iCCA, while KRAS and TP53 mutations cluster in large bile duct iCCA. Protein Kinase
These data indicate the need and utility of elucidating the molecular biology of iCCA to identify possible preventive measures and novel treatment options for these conditions. Recently, the identification of some of the above-indicated molecular alterations has paved the way for the development of targeted therapies, such as IDH-1, FGFR2, and BRAF inhibitors. Unfortunately, most of these therapies have worked no better than chemotherapy alone, and the true effectiveness of these treatments is yet to be proven[12].
The TME surrounding tumor cells has attracted growing interest in the last few years. TME combines heterogeneous components, including different infiltrating and resident cell types, secreted factors, and extracellular matrix. Each part exerts a specific role in promoting tumor progression, cancer cell survival, or metastatic dissemination[13]. TME is increasingly acquiring a greater role as a player both in the progression and aggressiveness of iCCA and in the response to therapy. Although the biliary epithelium following chronic inflammation is the main target of neoplastic transformation, tumor stroma is also modified by the regenerative process during bile duct repair[14,15]. Among the different TME components (myofibroblasts, inflammatory cells, endothelial cells, and mesenchymal stem cells), the main role is performed by cancer-associated fibroblast (CAFs), which secrete several substances that promote both cancer progression and tumor proliferation and angiogenesis[16]. Although how this process works in cancer progression is well known, it has been underappreciated in iCCA evolution, especially as a potential therapeutic target. Therefore, in this review, we will focus on the main angiogenic factors involved in iCCA progression and their current and future use in therapy.
ANGIOGENESIS IN ICCA
The relationship between tumor-associated angiogenesis and TME is fundamental for sustaining tumor progression. A tumor needs a huge vascular network to nourish itself for a rapid growth. The first description of a correlation between cancer development and its sustenance through blood supply was made more than ten years ago[17]. However, not all new blood vessels are functional. Many of them are characterized by an immature phenotype with decreased performance, resulting in a low oxygen rate and scarce nutrient supply. This deficiency in the vascular compartment is responsible for establishing a hypoxic TME. Hypoxia relates closely with tumor progression and metastasis[18]. The main effects associated with hypoxia are mediated by Hypoxia Induced Factor (HIF)-1α, whose overexpression positively relates to tumor size and decreased disease-free survival in iCCA[19]. HIF-1α expression promotes the expression of angiogenic factors, such as the Vascular Endothelial Growth Factors (VEGF) family and Angiopoietin (Ang)-2, responsible for the neoangiogenesis process and cholangiocyte proliferation[20,21]. However, the low oxygen rate may determine a balance between cell proliferation and apoptosis, which can inhibit tumor growth. This process is orchestrated by growth factors and cytokines secreted by TME in a paracrine manner during the angiogenic dormancy[22,23]. It is a balanced condition regulated by both pro- and anti-angiogenic factors [e.g., Thrombospondin (TPS) -1], which maintain tumors in a sort of avascular or poorly vascularized dormant conditions. Any perturbation of this quiescent state determines a disequilibrium towards pro-angiogenic factors, called "angiogenic-switch", which favors a hypervascularized phenotype to escape dormancy[23,24]. One of the biological events emerging as crucial for dormancy state maintenance is autophagy. It has been widely demonstrated that this fundamental physiological process, essential for cellular homeostasis and energy balance, also plays a key role during cancer progression[25]. At an early tumor stage, functional autophagy grants maintenance of cellular stability by preventing genome damage and ROS accumulation. At an advanced stage or in fast-growing tumors, autophagy is essential to promote survival and compensate for metabolic requirements due to nutrient deficiency and hypoxia[26,27]. Therefore, a hypoxic TME may affect and boost autophagic events strictly correlated to the preservation of the dormancy state.
The iCCA is characterized by a dense desmoplastic stroma, the main cellular component of which is CAF expressing a-smooth muscle actin (a-SMA)[28]. CAFs promote not only tumor growth and invasion but also angiogenesis through the release of several molecules, such as VEGF, fibroblast growth factor (FGF), and interleukin-6 (IL-6)[29]. Moreover, the presence of VEGF-A, IL-10 and transforming growth factor beta (TGFb) in the TME promotes the polarization of macrophage towards the pro-tumorigenic and pro-angiogenic phenotype M2[30]. Thus, TME is shaped by cancer cells to be specialized to assist cancer progression, becoming, among other things, an ideal target for anticancer treatment[31].
The iCCA was previously considered a hypovascular tumor in contrast to hepatocellular carcinoma (HCC)[32]. It is clear that tumor angiogenesis is marked in iCCA and is a feature enhancing biological aggressiveness and metastatic capacity[33]. Further knowledge of angiogenic factors and their role in iCCA is needed to better understand how they may influence the progression and, eventually treatment of this tumor. The data available so far with anti-angiogenic drugs, alone or in combination, are not straightforward to interpret, although individual studies seem to suggest an improvement in outcome, especially for iCCA [Table 1].
Main clinical trials with anti-angiogenic drugs (alone or in combination) in advanced biliary cancer
Author | Design | Drug | Regimen | Condition | Primary outcome | Result |
Zhu et al.[34] | Phase II | MAb + CT | Bevacizumab + GEMOX | 35 pts with metastatic BTC; 22 (62.8%) | PFS | Median PFS was 7·0 mo and mOS 12.7 mo; median PFS in pts with iCCA was 7.6 mo and mOS 14.2 mo |
Lubner et al.[35] | Phase II | mAb + TKI | Bevacizumab + erlotinib | 53 pts with BT; 35 (66.0%) with iCCA | Response rate | PR 12%; SD 51% mOS: 9.9 mo; TTP: 4.4 mo iCCA: no differences reported |
Guion-Dusserre et al. | Phase II | mAb + CT | Bevacixumab + FOLFIRI | 13 pts with iCCA, GEMOX pre-treated | Tumor control | The disease control rate was 84.5%, PFS 8 mo, median OS 20 mo |
Iyer et al.[37] | Phase II | mAb + CT | Bevacizumab + Cape + Gem | 50 pts with advanced BTC 29 with iCCA | Safety/efficacy | Grade 3-4 toxicities: 24% of pts. mPFS 8.1 mo No improvement with the addition of Bevacizumab iCCA: PR 21%; SD 52% |
Brechon et al.[38] | Phase II | mAB + CT vs. CT | GEMOX-bevacizumab vs. GEMOX | 32 pts with advanced BTC | PFS | CT + Beva vs. CT: 6.48 mo vs. 3.72 mo |
Larsen et al.[39] | Phase II | mAb + CT | Bevacizumab + Cape + Gem + irinotecan | 40 advanced BTC, 29 iCCA | PFS | Overall: mPFS 3.6 mo, mOS 6.4 mo iCCA: no differences reported |
Pei et al.[40] | Phase II | mAb + CT | Bevacizumab + Cape + Cis | 30 advanced BTC, 28 iCCA | ORR | ORR was 50.0% Partial response: 15 (50.0%) Stable disease: 9 (30.0%) All but one iCCA |
Bengala et al. | Phase II | TKI | Sorafenib | 46 pts with advanced BTCs, 27 (60.0%) iCCA | Disease control rate at 12 weeks | Disease control rate at 12 w: 32.6%; mPFS No difference between intra- and extrahepatic CCA |
Yi et al.[42] | Phase II | TKI | Sunitinib | 56 pts with advanced CCA | mTTP: 1.7 mo; Objective RR: 8.9%; Grade 3-4 toxicities in 46.4% of patients | |
El-Khoueiry et al.[43] | Phase II | TKI | Sorafenib | 31 pts with advanced BTCs [19, (61%) with CCA, not defined if iCCA or eCCA] | ORR | ORR: 0% (not achieved primary endpoint); SD 39%; PFS 3 mo; mOS 9 mo iCCA: no differences reported |
Lee et al.[44] | Phase II | TKI + CT | Sorafenib + Gem/Cis | 39 pts with advanced BTC, I line. 23 (59%) had iCCA | 6-mo PFS | PFS 6 mo 51% Median PFS: 6.5 mo iCCA: no differences reported |
El-Khoueiry et al.[45] | Phase II | TKI | Sorafenib + erlotinib | 34 pts with metastatic BTCs I line. 20 (59%) had CCA | PFS | Early termination for failure to meet predefined criteria. mPFS: 2 mo; mOS: 6 mo iCCA: no differences reported |
Moehler et al. | Double-blind | TKI | Sorafenib + Gem vs. placebo + Gem | 102 unresectable or metastatic BTC. iCCA: 33/49 Sor/Gem vs. 29/48 Plac/Gem | PFS | PFS: 4.9 vs. 3.0 mo (P = 0.859); mOS: 11.2 Subgroup analysis: better PFS for patients with iCCA |
Santoro et al. | Randomized phase II | TKI | Vandetanib vs. vandetanib + Gem vs. Gem | 173 patients with advanced BTCs. 87 (50.6) with iCCA | mPFS | mPFS: 105 vs. 114 vs. 148 days (P = 0.18). iCCA: no differences reported |
Valle et al.[48] | Randomized phase II | TKI | Cediranib vs. placebo + cisplatin/Gem | 124 pts with advanced BTC, 62 assigned to the intervention arm (14 iCCA in the latter) | mPFS | mPFS: 8 vs. 7.4 mo (P = 0.72) iCCA: no differences reported |
Dreyer et al.[49] | Case report | TKI | Sunitinb | 3 pts with progressive advanced CT-resistant intrahepatic cholangiocarcinoma | Disease control | Sustained disease control > to 4 mo |
Kessler et al. | Phase 1 | TKI | Vandetanib + Gem/Cape | 9 pts in the dose escalation (1 CCA) and 14 in the dose expansion cohort (6 with CCA) advanced BTC. | MTD/safety | Satisfactory safety profile |
Shroff et al.[51] | Open-label, multicenter, single-arm trial | TKI + mek inhibitor | Pazopanib + trametinib | 25 pts with advanced CAA. 5 (20%) iCCA | PFS | 4-mo PFS not significantly different from a prespecified null hypothesized 4-mo 25%PFS |
Sun et al.[52] | Phase II | TKI | Regorafenib | 43 pts with advanced BTC, 27 (62%) with iCCA | Grade 3-4 toxicities: 40% of pts. mPFS 15.6 weeks; mOS 31.8 weeks iCCA: no differences reported | |
Kim et al.[53] | Phase II | TKI | Regorafenib | 39 pts with advanced BTCs, 27 (69.2%) iCCA | 6-mo OS | mPFS 3.7 mo, mOS 5.4 mo Three PR, all iCCA |
Cousin et al. | Phase II | TKI + ICI | Regorafenib + avelumab | 34 pts with pre-treated BTC, 26 (76.5%) with iCCA | ORR | 4 PR, 11 SD. 14 of these patients had an iCCA. |
Ding et al.[55] | Multicenter observational study | TKI + ICI | Sintilimab + lenvatinib | 41 patients with advanced iCCA | TTP | Median TTP: 6.6 mo TTP was significantly improved (16.9 mo) in pts with PD-L1 TPS ≥ 10%, |
Zhu et al.[56] | Multicenter real-world study | TKI + ICI + CT | Lenvatinib+ toripalimab + gemcitabine + oxaliplatin | 53 patients with advanced ICCA | OS and PFS | OS 14.3 mo PFS 8.63 mo |
Shi et al.[57] | Phase II | TKI + ICI | Toripalimab + lenvatinib + gemcitabine + oxaliplatin | 30 pts with pathologically confirmed advanced ICC | ORR | ORR: 80% |
Different angiogenic factors involved in iCCA
The role of angiogenic factors and their complex interactions in iCCA progression are discussed in the following paragraphs and depicted in Figure 1.
Figure 1. Role of the angiogenic factors in iCCA progression. In this schematic Figure 1 (A) we demonstrate the main function exerted by angiogenic factors during iCCA progression. VEGF promotes cancer cell proliferation and metastatic spread, both inhibited by ET-1 and Ang-1. Ang-2 and TSP1, on the other hand, are mainly involved in vascular remodeling during the neoangiogenic process and lymphangiogenesis, respectively, becoming key players in iCCA invasiveness; (B) depicts the complex interplay between angiogenic factors in the CCA microenvironment. Only the most relevant complex exchanges and cellular components characterizing TME are shown in the figure. TAMs: Tumor-associated macrophages; CCA cells: cholangiocarcinoma cells; CAFs: Cancer-associated fibroblasts. The figure was created with Biorender.com
VEGF
VEGF is a family of signaling molecules comprising VEGF-A, -B, -C, -D and placental growth factor (PLGF), which exert their role through a group of cognate protein, tyrosine kinase receptors (VEGFRs)[58]. VEGF-A is the key modulator of angiogenesis in human diseases, including cancer. It exerts its role through VEGFR-1 (Flt-1), which, together with VEGFR-2 (Flk-1), represent the two isoforms mainly involved in angiogenesis and vasculogenesis, while VEGFR-3 (Flt-4) mediates lymphangiogenesis[59]. VEGF has a recognized fundamental role in determining tumor progression. It is overexpressed in many types of tumors (i.e., breast, lung, melanoma)[60-62].
Several studies demonstrate that VEGF signaling has a crucial role in biliary tree development as well as in iCCA progression. VEGF/VEGFR signaling pathway is involved in bile duct maturation at the early stage, while it is absent in the mature stage. However, this pathway can be reactivated during disease conditions[63].
In response to a hypoxic microenvironment, VEGF can also be secreted by tumor cholangiocytes, especially those expressing the angiogenic receptors VEGFR and Tie2 to promote cell proliferation[20,21]. CCA cells also secrete and express VEGF and its receptors via the action exerted by other factors, including the estrogen receptors through which VEGF stimulates cell proliferation[64]. However, the prognostic relevance of VEGF is still under debate due to controversial findings in the literature.
In eCCA patients with VEGF positivity, the overall survival is worse compared to those with negative VEGF[65]. Conversely, Kawahara et al. showed evidence of a lower VEGF expression in CCA patients compared to control[32]. More recently, Möbius et al. evaluated the potential prognostic role of microvessel density (MVD) in eCCA, showing a better prognosis in patients with low MVD than patients with high MVD[66]. Moreover, according to Kawahara et al., they found no correlation between VEGF expression and survival[32].
In contrast with the studies mentioned above, other research groups have positively associated VEGF levels and patients' survival. Yoshikawa et al. demonstrated that both iCCA and eCCA express higher levels of VEGF than controls and that high expression of VEGF is clinically correlated to intrahepatic metastasis in iCCA[67]. Cai et al. showed that iCCA patients with high expression of VEGF had shorter overall survival[68]. Currently, most studies in the literature positively correlate VEGF expression with a worse prognosis in patients with iCCA, as also shown by Calastri et al.[69]. Consequently, VEGF overexpression has become a strategic therapeutic target, suggesting using anti-VEGF drugs in association with traditional chemotherapy treatments. In phase II studies of single-agent sorafenib (a tyrosine kinase inhibitor with a weak VEGF capacity), limited benefit has been reported in patients with advanced biliary tract carcinoma[41,45].
In a multicenter prospective open-labeled prospective study conducted to evaluate the effectiveness and safety of sorafenib in combination with the best supportive care in patients with advanced iCCA, the median progression-free survival was 3.2 months and the median overall survival was 5.7 months[70]. Monoclonal antibody bevacizumab, a recombinant humanized monoclonal antibody against VEGF, is currently used in clinical practice for cancer treatment. Recently, a study in patients with metastatic carcinoma of the biliary tract demonstrated that the addition of bevacizumab to standard chemotherapy (gemcitabine and oxaliplatin) significantly increased the progression-free survival of patients compared to chemotherapy alone[38]. Another VEGF inhibitor, Apatinib, has been shown to effectively block proliferation, migration, and angiogenesis[71] and promote apoptosis[72] in iCCA cell lines, suggesting potential usefulness in patients with advanced iCCA[73]. Conversely, the combination of pazopanib-a multikinase inhibitor of VEGFR, platelet-derived growth factor receptor (PDGFR), fibroblast growth factor receptor (FGFR)- with trametinib-a highly specific MEK inhibitor- did not achieve a statistically significant improvement in PFS in patients with refractory CCA[51]. However, the promising results in this field demonstrate that the path of angiogenic pathway inhibition deserves further exploration.
Angiopoietins
The Angiopoietin system comprises Angiopoietin 1 (Ang-1) and Angiopoietin-2 (Ang-2), both acting as vascular remodeling mediators through their cognate tyrosine kinase receptor Tie-2 that promote the stabilization or destabilization of newly formed vessels in several vascularized tumors[74]. Both Ang-1 and Ang-2 interact with VEGF-A, but quite differently. Ang-1 and VEGF-A cooperate to promote angiogenesis. Ang-1 can exert a synergistic action with VEGF-A, amplifying its initial pro-angiogenic response[75]. The relationship between Ang-2 and VEGF-A is more complex, as these two factors exert a reciprocal influence. Ang-2 overexpression results in the loosening of vascular structures and exposure of endothelial cells to VEGF, which acts on endothelial cells, allowing further Ang-2 action[76]. The VEGFR and Tie-2 pathway are depicted in Figure 2. Tang et al. observed Ang-2 expression in 57.6% of cases and MVD significantly higher in VEGF-positive and Ang-2-positive cases, suggesting cooperation in promoting angiogenesis in CCA[77]. Ang-2 has been detected in the serum and bile of CCA patients, with a positive correlation with the disease only in the former, suggesting its possible use in differentiating CCA against primary sclerosis cholangitis (PSA)[78]. Comparing CCA with non-CCA patients, Kimawaha et al. reported that high levels of Ang-2 could correlate with severe cancer stage and metastasis[79]. Our recently submitted work demonstrated that Ang-2 alone or in association with VEGF increased migration and invasiveness in a 3D in vitro model of iCCA (personal communication).
Figure 2. VEGFR and Tie-2 pathway. On the endothelial cell surface, VEGF binding to its cognate receptor family lead to the activation of a variety of signaling pathway involved in survival (PI3K-AKT), migration (P38/MAPK and FAK/Paxillin), and proliferation (MAPK). Ang-2 is the main competitor of Ang-1 for Tie-2 binding. Under normal conditions, Ang-1 binds Tie-2 to maintain vascular integrity. In pathological conditions, Ang-2 is overexpressed and induces endothelial destabilization, inflammation, and vascular remodeling. Both VEGFR and Tie-2 pathways promote the initiation of new vessel formation and maturation. A hypoxic TME determines an overexpression of VEGF and Ang-2 and induces neoangiogenic processes. The figure was created with Biorender.com.
Conversely, Ang-1 expression correlates with lower metastatic spread risk in hilar cholangiocarcinoma (HC), as well as the presence of Tie2-positive monocytes (TEMs) in proximity to microvasculature is associated with reduced tumor recurrence[80]. The positive impact of Ang-1 and TEMs on CCA is further confirmed by another study by Atanasov et al.[81]. They hypothesized a possible influence of miR-126 in the upregulation of Ang-1, which recruits more TEMs, exerting an inhibitory effect on CCA progression.
Thrombospondin 1
Another important factor involved in the angiogenic process is TSP-1. TSP-1 is a multifunctional matrix protein[82] whose inhibitory effect on CCA angiogenesis has already been reported[32,83].
In agreement with these studies, Tang et al. observed that the TSP-1-positive group had a lower MVD than the negative group, confirming a possible inhibitory role in CCA angiogenesis[77]. Moreover, TSP-1-positive patients were more prone to develop metastasis than negative ones, highlighting an association between TSP-1 expression and CCA invasiveness. It has been observed that high TSP-1 expression in iCCA is related to increased lymphangiogenesis[83,84]. Conversely, a positive correlation between TSP-1 and VEGF expression was found in hepatocellular carcinoma (HCC), together with an increased rate of venous invasion[85]. These findings further explain the structural differences between HCC and iCCA, mentioned previously in terms of tumor vasculature, making the progression of the former strictly dependent on neovascularization rather than the progression of the latter, which is more related to lymphatic network development. A recent study designates TSP-1 as a potential serological marker predictive of the effect of gemcitabine-based chemotherapy in iCCA[86].
Endothelins
In addition to their role as potent endogenous vasoconstrictors and in cardiovascular and renal disorders, endothelins (ETs) are also responsible for diverse mechanisms in many types of cancer. For example, they play roles in proliferation, escaping from apoptosis, modulating immunity, and contributing to angiogenesis. ETs are a group of three peptides of 21aa ET-a, ET-2, and ET-3, which exert their function via the activation of two G-protein-coupled receptors (GPCR), ETA receptor (ETAR) and ETB receptor
Different studies demonstrated the blockage of ET axis in different types of cancer. Colorectal carcinoma[89], gastric cancer[90], and pancreatic cancer[91] could enhance the effect of conventional chemotherapeutic treatments and arrest cancer progression.
In this context, ET1 is particularly active in the neovascularization process, exerting a potent additive effect with VEGF[92]. Of note, in certain types of cancer, the overexpression of ET1 is strictly related both to the expression of VEGF and its receptors and to MVD[93]. Conversely, in CCA, ET1 inhibits the proliferation of malignant cells and the expression of VEGF proteins and their receptors. Considering the previously mentioned role of VEGF in CCA progression, these findings corroborate the close relationship between VEGF expression and ET1[94].
Thus, clarifying the role and frequencies of the ET axis makes it possible to use endothelin receptor antagonists to improve chemotherapeutic treatments.
CONCLUSION
Angiogenesis is a fundamental process during many physiological and pathological conditions. It is now recognized as a crucial aspect of cancer progression and dissemination. It is necessary to point out that although the main angiogenic factors involved in the progression of iCCA have been discussed, other equally important ones, such as FGF or PDGF, have not been explored in depth in this work. However, considering the role of each angiogenic factor in iCCA pathogenesis, as we have done in this review, it is possible to identify many controversial aspects in their action. The aforementioned studies highlight the opposite effects mediated by thrombospondin and endothelin. For example, they clarify the dual aspect of angiogenesis in iCCA, a “good” and a “bad” one, as in the "yin and yang" theory. Going deeper into this aspect could be an important goal for therapeutic advancement. As recently highlighted by Rizzo et al., in patients with advanced biliary tract cancer, the combination of cisplatin plus gemcitabine remains the only therapeutic option, although many targeted therapies developed in recent years have modified therapeutic scenarios[95]. Some therapeutic strategies targeting angiogenic pathways are already available, but further investigations are needed to develop and optimize tailored therapies. For example, developing technologies that improve drug deliverability to the target, such as nanoparticles, could represent a good option in the future of iCCA treatment, as the increasing development and use of artificial intelligence in clinical practice could improve the diagnosis and management of patients.
DECLARATIONS
Authors' contributionsMade substantial contributions to the conception and writing of the manuscript: Romanzi A, Villa E
Availability of data and materialsNot applicable.
Financial support and sponsorshipThis work was supported by Associazione Italiana sulla Ricerca sul Cancro (AIRC; Grant No. IG 2020-ID. 24858 project-P.I. Villa Erica).
Conflicts of interestAll authors declared that there are no conflicts of interest.
Ethical approval and consent to participateNot applicable.
Consent for publicationNot applicable.
Copyright© The Author(s) 2023.
REFERENCES
1. Banales JM, Marin JJG, Lamarca A, et al. Cholangiocarcinoma 2020: the next horizon in mechanisms and management. Nat Rev Gastroenterol Hepatol 2020;17:557-88.
2. Yamasaki S. Intrahepatic cholangiocarcinoma: macroscopic type and stage classification. J Hepatobiliary Pancreat Surg 2003;10:288-91.
4. Rodrigues PM, Olaizola P, Paiva NA, et al. Pathogenesis of cholangiocarcinoma. Annu Rev Pathol 2021;16:433-63.
5. Hucke F, Pinter M, Hucke M, et al. Changing epidemiological trends of hepatobiliary carcinomas in Austria 2010-2018. Cancers 2022;14:3093.
6. Turati F, Bertuccio P, Negri E, La Vecchia C. Epidemiology of cholangiocarcinoma. Hepatoma Res 2022;8:19.
7. Mauro E, Ferrer-Fàbrega J, Sauri T, et al. New challenges in the management of cholangiocarcinoma: the role of liver transplantation, locoregional therapies, and systemic therapy. Cancers 2023;15:1244.
8. Valle J, Wasan H, Palmer DH, et al. ABC-02 Trial Investigators. Cisplatin plus gemcitabine versus gemcitabine for biliary tract cancer. N Engl J Med 2010;362:1273-81.
9. Ohaegbulam KC, Koethe Y, Fung A, et al. The multidisciplinary management of cholangiocarcinoma. Cancer 2023;129:184-214.
10. Rizvi S, Gores GJ. Pathogenesis, diagnosis, and management of cholangiocarcinoma. Gastroenterology 2013;145:1215-29.
11. Nakamura H, Arai Y, Totoki Y, et al. Genomic spectra of biliary tract cancer. Nat Genet 2015;47:1003-10.
12. Verlingue L, Hollebecque A, Boige V, Ducreux M, Malka D, Ferté C. Matching genomic molecular aberrations with molecular targeted agents: Are biliary tract cancers an ideal playground? Eur J Cancer 2017;81:161-73.
14. Lorenzini S, Bird TG, Boulter L, et al. Characterisation of a stereotypical cellular and extracellular adult liver progenitor cell niche in rodents and diseased human liver. Gut 2010;59:645-54.
15. Sirica AE, Gores GJ. Desmoplastic stroma and cholangiocarcinoma: clinical implications and therapeutic targeting. Hepatology 2014;59:2397-402.
16. Brivio S, Cadamuro M, Strazzabosco M, Fabris L. Tumor reactive stroma in cholangiocarcinoma: the fuel behind cancer aggressiveness. World J Hepatol 2017;9:455-68.
18. Muz B, de la Puente P, Azab F, Azab AK. The role of hypoxia in cancer progression, angiogenesis, metastasis, and resistance to therapy. Hypoxia 2015;3:83-92.
19. Morine Y, Shimada M, Utsunomiya T, et al. Hypoxia inducible factor expression in intrahepatic cholangiocarcinoma. Hepatogastroenterology 2011;58:1439-44.
20. Fabris L, Cadamuro M, Fiorotto R, et al. Effects of angiogenic factor overexpression by human and rodent cholangiocytes in polycystic liver diseases. Hepatology 2006;43:1001-12.
21. Cadamuro M, Stecca T, Brivio S, et al. The deleterious interplay between tumor epithelia and stroma in cholangiocarcinoma. Biochim Biophys Acta Mol Basis Dis 2018;1864:1435-43.
22. Akkoc Y, Peker N, Akcay A, Gozuacik D. Autophagy and Cancer Dormancy. Front Oncol 2021;11:627023.
23. Balayan V, Guddati AK. Tumor dormancy: biologic and therapeutic implications. World J Oncol 2022;13:8-19.
24. Baeriswyl V, Christofori G. The angiogenic switch in carcinogenesis. Semin Cancer Biol 2009;19:329-37.
27. Karakaş D, Cevatemre B, Ulukaya E. Cancer stem cells: emerging actors in both basic and clinical cancer research. Turk J Biol 2014;38:829-38.
28. Sirica AE. The role of cancer-associated myofibroblasts in intrahepatic cholangiocarcinoma. Nat Rev Gastroenterol Hepatol 2011;9:44-54.
29. Leyva-Illades D, McMillin M, Quinn M, Demorrow S. Cholangiocarcinoma pathogenesis: role of the tumor microenvironment. Transl Gastrointest Cancer 2012; 1:71-80.
30. Hasita H, Komohara Y, Okabe H, et al. Significance of alternatively activated macrophages in patients with intrahepatic cholangiocarcinoma. Cancer Sci 2010;101:1913-9.
31. Jin MZ, Jin WL. The updated landscape of tumor microenvironment and drug repurposing. Signal Transduct Target Ther 2020;5:166.
32. Kawahara N, Ono M, Taguchi K, et al. Enhanced expression of thrombospondin-1 and hypovascularity in human cholangiocarcinoma. Hepatology 1998;28:1512-7.
33. Roy S, Glaser S, Chakraborty S. Inflammation and progression of cholangiocarcinoma: role of angiogenic and lymphangiogenic mechanisms. Front Med 2019;6:293.
34. Zhu AX, Meyerhardt JA, Blaszkowsky LS, et al. Efficacy and safety of gemcitabine, oxaliplatin, and bevacizumab in advanced biliary-tract cancers and correlation of changes in 18-fluorodeoxyglucose PET with clinical outcome: a phase 2 study. Lancet Oncol 2010;11:48-54.
35. Lubner SJ, Mahoney MR, Kolesar JL, et al. Report of a multicenter phase II trial testing a combination of biweekly bevacizumab and daily erlotinib in patients with unresectable biliary cancer: a phase II Consortium study. J Clin Oncol 2010;28:3491-7.
36. Guion-Dusserre JF, Lorgis V, Vincent J, Bengrine L, Ghiringhelli F. FOLFIRI plus bevacizumab as a second-line therapy for metastatic intrahepatic cholangiocarcinoma. World J Gastroenterol 2015;21:2096-101.
37. Iyer RV, Pokuri VK, Groman A, et al. A multicenter phase II study of gemcitabine, capecitabine, and bevacizumab for locally advanced or metastatic biliary tract cancer. Am J Clin Oncol 2018;41:649-55.
38. Bréchon M, Dior M, Dréanic J, et al. Addition of an antiangiogenic therapy, bevacizumab, to gemcitabine plus oxaliplatin improves survival in advanced biliary tract cancers. Invest New Drugs 2018;36:156-62.
39. Larsen FO, Markussen A, Diness LV, Nielsen D. Efficacy and safety of capecitabine, irinotecan, gemcitabine, and bevacizumab as second-line treatment in advanced biliary tract cancer: a phase II study. Oncology 2018;94:19-24.
40. Pei SN, Liao CK, Chen YS, et al. A novel combination of bevacizumab with chemotherapy improves therapeutic effects for advanced biliary tract cancer: a retrospective, observational study. Cancers 2021;13:3831.
41. Bengala C, Bertolini F, Malavasi N, et al. Sorafenib in patients with advanced biliary tract carcinoma: a phase II trial. Br J Cancer 2010;102:68-72.
42. Yi JH, Thongprasert S, Lee J, et al. A phase II study of sunitinib as a second-line treatment in advanced biliary tract carcinoma: a multicentre, multinational study. Eur J Cancer 2012;48:196-201.
43. El-Khoueiry AB, Rankin CJ, Ben-Josef E, et al. SWOG 0514: a phase II study of sorafenib in patients with unresectable or metastatic gallbladder carcinoma and cholangiocarcinoma. Invest New Drugs 2012;30:1646-51.
44. Lee JK, Capanu M, O'Reilly EM, et al. A phase II study of gemcitabine and cisplatin plus sorafenib in patients with advanced biliary adenocarcinomas. Br J Cancer 2013;109:915-9.
45. El-Khoueiry AB, Rankin C, Siegel AB, et al. S0941: a phase 2 SWOG study of sorafenib and erlotinib in patients with advanced gallbladder carcinoma or cholangiocarcinoma. Br J Cancer 2014;110:882-7.
46. Moehler M, Maderer A, Schimanski C, et al. Working Group of Internal Oncology. Gemcitabine plus sorafenib versus gemcitabine alone in advanced biliary tract cancer: a double-blind placebo-controlled multicentre phase II AIO study with biomarker and serum programme. Eur J Cancer 2014;50:3125-35.
47. Santoro A, Gebbia V, Pressiani T, et al. A randomized, multicenter, phase II study of vandetanib monotherapy versus vandetanib in combination with gemcitabine versus gemcitabine plus placebo in subjects with advanced biliary tract cancer: the VanGogh study. Ann Oncol 2015;26:542-7.
48. Valle JW, Wasan H, Lopes A, et al. Cediranib or placebo in combination with cisplatin and gemcitabine chemotherapy for patients with advanced biliary tract cancer (ABC-03): a randomised phase 2 trial. Lancet Oncol 2015;16:967-78.
49. Dreyer C, Sablin MP, Bouattour M, et al. Disease control with sunitinib in advanced intrahepatic cholangiocarcinoma resistant to gemcitabine-oxaliplatin chemotherapy. World J Hepatol 2015;7:910-5.
50. Kessler ER, Eckhardt SG, Pitts TM, et al. Phase I trial of vandetanib in combination with gemcitabine and capecitabine in patients with advanced solid tumors with an expanded cohort in pancreatic and biliary cancers. Invest New Drugs 2016;34:176-83.
51. Shroff RT, Yarchoan M, O'Connor A, et al. The oral VEGF receptor tyrosine kinase inhibitor pazopanib in combination with the MEK inhibitor trametinib in advanced cholangiocarcinoma. Br J Cancer 2017;116:1402-7.
52. Sun W, Patel A, Normolle D, et al. A phase 2 trial of regorafenib as a single agent in patients with chemotherapy-refractory, advanced, and metastatic biliary tract adenocarcinoma. Cancer 2019;125:902-9.
53. Kim RD, Sanoff HK, Poklepovic AS, et al. A multi-institutional phase 2 trial of regorafenib in refractory advanced biliary tract cancer. Cancer 2020;126:3464-70.
54. Cousin S, Cantarel C, Guegan JP, et al. Regorafenib-avelumab combination in patients with biliary tract cancer (REGOMUNE): a single-arm, open-label, phase II trial. Eur J Cancer 2022;162:161-9.
55. Ding X, Li G, Sun W, et al. Sintilimab combined with lenvatinib for advanced intrahepatic cholangiocarcinoma in second-line setting-a multi-center observational study. Front Oncol 2022;12:907055.
56. Zhu C, Li H, Yang X, et al. Efficacy, safety, and prognostic factors of PD-1 inhibitors combined with lenvatinib and Gemox chemotherapy as first-line treatment in advanced intrahepatic cholangiocarcinoma: a multicenter real-world study. Cancer Immunol Immunother 2023;72:2949-60.
57. Shi GM, Huang XY, Wu D, et al. Toripalimab combined with lenvatinib and GEMOX is a promising regimen as first-line treatment for advanced intrahepatic cholangiocarcinoma: a single-center, single-arm, phase 2 study. Signal Transduct Target Ther 2023;8:106.
58. Holmes DI, Zachary I. The vascular endothelial growth factor (VEGF) family: angiogenic factors in health and disease. Genome Biol 2005;6:209.
60. Rajabi P, Neshat A, Mokhtari M, Rajabi MA, Eftekhari M, Tavakoli P. The role of VEGF in melanoma progression. J Res Med Sci 2012;17:534-9.
61. De Paola F, Granato AM, Scarpi E, et al. Vascular endothelial growth factor and prognosis in patients with node-negative breast cancer. Int J Cancer 2002;98:228-33.
62. Niklińska W, Burzykowski T, Chyczewski L, Nikliński J. Expression of vascular endothelial growth factor (VEGF) in non-small cell lung cancer (NSCLC): association with p53 gene mutation and prognosis. Lung Cancer 2001;34 Suppl 2:S59-64.
63. Fabris L, Cadamuro M, Libbrecht L, et al. Epithelial expression of angiogenic growth factors modulate arterial vasculogenesis in human liver development. Hepatology 2008;47:719-28.
64. Mancino A, Mancino MG, Glaser SS, et al. Estrogens stimulate the proliferation of human cholangiocarcinoma by inducing the expression and secretion of vascular endothelial growth factor. Dig Liver Dis 2009;41:156-63.
65. Hida Y, Morita T, Fujita M, et al. Vascular endothelial growth factor expression is an independent negative predictor in extrahepatic biliary tract carcinomas. Anticancer Res 1999;19:2257-60.
66. Möbius C, Demuth C, Aigner T, et al. Evaluation of VEGF a expression and microvascular density as prognostic factors in extrahepatic cholangiocarcinoma. Eur J Surg Oncol 2007;33:1025-9.
67. Yoshikawa D, Ojima H, Iwasaki M, et al. Clinicopathological and prognostic significance of EGFR, VEGF, and HER2 expression in cholangiocarcinoma. Br J Cancer 2008;98:418-25.
68. Cai C, Wang X, Fu Q, Chen A. The VEGF expression associated with prognosis in patients with intrahepatic cholangiocarcinoma: a systematic review and meta-analysis. World J Surg Oncol 2022;20:40.
69. Calastri MCJ, Ferreira RF, Tenani GD, et al. Investigating VEGF. miR-145-3p, and miR-101-3p expression in patients with cholangiocarcinoma. Asian Pac J Cancer Prev 2022;23:2233-41.
70. Luo X, Jia W, Huang Z, et al. Effectiveness and safety of sorafenib in the treatment of unresectable and advanced intrahepatic cholangiocarcinoma: a pilot study. Oncotarget 2017;8:17246-57.
71. Huang MP, Gu SZ, Huang B, et al. Apatinib inhibits angiogenesis in intrahepatic cholangiocarcinoma by regulating the vascular endothelial growth factor receptor-2/signal transducer and activator of transcription factor 3/hypoxia inducible factor 1 subunit alpha signaling axis. Pharmacology 2021;106:509-19.
72. Peng H, Zhang Q, Li J, et al. Apatinib inhibits VEGF signaling and promotes apoptosis in intrahepatic cholangiocarcinoma. Oncotarget 2016;7:17220-9.
73. Lin G, Wang B, Wu X, et al. Efficacy and safety of apatinib treatment for patients with advanced intrahepatic cholangiocarcinoma. Cancer Manag Res 2020;12:11523-6.
75. Zadeh G, Koushan K, Pillo L, Shannon P, Guha A. Role of ang1 and its interaction with VEGF-A in astrocytomas. J Neuropathol Exp Neurol 2004;63:978-89.
76. Moon WS, Rhyu KH, Kang MJ, et al. Overexpression of VEGF and angiopoietin 2: a key to high vascularity of hepatocellular carcinoma? Mod Pathol 2003;16:552-7.
77. Tang D, Nagano H, Yamamoto H, et al. Angiogenesis in cholangiocellular carcinoma: Expression of vascular endothelial growth factor, angiopoietin-1/2, thrombospondin-1 and clinicopathological significance. Oncol Rep 2006;15:525-32.
78. Voigtländer T, David S, Thamm K, et al. Angiopoietin-2 and biliary diseases: elevated serum, but not bile levels are associated with cholangiocarcinoma. PLoS One 2014;9:e97046.
79. Kimawaha P, Jusakul A, Junsawang P, et al. Establishment of a potential serum biomarker panel for the diagnosis and prognosis of cholangiocarcinoma using decision tree algorithms. Diagnostics 2021;11:589.
80. Atanasov G, Hau HM, Dietel C, et al. Prognostic significance of TIE2-expressing monocytes in hilar cholangiocarcinoma. J Surg Oncol 2016;114:91-8.
81. Atanasov G, Dietel C, Feldbrügge L, et al. Angiogenic miRNAs, the angiopoietin axis and related TIE2-expressing monocytes affect outcomes in cholangiocarcinoma. Oncotarget 2018;9:29921-33.
82. Adams JC. Thrombospondins: multifunctional regulators of cell interactions. Annu Rev Cell Dev Biol 2001;17:25-51.
83. Aishima S, Taguchi K, Sugimachi K, et al. The role of thymidine phosphorylase and thrombospondin-1 in angiogenesis and progression of intrahepatic cholangiocarcinoma. Int J Surg Pathol 2002;10:47-56.
84. Carpino G, Cardinale V, Di Giamberardino A, et al. Thrombospondin 1 and 2 along with PEDF inhibit angiogenesis and promote lymphangiogenesis in intrahepatic cholangiocarcinoma. J Hepatol 2021;75:1377-86.
85. Poon RT, Chung KK, Cheung ST, et al. Clinical significance of thrombospondin 1 expression in hepatocellular carcinoma. Clin Cancer Res 2004;10:4150-7.
86. Ding DY, Gan XJ, Zhang JN, et al. Serum thrombospondin-1 serves as a novel biomarker and agonist of gemcitabine-based chemotherapy in intrahepatic cholangiocarcinoma. Br J Cancer 2023;128:907-17.
88. Nelson J, Bagnato A, Battistini B, Nisen P. The endothelin axis: emerging role in cancer. Nat Rev Cancer 2003;3:110-6.
89. Eberl L, Bovey R, Juillerat-Jeanneret L. Endothelin-receptor antagonists are proapoptotic and antiproliferative in human colon cancer cells. Br J Cancer 2003;88:788-95.
90. Shen W, Xi H, Li C, et al. Endothelin-A receptor in gastric cancer and enhanced antitumor activity of trastuzumab in combination with the endothelin-A receptor antagonist ZD4054. Ann N Y Acad Sci 2019;1448:30-41.
91. Ahn HM, Kim DG, Kim YJ. Blockade of endothelin receptor A enhances the therapeutic efficacy of gemcitabine in pancreatic cancer cells. Biochem Biophys Res Commun 2020;527:568-73.
92. Rosanò L, Spinella F, Bagnato A. Endothelin 1 in cancer: biological implications and therapeutic opportunities. Nat Rev Cancer 2013;13:637-51.
93. Salani D, Di Castro V, Nicotra MR, et al. Role of endothelin-1 in neovascularization of ovarian carcinoma. Am J Pathol 2000;157:1537-47.
94. Fava G, Demorrow S, Gaudio E, et al. Endothelin inhibits cholangiocarcinoma growth by a decrease in the vascular endothelial growth factor expression. Liver Int 2009;29:1031-42.
Cite This Article
How to Cite
Download Citation
Export Citation File:
Type of Import
Tips on Downloading Citation
Citation Manager File Format
Type of Import
Direct Import: When the Direct Import option is selected (the default state), a dialogue box will give you the option to Save or Open the downloaded citation data. Choosing Open will either launch your citation manager or give you a choice of applications with which to use the metadata. The Save option saves the file locally for later use.
Indirect Import: When the Indirect Import option is selected, the metadata is displayed and may be copied and pasted as needed.
About This Article
Special Issue
Copyright
Data & Comments
Data
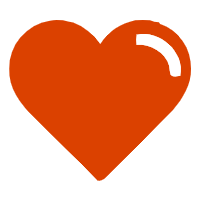
Comments
Comments must be written in English. Spam, offensive content, impersonation, and private information will not be permitted. If any comment is reported and identified as inappropriate content by OAE staff, the comment will be removed without notice. If you have any queries or need any help, please contact us at [email protected].