Recreational activities as a major source of microplastics in aquatic environments
Abstract
Microplastics (MPs) have been extensively studied in the marine environment in recent years, but their occurrence in recreational waters, and recreational activities as a source of MPs, have been less explored. In this study, we investigate the temporal variation of MPs in the Salt River, a natural surface waterway heavily used for recreation, and in community swimming pools in nearby Tempe, Arizona. Samples were processed using established methodologies and MP shapes and number concentrations were obtained by optical microscopy. The MP concentrations in samples of surface water collected during recreational activity ranged from 27,798 to
Keywords
INTRODUCTION
Environmental contamination by plastics has recently developed into an emerging concern, with microplastics (MPs) detection becoming ubiquitous in the environment[1-4]. Environmental MPs - typically defined as plastic particles measuring 5 mm or less in the longest dimension - can originate from the release of primary particles, intentionally engineered and in uniform shapes as microbeads and pre-production pellets[5-7]. More common are secondary MPs, which typically result from the environmental weathering of larger plastics through processes such as photodegradation or mechanical abrasion and tend to have more irregular shapes such as fibers and fragments[8-10].
Environmental research has predominantly focused on MPs in aquatic environments[8,11-20], while fewer studies have been conducted on their presence in atmospheric[21-29] and terrestrial ecosystems[30-35]. Within aqueous environments, most research has concentrated on marine ecosystems, with less than 4% of MP research addressing freshwaters[36]. Freshwater environments, including sources of drinking water, tend to be in closer proximity to the actual sources of MPs. Occurrence data show that the concentrations of MPs in surface waters and the corresponding sediments are higher in areas of high population density[37]. Mismanagement of plastic waste, sewage treatment, agricultural and road runoff, as well as commercial fishing, has been shown to be responsible for MP input into freshwater ecosystems, resulting in rivers being significant to investigate for synthetic MPs[38-40]. MPs, after being released into surface waters, can exhibit remarkable persistence, travel long distances, interact with aquatic organisms, and eventually end up in the oceans[41]. Beaches, often used for recreational activities, have been shown to be significantly contaminated by MPs[42-44]. However, few studies have investigated the occurrence and distribution of MPs in freshwater bodies used for recreational activities, such as lakes, rivers, and swimming pools.
MPs can occur in a variety of shapes, sizes, and colors, but morphologically, fibers appear most common in the gastrointestinal tracts of biota at any trophic level[45-47]. Despite the obvious environmental significance of fibers, shards, films and other fragments tend to be more commonly studied and the environmental sources of fibers remain poorly investigated. Some research reported that the weathering of one gram of polyester (PES) textiles resulted in the release of hundreds of thousands of fibers[48]. Laundry of synthetic fabric and swimwear has also been documented as a substantial source of fibrous MPs[49,50]. To date, there have been few studies that establish the importance of clothing as a source of MP fibers to the environment.
It has been shown that organisms in freshwater, across different habitats and trophic levels, ingest synthetic micro-particles[51]. Freshwater invertebrates such as gammarid amphipods play important roles in the breakdown of particulate organic matter and, therefore, can similarly digest MPs[52]. In addition to their direct toxic effects, these MPs can also act as vectors for other organic contaminants[53-55]. Therefore, investigating freshwater recreational activities as a source of contamination, particularly for synthetic materials such as fiber MPs, is important.
Swimming pools are another class of water system where MPs can be emitted by recreational water use and potentially accumulate. Pools are known to be hotspots for human exposure to pathogens[56] but might also be important in terms of exposure to MPs. Pathogens, including E. coli, Cryptosporidium, and Giardia, are present in such aquatic environments owing to human clustering[57]. MPs might act as a vector for pathogens to be ingested and hence infect humans, especially as smaller microfibers have been documented to evade water treatment processes owing to their size and density[58].
The present work investigates the occurrence of MPs in the Salt River in Arizona, which is used for both recreation and as a drinking water supply. Previous studies have investigated ecological indicators in the river and studied the occurrence of nanomaterials as well as pharmaceuticals and personal care products such as sunscreen[59-62]. A water sampling-based survey was conducted from a single location in the Salt River, in the presence and absence of recreational activities, to assess their impact on MP occurrence. To provide additional context for the occurrence of MPs in recreational waters, community swimming pools in apartment buildings in Tempe, Arizona, were also sampled. MP number concentration and morphology were analyzed using optical microscopy, while the chemistry of the MP was determined by micro-Raman spectroscopy.
EXPERIMENTAL
Sampling of MPs
The Salt River is a surface water system located mostly within the Tonto National Forest (AZ, USA), northeast of Phoenix, Arizona and includes four water reservoirs that form a series of lakes nearly 100 km long[63]. The river is a source of drinking water for the Phoenix metropolitan area and, in the summer months, is a popular destination for recreational activities. There are no commercial activities on the river, such as boating. The Goldfield Recreation area (Mesa, AZ) is a popular river access point (RAP) for recreational activities along the Lower Salt River, and witnesses substantial crowds during the months of June-September [Supplementary Figure 1].
Surface water samples (1 L) were collected at a single location in the Lower Salt River at the Goldfield Recreation area (33.5544° N, 111.6236° W). The samples were collected into clean, pre-fired (450 °C) amber glass bottles from 8 AM to 4 PM on Saturday, July 1st, 2023 (recreational season) and Friday, October 13th, 2023 (absence of recreational activities). The clean sampling bottles were rinsed three times with river water prior to gathering the actual sample, with each time discarding the rinse water downstream.
Triplicate samples were obtained at representative hours of the day (8:00, 12:00, and 16:00) in July to investigate the standard deviation of MP concentrations in water. However, triplicate samples were not obtained for each hourly time stamp due to limitations in logistics, and the relatively low standard deviation between replicates.
Sampling in apartment swimming pools (n = 7) in Tempe, Arizona, in July 2023 [Supplementary Figure 2] and October 2023 followed the same protocol. The selected community swimming pools were located in student apartment complexes in Tempe, Arizona, and were accessible for water sampling. Each pool was approximately 20’ × 40’ in size and was used by a substantial number of students during the summer in Arizona. Triplicates were analyzed for three pools with sampling IDs - SP2, SP5, and SP7.
All water samples were refrigerated during transport and stored at 4 °C in the dark until processing in the lab.
Sample processing
All aqueous samples were first passed through a sieve (8”-FH-SS-SS-US-#3-1/2, Hogentogler & Co.Inc., MD, USA) to remove any material larger than 5.6 mm in size. The sample bottles were triple rinsed with ultrapure water (> 18.4 MΩ·cm, Purelab Flex, IL, USA) to extract all MPs sticking to the glass surface of the bottles. The samples were then further processed using a methodology based on recommendations from the National Oceanic and Atmospheric Administration (NOAA) and adapted to water samples[64]. On brief, the first natural organic matter was removed by wet peroxide oxidation using 10 mL of an aqueous 0.05 M Fe (II) solution prepared from FeSO4·7H2O (Sigma Aldrich, MO, USA), followed by the addition of 10 mL of a 30% hydrogen peroxide (Sigma Aldrich, MO, USA) solution. After letting the resulting mixture rest for
Since MPs are ubiquitous in all environments, preventive measures must be adopted to minimize contamination of samples and overestimation of MP concentrations[22,65]. In the current study, a blank was obtained during sample handling and processing to evaluate the potential of cross-contamination. An ultrapure water sample (> 18.4 MΩ·cm, Purelab Flex, IL, USA) was transported to the field, was poured into a pre-cleaned and pre-baked (450 °C, overnight) amber glass bottle (similar to the sampling bottle) at the same sampling location, and transported to the lab similar to other water samples. Glassware used during all stages of sample handling, processing, and analyses was washed using a detergent and triple rinsed with ultrapure water (> 18.4 MΩ·cm, Purelab Flex, IL, USA) prior to use. Decontamination measures included subsequent baking of glassware (450 °C overnight). Potential contamination through the deposition of airborne MPs was minimized by covering the clean glassware and equipment used for sample processing and analysis. As MPs are widespread particles that could be found on any surface, lab bench and fume hood surfaces were cleaned with ultrapure water (> 18.4 MΩ·cm, Purelab Flex, IL, USA) and isopropyl alcohol (Fisher Scientific, MA, USA) before sample processing and analyses. The usage of glass materials is generally recommended when processing and analyzing MPs in samples, as plastic materials can release particles and result in contamination and overestimation of MPs[22]. The usage of plastics was minimized during sample handling and analysis, and the recovery rate of MPs from filters was determined to be 93% through matrix spikes with a known number of MPs in the size range 5-5,000 µm (see[25,33] for additional details).
Optical microscopy - shape and number concentrations
Filters were visually analyzed by a digital microscope (Leica DM6B-Z, Germany) (see[33] for details). In brief, the size range was 5-5,000 µm with the higher limit obtained through sieving and based on the typical definition of MPs, while the lower limit of 5 µm was due to the particle size detection limit of the microscope. In terms of shape, an object was considered a fiber when it looked cylindrical and presented an aspect ratio (length/diameter) ≥ 3. The sizes of the MPs were measured along their largest dimension using the software program ImageJ (version 1.5, National Institute of Health, USA, http://imagej.nih.gov/i). MPs were identified under the digital microscope based on criteria reported in literature, including the absence of cellular structures and fibers depicting uniform thickness along their entire length[33,66].
Chemical characterization using micro-Raman spectroscopy
Micro-Raman data were collected using a custom-built Raman spectrometer with a spatial resolution of
RESULTS AND DISCUSSION
Temporal variation of MPs in recreational surface waters of the Salt River
MPs were detected in all Salt River surface water samples and an increasing trend in concentrations was observed during the day (July 1st, 2023; Figure 1). The MP concentrations in the surface water samples during recreational activity varied from 27,798 MPs/m3 in the early morning, prior to recreational activities, to 222,391 MPs/m3 at 4 PM, at the peak of recreational activities, an increase of a factor of 8 [Figure 1]. The Salt River is accessible in this region for recreational activities from 9:00 daily from June-September. The observed variation in MP concentrations can be ascribed to the micro-particles released from human activities during recreational activities in the Salt River. The relative standard deviation between replicates obtained at 8:00, 12:00 and 16:00 during the sampling period in July [Supplementary Table 1] was 10%. MPs were also detected in all samples on October 13th, 2023, in the absence of recreational activities, showing a ubiquitous presence in the Salt River. The MP concentrations observed in October did not vary substantially over the course of the day, ranging from 17,347 to 21,905 MPs/m3 (the relative standard deviation of concentrations over the day was 11%). The number concentrations in October were similar to that in the surface water sample collected at 8:00 (no recreational activities) in July. MP baseline concentrations during the off-season in the absence of recreational activities are an order of magnitude lower than those observed on July 1st, 2023 [Figure 1]. The Salt River water flow data were retrieved from the closest monitoring station upstream, below Stewart Mountain Dam, from the United States Geological Survey (USGS). The average water flow rates in the river did not show substantial differences (898 ft3/s on July 1st and 827 ft3/s on October 13th). Therefore, it is possible to compare the MP concentrations in the Salt River in July and October, negating any dilution effects. Additionally, Mesa (AZ) did not experience rain or dust storms on either of the sampling days (July 1st, 2023 and October 13th, 2023). However, the influence of wind parameters (wind speed and wind direction) on MP concentrations in water on the two sampling days is unknown to us.
Figure 1. MP occurrence in the Salt River in the presence (July) and absence (October) of recreational activities. MP: Microplastic.
Filter blanks used to track contamination showed the presence of 1 to 5 MPs/filter with an average of
Placing the concentrations of MPs in the current study within the context of the existing literature is challenging due to differences in sampling and analytical protocols. Studies on MP occurrence in surface water, especially those used for recreational activities, are sparse. MP concentrations ranging from 0.44 to 9.7 MPs/m3 have been reported in the waters of Lakes Mead and Mohave, reservoirs along the Colorado River that see some recreational use and are also located in the arid southwestern USA[67]. Even though these basins are sparsely populated, the authors attribute the concentrations observed to the large number of visitors the locations witness[67]. The lower MP size limit in this study was 355 µm, while that of the current study was 5 µm. Even considering only the larger MPs in the Salt River (≥ 355 µm) in the presence of recreational activity at peak time (16:00), the concentrations are 4-5 orders of magnitude higher than those observed in Lakes Mead and Mohave (≥ 355 µm).
This could be attributed to the vast area covered by the two large Colorado River reservoirs (759 km2) compared to the Salt River, which would result in the dilution of overall MP concentrations in water[67]. Baldwin et al. also studied the St. Croix and Mississippi rivers in an earlier study, noting that both were impacted by recreational activities[68]. MP concentrations in these rivers (≥ 333 µm) were similar and of the same order of magnitude as those observed in Lakes Mead and Mohave (Colorado River), and could possibly be ascribed to similar lower size limits of interest. The MP concentrations in the Los Angeles River and Colorado Boulder Creek are 1-3 orders of magnitude lower than those in the Salt River during the absence of recreational activities[69,70]. This observation could be partially ascribed to the smaller lower size cut used in the current study (5 µm), compared to that reported in the aforementioned studies (1,000 µm).
A previous study on the Salt River by Venkatesan et al. studied the impact of recreational activities (tubing and swimming) on Ti-containing particles and sunscreen additives. Their findings were consistent with ours in that they saw a significant (80%) increase in the particle concentrations at peak activity time (16:00) over their initial baseline concentration[62]. The change was attributed to the increased recreational activity occurring upstream. Similarly, Chiu et al. showed a significant increase in oxybenzone (sunscreen) concentrations in Salt River waters during the day, consistent with an increase in recreational activities[59]. The overall human impact in the study region is documented by ecological studies, such as research that shows a decrease in the herpetofauna diversity along the Salt River, with the increase in urbanization[60]. The Salt River in Arizona is home to fish species including the Castostomus insignis, Gila robusta, Rhinichthys osculus, and the Catostomus clarkii, and the presence of MPs in the riverine ecosystem of interest in our current study can potentially reduce the feeding uptake of such aquatic organisms[71].
Morphological analysis showed that a majority of MPs in the Salt River were fibers
A previous study reporting soil MPs in the same arid region of Phoenix, Arizona, reported samples comprising large fractions of fibers[33], while a study on airborne MPs in nearby Tempe, Arizona, showed that a large fraction were fibers (≥ 82%)[25]. The present study of MPs in the riverine system shows that MPs were largely fibrous with relatively higher fractions than in these other local environmental systems.
Figure 2 shows the evolution of the size distributions of MPs in surface water samples from the Salt River during recreational activity over the course of 8 h. MPs were predominately found in the smallest size fraction (5-50 µm) that we were able to quantify, in water samples collected early in the day (8:00, 9:00, 10:00, and 12:00). Owing to their small size, MPs are ingested by a range of freshwater aquatic organisms that can eventually cause ecotoxicological and physiological changes[75]. Later in the day, MPs were predominately present in larger size classes. A Mann-Whitney Test (P ≤ 0.05) using OriginPro, Version 2023 (Origin Lab Corporation, Northampton, MA, USA) indicates that the size distribution of MPs at 8:00 is significantly different to that at 16:00 (P = 0.0064). As the day progressed, the river saw an increasing number of recreational users, which might have resuspended larger micro-particles previously deposited in sediments. It should be noted that the vertical dynamics of MP distribution within the sediments remain largely understudied and unknown. However, bioturbation can affect the distribution of MPs and litter in river sediments[76].
MPs in apartment community swimming pools
Figure 2 shows the range of MP concentrations observed in surface water samples from community swimming pools (n = 7) in Tempe, Arizona. The MP concentrations ranged from 59,160 to 254,574 MPs/m3 in October 2023 [Figure 3]. While MPs were detected in each pool, the concentrations varied significantly (the average relative standard deviation was 39%). The highest MP concentration was found at site SP5 and the lowest MP concentration was observed at sampling site SP4. Triplicate samples show a low measurement variability, smaller than the observed differences. The variability in MP concentrations between pools likely results from the difference in filter media used. Most swimming pools use sand, cartridge or diatomaceous earth (DE) filters to remove suspended solids as part of the water treatment process implemented to keep water physically attractive, clean, and safe[77]. MP concentrations in swimming pools in the summer (July) were of the same order of magnitude as those observed in October [Figure 3]. This could possibly be due to the rather low drainage frequency of swimming pools (3-7 years)[78]. MPs released from secondary degradation of fabrics could accumulate over time, and may possibly explain the relatively high concentrations observed in our study.
Figure 3. MP concentrations in swimming pools in July and October 2023. Each error bar represents the standard deviation of the mean obtained from three replicate measurements. SP1-SP7 are sampling location IDs of swimming pools. MP: Microplastic.
Comparing the results of the current study with existing research is important for contextualizing MP concentrations. However, to date, there are no reported studies investigating the presence of MPs in swimming pools. Overall, the concentrations in the swimming pools are comparable to those in the Salt River during recreational activity in July, and an order of magnitude higher than those in the absence of recreational activities in October. Sources of MPs in swimming pools may also be more limited compared to those in natural environments (Salt River). However, our chemical characterization results for MPs in the Salt River and swimming pools imply the release of synthetic fibers from fabrics. Results from a pilot study investigating MPs in Tempe Town Lake depict concentrations two orders of magnitude lower than those in swimming pools in Tempe [Figure 4]. The Tempe Town Lake is restricted for swimmers and the relatively high water flow in the lake (several hundred to thousand cubic feet per second depending on the season and episodic inflows), as opposed to no flow in swimming pools, could possibly explain the lower concentrations in the lake. The MP concentrations in the swimming pools in Tempe during July are comparable, and of similar magnitude to that in the Salt River during recreational activity [Figure 4]. The concentrations in the swimming pools are of similar magnitude to those in the Tres Rios wetland outflow, a constructed wetland that receives waste-water treatment effluent[79].
Fiber MPs were also the dominant morphology in the swimming pool survey, accounting for as much as 87% (in SP5). Fragments and spherical transparent beads [Supplementary Figure 4] were observed as the only other morphologies observed in these samples. Overall, the highest counts for MPs were observed in the 5-100 µm size fraction in the pool samples.
Chemical identification of MPs
Micro-Raman spectroscopy was used to assess the nature of the polymers in the MPs present in the Salt River (July and October) and swimming pools. A large diversity of polymers, including polyethylene (PE), polyvinyl chloride (PVC), polyamide (PA), PES, and polypropylene (PP), were observed and some MPs remained unidentified [Figure 5, Supplementary Figures 5 and 6]. Overall, a majority of the MPs identified were PA and PES. Swimwear is a significant source of microfibers to the environment and consists of interwoven nylon and PES fibers[50]. Raman characterization for MPs in water samples from July revealed that 4% to 40% of the MPs remained chemically unidentified. However, the fraction of unidentified MPs in the aquatic environments (Salt River) was lower than that found in atmospheric and soil environments in Phoenix, Arizona[25,33]. We showed in a previous study that environmental weathering processes can lead to surface modifications that render the MPs unidentifiable by micro-Raman spectroscopy[25]. The relatively lower fractions of unidentified MPs from this study area could possibly be an indication of the recent introduction of synthetic micro-particles from their sources to the aquatic environment (less time for environmental weathering).
Figure 5. Raman characterization of MPs of heavily used recreational surface water samples in the Salt River. MPs: Microplastics.
PA was present in 67% of the samples from the Salt River during recreational activities and tended to be the most abundant polymer (on average) in all our water samples [Figures 5 and 6]. At peak recreational activity (4 PM), PA accounted for 78% of all MPs. PES was also prominently present in the identified fraction of MPs in samples. These observations, combined with the morphology results, again suggest a high release of MP fibers from synthetic textiles[73]. PES is widely used in fabrics for clothing and is the most produced synthetic fiber on a global scale (~50% of the global fiber market)[9]. PA fibers rank second in the list of the most produced synthetic fibers[80]. The polymer distribution in the swimming pools in Tempe, Arizona [Supplementary Figure 6] was similar to the river samples[81]. A recent risk assessment study investigating the impact of different polymeric materials on aquatic organisms states that PA poses the greatest risk, followed by PET[58]. This suggests a non-negligible risk posed by these synthetic materials released to the Salt River in the current study.
CONCLUSION
MPs were ubiquitous in the heavily used recreational waters of the Salt River and local community swimming pools. MP concentrations in surface water samples in the Salt River showed an increase by 8 times at peak recreational activity time compared to baseline concentrations where no recreational activities occurred. MP concentrations depicted substantial variability between apartment swimming pools in Tempe, Arizona, at times as high as 254,574 MPs/m3. Fibers were the dominant shape of MPs in the tested surface waters, with a majority present in relatively smaller size categories (5-200 µm). Raman characterization for MPs in water samples revealed a diversity of polymers, but 4%-40% of the MPs remained chemically unidentified. The latter is likely the result of weathering processes-induced changes that render the polymers unidentifiable by micro Raman Spectroscopy. However, the fraction of unidentified MPs was smaller in the river during recreational activity compared to those in swimming pools, air and soil samples in the same arid region, indicating the recent introduction of the micro-particles to the riverine system. PA and PES were generally the most abundant polymers, suggesting a secondary origin from synthetic fabrics. Overall, the observations show that recreational activities can be a significant source of MPs in surface waters.
DECLARATIONS
Acknowledgments
We thank Gabrielle Cano, Christine Hubert, Jason Miech, Jesse Molar, and Amelia Stout for their assistance with field sampling for this project. Parts of this paper have appeared in Chandrakanthan K’s doctoral thesis.
Authors’ contributions
Conceptualization, methodology, data, curation, formal analysis, writing - original draft, Writing - review and editing: Chandrakanthan K
Supervision, writing - review and editing: Fraser MP
Writing - review and editing, validation, supervision, project administration: Herckes P
Availability of data and materials
The data that support the findings of this study are available from the corresponding author upon reasonable request.
Financial support and sponsorship
None.
Conflicts of interest
Herckes P is an Editorial Board member of the journal Water Emerging Contaminants & Nanoplastics, while the other authors have declared that there are no conflicts of interest.
Ethical approval and consent to participate
Not applicable.
Consent for publication
Not applicable.
Copyright
© The Author(s) 2024.
Supplementary Materials
REFERENCES
1. Geyer R, Jambeck JR, Law KL. Production, use, and fate of all plastics ever made. Sci Adv 2017;3:e1700782.
2. Zhao H, Zhou Y, Han Y, et al. Pollution status of microplastics in the freshwater environment of China: a mini review. Water Emerg Contam Nanoplastics 2022;1:5.
3. Bhatia SK, Kumar G, Yang YH. Understanding microplastic pollution: tracing the footprints and eco-friendly solutions. Sci Total Environ 2024;914:169926.
4. Pomata D, La Nasa J, Biale G, et al. Plastic breath: quantification of microplastics and polymer additives in airborne particles. Sci Total Environ 2024;932:173031.
5. Arthur C, Baker JE, Bamford HA. Proceedings of the International Research Workshop on the Occurrence, Effects and Fate of Microplastic Marine Debris, September 9-11, 2008. University of Washington Tacoma, Tacoma, WA, USA. 2009. Available from: https://repository.library.noaa.gov/view/noaa/2509. [Last accessed on 9 Sep 2024]
6. Turner A, Wallerstein C, Arnold R. Identification, origin and characteristics of bio-bead microplastics from beaches in western Europe. Sci Total Environ 2019;664:938-47.
7. Tran TV, Jalil AA, Nguyen TM, Nguyen TTT, Nabgan W, Nguyen DTC. A review on the occurrence, analytical methods, and impact of microplastics in the environment. Environ Toxicol Pharmacol 2023;102:104248.
8. Peller JR, Nelson CR, Babu BG, Iceman C, Kostelnik E. A review of microplastics in freshwater environments: locations, methods, and pollution loads. In: Ahuja S, Loganathan BG, editors. Contaminants in our water: identification and remediation methods. Washington: American Chemical Society; 2020. pp. 65-90.
9. Rebelein A, Int-Veen I, Kammann U, Scharsack JP. Microplastic fibers - underestimated threat to aquatic organisms? Sci Total Environ 2021;777:146045.
10. Ali AAM, Khalid AA, Razak NIA, et al. A review on the presence of microplastics in environmental matrices within Southeast Asia: elucidating risk information through an analysis of microplastic characteristics such as size, shape, and type. Water Emerg Contam Nanoplastics 2024;3:12.
12. Bordós G, Urbányi B, Micsinai A, et al. Identification of microplastics in fish ponds and natural freshwater environments of the Carpathian basin, Europe. Chemosphere 2019;216:110-6.
13. Yuan W, Liu X, Wang W, Di M, Wang J. Microplastic abundance, distribution and composition in water, sediments, and wild fish from Poyang Lake, China. Ecotoxicol Environ Saf 2019;170:180-7.
14. Li C, Busquets R, Campos LC. Assessment of microplastics in freshwater systems: a review. Sci Total Environ 2020;707:135578.
15. Xu S, Ma J, Ji R, Pan K, Miao AJ. Microplastics in aquatic environments: occurrence, accumulation, and biological effects. Sci Total Environ 2020;703:134699.
16. Abbasi S, Turner A. Sources, concentrations, distributions, fluxes and fate of microplastics in a hypersaline lake: Maharloo, south-west Iran. Sci Total Environ 2022;823:153721.
17. Esfandiari A, Abbasi S, Peely AB, et al. Distribution and transport of microplastics in groundwater (Shiraz aquifer, southwest Iran). Water Res 2022;220:118622.
18. Ginebreda A. Emerging contaminants and nanoplastics in the water environment: a matter of rising concern. Water Emerg Contam Nanoplastics 2022;1:1.
19. Picó Y, Manzoor I, Soursou V, Barceló D. Microplastics in water, from treatment process to drinking water: analytical methods and potential health effects. Water Emerg Contam Nanoplastics 2022;1:13.
20. Leterme SC, Tuuri EM, Drummond WJ, Jones R, Gascooke JR. Microplastics in urban freshwater streams in Adelaide, Australia: a source of plastic pollution in the Gulf St Vincent. Sci Total Environ 2023;856:158672.
21. Eberhard T, Casillas G, Zarus GM, Barr DB. Systematic review of microplastics and nanoplastics in indoor and outdoor air: identifying a framework and data needs for quantifying human inhalation exposures. J Expo Sci Environ Epidemiol 2024;34:185-96.
22. Prata JC, Castro JL, da Costa JP, Duarte AC, Rocha-Santos T, Cerqueira M. The importance of contamination control in airborne fibers and microplastic sampling: experiences from indoor and outdoor air sampling in Aveiro, Portugal. Mar Pollut Bull 2020;159:111522.
23. Giechaskiel B, Grigoratos T, Mathissen M, et al. Contribution of road vehicle tyre wear to microplastics and ambient air pollution. Sustainability 2024;16:522.
24. Abbasi S, Turner A. Dry and wet deposition of microplastics in a semi-arid region (Shiraz, Iran). Sci Total Environ 2021;786:147358.
25. Chandrakanthan K, Fraser MP, Herckes P. Airborne microplastics in a suburban location in the desert southwest: occurrence and identification challenges. Atmos Environ 2023;298:119617.
26. Liu S, Bai F, Men Z, et al. Spatial distribution, source apportionment and potential ecological risk assessment of suspended atmosphere microplastics in different underlying surfaces in Harbin. Sci Total Environ 2023;901:166040.
27. Zhao X, Zhou Y, Liang C, et al. Airborne microplastics: occurrence, sources, fate, risks and mitigation. Sci Total Environ 2023;858:159943.
28. Abbasi S, Ahmadi F, Khodabakhshloo N, et al. Atmospheric deposition of microplastics in Shiraz, Iran. Atmos Pollut Res 2024;15:101977.
29. Din K, Khokhar MF, Butt SI, Qadir A, Younas F. Exploration of microplastic concentration in indoor and outdoor air samples: morphological, polymeric, and elemental analysis. Sci Total Environ 2024;908:168398.
30. Scheurer M, Bigalke M. Microplastics in Swiss Floodplain Soils. Environ Sci Technol 2018;52:3591-8.
31. Zhang GS, Liu YF. The distribution of microplastics in soil aggregate fractions in southwestern China. Sci Total Environ 2018;642:12-20.
32. Abbasi S, Turner A, Hoseini M, Amiri H. Microplastics in the Lut and Kavir Deserts, Iran. Environ Sci Technol 2021;55:5993-6000.
33. Chandrakanthan K, Fraser MP, Herckes P. Microplastics are ubiquitous and increasing in soil of a sprawling urban area, Phoenix (Arizona). Sci Total Environ 2024;906:167617.
34. Palazot M, Soccalingame L, Froger C, et al. First national reference of microplastic contamination of French soils. Sci Total Environ 2024;918:170564.
35. Liu B, Shen Z, Zhou Q, et al. Microplastics in soil aggregates: analytical methods, occurrence patterns, impact analyses and removal approaches. TrAC Trend Anal Chem 2024;178:117855.
36. Lambert S, Wagner M. Microplastics are contaminants of emerging concern in freshwater environments: an overview. In: Wagner M, Lambert S, editors. Freshwater microplastics. Cham: Springer International Publishing; 2018. pp. 1-23.
37. Eerkes-Medrano D, Thompson RC, Aldridge DC. Microplastics in freshwater systems: a review of the emerging threats, identification of knowledge gaps and prioritisation of research needs. Water Res 2015;75:63-82.
38. Gatidou G, Arvaniti OS, Stasinakis AS. Review on the occurrence and fate of microplastics in Sewage Treatment Plants. J Hazard Mater 2019;367:504-12.
39. Phuong NN, Duong TT, Le TPQ, et al. Microplastics in Asian freshwater ecosystems: current knowledge and perspectives. Sci Total Environ 2022;808:151989.
40. Gomiero A, Øysæd KB, Jaén-gil A, Navrestad V, Skogerbø G. To what extent are wastewater treatment systems a gateway for microplastic particles in the aquatic and terrestrial environments? Water Emerg Contam Nanoplastics 2024;3:13.
41. Koelmans AA, Mohamed Nor NH, Hermsen E, Kooi M, Mintenig SM, De France J. Microplastics in freshwaters and drinking water: critical review and assessment of data quality. Water Res 2019;155:410-22.
42. Claessens M, De Meester S, Van Landuyt L, De Clerck K, Janssen CR. Occurrence and distribution of microplastics in marine sediments along the Belgian coast. Mar Pollut Bull 2011;62:2199-204.
43. Stolte A, Forster S, Gerdts G, Schubert H. Microplastic concentrations in beach sediments along the German Baltic coast. Mar Pollut Bull 2015;99:216-29.
44. Karthik R, Robin RS, Purvaja R, et al. Microplastics along the beaches of southeast coast of India. Sci Total Environ 2018;645:1388-99.
45. Barboza LGA, Dick Vethaak A, Lavorante BRBO, Lundebye AK, Guilhermino L. Marine microplastic debris: an emerging issue for food security, food safety and human health. Mar Pollut Bull 2018;133:336-48.
46. O’Brien S, Okoffo ED, O’Brien JW, et al. Airborne emissions of microplastic fibres from domestic laundry dryers. Sci Total Environ 2020;747:141175.
47. Soltani N, Amini-Birami F, Keshavarzi B, et al. Microplastic occurrence in selected aquatic species of the Persian Gulf: no evidence of trophic transfer or effect of diet. Sci Total Environ 2023;892:164685.
48. Pinlova B, Nowack B. Characterization of fiber fragments released from polyester textiles during UV weathering. Environ Pollut 2023;322:121012.
49. O’Loughlin C. The impact of activewear/swimwear laundering: investigating microplastic-fibre emissions from recycled and non-recycled synthetic textiles. J Home Econ Inst Aust 2018;25:2-12. Available from: https://search.informit.org/doi/10.3316/ielapa.107251401188651. [Last accessed on 9 Sep 2024]
50. Rathinamoorthy R, Raja Balasaraswathi S, Madhubashini S, Prakalya A, Rakshana JB, Shathvika S. Investigation on microfiber release from elastane blended fabrics and its environmental significance. Sci Total Environ 2023;903:166553.
51. Imhof HK, Ivleva NP, Schmid J, Niessner R, Laforsch C. Contamination of beach sediments of a subalpine lake with microplastic particles. Curr Biol 2013;23:R867-8.
52. D’Avignon G, Gregory-Eaves I, Ricciardi A. Microplastics in lakes and rivers: an issue of emerging significance to limnology. Environ Rev 2022;30:228-44.
53. Costigan E, Collins A, Hatinoglu MD, et al. Adsorption of organic pollutants by microplastics: overview of a dissonant literature. J Hazard Mater Adv 2022;6:100091.
54. Whiting QT, O’Connor KF, Potter PM, Al-Abed SR. A high-throughput, automated technique for microplastics detection, quantification, and characterization in surface waters using laser direct infrared spectroscopy. Anal Bioanal Chem 2022;414:8353-64.
55. Hatinoglu MD, Perreault F, Apul OG. Modified linear solvation energy relationships for adsorption of perfluorocarboxylic acids by polystyrene microplastics. Sci Total Environ 2023;860:160524.
56. Schets FM, Schijven JF, de Roda Husman AM. Exposure assessment for swimmers in bathing waters and swimming pools. Water Res 2011;45:2392-400.
57. Ramírez-Castillo FY, Loera-Muro A, Jacques M, et al. Waterborne pathogens: detection methods and challenges. Pathogens 2015;4:307-34.
58. Albarano L, Maggio C, La Marca A, et al. Risk assessment of natural and synthetic fibers in aquatic environment: a critical review. Sci Total Environ 2024;934:173398.
59. Chiu CA, Westerhoff P, Herckes P, Masles M, Elliott G. Monitoring of trace-level pharmaceuticals and personal care products in Salt River Project Waters Final Report. 2009. Available from: https://www.researchgate.net/publication/383877016_Monitoring_of_Trace-Level_Pharmaceuticals_and_Personal_Care_Products_in_Salt_River_Project_Waters_Final_Report_Submitted_to_Salt_River_Project. [Last accessed on 9 Sep 2024].
60. Banville MJ, Bateman HL. Urban and wildland herpetofauna communities and riparian microhabitats along the Salt River, Arizona. Urban Ecosyst 2012;15:473-88.
61. Yang Y, Reed R, Schoepf J, Hristovski K, Herckes P, Westerhoff P. Prospecting nanomaterials in aqueous environments by cloud-point extraction coupled with transmission electron microscopy. Sci Total Environ 2017;584-5:515-22.
62. Venkatesan AK, Reed RB, Lee S, et al. Detection and sizing of Ti-containing particles in recreational waters using single particle ICP-MS. Bull Environ Contam Toxicol 2018;100:120-6.
63. Metcalfe AN, Muehlbauer JD, Ford MA, Kennedy TA. Chapter 11 - Colorado River Basin. In: Rivers of North America. Elsevier; 2023. pp. 462-509.
64. Masura J, Baker J, Foster G, Arthur C. Laboratory methods for the analysis of microplastics in the marine environment: recommendations for quantifying synthetic particles in waters and sediments. 2015. Available from: https://repository.oceanbestpractices.org/handle/11329/1076. [Last accessed on 9 Sep 2024].
65. Dawson AL, Santana MFM, Nelis JLD, Motti CA. Taking control of microplastics data: a comparison of control and blank data correction methods. J Hazard Mater 2023;443:130218.
66. Hidalgo-Ruz V, Gutow L, Thompson RC, Thiel M. Microplastics in the marine environment: a review of the methods used for identification and quantification. Environ Sci Technol 2012;46:3060-75.
67. Baldwin AK, Spanjer AR, Rosen MR, Thom T. Microplastics in Lake Mead National Recreation Area, USA: occurrence and biological uptake. PLoS One 2020;15:e0228896.
68. Baldwin AK, King KA, Mason SA, Hoellein TJ, Kim LH, Karns B. Data release for microplastics in water, sediment, fish, and mussels in the St. Croix National Scenic Riverway and Mississippi National River and Recreation Area, Wisconsin and Minnesota, 2015. 2017. Available from: https://www.usgs.gov/data/data-release-microplastics-water-sediment-fish-and-mussels-st-croix-national-scenic-riverway. [Last accessed on 9 Sep 2024].
69. Moore CJ, Lattin GL, Zellers AF. Quantity and type of plastic debris flowing from two urban rivers to coastal waters and beaches of Southern California. RGCI 2011;11:65-73.
70. Inland Ocean Coalition. Pilot study finds evidence of microplastics in Colorado’s headwater stream. Available from: https://inlandoceancoalition.org/learn/plastic/microplastics-working-group/. [Last accessed on 9 Sep 2024].
71. Mallik A, Xavier KAM, Naidu BC, Nayak BB. Ecotoxicological and physiological risks of microplastics on fish and their possible mitigation measures. Sci Total Environ 2021;779:146433.
72. Hu E, Sun C, Yang F, et al. Microplastics in 48 wastewater treatment plants reveal regional differences in physical characteristics and shape-dependent removal in the transition zone between North and South China. Sci Total Environ 2022;834:155320.
73. Shamskhany A, Li Z, Patel P, Karimpour S. Evidence of microplastic size impact on mobility and transport in the marine environment: a review and synthesis of recent research. Front Mar Sci 2021;8:760649.
74. Napper IE, Thompson RC. Release of synthetic microplastic plastic fibres from domestic washing machines: effects of fabric type and washing conditions. Mar Pollut Bull 2016;112:39-45.
75. Thacharodi A, Meenatchi R, Hassan S, et al. Microplastics in the environment: a critical overview on its fate, toxicity, implications, management, and bioremediation strategies. J Environ Manage 2024;349:119433.
76. Martin J, Lusher A, Thompson RC, Morley A. The deposition and accumulation of microplastics in marine sediments and bottom water from the Irish Continental Shelf. Sci Rep 2017;7:10772.
77. Wood M, Simmonds L, MacAdam J, Hassard F, Jarvis P, Chalmers RM. Role of filtration in managing the risk from Cryptosporidium in commercial swimming pools - a review. J Water Health 2019;17:357-70.
78. United States EPA-832-F-22-015. Jump into pool water efficiency. 2022. Available from: https://www.epa.gov/system/files/documents/2022-09/ws-outdoor-pool-guide.pdf. [Last accessed on 9 Sep 2024].
79. Cisco J. The fate of microplastics in tres rios, a constructed treatment wetland. 2023. Available from: https://keep.lib.asu.edu/system/files/c7/Cisco_asu_0010N_22995.pdf. [Last accessed on 9 Sep 2024].
80. Carr SA. Sources and dispersive modes of micro-fibers in the environment. Integr Environ Assess Manag 2017;13:466-9.
81. Chandrakanthan K. Microplastics in the Desert Southwest: occurrence and characterization in atmospheric, aquatic, and terrestrial environments. 2024. Available from: https://keep.lib.asu.edu/system/files/c7/Chandrakanthan_asu_0010E_23729.pdf. [Last accessed on 9 Sep 2024].
Cite This Article
How to Cite
Download Citation
Export Citation File:
Type of Import
Tips on Downloading Citation
Citation Manager File Format
Type of Import
Direct Import: When the Direct Import option is selected (the default state), a dialogue box will give you the option to Save or Open the downloaded citation data. Choosing Open will either launch your citation manager or give you a choice of applications with which to use the metadata. The Save option saves the file locally for later use.
Indirect Import: When the Indirect Import option is selected, the metadata is displayed and may be copied and pasted as needed.
About This Article
Special Issue
Copyright
Data & Comments
Data
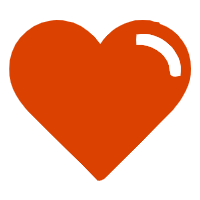
Comments
Comments must be written in English. Spam, offensive content, impersonation, and private information will not be permitted. If any comment is reported and identified as inappropriate content by OAE staff, the comment will be removed without notice. If you have any queries or need any help, please contact us at [email protected].