Assessment of background plastics contamination from human respiratory tract sample collection vessels
Abstract
Humans are increasingly exposed to airborne plastic particles due to their widespread contamination of all parts of the environment, yet the extent of inhalation exposure is still widely unknown. Bronchoalveolar lavage (BAL) and Transbronchial needle aspiration (TBNA) are two methodologies that are routinely used to detect micron or sub-micron foreign particles lodged in the human respiratory system. However, disposable plastic sampling and collection equipment is commonly used in these procedures. In this study, the potential of sample contamination due to the migration of particles from a range of containers commonly used in BAL and TBNA sample collection was investigated. PE and PVC were detected at the highest concentrations (1.5-5.6 and 1.2-8.0 g/sample, respectively) and likely originated from the container (PE) or background contamination from the manufacture/shipping process (PVC). The results demonstrated that samples collected with BAL equipment could be confidently used for the quantification of PP, PMMA, PC and PVC. Samples collected with the TBNA equipment could be confidently used for quantification of PMMA and PC, but with further assessment of trace levels from certain pieces of equipment, PP, PET, and PS could also be quantified. The results of this study demonstrate that there is potential to analyze samples collected in plastic hospital collection equipment for certain polymers. As a recommendation, background contamination from materials to be used in sample collection should be assessed before sample collection, and if consistent, then there is the potential for the analysis of a range of target plastics, with the addition of blank subtraction.
Keywords
INTRODUCTION
The issue of plastic pollution in the environment is well documented, with a continually increasing number of studies investigating occurrence and exposure. While it is known that humans are exposed to micro and nanosized plastics through ingestion and inhalation[1], there is still very little human biomonitoring data available, i.e., reporting concentrations within human matrices. One of the biggest challenges with these studies is background contamination (i.e., contamination from the surrounding environment during sampling, collection, and processing and/or leaching from sample collection equipment), including assessing contamination and implementing appropriate mitigation controls[2,3]. This is particularly relevant for human biomonitoring studies where disposable plastic collection materials are a staple for hospital sample collection methods and for storing archive-collected samples.
Two common hospital procedures that provide important information on inhalation exposure to various pollutants and associated respiratory diseases are Bronchoalveolar lavage (BAL) and Transbronchial needle aspiration (TBNA). BAL is a diagnostic tool that samples cells and proteins from the lower respiratory tract and can facilitate the diagnosis of interstitial lung diseases[4]. The process involves inserting a bronchoscope, introducing fluid (usually saline) and then aspirating the fluid collected for analysis[5]. This can provide information on respiratory secretions including leukocytes, other cellular components such as invading bacteria, and acellular components such as cytokines, viral particles, and microbial signatures (e.g., proteins and nucleic acids)[4]. TBNA is also a common respiratory diagnosis technique employed during a bronchoscopy and used for the diagnosis and staging of lung and other neoplasms in addition to a variety of inflammatory diseases[6,7]. The samples are collected through needle aspirations of pulmonary lymph nodes or directly from nodules and mass lesions.
Both BAL and TBNA are routinely used to detect micron or even sub-micron-sized foreign particles within the respiratory tract, including silica particles and asbestos fibres[4,8,9]. Therefore, there is the potential for these samples also to collect micro or nanosized plastics that have entered human respiratory pathways. Disposable plastics are used throughout these surgical procedures, which present opportunities for plastic contamination of the collected samples due to the migration or leaching of particles from the containers into the samples. A previous study has demonstrated that metal particles can be released from needles during the collection of TBNA samples[10] and that opening plastic materials, in general, can generate a significant number of plastic particles[11], but to the author’s knowledge, the influence of using plastic equipment during routine procedures has not previously been reported.
The aim of the current study was to assess and quantify background contamination from common disposable plastic equipment used in BAL and TBNA procedures. Samples were evaluated by simulating a sample collection with ultrapure water followed by an analysis of captured particles > 1µm with pyrolysis-gas chromatography-mass spectrometry (Pyr-GC/MS)[12-14] to quantify the seven most commonly reported high production-polymers: polyethylene (PE), polypropylene (PP), polystyrene (PS), polymethyl methacrylate (PMMA), polyvinylchloride (PVC), polyethylene terephthalate (PET) and polycarbonate (PC)[15,16]. Finally, the study aimed to determine if samples collected in this manner could be used for the identification and quantification of polymers and, if so, which ones.
EXPERIMENTAL
Materials and reagents
Liquid chromatography grade dichloromethane (DCM) was purchased in glass bottles from Merck (Darmstadt, Germany). Ultrapure water was purified with a Milli-Q system (Millipore, Bedford, USA) and additionally filtered through a 47 mm, 0.7 µm pore size Whatman glass fiber filter (ThermoFisher Scientific, Waltham, MA) prior to use. Analytical standards of polymethyl methacrylate (PMMA), polyvinyl chloride (PVC) and polystyrene (PS) were purchased from Sigma-Aldrich (St. Louis, MO, USA) and polyethylene (PE) (low-density polyethylene) from Alfa Aesar (ThermoFisher Scientific, Waltham, MA). Polycarbonate (PC), polyethylene terephthalate (PET) and deuterated polystyrene internal standard (d5-PS) were purchased from Polymer Source, Inc. (Dorval, Canada). Polypropylene (PP) was donated by a plastic manufacturer from Melbourne, Australia (LyondellBasell, VIC). All the reagents or solvents used in this study were purchased in glass bottles and were used as obtained without further purification.
Sample selection and distribution
Nine different types of disposable plastic equipment used in common BAL and TBNA collections were donated by a hospital in Brisbane, Australia, for use in experiments. The equipment consisted of three types of syringes (10, 30 and 60 mL, SY1, SY2, SY3, respectively), two types of Falcon tubes sourced from different manufacturers (10 and 50 mL, FT1, FT2), replicates of one type of small container (SMC), one type of saline solution container (500 mL, SSC), one type of large container (LG), one type of specimen trap (70 mL, SPT), one type of suction tube (1.5 m, ST), and one type of specimen container (250 mL, SPC), Supplementary Figure 1. The individual pieces used for each procedure are listed in Table 1 and Table 2, with SPC, SY2, SY3, SCC, ST and SPT used for BAL and SPC, LG, SMC, SY1, SY2, FT1 and FT2 used for the TBNA procedure.
Composition and polymer type of the plastic materials
Plastic components | |||||
ID | Lid | Container material | Plunger | Others | |
Specimen container | SPC | PE | PP | ||
Saline solution container | SSC | PP | PP | PE/PP seal under the lid | |
Suction tube | ST | PVC + benzoic copolymer | |||
Large container | LG | PE | PP | ||
Small container | SMC | PE | PP | ||
Specimen trap | SPT | PP | PS | PE/PS tubing | |
Syringes (all) | SY1-3 | PP | PE/PET/PVC/PS | ||
Falcon tubes (all) | FT1-2, | PE | PP |
Mass concentrations of plastics > 1 µm in size detected and quantified (µg/injection) in the plastic material types. The average process blank concentration and calculated LOD for each polymer are also listed (µg/injection), with further details on the blanks listed in Supplementary Table 3
Summary | PE | PP | PET | PS | PMMA | PVC | PC | |
BAL | Specimen container 250 mL (SPC) | < 2.1 | < 0.1 | < 0.1 | < 0.05 | < 0.01 | < 1.6 | < 0.002 |
60 mL syringe #1 (SY3) | < 2.1 | < 0.1 | < 0.1 | < 0.05 | < 0.01 | < 1.6 | < 0.002 | |
60 mL syringe #2 (SY3) | < 2.1 | < 0.1 | 0.10 | 0.27 | < 0.01 | < 1.6 | < 0.002 | |
Saline container (SCC) | < 2.1 | < 0.1 | < 0.1 | < 0.05 | < 0.01 | < 1.6 | < 0.002 | |
Suction tube 1.5 m (ST) | < 2.1 | < 0.1 | < 0.1 | < 0.05 | < 0.01 | < 1.6 | < 0.002 | |
Specimen trap 70 mL (SPT) | < 2.1 | < 0.1 | < 0.1 | < 0.05 | < 0.01 | < 1.6 | < 0.002 | |
30 mL syringe #1 (SY2) | 2.83 | < 0.1 | < 0.1 | < 0.05 | < 0.01 | < 1.6 | < 0.002 | |
30 mL syringe #2 (SY2) | < 2.1 | < 0.1 | < 0.1 | < 0.05 | < 0.01 | < 1.6 | < 0.002 | |
TBNA + other | Specimen container 250 mL #1 (SPC) | < 2.1 | < 0.1 | 0.17 | 0.09 | < 0.01 | < 1.6 | < 0.002 |
Specimen container 250 mL #2 (SPC) | 2.69 | < 0.1 | < 0.1 | 0.09 | < 0.01 | < 1.6 | < 0.002 | |
Large container #2 (LG) | 3.03 | 0.13 | < 0.1 | < 0.05 | < 0.01 | 6.83 | < 0.002 | |
Large container #1 (LG) | 2.98 | < 0.1 | < 0.1 | < 0.05 | < 0.01 | 8.04 | < 0.002 | |
Small container #1 (SMC) | 5.55 | < 0.1 | < 0.1 | < 0.05 | < 0.01 | 1.15 | < 0.002 | |
Small container #2 (SMC) | 1.51 | 0.16 | < 0.1 | < 0.05 | < 0.01 | 3.52 | < 0.002 | |
Small container #3 (SMC) | 2.72 | < 0.1 | < 0.1 | < 0.05 | < 0.01 | < 1.6 | < 0.002 | |
10 mL syringe (SY1) | < 2.1 | < 0.1 | < 0.1 | < 0.05 | < 0.01 | < 1.6 | < 0.002 | |
30 mL syringe (SY2) | < 2.1 | < 0.1 | < 0.1 | < 0.05 | < 0.01 | < 1.6 | < 0.002 | |
50 mL Falcon tube #1 (FT2) | < 2.1 | < 0.1 | < 0.1 | < 0.05 | < 0.01 | < 1.6 | < 0.002 | |
50 mL Falcon tube #2 (FT2) | < 2.1 | < 0.1 | < 0.1 | < 0.05 | < 0.01 | < 1.6 | < 0.002 | |
10 mL Falcon tube #1 (FT1) | 13 | < 0.1 | < 0.1 | < 0.05 | < 0.01 | < 1.6 | < 0.002 | |
10 mL Falcon tube #2 (FT1) | 16 | < 0.1 | < 0.1 | < 0.05 | < 0.01 | < 1.6 | < 0.002 | |
Average Blank Concentration | 1.1 | < 0.1 | < 0.1 | < 0.05 | < 0.01 | 0.07 | 0.001 | |
LOD | 2.1 | < 0.1 | < 0.1 | < 0.05 | < 0.01 | 1.6 | 0.002 |
Measures for reducing contamination during sample processing
Special care and precautions were taken throughout the experiment and analysis to reduce sample contamination from the laboratory environment. 100% cotton laboratory coats were worn during all steps of sample processing and analysis including sample handling. Glass or metal equipment was used whenever possible during laboratory procedures (i.e., application of plastic tools was omitted where possible). The experiments were performed in a fume-hood to minimize contamination by airborne plastics, with samples and materials covered with aluminum foil, glass lids, or stainless-steel lids when not in use, being processed or during storage. All working areas were wiped down with 70% ethanol prior to starting of experiments. All laboratory glassware and equipment (e.g., glass beakers, steel tweezers, etc.) were successively cleaned in a dishwasher, muffle furnaced at 500 °C, then rinsed thoroughly three times with Milli-Q water followed by DCM prior to use. Prior to the Pyr-GC/MS analysis, system cleans (no pyrolysis cups) were injected to demonstrate the absence of plastic contamination in the Pyr-GC/MS system, and plastic was not detected in these injections. All pyrolysis cups used in this study were new and washed in DCM before use.
Sample processing and study design
To simulate sample collection volumes used in bronchoalveolar lavage (BAL) sampling, Baughman[17] was used as a reference, where it was reported that at least 120 mL must be instilled and at least 50% of the fluid aspirated for an acceptable BAL procedure. Therefore, in these experiments, a sample volume of 60 mL of Milli-Q water was used for rinsing each piece of sampling equipment. The exception was the syringes, where the total volume of the syringe was sampled (e.g., a 10 mL syringe was washed by drawing up 10 mL of Milli-Q water). For consistency and comparability of results, the same volume (60 mL) was used for the transbronchial needle aspiration (TBNA) sampling equipment. The samples were filled with the set volume of Milli-Q and vortexed for 30 seconds; then, the sample was collected into a precleaned (DCM) glass jar. The syringes were filled and then inverted three times to mix before dispensing the sample into a precleaned glass jar.
The collected samples were filtered under vacuum through a 13 mm, 1.0 µm pore size Whatman glass fiber filter (ThermoFisher Scientific, Waltham, MA) to capture plastic particles > 1 µm. The glass jars were rinsed 3 times with filtered Milli-Q water to make sure all adhered particles were transferred. The filters were rolled up and loaded into an 80 µL pyrolysis cup (Eco-Cup LF, Frontier Labs, Japan), covered with aluminum foil and placed on a heating plate at 40 °C for 2 hours to remove residual water. All cups were then spiked with 40 µg of d5-PS internal standard prior to analysis. The cups were loaded onto an autosampler of a Pyr-GC/MS system and analyzed for polymer mass concentrations following previously reported methods[13].
The plastic containers themselves were also sampled to identify their polymer content. Small (~1 mg) slivers of each container, lid, plunger in the syringes and tubing of the specimen trap were collected with a precleaned (DCM) scalpel in duplicate subsamples. The subsamples were added to pyrolysis cups, spiked with 40 µg of d5-PS internal standard, and analyzed with Pyr-GC/MS for polymer identification.
Plastics analysis
The identification and quantification of the target plastics (PS, PC, PMMA, PP, PET, PE and PVC) were performed using the double-shot component of a Multi-Shot Micro-furnace Pyrolyzer (EGA/PY-3030D) equipped with an auto-shot sampler (AS-1020E) (Frontier Lab Ltd., Fukushima, Japan) and coupled to a GC/MS-QP2010-Plus (Shimadzu Corporation, Japan) as previously reported[13,16,18,19]. The first pyrolysis shot (ramped from 100° to 300 °C) of the double-shot method was used as a clean-up step to thermally remove interfering volatile and semi-volatile organic chemicals. The second pyrolysis shot (at 650 °C) was used to quantitatively measure the plastics[13]. Detailed double-shot Pyr-GC/MS parameters and the indicator ions used for the target plastics are given in Supplementary Tables 1 and 2, respectively, of the Supporting Information (SI).
All samples were collected in full scan mode (m/z 40 to 600) and the collected pyrograms were quantified for the essential 7 seven plastics and screened for additional dominant peaks that may indicate the presence of additional copolymers. Tentative polymer identifications from these additional peaks were conducted by matching spectra and retention times to reference spectra in Tsuge et al.[20].
QA/QC
Procedural blanks were collected with each batch of samples (n = 4) to assess potential contamination during sample preparation and processing and consisted of 60 mL of filtered Milli-Q run through the filtration apparatus, collecting polymers on 1 µm filters. These were treated and analyzed in the same batches as the samples and as described above. The results from all the laboratory blank samples are presented in Supplementary Table 3, with only PC, PVC and PE detected in blank samples.
Limits of detection (LODs) were calculated from concentrations in blanks as the mean concentration + 3 × standard deviation and ranged from 0.002 µg/injection for PC to 2.1 µg/injection for PE [Supplementary Table 3]. Where plastics were not detected in the blanks, LODs were calculated as the lowest concentration of a peak with a signal-to-noise ratio of 10:1. All reported data were blank subtracted.
RESULTS AND DISCUSSION
Composition of plastic collection containers
Results of the Pyr-GC/MS characterization of the plastic material types used in this study are presented in Table 1. PE was the dominant plastic type for the lids of most collection containers (SPC, LG, SMC, SPT, FTI), with PP dominating the plastic container bodies for SPC, LG, SMC, SPT, SY and FT [Table 1]. The suction tube (ST) was identified as PVC, with a benzoic copolymer peak also tentatively identified. The Specimen trap (SPT) was identified to have a PP lid and PS container with PE/PS tubing. All the Syringes (SY1-3) were identified to have a PP outer container and a combination of PE/PET/PVC/trace PS in their plungers [Table 1].
Polymer background in the plastic containers
For the BAL plastic-related materials, polymers were only quantified above detection limits in the syringe samples, with trace levels of PET and PS in 1 replicate of the 60 mL syringe (0.1 and 0.27 µg/sample, respectively), and PE in 1 replicate of the 30 mL syringe (2.8 µg/sample), Table 2. With the higher detection limits of PE (due to concentrations in blanks), any trace levels of PE were not observed in the other samples, and with the 1 higher detection in the syringe, it is concluded that these materials are unsuitable for analysis of PE. The trace levels of PET and PS in the 60 mL syringe sample may have originated from the plunger material or be trace contaminants from the production of the materials (e.g., atmospheric deposition during manufacture or transport). Further analysis of replicate 60 mL syringes would provide more information on the occurrence of these trace levels, reproducibility and whether a blank subtraction could be employed. The other polymers of PP, PMMA, PVC and PC were not detected in any sample, and there is potential to analyze samples collected in this manner for these polymers.
For the TBNA plastic-related materials, PE concentrations (1.5-16 µg/sample) were detected in 1 specimen container, the large containers, small containers and 10 mL Falcon tubes, Table 2, likely originating from the lids of these containers. Substantial PVC concentrations (1.2-8.0 µg/sample) were also detected in the large containers and small containers. Other than PE and PVC, trace levels of PET and PS were detected in the specimen containers (0.17 and 0.09 µg/sample, respectively), which again may be background contamination from the production of the items. PP was also observed in 1 large container and 1 small container in trace concentrations very close to detection limits (0.13-0.16 µg/sample). Further replicates would provide more information on if this is transfer from the container itself or also background contamination. It is interesting that PP was rarely observed in this study, even though most of the container bodies were PP.
It is uncertain where the elevated concentrations of PVC in these samples originated from as this polymer was not incorporated in the containers. The concentrations were relatively consistent between replicate containers suggesting these detections were not sampling artifacts but were collected contamination from inside the containers. To the authors' knowledge, TBNA samples have not been analyzed previously for polymers and the expected concentration range within samples is unknown, but due to the small particle size that would be expected to enter these airways (e.g., < 2.5 µm or PM2.5) and small aspirated sample mass, sample concentrations are expected to be close to detection limits and within the range of these background levels. Therefore, it is concluded that TBNA samples collected in this manner are not suitable for analysis of PVC; however, if concentrations in future samples are considerably higher than background concentrations, this could be reconsidered.
The PE concentrations were close to detection limits for all positive detections (1.5-5.6 µg/sample), except for the 10 mL Falcon tubes where elevated concentrations were observed in both replicates (13-16 µg/sample). The pyrolysis analysis of PE produces a range of long-chain alkanes, alkenes and dienes, which are monitored and quantified to calculate PE concentrations in the sample. However, other chemicals containing hydrocarbon chains (e.g., lipids, oils, surfactants) also break down into the same pyrolysis products producing significant interferences with the quantification of PE12. Monitoring the ratios of different pyrolysis products can provide an indicator if interference is present, as these interferences will form different ratios of certain pyrolysis products as compared to pure PE12. In this study, PE was quantified from the C10 alkene, with C12 and C14 alkenes and the C21 alkadiene also monitored to assess ratios [Supplementary Table 4].
In samples with positive detections, the ratios (0.5-2.4) were similar to a quality control (QC) spike (1.1-2.3), except for the 10 mL Falcon tubes where ratios were highly variable (0.4-55), Supplementary Table 4. This suggests that the elevated concentrations from the 10 mL Falcon tubes are likely an interference, e.g., a stearate or lubricant used in the manufacture of the Falcon tube12. Interferences with PE signals are not restricted to Pyr-GC/MS quantification methods, with previous studies indicating a similar PE interference using spectroscopy methods[21]. As such, in a similar manner to PVC, it was concluded that PE cannot be quantified in samples collected in this manner, particularly with this matrix interference present. From the results, the TBNA-acquired samples are potentially suitable for the analysis of PMMA and PC. If trace level background contamination is further assessed to be consistent, then there is also the potential for analysis of PP, PET, PS, PMMA and PC in these samples, with the addition of blank subtraction. This may also provide opportunities for analysis of archive samples collected before plastics minimized procedures could be adopted for the sampling process. However, this would require further background testing of the same equipment used during the archived sampling procedure.
CONCLUSIONS
The present study demonstrates that there is the potential to analyze samples collected in plastic hospital collection equipment for certain polymers. The assessment of BAL equipment indicated that PP, PMMA, PVC and PC could be quantified in these samples. The assessment of the TBNA equipment indicated that PMMA and PC could be quantified, but with further assessment of trace levels from some equipment, PP, PET, and PS could also be quantified. The polymers that pose the greatest risk and cannot be confidently quantified in the BAL samples are PS, PET, and PE, and in the TBNA samples are PE and PVC.
Future recommendations:
● → One should note that this study only reports plastic particles > 1 µm and there is the potential for nanosized particles to be present that were not assessed. Future studies should therefore assess contamination of plastic particles < 1 µm.
● → It should be recognized that this study focused on quantifying contamination from the containers themselves; however, strict sampling protocols and mitigation strategies are needed, particularly during sample collection, to minimize contamination from the surrounding environment during all steps.
● → Continual blank testing of the collection equipment investigated in this study is recommended to provide vital information on interferences (e.g., PE) as reported. If background concentrations from such equipment are consistent through blank testing, then there is the potential for background subtraction; however, this should be treated carefully.
DECLARATIONS
AcknowledgmentsThe Queensland Alliance for Environmental Health Sciences, The University of Queensland, gratefully acknowledges the financial support of Queensland Health, Australia. Cassandra Rauert’s salary is from the Minderoo Foundation, an independent not-for-profit philanthropic organization. The authors would like to thank Lyondellbasell, Melbourne (Australia) for providing reference PP plastic standards.
Authors’ contributionsMade substantial contributions towards the conception and design of the study, sample extractions or data analysis and interpretation: Okoffo ED, Rauert C, Boots R, Fielding D, Bashiradeh F, Singh M, Thomas KV
Availability of data and materialsNot applicable.
Financial support and sponsorshipCassandra Rauert’s salary is from the Minderoo Foundation, an independent not-for-profit philanthropic organization.
Conflicts of interestThis paper is an independent study. Neither Minderoo Foundation nor its benefactors had any influence over the conduct, the findings, or the recommendations of this paper.
Ethical approval and consent to participateNot applicable.
Consent for publicationNot applicable.
Copyright© The Author(s) 2023.
Supplementary MaterialsREFERENCES
2. Cowger W, Booth AM, Hamilton BM, et al. Reporting guidelines to increase the reproducibility and comparability of research on microplastics. Appl Spectrosc 2020;74:1066-77.
3. Wright SL, Gouin T, Koelmans AA, Scheuermann L. Development of screening criteria for microplastic particles in air and atmospheric deposition: critical review and applicability towards assessing human exposure. Micropl &Nanopl 2021;1.
4. Meyer KC. Bronchoalveolar lavage as a diagnostic tool. Semin Respir Crit Care Med 2007;28:546-60.
5. Baughman RP. Technical aspects of bronchoalveolar lavage: recommendations for a standard procedure. Semin Respir Crit Care Med 2007;28:475-85.
7. Dasgupta A, Mehta AC. Transbronchial needle aspiration. an underused diagnostic technique. Clin Chest Med 1999;20:39-51.
8. Forest V, Pourchez J, Guibert C, et al. Nano to micron-sized particle detection in patients' lungs and its pathological significance. Environ Sci : Nano 2019;6:1343-50.
9. Thanthitaweewat V, Leelayuwatanakul N, Sriprasart T. Silicosis: role of endobronchial ultrasound and transbronchial needle aspiration. Eur Res J 2020;56:3155.
10. Gounant V, Ninane V, Janson X, et al. Release of metal particles from needles used for transbronchial needle aspiration. Chest 2011;139:138-43.
11. Sobhani Z, Lei Y, Tang Y, et al. Microplastics generated when opening plastic packaging. Sci Rep 2020;10:4841.
12. Rauert C, Pan Y, Okoffo ED, O’brien JW, Thomas KV. Extraction and pyrolysis-GC-MS analysis of polyethylene in samples with medium to high lipid content. J Environ Expo Assess 2022;1:13.
13. Okoffo ED, Ribeiro F, O'Brien JW, et al. Identification and quantification of selected plastics in biosolids by pressurized liquid extraction combined with double-shot pyrolysis gas chromatography-mass spectrometry. Sci Total Environ 2020;715:136924.
14. Okoffo ED, Rauert C, Thomas KV. Mass quantification of microplastic at wastewater treatment plants by pyrolysis-gas chromatography-mass spectrometry. Sci Total Environ 2023;856:159251.
15. Okoffo ED, Donner E, McGrath SP, et al. Plastics in biosolids from 1950 to 2016: a function of global plastic production and consumption. Water Res 2021;201:117367.
16. Okoffo ED, Tscharke BJ, O'Brien JW, et al. Release of plastics to australian land from biosolids end-use. Environ Sci Technol 2020;54:15132-41.
17. Baughman, Robert P, et al. How I do bronchoalveolar lavage. Available from:
18. Okoffo ED, O'Brien S, O'Brien JW, et al. Does size matter? Sci Total Environ 2022;811:152382.
19. Ribeiro F, Okoffo ED, O'Brien JW, et al. Quantitative analysis of selected plastics in high-commercial-value australian seafood by pyrolysis gas chromatography mass spectrometry. Environ Sci Technol 2020;54:9408-17.
20. Tsuge S, Ohtani H, Watanabe C, et al. Pyrolysis-GC/MS data book of synthetic polymers: pyrograms, thermograms and MS of pyrolyzates.
Cite This Article

How to Cite
Download Citation
Export Citation File:
Type of Import
Tips on Downloading Citation
Citation Manager File Format
Type of Import
Direct Import: When the Direct Import option is selected (the default state), a dialogue box will give you the option to Save or Open the downloaded citation data. Choosing Open will either launch your citation manager or give you a choice of applications with which to use the metadata. The Save option saves the file locally for later use.
Indirect Import: When the Indirect Import option is selected, the metadata is displayed and may be copied and pasted as needed.
About This Article
Copyright
Data & Comments
Data
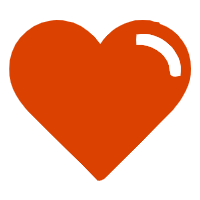
Comments
Comments must be written in English. Spam, offensive content, impersonation, and private information will not be permitted. If any comment is reported and identified as inappropriate content by OAE staff, the comment will be removed without notice. If you have any queries or need any help, please contact us at [email protected].