Analytical upgrade of a methodology based on UHPLC-MS/MS for the analysis of endocrine disrupting compounds in greywater
Abstract
A multi-residue analytical methodology for the determination of 25 endocrine disrupting compounds (EDCs), encompassing various chemical classes (hormones, antimicrobials, preservatives, plasticizers, stimulants, alkylphenolic compounds, anticorrosives, and organophosphorus flame retardants), has been upgraded for the analysis of greywater. The methodology is based on solid phase extraction (SPE) followed by ultra-high-performance liquid chromatography-tandem mass spectrometry (UHPLC-MS/MS). The optimized methodology achieved recoveries between 63% and 146% for all compounds, with MDLs ranging from 0.3 to 141 ng/L. Most of the compounds showed a pronounced signal suppression in the laundry greywater tested, and therefore, quantification was performed with a matrix-matched calibration curve to surpass the matrix effects observed (between -100% and 106%). Additionally, polyvinylidene fluoride (PVDF) membrane filters were selected among several filter types as the most suitable for greywater filtration. The upgraded methodology allowed the detection of 14, four and four EDCs in laundry, kitchen and shower/sink greywater, respectively. Caffeine was the only compound detected in all types of greywater, showing the highest concentrations (> 40,000 ng/L in kitchen greywater, and 2,360 ng/L in laundry greywater), followed by methylparaben and 1H-benzotriazole (1,607 and 776 ng/L, respectively, in laundry greywater). This analytical methodology constitutes an important tool for monitoring different families of contaminants in greywater, a poorly studied matrix, which is nowadays being considered as a future source of freshwater, contributing to overcoming problems of water scarcity. Water monitoring thus helps to guarantee water quality in water reuse practices and to understand EDC exposure patterns and their potential environmental impact.
Keywords
INTRODUCTION
Greywater is the wastewater most frequently generated in households, accounting for 70%-75% of the total volume of domestic wastewater[1]. This type of wastewater is generated in showers, hand basins, kitchen sinks, laundry machines, dishwashers, and bathtubs, and it concentrates a part of the total organic micropollutant load[2,3]. Each of these greywaters has its respective characteristics. For instance, laundry greywater contains detergents, oils, solvents, bleach, nonbiodegradable fibers from clothing, and also high concentrations of suspended solids, organic carbon, and bacteria[4], which makes it a very complex matrix. Currently, greywater is mixed with wastewater from other sources in sewer systems and transported to conventional wastewater treatment plants, which have a limited ability to remove numerous contaminants present in waste and greywaters, leading to their discharge into the environment[5]. Among these contaminants, the endocrine disrupting compounds (EDCs) can, on some occasions and despite their low concentrations, exert direct negative effects on aquatic ecosystems and human health[6]. EDCs are a class of emerging contaminants that encompasses exogenous organic substances naturally or anthropogenically occurring in the environment, which can act as a substitute for natural hormones in the bodies of humans and animals. These substances can inhibit or imitate the effects of the target hormones, affecting the health of those contaminated, and therefore, they are capable of bioaccumulating in aquatic organisms because of their stability and persistence[7]. Natural and synthetic hormones are included in this group, as well as industrial chemicals and household products, i.e., alkylphenolic compounds, pharmaceuticals, polycyclic aromatic compounds, additives, personal care products, plastics, textiles and construction materials, and pesticides[8,9].
There are several analytical methodologies for the analysis of EDCs in environmental matrices, though most of them focus on specific families of EDCs. Gas chromatography coupled to mass spectrometry (GC-MS) and tandem mass spectrometry (GC-MS/MS) is an excellent choice for the analysis of estrogenic hormones, alkylphenolic compounds or parabens in environmental matrices[10,11], being one of the most powerful techniques for the analysis of volatile multicomponent samples. Indeed, several studies analyzing natural and synthetic hormones, triclosan, benzophenones, and parabens by GC-MS are reported in literature[12-16]. However, some of these compounds, as is the case of bisphenols or triclosan, require a derivatization step to improve their volatility[17], which is time-consuming. Liquid chromatography (LC) is also used, with a range of detectors, including diode array detector (DAD)[18], fluorescence (FLV)[19], and MS/MS[20]. Recently, an increased number of articles have reported the use of LC-MS/MS technique because it allowed the development of multi-residue methodologies, embracing a higher number of EDCs belonging to different families, and providing more selectivity, specificity, and sensitivity[21]. Besides that, LC-MS/MS does not require a derivatization step for the analysis of different families of EDCs (e.g., antimicrobials, bisphenols, hormones, parabens, alkylphenols) in several matrices, allowing a quick analysis[22-25].
Several EDCs have been detected in various environmental matrices at low concentrations, such as drinking water[26], wastewater[27], surface water[28], or biota[29], typically measured in parts per trillion (ng/L) and parts per billion (µg/L). Given the complex nature of these matrices, advancements in sample preparation and instrumental techniques are necessary for their accurate detection. Nevertheless, there is still a gap of knowledge on the presence of EDCs in greywater, particularly considering its diverse origins whereby each source introduces distinct compounds. Few literature references have been found using LC or GC techniques-based methodologies to analyze EDCs in different types of greywater. For instance, Palmquist and Hanaeus[30] detected flame-retardants, nonylphenol, triclosan, and octylphenol in greywater from bathrooms, hand washbasins, laundry, and kitchens in µg/L level analyzed by GC-MS. Zedek et al. employed LC-MS/MS to analyze triclosan, triclocarban, and parabens in washing machines and shower greywater, finding concentrations in the range of ng/L[31]. Finally, Noutsopoulos et al. showed that bath and shower activities were the most important contributors of nonylphenol, triclosan, and bisphenol A (BPA), followed by laundry and the kitchen sink, using GC-MS for the analysis of these compounds[32].
The environmental relevance of EDCs, due to their negative effects at low concentrations and their current presence in the environment, highlights the need to optimize analytical methodologies for their sensitive and accurate detection. Furthermore, the wide range of compounds with distinct physico-chemical properties that encompass the EDC classifications requires multi-residue analytical methodologies. In this context, the aim of this work was to upgrade an analytical methodology[33] based on UHPLC-MS/MS for the multi-residue analysis of 25 EDCs belonging to several chemical classes - natural and synthetic hormones, antimicrobials, preservatives, plasticizers, stimulants, alkylphenolic compounds, anticorrosives and organophosphorus flame retardants - and to validate it by its application in different types of greywater, namely shower, kitchen sink, and laundry greywater, all poorly studied separately.
EXPERIMENTAL
Chemical and reagents
The list of the 25 standards of the selected EDCs (high purity grade > 95%) is shown in Table 1, including their CAS and supplier. Stock standard solutions of 1,000 mg/L were prepared in methanol and kept frozen at -20 ºC. Working standard solutions for the preparation of calibration curves were prepared in methanol:water (20:80, v/v) following these concentrations: 0.05, 1, 5, 10, 25, 50 and 100 µg/L. Isotopically labeled compounds (high purity grade > 95%), which were used as internal standards, were: estrone-d4, estradiol-d2, ethinylestradiol-d4, bisphenol A-d4 (BPA-d4), caffeine-d3, octylphenol-d17 and nonylphenol-d4 from CDN Isotopes; progesterone-d9, triclosan-d3 and bisphenol B-d8 (BPB-d8) from LGC Standards; methylparaben-d4 and triphenyl phosphate-d15 (TCPP-d15) from Sigma-Aldrich.
List of the analyzed target endocrine disrupting compounds (EDCs) organized by their class and including the CAS number and the supplier
Class | Compound | CAS number | Supplier |
Hormones | Diethylstibesterol | 56-53-1 | LGC Standards |
Estradiol | 50-28-2 | Santa Cruz Biotechnology | |
Estriol | 50-27-1 | VWR International Eurolab | |
Estrone | 53-16-7 | LGC Standards | |
Estrone-3-sulfate | 1240-04-6 | Santa Cruz Biotechnology | |
Ethinylestradiol | 57-63-6 | LGC Standards | |
Progesterone | 57-83-0 | Vitro Master Diagnóstica | |
Antimicrobial | Triclosan | 5150-64-1 | Sigma-Aldrich |
Preservatives | Benzylparaben | 94-18-8 | Sigma-Aldrich |
Ethylparaben | 120-47-8 | Santa Cruz Biotechnology | |
Methylparaben | 99-76-3 | Sigma-Aldrich | |
Propylparaben | 94-13-3 | Sigma-Aldrich | |
Plasticizers | Bisphenol A (BPA) | 80-05-07 | Sigma-Aldrich |
Bisphenol AF (BPAF) | 1478-61-1 | Sigma-Aldrich | |
Bisphenol B (BPB) | 77-40-7 | Sigma-Aldrich | |
Bisphenol F (BPF) | 620-92-3 | Sigma-Aldrich | |
Bisphenol S (BPS) | 80-09-1 | Sigma-Aldrich | |
Stimulant | Caffeine | 58-08-2 | Santa Cruz Biotechnology |
Alkylphenolic compounds | Nonylphenol | 84852-15-3 | Santa Cruz Biotechnology |
Octylphenol | 1806-26-4 | Sigma-Aldrich | |
Anticorrosives | 1H-benzotriazole | 95-14-7 | Thermo Fisher Scientific |
Benzotriazole-5-Methyl-1H (Tolyltriazole) | 136-85-6 | Sigma-Aldrich | |
Organophosphorus flame retardants | Tris(2-butoxyethyl)phosphate (TBEP) | 78-51-3 | Cymit Quimica |
Tris(2-chloroethyl)phosphate (TCEP) | 115-96-8 | Sigma-Aldrich | |
Tris(chloroisopropyl)phosphate (TCPP) | 13674-84-5 | Cymit Quimica |
MS-grade methanol and water were purchased from Merck (Germany). Acetic acid of high purity (100%) was purchased from Panreac (Spain). Ammonia solution (28% v/v) and EDTA 0.1M were obtained from Scharlau (Spain).
Sample collection
Three greywater samples were characterized: (1) greywater from showers of a hotel located in Lloret de Mar, 40 km from Girona (Catalonia, Spain) (Sh-GW); (2) water output from the kitchen sink of a research center in Girona (Catalonia, Spain) (Ki-GW); and (3) greywater from a laundry from Girona (Catalonia, Spain) (La-GW). Sh-GW was taken as a grab sample from a storage tank before its reuse. The hotel applies full-scale source separation (i.e., shower greywater segregation from other wastewater sources) and partial reuse of treated greywater for toilet flushing (approx. 13,500 to 15,000 m3 per year[34]). Ki-GW was a composite sample of three days of the same week, 6 h per day. In the case of La-GW, a day composite sample for 5 days (6 h per day) was used. Restaurants, tourist facilities, rest homes, a clinical analysis laboratory, and a research institute are included in the list of clients of the laundry. In all cases, greywater samples were stored for one week at 4 ºC before analysis.
Physico-chemical analysis
The pH value was measured with a pH meter (GLP21+, Crison Instruments SA, Alella, Spain) and the electrical conductivity was measured with a conductivity meter (GLP31+, Crison Instruments SA, Alella, Spain). Total suspended soils (TSS) were measured gravimetrically after sample filtration with a glass microfiber filter and subsequent drying to constant weight. Chemical oxygen demand (COD) was analyzed according to the dichromate method UNE 77004:2002. The concentration of soluble cations (i.e., ammonium (NH4+), sodium (Na+), potassium (K+), magnesium (Mg2+) and calcium (Ca2+), and the concentrations of the soluble anions [i.e., nitrite (NO2-), nitrate (NO3-), phosphate (PO43-), chloride (Cl-), sulfate (SO42-)] were determined by ion chromatography (ICS 5000, Dionex, Sunnyvale, USA) after filtering the samples with 0.2 µm nylon filters.
Sample pretreatment (Filtration)
Due to the content of suspended particulate matter in the greywaters, sample pretreatment is needed to prevent the clogging of SPE cartridges during the extraction. This step includes sample filtration, which may lead to the loss of analytes due to their retention in the filter material. Therefore, an experimental filter test was performed using laundry greywater to evaluate the potential losses of the selected emerging contaminants during the sample filtration step before sample preconcentration. 25 mL of La-GW, after prefiltered with glass fiber membrane (2.7 µm pore size), was spiked with a mixture of all EDCs to get a final concentration of 50 µg/L, and then was filtered with four different types of 47 mm diameter membrane filters: nylon (Merck), PVDF (Merck) and cellulose acetate (Allcrom) filter with 0.45 µm pore size and glass fiber (Whatman) filter with 0.70 µm pore size. The percentage of the recovered analyte mass was calculated using Equation 1:
where the “mass analytesample filtered” refers to the mass of each EDC in the spiked laundry greywater sample that underwent filtration using different filters, while the “mass analytesample no-filtered” corresponds to the mass of each EDC in the spiked laundry greywater sample that did not undergo any filtration.
Extraction
Greywater samples were extracted with a method adapted from Gros et al.[35]. Briefly, 50 mL of Sh-GW and 25 mL from Ki-GW and La-GW were filtered through 0.45 µm PVDF membrane filters. For Ki-GW and La-GW, 2.7 and 0.7 µm glass-membrane fiber filters were used as prefilters because of the turbidity of the matrices. 750 µL of EDTA 0.1 M were added to Ki-GW and La-GW, whereas 1,500 µL were added to
UHPLC-MS/MS analysis
Method optimization
For the optimization of the chromatographic separation, the analysis of EDCs by UHPLC-MS/MS was upgraded on the basis of the method developed by Jakimska et al.[33]. Briefly, this method uses an Acquity BEH C18 column (50 mm × 2.1 mm i.d., 1.7 µm particle size) (Waters Corporation) for positive and negative mode with (A) methanol (B) water (pH 9, adjusted with ammonia) as the mobile phase with a flow rate of 0.4 mL/min. The column temperature was maintained at 40 ºC for negative electrospray ionization (NI) and at room temperature for positive electrospray ionization (PI), and the injection volume was 5 µL. In the upgrade methodology proposed herein chromatographic conditions were evaluated, namely LC column - Acquity BEH C18 column (50 mm × 2.1 mm i.d., 1.7 µm particle size) (Waters Corporation), and Kinetex Biphenyl column (50 mm × 2.1 mm i.d., 2.6 µm particle size) (Phenomenex), mobile phase, elution gradient, and column temperature, in order to determine the best ones for the analysis of the selected EDCs. To evaluate which of these LC conditions improved the analysis, different instrumental tests using a calibration curve in methanol:water (20:80, v/v) were done.
Upgraded analytical methodology
Chromatographic analysis was performed on a Waters Acquity Ultra-PerformanceTM liquid chromatography system equipped with two binary pump systems (Milford, MA, USA). A Kinetex Biphenyl (50 mm × 2.1 mm i.d., 2.6 µm particle size) analytical column (Phenomenex, Spain) was used for both PI and NI modes equipped with a guard column (Phenomenex, Spain). The optimized chromatographic conditions were as follows: for PI mode, solvent (A) methanol and (B) water MS grade; for NI mode were (A) methanol and (B) water MS grade (adjusted with ammonia,
The UHPLC instrument was coupled to a 5500 QTRAP hybrid triple quadrupole-linear ion trap mass spectrometer (Applied Biosystems, Foster City, CA, USA) with a turbo Ion Spray source. In both modes, target scan time (TST) was set at 1 s, with a selected reaction monitoring (SRM) detection window of 60 s. The resolution at the first quadrupole (Q1) was set at the unit, and the third quadrupole (Q3) was set at low and the pause between mass ranges was 5 ms. The settings for source-dependent parameters were set up as follows: for PI mode, curtain gas, 30 V; Nitrogen collision gas medium; source temperature of 600 ºC; ion spray voltage at
Quantification of analytes was performed by SRM by monitoring two mass transitions between the precursor ion and the two most abundant fragment ions for each compound. The one at higher intensity was used for quantification purposes, while the second one was used for confirmation of the compound identification.
RESULTS AND DISCUSSION
Instrumental method optimization
The mass spectrometry (MS) optimization was achieved by directly infusing standard solutions of target analytes into the MS system. For quantification, the most intense ion fragmentation was selected, while for confirmation, the second most intense ion was chosen, although in some cases, the confirmation ion fragment could not be defined. Additionally, the compound SRM ratio was calculated to confirm the peak identity of the selected analytes, which is defined as the ratio between the areas of the peaks of quantification and confirmation ions (maximum tolerance of 20%)[36]. A summary of the compound-dependent MS parameters, including declustering potential (DP), collision energy (CE) and collision cell exit potential (CXP), as well as the SRM transitions, is shown in Table 2.
Target compounds organized by their ionization mode, including their RT, SRM transitions, compound-dependent MS parameters and SRM ratio
Compounds | RT min | Precursor ion m/z | Quantification | Confirmation | SRM ratio (± SD) | ||
Q3 | DP/CE/CXP | Q3 | DP/CE/CXP | ||||
Compounds analyzed under PI mode | |||||||
1H-benzotriazole | 1.40 | 120.1 [M+H]+ | 64.9 | 141/29/10 | 92.1 | 141/23/8 | 3.62 (± 0.15) |
1H-benzotriazole-d4 (IS) | 1.40 | 124.1 [M+H]+ | 69.1 | 21/29/12 | - | - | - |
Caffeine-d3 (IS) | 1.76 | 198.0 [M+H]+ | 138.0 | 71/27/10 | - | - | - |
Caffeine | 1.80 | 195.0 [M+H]+ | 138.0 | 86/27/20 | 42.0 | 86/63/8 | 4.23 (± 0.37) |
Tolyltriazole | 2.00 | 134.1 [M+H]+ | 76.9 | 41/35/10 | 78.9 | 41/27/8 | 1.81 (± 0.08) |
Tris(2-chloroethyl)phosphate (TCEP) | 3.01 | 284.8 [M+H]+ | 63.0 | 11/49/12 | 98.9 | 11/33/16 | 1.21 (± 0.05) |
Tris(chloroisopropyl)phosphat (TCPP) | 3.74 | 327.1 [M+H]+ | 98.9 | 121/25/48 | 80.8 | 121/83/12 | 1.57 (± 0.03) |
Trisphenylphosphate-d15 (IS) | 4.37 | 342.0 [M+H]+ | 54.0 | 141/117/14 | - | - | - |
Tris(2-butoxyethyl)phosphate (TBEP) | 4.46 | 399.1 [M+H]+ | 99.0 | 46/51/18 | - | - | - |
Progesterone | 4.94 | 315.0 [M+H]+ | 97.1 | 86/33/8 | 106.0 | 86/39/8 | 1.06 (± 0.02) |
Progesterone-d8 (IS) | 4.91 | 323.2 [M+H]+ | 100.0 | 91/29/16 | - | - | - |
Compounds analyzed under NI mode | |||||||
Bisphenol S (BPS) | 0.65 | 249.0 [M-H]- | 107.9 | -115/-36/-7 | 92.0 | -115/-42/-13 | 1.81 (± 0.08) |
Methylparaben-d4 (IS) | 1.34 | 155.0 [M-H]- | 96.1 | -65/-28/-1 | - | - | - |
Methylparaben | 1.53 | 151.0 [M-H]- | 92.0 | -30/-20/-7 | 136.0 | -55/-20/-7 | 1.54 (± 0.07) |
Estrone-3-sulfate | 1.75 | 349.0 [M-H]- | 269.0 | -10/-36/-13 | 145.0 | -15/-60/-13 | 2.81 (± 0.10) |
Ethylparaben | 2.13 | 165.0 [M-H]- | 92.0 | -29/-25/-7 | 136.0 | -50/-22/-7 | 1.89 (± 0.08) |
Estriol | 2.52 | 287.0 [M-H]- | 171.1 | -120/-50/-11 | 144.9 | -120/-56/-9 | 1.32 (± 0.03) |
Bisphenol F (BPF) | 2.57 | 199.1 [M-H]- | 92.9 | -100/-28/-5 | 104.9 | -100/-26/-13 | 1.73 (± 0.13) |
Propylparaben | 2.69 | 179.0 [M-H]- | 92.0 | -70/-32/-11 | 136.0 | -70/-22/-9 | 2.23 (± 0.25) |
Octylphenol-d17 (IS) | 2.71 | 222.9 [M-H]- | 74.9 | -205/-50/-7 | - | - | - |
Triclosan-d3 (IS) | 2.88 | 303.0 [M-H]- | 79.9 | -55/-56/-13 | - | - | - |
BPA-d4 (IS) | 3.06 | 231.0 [M-H]- | 216.0 | -85/-26/-7 | - | - | - |
Bisphenol A (BPA) | 3.08 | 227.0 [M-H]- | 212.0 | -60/-26/-11 | 133.2 | -60/-34-7 | 2.70 (± 0.20) |
Bisphenol AF (BPAF) | 3.15 | 335.1 [M-H]- | 265.1 | -125/-32/-13 | 68.9 | -125/-64/-9 | 9.16 (± 1.00) |
Bisphenol B-d8 (BPB-d8) (IS) | 3.33 | 249.1 [M-H]- | 219.1 | -40/-38/-11 | - | - | - |
Bisphenol B (BPB) | 3.36 | 241.1 [M-H]- | 212.0 | -95/-22/-27 | 211.1 | -95/-38/-9 | 1.41 (± 0.06) |
Diethylstilbesterol | 3.41 | 267.0 [M-H]- | 237.0 | -75/-38/-11 | 222.0 | -45/-46/-11 | 1.09 (± 0.02) |
Benzylparaben | 3.43 | 227.0 [M-H]- | 92.1 | -50/-36/-7 | 135.9 | -50/-20/-9 | 1.49 (± 0.17) |
Estradiol-d2 (IS) | 3.54 | 273.0 [M-H]- | 147.0 | -35/-78/-13 | - | - | - |
Estradiol | 3.56 | 271.0 [M-H]- | 145.0 | -35/-52/-9 | 183.0 | -35/-54/-9 | 1.17 (± 0.02) |
Ethinylestradiol-d4 (IS) | 3.56 | 299.1 [M-H]- | 145.0 | -60/-76/-9 | - | - | - |
Ethinylestradiol | 3.57 | 295.1 [M-H]- | 145.0 | -50/-56/-7 | 143.0 | -50/-76/-9 | 1.11 (± 0.01) |
Estrone | 4.05 | 269.1 [M-H]- | 145.0 | -70/-48/-9 | 143.1 | -70/-76/-9 | 1.91 (± 0.08) |
Estrone-d4 (IS) | 4.05 | 273.1 [M-H]- | 145.0 | -65/-74/-7 | - | - | - |
Triclosan | 4.19 | 286.8 [M-H]- | 34.9 | -60/-44/-5 | - | - | - |
Octylphenol | 4.30 | 205.0 [M-H]- | 106.0 | -55/-28/-5 | - | - | - |
Nonylphenol-d4 (IS) | 4.42 | 223.0 [M-H]- | 110.2 | -195/-30/-5 | - | - | - |
Nonylphenol | 4.44 | 219.1 [M-H]- | 106.0 | -85/-28/-13 | - | - | - |
To achieve a good separation and improve the sensitivity of the analytical methodology for the analysis of the selected EDCs, different mobile phases were tested by the injection of mix standards solutions using two chromatographic columns: Acquity BEH C18 column (50 mm × 2.1 mm i.d., 1.7 µm particle size), and Kinetex Biphenyl column (50 mm × 2.1 mm i.d., 2.6 µm particle size). Different mobile phases were tested in this study consisting of MeOH as the organic phase and water with different additives as the aqueous phase: (a) H2O; (b) 0.1% acetic acid in water; and (c) H2O (pH 9.8 adjusted with ammonia). In terms of LC column, in comparison to Kinetex Biphenyl, a slight tailing was observed using BEH C18 in all the analyzed compounds for positive and negative modes. This effect was probably because of separation issues caused by the LC column. The presence of polar phenyl groups in the stationary phase of the Kinetex Biphenyl column enhances the selectivity for cyclic and aromatic compounds through the promotion of π-π interactions[37], being more suitable for the analysis of hydrophobic, aromatic, and polar compounds[38].
In the NI, several analytes exhibited tailing and double peaks when using H2O and MeOH as the mobile phase, except for estrone-3-sulfate, ethylparaben, methylparaben, propylparaben, and bisphenol S (BPS). This observation can be attributed to the fact that these compounds have the lowest pKa values for the negative mode (-1.75 - 8.5). Conversely, when MeOH was combined with the acidic aqueous mobile
After that, different column temperatures and injection volumes were tested. The column temperature was set at 30 and 40 ºC, although no differences had been observed, so for both PI and NI modes, the temperature was determined as 40 ºC. In both ionization modes, changing the injection volume from 5 to 10 µL did not improve the quality of the peaks. Therefore, 5 µL was the volume selected in order to use less sample volume and prevent any possible contamination of the column, which could cause carry-over of compounds between analyses. Two different gradients were tested in order to reduce the total analysis time while maintaining the chromatographic resolution: (G1) For PI and NI modes: 0-7 min, 20%-100% A;
In summary, the main differences respect to the previous method[33] were as follows: the LC column was changed from Acquity BEH C18 to Kinetex Biphenyl; the mobile phase for PI mode was changed from MeOH and H2O (pH 9, adjusted with ammonia) to MeOH and H2O; the temperature in PI was set at 40 ºC; and the elution gradient was changed to: 0-5 min, 20%-100% A; 5-6 min, 100% A; 6-6.5 min, return to initial conditions; 6.5-8 min, equilibration of the column for both ionization modes. The optimized chromatographic conditions enabled the attainment of low instrumental detection limits (0.004-0.990 µg/L) for all the EDCs, except ethinylestradiol, bisphenol B, and bisphenol S [Supplementary Table 1].
Validation of the method
The upgraded analytical methodology was validated in terms of linearity, recoveries, precision, and method detection limits (MDL) and quantification limits (MQL), using laundry greywater, in order to evaluate the method performance. The recoveries were evaluated by spiking the samples with a standard mixture of all EDCs in methanol:water (20:80, v/v) at a final concentration of 2 µg/L. Recoveries were made in triplicate. MDL and MQL were calculated in spiked samples (n = 3) of La-GW as the minimum amount detectable of analyte with a signal-to-noise ratio of 3 and 10, respectively. Quality parameters are shown in Table 3.
Quality parameters of the upgraded instrumental methodology in laundry greywater, including recovery expressed in percentage (%), method detection limit (MDL) and quantitation limit (MQL), expressed in ng/L, linearity (R2), matrix effect (ME) expressed in percentage (%) and the internal standard used for their quantification
Class | Compound | Recovery (%) | RSD (%) | MDL (ng/L) | MQL (ng/L) | Linearity (R2) | ME (%) | Internal Standard |
Hormones | Diethylstilbesterol | 78 | 12.8 | 10.9 | 36.5 | 0.9961 | -100 | Bisphenol A-d4 (BPA-d4) |
Estradiol | 105 | 16.4 | 23.6 | 78.6 | 0.9974 | -98 | Estradiol-d2 | |
Estriol | 110 | 11.1 | 15.2 | 50.6 | 0.9967 | -89 | Estrone-d4 | |
Estrone | 102 | 16.9 | 13.2 | 44.1 | 0.9910 | -93 | Estrone-d4 | |
Estrone-3-sulfate | 97 | 10.7 | 8.94 | 29.8 | 0.9969 | -13 | Estrone-d4 | |
Ethinylestradiol | 104 | 5.67 | 27.3 | 91.1 | 0.9924 | -72 | Ethinylestradiol-d4 | |
Progesterone | 114 | 11.4 | 21.6 | 72.1 | 0.9925 | -97 | Progesterone-d9 | |
Antimicrobial | Triclosan | 93 | 6.79 | 29.1 | 97.0 | 0.9974 | -50 | Triclosan-d3 |
Preservatives | Benzylparaben | 103 | 8.01 | 6.94 | 23.1 | 0.9988 | -79 | Methylparaben-d4 |
Ethylparaben | 114 | 12.7 | 3.94 | 13.1 | 0.9996 | -43 | Methylparaben-d4 | |
Methylparaben | 99 | 3.29 | 29.0 | 96.7 | 0.9996 | -15 | Methylparaben-d4 | |
Propylparaben | 63 | 19.8 | 19.5 | 64.8 | 0.9994 | -98 | Methylparaben-d4 | |
Plasticizers | Bisphenol A (BPA) | 103 | 10.7 | 25.7 | 85.5 | 0.9985 | -99 | BPA-d4 |
Bisphenol AF (BPAF) | 68 | 18.8 | 105 | 349 | 0.9930 | -99 | BPA-d4 | |
Bisphenol B (BPB) | 93 | 14.4 | 131 | 438 | 0.9839 | -99 | Bisphenol B-d8 (BPB-d8) | |
Bisphenol F (BPF) | 81 | 15.2 | 141 | 469 | 0.9990 | -85 | BPA-d4 | |
Bisphenol S (BPS) | 94 | 14.7 | 19.2 | 64.1 | 0.9943 | -61 | BPA-d4 | |
Stimulant | Caffeine | 93 | 5.17 | 27.0 | 90.0 | 0.9966 | -99 | Caffeine-d3 |
Alkylphenolic compounds | Nonylphenol | 90 | 2.97 | 8.05 | 26.8 | 0.9995 | 106 | Nonylphenol-d4 |
Octylphenol | 146 | 6.00 | 17.1 | 57.0 | 0.9998 | -4 | Octylphenol-d17 | |
Anticorrosives | 1H-benzotriazole | 72 | 13.6 | 54.5 | 181 | 0.9994 | -99 | 1H-benzotriazole-d4 |
Tolyltriazole | 94 | 7.12 | 6.59 | 22.0 | 0.9934 | -99 | 1H-benzotriazole-d4 | |
Organophosphorus flame retardants | Tris(2-butoxyethyl)phosphate (TBEP) | 123 | 10.1 | 0.27 | 0.92 | 0.9963 | -94 | Triphenyl phosphate-d15 (TCPP-d15) |
Tris(2-chloroethyl)phosphate (TCEP) | 109 | 3.01 | 3.43 | 11.4 | 0.9991 | -94 | TCPP-d15 | |
Tris(chloroisopropyl)phosphate (TCPP) | n.r. | n.r. | n.r. | n.r. | - | - | TCPP-d15 |
To calculate the analyte concentrations, a matrix-matched calibration curve, together with an internal standard, was used for laundry greywater to overcome the pronounced matrix effect of this type of greywater. The obtained recoveries ranged from 63% to 123% (excluding octylphenol), which was within acceptable limits for a multi-residue method (60%-140%)[36]. Thus, the compounds with the lowest recovery values in laundry greywater were propylparaben (63%) and bisphenol AF (BPAF) (68%), while only octylphenol exceeded acceptable recovery values (146%). In all cases, the coefficient of determination was higher than 0.99 (except for BPB). It is important to clarify that TCPP is indicated in Table 3 as not recovered, due to a punctual equipment contamination incident during the method validation process and, thus, this contamination prevented the accurate quantification of TCPP. Precision was determined as the absolute value of the coefficient variation, which was acceptable for all compounds (RSD lower than 20%).
MDLs ranged from 0.3 to 141 ng/L and MQLs were from 0.9 to 469 ng/L. In comparison to previous studies, Leal et al. reported higher MQLs for triclosan (0.21 µg/L) and parabens (0.23-3.19 µg/L) in greywater samples using GC-MS/MS, whereas their values were comparable to BPA (0.08 µg/L)[40]. Nevertheless, given the limited data available in literature on laundry matrix, or even greywater in general, our validation results were compared with wastewater matrix, because it is the most comparable option. For hormones, our MDLs were comparable to values obtained using online LC-MS/MS in wastewater matrix (0.5-20 ng/L)[41] and lower than those obtained by GC-MS (11-60 ng/L)[42]. Similarly, MDLs for BPA were comparable to an analysis by GC-MS/MS (15-27 ng/L)[32,43] and lower than GC-MS (64 ng/L)[44], while they were higher for BPB (4 ng/L) and bisphenol F (BPF) (5 ng/L). Regarding parabens and alkylphenols, their MDLs were also comparable to the values obtained by Senta et al.[20] using online LC-MS/MS and higher compared to Gorga et al.[21].
The matrix effect (ME), which could induce interferences in the measurements of the target analytes, was evaluated for La-GW, the most complex matrix of this study. The degree of the matrix effect (ion suppression or enhancement) was evaluated using Equation 2[45].
where the Areamatrix is the peak area of the analyte in the spiked extract of La-GW sample, the Areasolvent is the peak area of the analyte in methanol:water (20:80, v/v), and Areablanc-matrix is the peak area of the analyte in the non-spiked La-GW sample.
Concerning the value of ME determined, if the result is less than 0 (negative value), there is a suppression in the response of the analyte, and if the value is positive, there is an enhancement. It can be observed that in all the analyzed compounds, there is a suppression of the signal except in the case of nonylphenol, which undergoes an enhancement (106%). As a general rule[46], if the value exceeds 20% of ME, it means that the effect of ME is interfering with the calculation of the concentrations. Therefore, measures should be taken to compensate for these effects and to obtain the best results, such as the use of matrix-matched calibration curves together with internal standards. In this study, estrone-3-sulfate (-13%), methylparaben (-15%), and octylphenol (-4%) were the compounds considered to have no matrix effect.
With respect to the calculations of the recoveries of each analyte by both calibration curves (CCD and CCM), an improvement is observed in the values using the calibration curve in the same matrix as the greywater samples, where most of the recoveries are within the acceptable range of 80%-120%. So, matrix-matched calibration curves, together with internal standards, were considered the best option for the quantification of endocrine disrupting compounds in La-GW.
It is important to note that for analytes, such as estradiol, ethinylestradiol, progesterone, methylparaben, BPA, 1H-benzotriazole, and caffeine, which have their own deuterated compound as internal standard, the calculated recoveries from both calibration curves are within the acceptable ranges, because the internal standard was enough to overcome the matrix effect. It is also true that there are compounds with their corresponding deuterated analyte that could not be well calculated with the calibration curve in solvent, for instance, the recovery of estrone and octylphenol calculated using the calibration curve in solvent was found to be above the acceptable limits (520% and 1,400%, respectively). Considering that octylphenol and its internal standard elute at different retention times (4.30 and 2.71 min, respectively), it seems plausible that octylphenol-d17 might not exactly mimic the target analyte behavior, especially in complex sample matrices such as laundry greywater. Nevertheless, acceptable recoveries for both compounds were obtained when matrix-matched calibration curve was used for quantification [Table 3].
Filtration test
Usually, water samples are prefiltered with 0.2-0.5 µm filters to eliminate suspended particulate material and, therefore, avoid clogging the cartridges during the solid-phase extraction (SPE), which is generally the subsequent step[47], thus reducing matrix interferences. However, the filtration step can cause the loss of some target compounds because they may be retained in the filter itself or sorbed to the suspended particulate matter. To prevent this, some authors rinse the filter with methanol to remove the target analytes retained on the filter. Other authors suggest using centrifugation as an alternative or in addition to filtration, although there is the possibility that suspended particles may co-precipitate[3]. The addition of diatomaceous earth is also used to prevent filter clogging[48]. In any case, the selection of the filter should be considered based on the selected target compounds.
The most common filters used in monitoring studies of water matrices for the analysis of emerging contaminants are glass fiber[2,49] and PVDF[38,50], followed by other materials such as nylon[51], cellulose acetate[52] or cellulose nitrate[53]. Indeed, the filtration step depends on the filter material, the chemistry of the matrix, and the contact time[54]. Therefore, several studies have been published evaluating the loss of microcontaminants due to the filtration step in different matrices, such as ultrapure water[20,55], seawater[56], or wastewater[57,58]. However, no studies have been found in the literature regarding the use of laundry greywater matrices. Thus, four types of filter materials were tested in this study: nylon, cellulose acetate, glass fiber, and PVDF. The mass losses of analytes during the filtration step were calculated in laundry greywater spiked with the target EDCs to evaluate the performance of the filters (see results in Figure 1).
Figure 1. Analyte mass recovered (%) of the selected EDCs for the different filter types used in this study. BPA: Bisphenol A; BPAF: bisphenol AF; BPB: bisphenol B; BPF: bisphenol F; BPS: bisphenol S; PVDF: polyvinylidene fluoride; TBEP: tris(2-butoxyethyl)phosphate; TCEP:
To understand the behavior of the compounds based on their mass loss depending on the type of filter used, a comparison has been made with different properties of the studied compounds (see values in Supplementary Table 2). Thus, with respect to the octanol/water distribution coefficient (Kow) - is defined as the ratio of the concentration of a chemical in n-octanol and water at equilibrium at a specified temperature -, compounds are classified as hydrophobic (log Kow > 1) or hydrophilic (log Kow < 1)[59]. However, no relationship has been observed between the mass loss and the Kow values for each of the filter materials. There was no correlation between the molecular weight of contaminants and their retention by filters.
Compounds in laundry greywater filtered by nylon filters showed around 100% recovery for a high number of compounds, being the best filter for anticorrosives and organophosphorus flame retardants. However, less than 20% recovery was achieved for 10 out of 25 studied contaminants, e.g., some bisphenols such as BPS (6%) and BPF (5%) or some hormones estradiol (1%) and estrone (2%). On the other hand, contaminant loss during filtration through glass fiber and cellulose acetate was not as significant as in the case of nylon, and only estriol (59%) and estrone-3-sulfate (57%) in the case of glass fiber and progesterone (18%) for cellulose acetate, recovery values were below 60%. PVDF filters exhibited the best performance for all the selected compounds (recovery higher than 60%), with recoveries higher than 80% for 16 compounds. Additionally, PVDF filters exhibited the highest reproducibility with the lowest standard deviation. Therefore, PVDF filters were chosen as the optimal choice for the sample filtration of laundry greywater. These findings are in agreement with those by Walker and Watson[60], who also carried out a filter test for the analysis of estrone, estradiol, and ethinylestradiol. Nylon filters retained a significant amount of the studied compounds, while cellulose acetate and PVDF showed the best results. Similarly, Sun et al. concluded that PVDF was the most suitable filter material for analyzing BPA, 4-t-octylphenol, nonylphenol and 4-nonylphenol in water samples[61].
Application to real samples
The optimized UHPLC-MS/MS methodology exhibited wide applicability, allowing the successful analysis of EDCs in various types of greywater, including greywater from a laundry facility in Girona (La-GW), greywater from showers/sinks of a hotel (Sh-GW), and greywater from a kitchen sink of a research center (Ki-GW). The selection of different greywater types enables an examination of their diverse characteristics in terms of usage and pollutant content, providing a comprehensive outlook on the versatility of the optimized analytical methodology across common greywater sources. Greywater composition of the three different greywaters is shown in Supplementary Table 3. Table 4 summarizes the concentrations of the selected EDCs detected in the three types of greywater investigated in this study.
Concentrations (± SD) of the selected endocrine disrupting compounds (EDCs) in the three types of greywater studied, expressed in ng/L
Class | Compound | Concentration ± SD (ng/L) | ||
Sh-GW | Ki-GW | La-GW | ||
Hormones | Diethylstilbesterol | < MDL | n.r. | < MDL |
Estradiol | < MDL | < MDL | < MDL | |
Estriol | < MDL | < MDL | < MQL | |
Estrone | < MDL | < MDL | < MDL | |
Estrone-3-sulfate | < MDL | < MDL | < MDL | |
Ethinylestradiol | < MQL | < MDL | < MQL | |
Progesterone | < MDL | < MDL | < MQL | |
Antimicrobial | Triclosan | n.r. | < MQL | 511 ± 30 |
Preservatives | Benzylparaben | < MDL | < MDL | < MDL |
Ethylparaben | < MDL | n.r. | 227 ± 31 | |
Methylparaben | < MDL | 379 ± 28 | 1,607 ± 122 | |
Propylparaben | < MDL | n.r. | 264 ± 68 | |
Plasticizers | Bisphenol A (BPA) | 104 ± 57 | < MDL | 262 ± 44 |
Bisphenol AF (BPAF) | < MDL | n.r. | < MDL | |
Bisphenol B (BPB) | < MDL | n.r. | 293 ± 122 | |
Bisphenol F (BPF) | < MQL | n.r. | < MDL | |
Bisphenol S (BPS) | < MDL | n.r. | 324 ± 120 | |
Stimulant | Caffeine | 670 ± 12 | > 40,000 | 2,360 ± 241 |
Alkylphenolic compounds | Nonylphenol | < MDL | < MDL | < MDL |
Octylphenol | n.r. | n.r. | < MQL | |
Anticorrosives | 1H-benzotriazole | < MDL | < MQL | 776 ± 47 |
Tolyltriazole | < MDL | < MDL | 127 ± 54 | |
Organophosphorus flame retardants | Tris(2-butoxyethyl)phosphate (TBEP) | n.r. | n.r. | < MDL |
Tris(2-chloroethyl)phosphate (TCEP) | n.r. | n.r. | < MDL | |
Tris(chloroisopropyl)phosphate (TCPP) | n.r. | n.r. | n.r. |
It should be considered that the concentrations of Sh-GW and Ki-GW were calculated with the calibration curve in solvent (methanol:water 20:80, v/v), while in the case of La-GW were calculated with matrix-matched calibration curve because of the strong matrix effects. Although Sh-GW and Ki-GW are considered less complex greywater matrices than laundry greywater, four and 11 out of 25 target analytes could not be quantified, respectively, probably due to matrix effect. Thus, it is recommended to use matrix-matched calibration curve in multi-residue analysis for greywater analysis in order to enable the quantification of all the target analytes. On the other hand, using the matrix-matched calibration curve for La-GW, the sensitivity of the analytical methodology was improved due to the correction of the matrix effect. However, the limits of detection were still high for some analytes (e.g., bisphenols) compared to the other two types of greywater analyzed (Sh-GW and Ki-GW, see Supplementary Table 4).
Only four out of 25 target EDCs analyzed were detected in Sh-GW, namely caffeine, ethinylestradiol, BPF, and BPA. In the case of Ki-GW, methylparaben was the only compound quantified, while caffeine was present at concentrations above the upper linear quantification range (40,000 ng/L), and triclosan and
Parabens are compounds commonly found in greywater due to their use as preservatives in personal care products. Short-chain parabens (methyl, ethyl, and propyl) are among the most commonly reported and were detected in bathrooms[62], showers and hand basins[63], and salon and laundry greywater samples[64]. In this study, parabens were not found in Sh-GW, contrary to what was described by Andersen et al., who found levels of parabens up to 40 µg/L in shower greywater[63]. In the case of Ki-GW, methylparaben was the only paraben identified at a concentration of 360 ng/L, which could be explained by its use as a preservative in food and pharmaceuticals[62]. Three types of parabens were also detected in La-GW, namely methylparaben, ethylparaben, and propylparaben. From those, methylparaben showed the highest concentration (1,607 ng/L), which is not surprising since this is the most common paraben[2]. Dwumfour-Asare et al. detected methylparaben in laundry greywater as well, but at higher levels (up to 1.8 mg/L), while the other parabens were not detected[64]. Caffeine was the only target analyte detected in all types of greywater, though it could be only quantified in Sh-GW and La-GW, reaching concentrations up to 670 and 2,360 ng/L, respectively [Table 4]. The observed results can be attributed to the fact that the laundry facility from which the samples were collected serves various restaurants as clients. Hence, the presence of caffeine in the greywater samples could be linked to its release from coffee stains present on the clothes of these establishments. Furthermore, caffeine is commonly used in skin products as an antioxidant[65], and consequently, after the application of skin products, caffeine can be transferred to clothes and later transferred to laundry greywater during the wash of the clothes. Different studies detected caffeine in greywater samples, however, at higher concentrations than in this study. For instance, Zraunig et al. detected 16.7 µg/L of caffeine in greywater of the showers and washbasins of a Mediterranean tourist facility[34], while Turner et al. found up to 450 µg/L of caffeine in greywater discharged from the bathrooms, laundry and kitchen of a residential area in Australia[49]. The presence of BPA was only reported in La-GW and Sh-GW at concentrations of 262 and 104 ng/L, respectively [Table 4]. Noutsopoulos et al. detected similar concentrations of BPA in greywater produced in Greek households, which followed the same trend observed in this study[32]. Triclosan is a synthetic antimicrobial agent used in many soaps, laundry detergents, and cosmetics, although the EU restricted its maximum allowed concentration in personal care products due to adverse effects on human health[66]. In this study, triclosan was found in La-GW samples, showing a concentration of 511 ng/L, most probably because of the residues of detergents that can be found in this type of greywater. Furthermore, triclosan was detected in Ki-GW (< MQL). Finally, very few studies determined hormones in greywater samples, and none of them were specified by greywater type. In this study, only estriol and progesterone were detected in La-GW, but they were below the method quantification limit (< 50.6 and < 72.1 ng/L, respectively) and ethinylestradiol in Sh-GW (< 22.4 ng/L). Zraunig et al. detected similar levels of estrone, estradiol, progesterone and testosterone in a mixed greywater (lower than 20 ng/L)[34].
CONCLUSIONS
An upgraded analytical methodology based on SPE extraction followed by UHPLC-MS/MS was validated for the simultaneous analysis of 25 endocrine disrupting compounds, encompassing natural and synthetic hormones, antimicrobials, preservatives, plasticizers, stimulants, alkylphenolic compounds, anticorrosives and organophosphorus flame retardants in water. Numerous modifications have been introduced to the previous method employed by Jakimska et al.[33]. These changes encompass alterations in the LC column, mobile phase, column temperature, and the elution gradient. The upgraded methodology enables rapid, robust, and sensitive analysis of the selected EDCs in greywater, resulting in enhanced instrumental detection limits, improved separation, and enhanced peak shapes. The procedure was validated by assessing quality parameters, including accuracy, precision, and sensitivity, specifically in laundry greywater. Recoveries ranging from 63% to 146% were achieved, with MDLs and MQLs from 0.3 to 141 ng/L and 0.9 to 469 ng/L, respectively, while the RSD values remained below 20%. An evaluation of the matrix effect in
The methodology was successfully applied to three different greywater types: laundry greywater (La-GW), shower greywater (Sh-GW), and kitchen sink greywater (Ki-GW), showing the occurrence of different EDCs, including hormones, antimicrobials, preservatives, plasticizers, stimulants, alkylphenolic compounds, and anticorrosives, at levels that can reach some hundreds of ng/L.
DECLARATIONS
Acknowledgments
The authors thank Maria Prats from GRUPFRN for her support in collecting the laundry greywater samples.
Authors’ contributions
Conceptualization, methodology, validation, formal analysis, writing original draft: Turull M
Administrative, technical, material support and writing review and editing: Buttiglieri G
Formal analysis and interpretation of data: Vazquez V
Conceptualization, writing review and editing, supervision, administrative, technical, and material support: Rodriguez-Mozaz S
Conceptualization, methodology, writing review and editing: Santos LHMLM
Availability of data and materials
Data are available upon request.
Financial support and sponsorship
The authors would like to thank the project LIFE-RECYCLO (LIFE20 ENV/RT/000205), and the contribution of the LIFE financial instrument of the European Community. Buttiglieri G acknowledges Spanish State Research Agency of the Spanish Ministry of Science, Innovation and Universities for the Grant to the Creation of a permanent position RYC-2014-16754. Vazquez V acknowledges the support and funding provided by the Agencia Nacional de Investigación e Innovación (ANII), from Uruguay, by granting financial assistance to her stay at ICRA. The authors also acknowledge the support from the Economy and Knowledge Department of the Catalan Government through a Consolidated Research Group (ICRA ENV- 2021 SGR 01282 and ICRA-TECH - 2021 SGR 01283). The Ultra-Performance Liquid Chromatography Triple Quadrupole Mass spectrometry (UPLC-MS) hybrid Linear Ion Trap (LIT), Acquity UPLC-MS QTRAP 5500, Waters-SCIEX facility received support from the CERCA Institute through the CERCAGINYS program, funded by the Spanish Ministry of Science and Innovation.
Conflicts of interest
All authors declared that there are no conflicts of interest.
Ethical approval and consent to participate
Not applicable.
Consent for publication
Not applicable.
Copyright
© The Author(s) 2023.
Supplementary Materials
REFERENCES
1. Friedler E, Hadari M. Economic feasibility of on-site greywater reuse in multi-storey buildings. Desalination 2006;190:221-34.
2. Vieira WT, de Farias MB, Spaolonzi MP, da Silva MGC, Vieira MGA. Endocrine-disrupting compounds: occurrence, detection methods, effects and promising treatment pathways - a critical review. J Environ Chem Eng 2021;9:104558.
3. Locatelli M, Sciascia F, Cifelli R, Malatesta L, Bruni P, Croce F. Analytical methods for the endocrine disruptor compounds determination in environmental water samples. J Chromatogr A 2016;1434:1-18.
4. Shaikh IN, Ahammed MM. Quantity and quality characteristics of greywater: a review. J Environ Manage 2020;261:110266.
5. Rogowska J, Cieszynska-Semenowicz M, Ratajczyk W, Wolska L. Micropollutants in treated wastewater. Ambio 2020;49:487-503.
6. Lazofsky A, Buckley B. Recent trends in multiclass analysis of emerging endocrine disrupting contaminants (EDCs) in drinking water. Molecules 2022;27:8835.
7. Auriol M, Filali-meknassi Y, Tyagi RD, Adams CD, Surampalli RY. Endocrine disrupting compounds removal from wastewater, a new challenge. Process Biochem 2006;41:525-39.
8. Aris AZ, Shamsuddin AS, Praveena SM. Occurrence of 17α-ethynylestradiol (EE2) in the environment and effect on exposed biota: a review. Environ Int 2014;69:104-19.
9. Esteban S, Gorga M, González-Alonso S, Petrovic M, Barceló D, Valcárcel Y. Monitoring endocrine disrupting compounds and estrogenic activity in tap water from Central Spain. Environ Sci Pollut Res 2014;21:9297-310.
10. Omar TFT, Ahmad A, Aris AZ, Yusoff FM. Endocrine disrupting compounds (EDCs) in environmental matrices: review of analytical strategies for pharmaceuticals, estrogenic hormones, and alkylphenol compounds. TrAC Trends Anal Chem 2016;85:241-59.
11. Sun C, Zhang T, Zhou Y, et al. Triclosan and related compounds in the environment: recent updates on sources, fates, distribution, analytical extraction, analysis, and removal techniques. Sci Total Environ 2023;870:161885.
12. Raman DR, Williams EL, Layton AC, et al. Estrogen content of dairy and swine wastes. Environ Sci Technol 2004;38:3567-73.
13. Filippe TC, de Almeida Brehm Goulart F, Mizukawa A, Rodrigues de Azevedo JC. Validation of analytical methodology for determination of personal care products in environmental matrix by GC-MSMS. Eclet Quim J 2018;43:30-6.
14. Casas Ferreira AM, Möder M, Fernández Laespada ME. GC-MS determination of parabens, triclosan and methyl triclosan in water by in situ derivatisation and stir-bar sorptive extraction. Anal Bioanal Chem 2011;399:945-53.
15. Azzouz A, Colón LP, Hejji L, Ballesteros E. Determination of alkylphenols, phenylphenols, bisphenol A, parabens, organophosphorus pesticides and triclosan in different cereal-based foodstuffs by gas chromatography-mass spectrometry. Anal Bioanal Chem 2020;412:2621-31.
16. Reichert G, Mizukawa A, Antonelli J, de Almeida Brehm Goulart F, Filippe TC, Rodrigues de Azevedo JC. Determination of parabens, triclosan, and lipid regulators in a subtropical urban river: effects of urban occupation. Water Air Soil Pollut 2020;231:133.
17. Pastor-Belda M, Drauschke T, Campillo N, et al. Dual stir bar sorptive extraction coupled to thermal desorption-gas chromatography-mass spectrometry for the determination of endocrine disruptors in human tissues. Talanta 2020;207:120331.
18. Campíns-Falcó P, Verdú-Andrés J, Sevillano-Cabeza A, Herráez-Hernández R, Molins-Legua C, Moliner-Martinez Y. In-tube solid-phase microextraction coupled by in valve mode to capillary LC-DAD: improving detectability to multiresidue organic pollutants analysis in several whole waters. J Chromatogr A 2010;1217:2695-702.
19. Pellegrino Vidal RB, Ibañez GA, Escandar GM. A green method for the quantification of plastics-derived endocrine disruptors in beverages by chemometrics-assisted liquid chromatography with simultaneous diode array and fluorescent detection. Talanta 2016;159:336-43.
20. Senta I, Rodríguez-Mozaz S, Corominas L, Covaci A, Petrovic M. Applicability of an on-line solid-phase extraction liquid chromatography - tandem mass spectrometry for the wastewater-based assessment of human exposure to chemicals from personal care and household products. Sci Total Environ 2022;845:157309.
21. Gorga M, Petrovic M, Barceló D. Multi-residue analytical method for the determination of endocrine disruptors and related compounds in river and waste water using dual column liquid chromatography switching system coupled to mass spectrometry. J Chromatogr A 2013;1295:57-66.
22. Iyer AP, Xue J, Honda M, et al. Urinary levels of triclosan and triclocarban in several Asian countries, Greece and the USA: association with oxidative stress. Environ Res 2018;160:91-6.
23. Goeury K, Munoz G, Vo Duy S, Prévost M, Sauvé S. Occurrence and seasonal distribution of steroid hormones and bisphenol A in surface waters and suspended sediments of Quebec, Canada. Environ Adv 2022;8:100199.
24. Silveira RS, Rocha BA, Rodrigues JL, Barbosa Jr. F. Rapid, sensitive and simultaneous determination of 16 endocrine-disrupting chemicals (parabens, benzophenones, bisphenols, and triclocarban) in human urine based on microextraction by packed sorbent combined with liquid chromatography tandem mass spectrometry (MEPS-LC-MS/MS). Chemosphere 2020;240:124951.
25. Mahalakshmi R, Pugazhendhi A, Brindhadevi K, Ramesh N. Analysis of Alkylphenol ethoxylates (APEOs) from tannery sediments using LC-MS and their environmental risks. Process Biochem 2020;97:37-42.
26. Liu S, Huang X, Hu K, Jin Q, Zhu G. Development of a multiresidue method for endocrine-disrupting pesticides by solid phase extraction and determination by UHPLC-MS/MS from drinking water samples. J Chromatogr Sci 2020;58:195-202.
27. Wilk BK, Fudala-Ksiazek S, Szopińska M, Luczkiewicz A. Landfill leachates and wastewater of maritime origin as possible sources of endocrine disruptors in municipal wastewater. Environ Sci Pollut Res 2019;26:25690-701.
28. Grobin A, Roškar R, Trontelj J. A robust multi-residue method for the monitoring of 25 endocrine disruptors at ultra-trace levels in surface waters by SPE-LC-MS/MS. Anal Methods 2023;15:2606-21.
29. Wang Y, Li G, Zhu Q, Liao C. A multi-residue method for determination of 36 endocrine disrupting chemicals in human serum with a simple extraction procedure in combination of UPLC-MS/MS analysis. Talanta 2019;205:120144.
30. Palmquist H, Hanaeus J. Hazardous substances in separately collected grey- and blackwater from ordinary Swedish households. Sci Total Environ 2005;348:151-63.
31. Zedek S, Bressy A, Deshayes S, Eudes V, Caupos E, Moilleron R. Triclosan, triclocarban and parabens in greywater: identification of their sources. In: 26th annual meeting of Society of Environmental Toxicology and Chemistry; 2016 May 22-26; Nantes, France. Springer; 2016. Available from: https://hal.science/hal-01699879. [Last accessed on 21 Aug 2023].
32. Noutsopoulos C, Andreadakis A, Kouris N, et al. Greywater characterization and loadings - physicochemical treatment to promote onsite reuse. J Environ Manage 2018;216:337-46.
33. Jakimska A, Huerta B, Bargańska Ż, Kot-Wasik A, Rodríguez-Mozaz S, Barceló D. Development of a liquid chromatography-tandem mass spectrometry procedure for determination of endocrine disrupting compounds in fish from Mediterranean rivers. J Chromatogr A 2013;1306:44-58.
34. Zraunig A, Estelrich M, Gattringer H, et al. Long term decentralized greywater treatment for water reuse purposes in a tourist facility by vertical ecosystem. Ecol Eng 2019;138:138-47.
35. Gros M, Rodríguez-Mozaz S, Barceló D. Fast and comprehensive multi-residue analysis of a broad range of human and veterinary pharmaceuticals and some of their metabolites in surface and treated waters by ultra-high-performance liquid chromatography coupled to quadrupole-linear ion trap tandem mass spectrometry. J Chromatogr A 2012;1248:104-21.
36. European Commission. Method validation and quality control procedures for pesticides residues analysis in food and feed. Available from: https://www.eurl-pesticides.eu/library/docs/allcrl/AqcGuidance_Sanco_2009_10684.pdf. [Last accessed on 21 Aug 2023]
37. Mirmont E, Bœuf A, Charmel M, et al. Development and implementation of an analytical procedure for the quantification of natural and synthetic steroid hormones in whole surface waters. J Chromatogr B 2021;1175:122732.
38. Gusmaroli L, Insa S, Petrovic M. Development of an online SPE-UHPLC-MS/MS method for the multiresidue analysis of the 17 compounds from the EU “Watch list”. Anal Bioanal Chem 2018;410:4165-76.
39. Su L, Khunjar WO, Aga DS. Analysis of trace organic pollutants in wastewater to assess biodegradation using wrong-way-round ionization in liquid chromatography/tandem mass spectrometry. Rapid Commun Mass Spectrom 2014;28:1265-72.
40. Leal LH, Vieno N, Temmink H, Zeeman G, Buisman CJ. Occurrence of xenobiotics in gray water and removal in three biological treatment systems. Environ Sci Technol 2010;44:6835-42.
41. Vaudreuil MA, Vo Duy S, Munoz G, Sauvé S. Pharmaceutical pollution of hospital effluents and municipal wastewaters of Eastern Canada. Sci Total Environ 2022;846:157353.
42. Pessoa GP, de Souza NC, Vidal CB, et al. Occurrence and removal of estrogens in Brazilian wastewater treatment plants. Sci Total Environ 2014;490:288-95.
43. Hernando MD, Mezcua M, Gómez MJ, Malato O, Agüera A, Fernández-Alba AR. Comparative study of analytical methods involving gas chromatography-mass spectrometry after derivatization and gas chromatography-tandem mass spectrometry for the determination of selected endocrine disrupting compounds in wastewaters. J Chromatogr A 2004;1047:129-35.
44. Kiejza D, Kotowska U, Polińska W, Karpińska J. USAEME-GC/MS method for easy and sensitive determination of nine bisphenol analogues in water and wastewater. Molecules 2022;27:4977.
45. Gineys N, Giroud B, Vulliet E. Analytical method for the determination of trace levels of steroid hormones and corticosteroids in soil, based on PLE/SPE/LC-MS/MS. Anal Bioanal Chem 2010;397:2295-302.
46. European Commission. Analytical quality control and method validation procedures for pesticide residues analysis in food and feed. Available from: https://www.eurl-pesticides.eu/userfiles/file/eurlall/aqcguidance_sante_2019_12682.pdf. [Last accessed on 21 Aug 2023]
47. Comerton AM, Andrews RC, Bagley DM. Practical overview of analytical methods for endocrine-disrupting compounds, pharmaceuticals and personal care products in water and wastewater. Philos Trans A Math Phys Eng Sci 2009;367:3923-39.
48. Atkinson SK, Marlatt VL, Kimpe LE, Lean DRS, Trudeau VL, Blais JM. The occurrence of steroidal estrogens in south-eastern Ontario wastewater treatment plants. Sci Total Environ 2012;430:119-25.
49. Turner RDR, Warne MSJ, Dawes LA, Thompson K, Will GD. Greywater irrigation as a source of organic micro-pollutants to shallow groundwater and nearby surface water. Sci Total Environ 2019;669:570-8.
50. Čelić M, Škrbić BD, Insa S, Živančev J, Gros M, Petrović M. Occurrence and assessment of environmental risks of endocrine disrupting compounds in drinking, surface and wastewaters in Serbia. Environ Pollut 2020;262:114344.
51. Liao C, Kannan K. A survey of alkylphenols, bisphenols, and triclosan in personal care products from China and the United States. Arch Environ Contam Toxicol 2014;67:50-9.
52. Baek SS, Yun D, Pyo J, Kang D, Cho KH, Jeon J. Analysis of micropollutants in a marine outfall using network analysis and decision tree. Sci Total Environ 2022;806:150938.
53. Zhang Q, Jekel M, Zhang Y, Ruhl AS. A quick test method for predicting the adsorption of organic micropollutants on activated carbon. Water Res 2022;226:119217.
54. Kumar R, Adhikari S, Halden RU. Comparison of sorption models to predict analyte loss during sample filtration and evaluation of the impact of filtration on data quality. Sci Total Environ 2022;817:152624.
55. Dong W, Yu X, Wang J, Sui Q. Is it the appropriate syringe filter? The loss of PPCPs during filtration by syringe filter. Water Emerg Contam Nanoplastics 2022;1:7.
56. Salgueiro-González N, Concha-Graña E, Turnes-Carou I, Muniategui-Lorenzo S, López-Mahía P, Prada-Rodríguez D. Blank and sample handling troubleshooting in ultratrace analysis of alkylphenols and bisphenol A by liquid chromatography tandem mass spectrometry. Talanta 2012;101:413-9.
57. Lath S, Knight ER, Navarro DA, Kookana RS, McLaughlin MJ. Sorption of PFOA onto different laboratory materials: filter membranes and centrifuge tubes. Chemosphere 2019;222:671-8.
58. Chen L, Lin H, Li H, Wang M, Qiu B, Yang Z. Influence of filtration during sample pretreatment on the detection of antibiotics and non-steroidal anti-inflammatory drugs in natural surface waters. Sci Total Environ 2019;650:769-78.
59. Hebig KH, Nödler K, Licha T, Scheytt TJ. Impact of materials used in lab and field experiments on the recovery of organic micropollutants. Sci Total Environ 2014;473-4:125-31.
60. Walker CW, Watson JE. Adsorption of estrogens on laboratory materials and filters during sample preparation. J Environ Qual 2010;39:744-8.
61. Sun M, Wu Q, Wang C, Wang Z. Thin-film microextraction for the preconcentration of some endocrine disrupting chemicals in aqueous samples before chromatographic analysis. Anal Methods 2014;6:6316-21.
62. Eriksson E, Andersen HR, Madsen TS, Ledin A. Greywater pollution variability and loadings. Ecol Eng 2009;35:661-9.
63. Andersen HR, Lundsbye M, Wedel HV, Eriksson E, Ledin A. Estrogenic personal care products in a greywater reuse system. Water Sci Technol 2007;56:45-9.
64. Dwumfour-Asare B, Adantey P, Nyarko KB, Appiah-Effah E. Greywater characterization and handling practices among urban households in Ghana: the case of three communities in Kumasi Metropolis. Water Sci Technol 2017;76:813-22.
65. Rodrigues R, Oliveira MBPP, Alves RC. Chlorogenic acids and caffeine from coffee by-products: a review on skincare applications. Cosmetics 2023;10:12.
Cite This Article

How to Cite
Download Citation
Export Citation File:
Type of Import
Tips on Downloading Citation
Citation Manager File Format
Type of Import
Direct Import: When the Direct Import option is selected (the default state), a dialogue box will give you the option to Save or Open the downloaded citation data. Choosing Open will either launch your citation manager or give you a choice of applications with which to use the metadata. The Save option saves the file locally for later use.
Indirect Import: When the Indirect Import option is selected, the metadata is displayed and may be copied and pasted as needed.
About This Article
Special Issue
Copyright
Data & Comments
Data
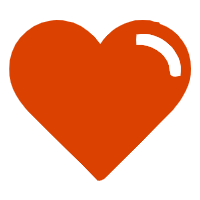
Comments
Comments must be written in English. Spam, offensive content, impersonation, and private information will not be permitted. If any comment is reported and identified as inappropriate content by OAE staff, the comment will be removed without notice. If you have any queries or need any help, please contact us at [email protected].