Oxy-biodegradable plastics in freshwater environments: degradation and biofouling
Abstract
Growing concern about the impact of plastic pollution on the environment has led to the creation of global public policies and the consumption of “environmentally friendly” products, such as oxy-biodegradable plastics. In this context, “greenwashing” practices can arise, i.e., the product promises more environmental benefits than it actually offers, which can lead to superfluous use, inappropriate disposal, and the generation of microplastics (MPs). However, the scientific literature lacks studies that evaluate the behavior of oxy-biodegradable plastic when exposed to the freshwater environment. In this respect, the aim of the current study was to evaluate the degradation process of oxy-biodegradable plastic bags made from high-density polyethylene (HDPE) in river water. To this end, the current study aimed to assess whether these bags actually meet the label information (which corresponds to the complete degradation of material) or whether they correspond to greenwashing practices. The physical and chemical alterations, and the formation of biological communities that occurred on the surface of the plastic material when exposed to natural aging and submerged in freshwater were monitored using mid-infrared absorption spectroscopy with attenuated total reflectance (FTIR-ATR) and scanning electron microscopy (SEM). The characterization of the samples after 180 days of exposure showed that the oxy-biodegradable bags were not completely degraded, with only fragmentation of the material and generation of MPs. In addition, it was also observed that microorganisms present in the water easily colonized the plastic surface from the start of the experiment. In this way, the oxy-biodegradable bags analyzed correspond to a greenwashing practice, which is extremely harmful, since it can influence the increase in consumption of these products, generating greater improper disposal of these materials, and consequently the generation of MPs and the formation of biofilms, which can carry pathogenic microorganisms to the aquatic biota and to humans.
Keywords
INTRODUCTION
The unique properties of plastic materials, such as their high durability, malleability, lightness, and low cost, justify their high consumption in various sectors of society; they are considered one of the most widely produced materials today, with recent estimates of global production of 400 million tons[1]. The high consumption of plastic materials combined with inadequate management and disposal has been a cause for great concern among the scientific community and society in general, due to their high persistence in the environment and potential ecological, economic, aesthetic, and public health impacts[2,3].
Plastic bags and disposable packaging made from polypropylene (PP) and polyethylene (PE) polymers are considered to be the most harmful in terms of the quantity of plastic waste in the environment[4], since between 500 billion and 1 trillion of these packages are produced every year[5]. It is estimated that after only 12 min of use, these products are already destined for the natural environment, where they accumulate for many years due to their resistance to degradation[6].
Faced with this problem, as a strategy for mitigating environmental liabilities, various public policies have been introduced, mainly in developed countries[7]. These policies encourage recycling, restrictive use, charging for the use of plastic bags by commercial establishments, and even their prohibition. Examples include California (USA), where the distribution of plastic bags was banned in 2016, and Ireland, where a tax on the use of plastic bags was introduced in 2002[8].
The plastics industry has also proposed alternatives aimed at “low environmental impact”, such as the production of oxy-biodegradable plastic. This type of plastic is made up of conventional polyolefin polymers, such as PE or PP, and also contains additives that accelerate the degradation of the material under certain conditions of temperature, light, and the presence of oxygen, thus causing the chemical bonds in the polymer chain to break, followed by the generation of smaller molecular fragments, until the material is completely degraded.
However, although the degradation of oxy-biodegradable plastic is faster than that of conventional plastic, previous studies have shown that in the marine environment, for example, only the fragmentation and generation of microplastics (MPs), which are pieces of plastic debris less than 5 mm in size, occur more quickly[2]. Despite this, there is still not enough of a reduction in molar mass for these fragments to be biodegraded and bioassimilated by microorganisms[9]. In addition, oxy-biodegradable plastic is a type of waste that makes recycling difficult due to the presence of the degrading additive in its composition. As a result, greenwashing practices emerge, i.e., advertising more ecological and environmental benefits than the product actually has, which influences consumers to buy these products. In addition, this information can encourage the consumption and improper disposal of oxy-biodegradable plastic.
In the aquatic environment, due to their small size, MP particles are easily ingested by biota, from lower trophic levels to higher tropic levels[10]. Depending on the aquatic organism and particle size, this ingestion can have lethal and sublethal consequences, such as obstruction of the digestive tract, malnutrition, suffocation, death, and even possible bioaccumulation and biomagnification processes[11]. MPs are also characterized by having a higher specific surface area - SSA, high porosity, and a hydrophobic character, which translates into greater potential for MPs to retain and transport organic and inorganic contaminants, as well as for the formation of microbial biofilms - which can be formed by both beneficial and harmful microorganisms. In addition, the presence of MPs can potentiate the emergence of antibiotic-resistant microorganisms (ARMs) and the spread of antibiotic resistance genes (ARGs)[12-14].
Knowing that freshwater ecosystems contribute around 80% of the plastic waste that reaches the marine environment and that most of the current research into the dynamics of plastic exposure is concentrated in the marine environment[9,13,15], the need to understand this phenomenon in the freshwater environment is clear. To date, studies that have more specifically evaluated the degradation and microbial formation in polyolefin plastics containing a degrading additive, i.e., oxy-biodegradable plastics, have focused only on the marine environment[9,16-21], with studies carried out in freshwater environments being extremely scarce[22,23].
Therefore, the aim of the current study was to evaluate the degradation process of commercial oxy-biodegradable plastic when exposed to a simulated freshwater environment. To this end, a parallel was drawn between the plastic materials evaluated and their compliance with the information on the product label, which classifies an oxy-biodegradable plastic as any plastic material that degrades completely within various environments and under different conditions[24]. The study also investigated the formation of microbial biofilms on the plastic surface when exposed to this aquatic matrix and whether materials marketed as oxy-biodegradable can be considered greenwashing.
METHODS
Obtaining plastic samples and experimental design
Conventional plastic bags of petrochemical origin, made from high-density polyethylene (HDPE), without a degrading additive (control), and oxy-biodegradable plastic bags made from the same polymer, with a degrading additive (d2w®), were purchased in the city of Piracicaba, São Paulo, Brazil. In the laboratory, experimental samples (n = 90) of each type of plastic bag were prepared by cutting them into strips of similar dimensions (3 cm × 20 cm). Thirty strip samples were added to each stainless steel aquarium filled with freshwater. The experiments were carried out in triplicate for each type of plastic material, totaling 6 different aquariums. Samples were then taken at random from each aquarium, in triplicate for each type of plastic, for chemical characterization at the start of the experiment (T0 = 0 days), after two months of exposure (T1 = 60 days), after four months of exposure (T2 = 120 days), and at the end of the experiment (T3 = 180 days), as well as for morphological characterization after 2 months of the experiment (T1 = 60 days).
Natural freshwater experiment
The simulated freshwater environment was prepared using 20 L of natural freshwater in each stainless steel aquarium. The natural freshwater used was collected from the Piracicaba River Reservoir, Barra Bonita Dam, Anhembi - SP, Brazil (S 22° 37.666’ WO 48° 10.430’). All the aquariums were kept outdoors from June to December 2019, in order to observe the kinetics of degradation and biofilm formation on the plastic surfaces, according to the different weather conditions, considering the light and dark cycles in the natural condition, and thus providing greater environmental relevance. The aquariums were equipped with circulation pumps to keep the water moving. The volume of water in each aquarium was also monitored throughout the experiment, and when necessary, ultrapure water was added. These interventions were necessary to avoid an abrupt increase in salinity in the aquariums[25].
Biological characterization of the river water
In the laboratory, the freshwater samples were diluted using the serial dilution technique and 100 µL were inoculated into petri dishes poured with rich culture medium for bacteria - Plate Count Agar - PCA, and for fungi - Potato Dextrose Agar (PDA). For this, aliquots of 1 mL of the river water sample were transferred to a series of test tubes containing 9 mL of sterile buffer solution - composed of phosphate buffer and magnesium chloride, in order to obtain serial dilutions of up to 10-4. The culture medium used was PCA, for plating and counting bacteria, with the following composition (m/v): 0.5% peptone; 0.25% yeast extract; 0.1% glucose; 1.4% agar; distilled water; pH 7.0 (± 0.2) at 35 °C, and PDA, for plating and counting fungi, with the composition (m/v): 3.9% PDA (potato dextrose agar); distilled water; pH 7.0 (± 0.2) at 28 °C. Plating was carried out on the day the river water sample was collected (T0 = 0 days), in triplicate, using the pour plate technique[26]. Microbial growth counts were expressed in colony forming units (CFU).
Sample characterization
FTIR-ATR
Confirmation of the chemical identity of the polymer and the chemical modifications undergone by the samples during natural aging were assessed using mid-infrared absorption spectroscopy with attenuated total reflectance (FTIR-ATR) (spectrophotometer Cary660, Agilent Technologies). The spectra were collected in absorbance mode and with a resolution of 4,000-400 cm-1 at 4 cm-1 using Agilent Resolution Pro software in 160 scans. This analysis was also used to identify the increased intensity of carbonyl groups, indicative of photo-oxidative degradation of the HDPE polymer, with a characteristic band around
Where a corresponds to the carbonyl band between 1,735 and 1,715 cm-1 and b corresponds to the reference band at 1,471 cm-1[27]. The carbonyl index values were compared using the Student’s t-test at a 5% significance level.
Scanning electron microscope
For morphological characterization of the plastic surfaces and qualitative analysis of biofilm formation, the samples were prepared on carbon strips, and metalized with gold for 180 s (LEICA ACE 600, Vienna). Scanned micrographs were obtained using a scanning electron microscope (SEM) (Jeol JSM - IT300 LV, Tokyo) operating at 15 kV.
Level of “greenwashing”
The evaluation and identification of greenwashing practices was based on the protocol described by the TerraChoice Environmental Marketing Agency, which points out the seven most common patterns or “sins” of greenwashing [Table 1]. The results obtained from the samples analyzed before and after natural aging were used to evaluate and classify the level of greenwashing in the oxy-biodegradable plastic investigated, according to the claims made on the product label.
The seven sins of greenwashing according to TerraChoice Environmental Marketing Agency[28]
The seven “sins” of greenwashing | Characteristics |
Camouflaging environmental costs | Suggesting that the product is sustainable based on a restricted set of attributes, without attention to other important environmental issues |
Lack of proof | An environmental claim that cannot be substantiated by easily accessible supporting information or reliable third-party certification |
Uncertainty | A claim so ill-defined or broad that its real meaning is likely to be misunderstood by the consumer |
Worship of false labels | A product that, through words or images, gives the impression of third-party endorsement where no such endorsement exists; false labels, in other words |
Irrelevance | An environmental claim that may be true, but is not important or does not help consumers looking for environmentally preferable products |
“Less worse” | A claim that may be true within the product category, but risks distracting the consumer from the larger environmental impacts of the category as a whole |
Lying | Environmental claims that are simply false |
RESULTS AND DISCUSSION
Colony counts of bacteria and fungi revealed a high abundance of the culturable microbial community in the freshwater of the Barra Bonita Dam [Table 2]. The CFU·mL-1 ranged from 4.5 × 108 to 6.6 × 108 (mean = 5.4 × 108) and 1.0 × 107 to 9.6 × 108 (mean = 3.2 × 108), respectively, for the culturable bacterial and fungal communities. The sampling site is located in an area that is a long way from polluting sources, because, according to SHELL and colleagues, environmental parameters, such as seasonal characteristics, physical-chemical properties of the water, properties of the biological communities, and, above all, proximity to anthropogenic inputs contribute directly to the behavior of plastic debris dispersed in the environment and the formation of biofilms. However, despite the absence of these inputs at the water sampling site, the presence of these microorganisms was expected, given that aquatic ecosystems host a vast diversity of microorganisms, associated with the quality and maintenance of ecosystem services in this environment[29]. Microbial populations play a crucial role in nutrient cycling, degradation of organic matter, and biodegradation of organic and inorganic pollutants - such as pesticides, antibiotics, oil and petroleum derivatives, and MPs - which are the contaminants frequently reported in bodies of water[30,31].
Quantitative analysis of microorganisms present in river water samples collected from the Piracicaba River Reservoir, Barra Bonita Dam, Anhembi-SP, Brazil
Samples | Bacteria (CFU·mL-1) | Fungi (CFU·mL-1) |
Sample 1 | 5.1 × 108 | 9.6 × 108 |
Sample 2 | 6.6 × 108 | 1.0 × 107 |
Sample 3 | 4.5 × 108 | 1.0 × 107 |
Average | 5.4 × 108 | 3.2 × 108 |
In addition, microorganisms in aquatic environments are the main precursors of the biodegradation of xenobiotic substances that reach water bodies, such as pesticides, drug residues, easily degraded organic loads, and more recalcitrant materials (e.g., MPs)[32]. Therefore, the microbial community with the highest species richness (cultivable microorganisms > 108-1010 CFU·mL-1), such as the results reported in this study [Table 2], can promote the rapid colonization of the plastic surface - biofilm formation - which extends both as a microbial mechanism of protection of microbiological life and as a strategy to promote the degradation of complex and recalcitrant substances via extracellular enzymatic activity[33].
The visual inspection of the surface of the oxy-biodegradable plastic by SEM, carried out 2 months after exposure to river water, indicated that, in agreement with the characterization of the water collected, microbial colonization occurred promptly, and it was possible to identify the presence of diatoms, fungi, and possible planktonic bacteria on the surface of the substrate [Figure 1].
Figure 1. SEM image showing the morphological characterization of the oxy-biodegradable plastic surface. (A) General view at the start of the experiment (T = 0); (B-D) Microbial colonization on the oxy-biodegradable bag substrate after 2 months of exposure (T = 1), where it is possible to see in detail the presence of diatom algae in different shapes (di), intense colonization by fungi (fu), and possible colonization by planktonic bacteria (arrow heads). (A-C) = 50 µm and (D) = 5 µm. SEM: Scanning electron microscopy.
In line with previous findings, where images obtained by SEM showed microbial colonization after a minimum incubation time of 1 month, and indicated through DNA sequencing results the presence of more than 52 bacterial phyla[13], this study also showed rapid microbial colonization on the plastic surface. The major problem with this phenomenon is associated with the possibility of trophic transfer of pathogenic species of bacteria and fungi to the biota, due to the possibility of ingestion of plastic debris in the aquatic environment, which occurs frequently. Therefore, this debris is identified as a potential vector of harmful microorganisms in these environments[10,12,31].
As a specific and complex microbial habitat, plastic debris can also support the selection of unique microorganisms and diverse communities. This fact is associated with the wide distribution and ease of vertical and horizontal transportation of this waste in the aquatic environment, which can lead to the migration of exotic species to other habitats, with profound impacts on the ecological effects of biological communities[12,31]. Furthermore, it is recognized by the scientific community that in addition to microbial attachment to the plastic surface, there is also sorption of contaminants present in the surrounding environment, such as antibiotics, which, in direct contact with certain bacteria, will inevitably lead to the continued increase in ARGs[34]. The production of these resistance genes in the freshwater environment increases the risk of exposure to plastic debris in this environmental matrix, since, in addition to the environmental and ecological importance, this is also a water source for human and animal consumption.
Coincidentally, during the same time period in which microorganisms settled on the plastic surface, changes in the material’s physical and chemical properties were observed, such as an increase in the density and sedimentation of the plastic strips at the bottom of the aquariums. The loss of buoyancy of the plastics made up of HDPE polymers, a polymer with a lower density than river water, represents a significant change in the fate of this debris in the water column. By losing buoyancy, this waste can move further from the photic zone in the aquatic environment and suffer less exposure to ultraviolet (UV) radiation, an essential factor in the degradation process of plastic made up of this polymer, which can have an impact on the abiotic degradation kinetics of this plastic waste[12,34].
The study by Lobelle and Cunliffe in 2011 also indicated that the formation of biofilms on the plastic surface led to a loss of buoyancy within 3 weeks. From a hydrodynamic point of view, this may have an impact on the fate of plastics in the aquatic environment, by changing the dynamics of the vertical transport of this low-density waste from the photic zone to the aphotic zone, and also on the bioavailability to benthic organisms.
Figure 2 presents the FTIR-ATR spectra obtained from the surface of the conventional plastic [Figure 2A] and oxy-biodegradable plastic [Figure 2B] samples at the start of the experiment, at increasing exposure intervals, and at the end of the experiment. As expected for the HDPE polymer, the following characteristic bands can be observed: 2,915 cm-1 (υC-H), 2,845 cm-1 (υC-H), 1,472 cm-1 (δCH2), 1,462 cm-1 (δCH2),
Figure 2. Comparative FTIR-ATR spectra of conventional plastic (A) and oxy-biodegradable plastic (B), at different stages of environmental exposure. Where T0 = initial time, T1 = 60 days of exposure, T2 = 120 days of exposure, and T3 = 180 days of exposure. FTIR: Fourier-transform infrared spectroscopy; ATR: attenuated total reflectance.
Plastics classified as polyolefins, i.e., made up of the PE polymer, among others, have only carbon and hydrogen in their structure, and their main abiotic degradation mechanism is photo-oxidative degradation, which is induced by the absorption of ultraviolet radiation in the presence of oxygen, allowing oxygen to be introduced into the polymer chain[36]. Thus, the presence of carbonyl groups in the polymer structure after environmental exposure indicates that the surface of the exposed material has undergone photo-oxidative degradation[37], which can be identified and monitored by analyzing the spectrum obtained by FTIR-ATR, or by quantifying the absorbance measurements of the typical carbonyl band, a characteristic band in infrared spectra in the region of 1,700 cm-1[27].
In the conventional plastic, it was possible to observe a slight carbonyl band directly proportional to the exposure time [Figure 2]. When calculating the carbonyl index values for both types of plastic [Figure 3], there was an increase in the carbonyl index during the experiment, indicating that both materials underwent the degradation process over the incubation period (180 days). The carbonyl index values were significantly higher in the 180 d period, where they practically tripled compared to the initial time (T = 0).
Figure 3. Boxplot of the carbonyl index of CP and OP after 180 days of exposure in the freshwater environment. *There was a difference between the treatments by the Student’s t-test at a 5% significance level. ns: not significant. CP: Conventional plastic bags; OP: oxy-biodegradable plastic bags.
As a consequence of photo-oxidative degradation, the plastic surface of both materials showed increased porosity and discoloration after 2 months of exposure to freshwater conditions. In the third month of the experiment, the oxy-biodegradable plastic samples began the fragmentation process, which became more pronounced over the course of the exposure time; the same did not occur with the conventional plastic, which remained intact until the end of the experiment, without suffering fragmentation.
However, despite the more pronounced physical changes in oxy-biodegradable plastic compared to conventional plastic, there was no complete degradation of the material, which corresponds to obtaining degradation products - H2O, CH4, or CO2, according to ASTM D6954 - 18[37], and only the generation of MPs, particles less than 5 mm in size, was observed. These particles had a breakable appearance, most of which were sedimented at the bottom of the aquarium along with the organic matter, while some were still floating, which made it difficult to carry out a quantitative analysis of the generation of MPs. However, with the absence of plastic strips in their initial dimensions and with just the presence of MP particles, it was only possible to see the fragmentation of the material and not its complete degradation in the aquatic environment.
The presence of MPs in the freshwater environment implies severe consequences for maintaining the quality of this ecosystem, as the removal of these particles is even more challenging than the removal of larger plastics. The ingestion of MPs by freshwater biota is also easier, as well as by organisms from the lower trophic levels, and this fact has already been reported for a wide range of species and, regardless of the type of plastic it comes from, which can cause severe effects depending on the properties and size of the particles and the species involved such as obstruction of the digestive tract, malnutrition, suffocation, death, and even possible bioaccumulation and biomagnification processes[10,11]. In addition, as observed in the current study, plastic waste is considered to be an ideal substrate for the attachment of microbial communities, with MPs being even more favorable due to their specific properties, such as a larger surface area and increased porosity resulting from their longer residence time in the environment, which implies greater potential for biological imbalance through the transfer of microorganisms by these particles and the formation of antibiotic resistance genes[13,34].
Thus, comparing the degradation of conventional plastic with oxy-biodegradable plastic in simulated freshwater environment conditions, we can point out that from an ecological and environmental point of view, oxy-biodegradable plastic did not behave in an advantageous way compared to conventional plastic, i.e., just like conventional plastic there was no complete degradation of the material, only fragmentation over the 180-day period investigated here. In addition, according to the results obtained in this study, we can indicate that oxy-biodegradable plastic is a greenwashing practice, since, according to the parameters listed by the TerraChoice Environmental Marketing Agency, the product has a cult of false labeling and camouflaged environmental costs. Other studies similar to this one, but carried out in simulated conditions of a marine environment and composting, also reached the same conclusion, which indicates the need for the scientific community to act in the assertive investigation of such practices and provide clarification to society, with the aim of raising public awareness[9,24,37]. Therefore, as our study is a pioneer in this field in Brazil, more research is needed to deepen our understanding of the degradation of oxy-biodegradable plastics and the generation of MPs in a freshwater environment, and over a longer period of exposure than that investigated here, considering the importance of this environmental compartment, given that the country holds around 13 percent of all freshwater in the world.
CONCLUSIONS
Oxy-biodegradable plastic bags are composed of the same non-biodegradable polymer of petrochemical origin as conventional plastic bags, with only the addition of a degrading additive. The general results of this study showed that despite indications of degradation, such as an increase in the carbonyl index on the surface of the material, oxy-biodegradable plastic only fragments into MP particles and does not present significantly reduced molecular mass to the point of being bioassimilated by microorganisms, i.e., it does not undergo complete degradation when exposed to fresh water in the presence of microorganisms over a 180-day period. Therefore, the bags analyzed, considering this period of exposure, correspond to a type of greenwashing practice, which is extremely harmful, since it can lead to increased consumption of these products, generating greater improper disposal of these materials and, consequently, the generation of MPs. In addition, microbial colonization on the plastic surface was also identified, which increases the risk of exposure of this waste in the freshwater environment. However, we recommend for future investigations that these same studies be carried out over a longer period of exposure in order to evaluate whether the product may present advantages over conventional plastic in the long term.
DECLARATIONS
Acknowledgments
The authors would like to thank the University of São Paulo (USP) for their infrastructure. This research was carried out with the support of the National Council for Scientific and Technological Development (CNPq) (process number 141021/2019-5 and process number 117292/2019-2) and Coordination for the Improvement of Higher Education Personnel (CAPES, Brazil, Finance Code 001) for granting a scholarship to AJS.
Authors’ contributions
Conceptualization, data curation, figures, table, writing - original draft preparation: Olivatto GP
Conceptualization, methodology, figures, writing - reviewing and editing: Lourenço ALA, de Souza AJ
Supervision: Tornisielo VL
Availability of data and materials
Not applicable.
Financial support and sponsorship
Conselho Nacional de Desenvolvimento Científico e Tecnológico (CNPq) process number 141021/2019-5 and Coordination for the Improvement of Higher Education Personnel (CAPES, Brazil, Finance Code 001) for granting a scholarship to de Souza AJ.
Conflicts of interest
All authors declared that there are no conflicts of interest.
Ethical approval and consent to participate
Not applicable.
Consent for publication
Not applicable.
Copyright
© The Author(s) 2024.
REFERENCES
1. Commission delegated regulation (EU) 2020/217 of 4 October 2019 amending, for the purposes of its adaptation to technical and scientific progress, Regulation (EC) No 1272/2008 of the European Parliament and of the Council on classification, labelling and packaging of substances and mixtures and correcting that Regulation. Available from: https://eur-lex.europa.eu/eli/reg_del/2020/217/oj. [Last accessed on 4 Mar 2024]
3. Nielsen TD, Hasselbalch J, Holmberg K, Stripple J. Politics and the plastic crisis: a review throughout the plastic life cycle. Wiley Interdiscip Rev Energy Environ 2020;9:e360.
4. Laskar N, Kumar U. Plastics and microplastics: a threat to environment. Environ Technol Innov 2019;14:100352.
5. Xanthos D, Walker TR. International policies to reduce plastic marine pollution from single-use plastics (plastic bags and microbeads): a review. Mar Pollut Bull 2017;118:17-26.
6. Geyer R, Jambeck JR, Law KL. Production, use, and fate of all plastics ever made. Sci Adv 2017;3:e1700782.
7. Van L, Hamid NA, Ahmad F, Ahmad ANA, Ruslan R, Tamyez PFM. Factors of single use plastic reduction behavioral intention. Emerg Sci J 2021;5:269-78.
8. Spranz R, Schlüter A, Vollan B. Morals, money or the master: the adoption of eco-friendly reusable bags. Mar Policy 2018;96:270-7.
9. Nazareth M, Marques MRC, Leite MCA, Castro ÍB. Commercial plastics claiming biodegradable status: is this also accurate for marine environments? J Hazard Mater 2019;366:714-22.
10. Azevedo-Santos VM, Brito MFG, Manoel PS, et al. Plastic pollution: a focus on freshwater biodiversity. Ambio 2021;50:1313-24.
11. Huang W, Song B, Liang J, et al. Microplastics and associated contaminants in the aquatic environment: a review on their ecotoxicological effects, trophic transfer, and potential impacts to human health. J Hazard Mater 2021;405:124187.
12. He S, Jia M, Xiang Y, et al. Biofilm on microplastics in aqueous environment: physicochemical properties and environmental implications. J Hazard Mater 2022;424:127286.
13. Martínez-Campos S, González-Pleiter M, Rico A, et al. Time-course biofilm formation and presence of antibiotic resistance genes on everyday plastic items deployed in river waters. J Hazard Mater 2023;443:130271.
14. Rummel CD, Jahnke A, Gorokhova E, Kühnel D, Schmitt-Jansen M. Impacts of biofilm formation on the fate and potential effects of microplastic in the aquatic environment. Environ Sci Technol Lett 2017;4:258-67.
15. Lobelle D, Cunliffe M. Early microbial biofilm formation on marine plastic debris. Mar Pollut Bull 2011;62:197-200.
16. Balestri E, Menicagli V, Vallerini F, Lardicci C. Biodegradable plastic bags on the seafloor: a future threat for seagrass meadows? Sci Total Environ 2017;605-6:755-63.
17. Emadian SM, Onay TT, Demirel B. Biodegradation of bioplastics in natural environments. Waste Manage 2017;59:526-36.
18. O’Brine T, Thompson RC. Degradation of plastic carrier bags in the marine environment. Mar Pollut Bull 2010;60:2279-83.
19. Brandon J, Goldstein M, Ohman MD. Long-term aging and degradation of microplastic particles: comparing in situ oceanic and experimental weathering patterns. Mar Pollut Bull 2016;110:299-308.
20. Nauendorf A, Krause S, Bigalke NK, et al. Microbial colonization and degradation of polyethylene and biodegradable plastic bags in temperate fine-grained organic-rich marine sediments. Mar Pollut Bull 2016;103:168-78.
21. Kedzierski M, D’Almeida M, Magueresse A, et al. Threat of plastic ageing in marine environment. Adsorption/desorption of micropollutants. Mar Pollut Bull 2018;127:684-94.
22. Arias-Villamizar CA, Vázquez-Morillas A. Degradation of conventional and oxodegradable high density polyethylene in tropical aqueous and outdoor environments. Rev Int Contam Ambie 2018;34:137-47.
23. Chiellini E, Corti A, D’Antone S. Oxo-biodegradable full carbon backbone polymers - biodegradation behaviour of thermally oxidized polyethylene in an aqueous medium. Polym Degrad Stabil 2007;92:1378-83.
24. Adamcová D, Radziemska M, Fronczyk J, Zloch J, Vaverkova MD. Research of the biodegradability of degradable/biodegradable plastic material in various types of environments. Sci Rev Eng Environ Sci 2017;26:3-14.
25. Kalogerakis N, Karkanorachaki K, Kalogerakis GC, et al. Microplastics generation: onset of fragmentation of polyethylene films in marine environment mesocosms. Front Mar Sci 2017;4:84.
26. Tebaldi VMR, Souza AM, de Souza YHS, de Carvalho Alves JN, Piccoli RH. Evaluation of the levels of microbiological contamination of the water of the paraíba do sul river in the urban perimeter of Barra Mansa-RJ. Braz J Health Rev 2021;4:17138-53.
27. Rodrigues MO, Abrantes N, Gonçalves FJM, Nogueira H, Marques JC, Gonçalves AMM. Spatial and temporal distribution of microplastics in water and sediments of a freshwater system (Antuã River, Portugal). Sci Total Environ 2018;633:1549-59.
28. The sins of greenwashing: home and family edition. 2010. Available from: https://www.twosides.info/wp-content/uploads/2018/05/Terrachoice_The_Sins_of_Greenwashing_-_Home_and_Family_Edition_2010.pdf. [Last accessed on 4 Mar 2024]
29. de Souza AJ, de Araújo Pereira AP, Pedrinho A, et al. Land use and roles of soil bacterial community in the dissipation of atrazine. Sci Total Environ 2022;827:154239.
30. Sun Y, Ren X, Rene ER, et al. The degradation performance of different microplastics and their effect on microbial community during composting process. Bioresour Technol 2021;332:125133.
31. Lear G, Kingsbury JM, Franchini S, et al. Plastics and the microbiome: impacts and solutions. Environ Microbiome 2021;16:2.
32. Yuan J, Ma J, Sun Y, Zhou T, Zhao Y, Yu F. Microbial degradation and other environmental aspects of microplastics/plastics. Sci Total Environ 2020;715:136968.
33. Badea MA, Balas M, Dinischiotu A. Microplastics in freshwaters: implications for aquatic autotrophic organisms and fauna health. Microplastics 2023;2:39-59.
34. Wang Y, Zhou B, Chen H, Yuan R, Wang F. Distribution, biological effects and biofilms of microplastics in freshwater systems - a review. Chemosphere 2022;299:134370.
35. Jung MR, Horgen FD, Orski SV, et al. Validation of ATR FT-IR to identify polymers of plastic marine debris, including those ingested by marine organisms. Mar Poll Bull 2018;127:704-16.
36. Hammer J, Kraak MHS, Parsons JR. Plastics in the marine environment: the dark side of a modern gift. In: Witacre D, editor. Reviews of environmental contamination and toxicology. New York: Springer; 2012. pp. 1-44.
37. ASTM International. Standard guide for exposing and testing plastics that degrade in the environment by a combination of oxidation and biodegradation. Available from: https://www.astm.org/d6954-18.html. [Last accessed on 4 Mar 2024].
Cite This Article
How to Cite
Download Citation
Export Citation File:
Type of Import
Tips on Downloading Citation
Citation Manager File Format
Type of Import
Direct Import: When the Direct Import option is selected (the default state), a dialogue box will give you the option to Save or Open the downloaded citation data. Choosing Open will either launch your citation manager or give you a choice of applications with which to use the metadata. The Save option saves the file locally for later use.
Indirect Import: When the Indirect Import option is selected, the metadata is displayed and may be copied and pasted as needed.
About This Article
Special Issue
Copyright
Data & Comments
Data
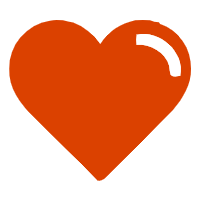
Comments
Comments must be written in English. Spam, offensive content, impersonation, and private information will not be permitted. If any comment is reported and identified as inappropriate content by OAE staff, the comment will be removed without notice. If you have any queries or need any help, please contact us at [email protected].