Modelling and spectroscopic investigation of 2,4-D adsorption in soil amended with pine sawdust, paunch grass and sewage sludge biochars
Abstract
2,4-Dichlorophenoxyacetic acid (2,4-D) is a widely used herbicide possessing high herbicidal activity; however, it is potentially toxic to humans and quite persistent in the environment. We investigated the adsorption of 2,4-D in two pasture soils amended with pine sawdust (PSD), paunch grass (PG) and sewage sludge (SS) biochars using batch studies. The results showed that PSD biochar produced at 700 oC exhibited the highest adsorption capacity for 2,4-D among the waste-derived biochars tested, which is 200-fold greater than the control and other biochar-amended soils. In general, the sorption affinity of 2,4-D for biochar amended soils followed an order: PSD > > PG > SS. The high adsorption capacity of the PSD may be attributed to its significantly higher specific surface area of 795 m2·g-1 as compared to other soils and biochars. Moreover, the results of the physicochemical characterization showed no difference in surface oxygen functional groups among the PSD, PG and SS. These findings indicate that oxygen-containing surface functional groups have a negligible role in 2,4-D adsorption, as evident from the spectroscopic investigation, thus emphasizing the role of surface area in the 2,4-D adsorption. Lastly, pore intrusion/filling, hydrogen bonding and π-π EDA interactions are postulated to be the plausible adsorption mechanisms for 2,4-D onto biochar amended soils.
Keywords
INTRODUCTION
Environmental contamination due to the wide application of pesticides in agriculture has become a matter of global concern because of the toxicity and persistence of these organic chemicals in soil and aquatic environment[1]. Although their occurrence in the environment has been reported for decades, some of their potential negative impacts on human health, animals, and ecosystems were recently recognized, which qualify some of the pesticides as emerging contaminants[2]. Among the herbicides, 2, 4-Dichlorophenoxyacetic acid (2,4-D, Cl2C6H3OCH2COOH) is widely used for plantation crops such as sugarcane, cocoa, and rubber[3]. It is less toxic to common grasses and can be manufactured at a low cost[4]. Due to its effectiveness and low cost, 2,4-D has been one of the most widely and frequently detected organic chemicals in the environment, including as an endocrine-disrupting compound[5-7]. 2,4-D is an ionizable (pKa at 25 oC = 3.4) chemical having high water solubility of 900 mg·L-1[8] and a low octanol/water partition coefficient (pH 7, 20 oC) of 0.015[9]. Therefore, the compound readily dissolves in rainwater and could potentially become highly mobile and transported into the soil environment during conditions conducive to leaching (i.e., heavy rainfall and storm events).
According to the World Health Organisation, 2,4-D is characterized as moderately toxic (class II) and potentially carcinogenic[10] to human beings and animals and has a drinking water limit of 70 μg/L[11]. 2,4-D has been extensively used in the cereal-growing regions of New Zealand (NZ) and comprised 40% of the total herbicide use in 1998 and a concentration level of 0.09 μg/L has been detected in groundwater of the Waikato region[12]. Since 2,4-D is openly sprayed, its residues can easily find their way into nearby water bodies, thus contaminating the media. It is to be noted that in NZ, the maximum allowable value for 2,4-D in water is set at 100 μg/L, and therefore no routine monitoring is conducted at present. However, prolonged exposure to low concentrations over an extended period could result in potential toxic effects on non-target organisms and human health. Past research has shown that the adsorption process dictates the mobility and bioavailability of organic compounds in a soil environment[13]; thus, the adsorption affinity of a 2,4-D compound is considered a governing process influencing its environmental fate.
Biochar, a carbon-rich solid product produced through the heating of organic biomass in an oxygen-limiting environment (pyrolysis), could be used as an engineered sorbent, mitigating the transport of these chemicals in-situ[14]. Biochar has been demonstrated to be effective in adsorbing various organic and inorganic compounds in aquatic and soil environments[15,16]. These past studies indicate that biochar addition to soil could potentially mitigate the movement of chemicals through the soil profile, thereby reducing their environmental risk to the receiving environment.
The sorptive ability of biochar is dependent on the feedstock source and pyrolysis conditions such as temperature, duration, etc.[15]. The physical and chemical properties that also play an important role in the adsorption process include specific surface area (SSA), pH, cation exchange capacity (CEC), surface heterogeneity, and chemical composition of the char[16,17]. Often, significant variations in the sorptive capacity have been observed between different biochars, suggesting that the adsorption affinity measured is unique to the biochar and could be attributed to the feedstock used. Plant-based biomass (hardwood, softwood, rice husks, seeds, and nuts) is the most common feedstock source used for producing biochar[18].
Studies have been conducted converting various waste products such as pine sawdust and sewage sludge, poultry litter, broiler litter, and dissolved flotation sludge from the chicken factory into biochars[19]. Additionally, several studies have been conducted to determine the adsorption affinity of biochars to various organic contaminants[20,21]. For herbicides, some authors used hardwood biochar, which could have other economic value than being used as a feedstock for biochar[22-24]. There is a lack of studies on the sorptive potential of biochar produced from other waste feedstocks such as pine sawdust, corncob, poultry litter, paunch grass (undigested grass from cow’s stomach), and sewage sludge for 2,4-D or related compounds. Though pine sawdust is regarded as a biomass source of stationary energy, it has lignocellulosic properties, which could be valuable in developing adsorbents. Paunch grass from the abattoir and sewage sludge production in wastewater treatment plants has a high cost associated with their disposal.
In a study by Jin et al. (2016), who investigated the adsorption potential of different biochar amended soils for pesticides, biochar application to soils was as high as 5% to 20% of soil weight[25]. This rate of biochar addition to soils seems to be unrealistic and unsustainable. For example, assuming a soil bulk density of 1,600 kg·m-3 and 0.1 m incorporation of biochar into topsoil, biochar application rate at 1% w/w basis would be 10-15 t·ha-1, so biochar amendment at 5% to 20% is not realistically feasible simply because a huge amount of biochar would be required. Also, for their adsorption experiments, the authors chose very high initial pesticide concentrations. Since partition coefficients are concentration-dependent, the Kd values reported in this study cannot be extrapolated to environmental concentrations that are often in the ng·kg-1 range. On the contrary, at 0.1% w/w basis, the pine needles pyrolyzed at 400 oC dominated the overall adsorption of naphthalene[26]. There have been a few instances where the authors used various carbon-based adsorbents to remediate the 2,4-D, such as ZnCl2 modified activated carbon[27] and red mud-carbon composite[28]. However, most of the adsorbents were chemically or physically modified to increase adsorption efficiency. Given the complexities associated with the large-scale production of adsorbents, no chemical modifications have been attempted to produce biochar for use in the study.
Therefore, the study investigated the key factors responsible for adsorption of pesticides onto biochar. Specifically, the study aims to: (1) determine the effects of feedstock on biochar production and on 2,4-D adsorption; and (2) elucidate the adsorption mechanisms of biochar amended soil for 2,4-D using a range of spectroscopic investigations. To achieve these objectives, we set out to explore the retention ability of two representative dairy farm soil amended with biochar produced from three diverse feedstock in the form of pine sawdust, paunch grass and sewage sludge using 2,4-D and employing laboratory batch adsorption studies. The central hypothesis was that biochar amended soils would enable the immobilization of 2,4-D and adsorption affinity for the compound would be dependent on types of biochar feedstock and the biochar’s inherent properties.
MATERIALS AND METHODS
Soils
Two topsoils (0-5 cm), Matawhero loam (Latitude: 38° 38’ 53” S; Longitude: 177° 56’ 24” E) and Te Kowhai silt loam (latitude: 37° 43’ 41” S; Longitude: 175° 9’ 0” E), regions of Hawke’s Bay and Hamilton, Waikato region of the North Island of New Zealand, were collected as representative samples of pasture growing. The samples were then dried in ambient conditions and sieved (< 2 mm). Properties of soils used in the study are listed in Table 1. The details of the soil and methods used to determine the physicochemical properties were based on the study of Srivnivasan et al.[19].
Selected properties of soils used in the adsorption study (Srinivasan et al.[19])
Soils | pH1:2 H2O | OC (%) | CEC (cmolc·kg-1) | Sand % | Silt % | Clay % | SSA (m2·g-1) | Exchangeable cations (cmolc·kg-1) | Clay mineralogy % | ||||||
Na | Mg | Al | K | Ca | Fe | Sia | |||||||||
Matawhero | 6.1 | 2.1 | 15.4 | 11 | 62 | 27 | 60 | 2.5 | 38.7 | 110.4 | 4.8 | 43.6 | 16.6 | 25.8 | q (5); f (3); 17 (i); mm (44); v (31) |
Te Kowhai | 6.7 | 5.0 | 22.3 | 9 | 54 | 37 | 32.5 | 0.6 | 4.0 | 205.2 | 0.7 | 8.7 | 27.0 | 38.8 | vg (50); ha (35); k (10); cr (4); f (1) |
Biochar
This study used biochars prepared from paunch grass (PG), sewage sludge (SS) and pinus radiata sawdust (PSD) and were produced by slow pyrolysis (residence time of 40 min) at 700 oC at Lakeland Steel Limited, Rotorua, NZ. The physicochemical properties of biochar used in this study are summarised in Table 2.
Chemical properties of biochar (produced at 700 oC) used in adsorption studies
Biochar | pH | Total C | Total | Total | Total | O/C | H/C | (O+N)/C | EC | Exchangeable cations* | Ash | SSA** | |||
H | N | O | cmol ( + ) kg-1 | ||||||||||||
(water) | (%) | (%) | (%) | (%) | (mS·cm-1) | Ca | Mg | K | Na | (%) | (m2·g-1) | ||||
Pine sawdust | 9.7 | 90.9 | 1.31 | 0.11 | 6.1 | 0.07 | 0.01 | 0.07 | 1.57 | 26.9 | 18.2 | 25.47 | 1.35 | 1.58 | 795 |
Paunch Grass | 8.8 | 64.79 | 2.28 | 1.8 | 12.4 | 0.19 | 0.035 | 0.22 | 8.61 | 1.06 | 0.12 | 0.39 | 0.29 | 28.73 | 1.96 |
Sewage sludge | 7.9 | 77.98 | 2.10 | 0.5 | 19.33 | 0.25 | 0.025 | 0.25 | 2.30 | 0.30 | 0.07 | 0.12 | 0.15 | 12.61 | 4.45 |
Biochar characterization
The biochars were ground to < 2 mm before soil amendment and pH of the biochars was measured using the standard pH meter (PHM62). In-House” conductance meter with a cell constant K = 0.69 cm-1 was used to measure the electrical conductivity (EC) of biochar samples. Briefly, a 1:10 ratio of biochar and water was shaken for 24 h using a rotary drum shaker. To determine the pH and EC, the mixture of biochar and water was centrifuged, and the supernatant was analyzed. Using proximate analysis, the elemental composition (C, H, N, S, O) and ash content of biochars were sent for analysis using a CHN analyzer to Campbell Microanalytical Laboratory (Dunedin, New Zealand). The inductively coupled plasma mass spectrometry (ICPMS) was used to determine the exchangeable cations of biochars, while Brunauer, Emmett and Teller (BET) using N2 adsorption isotherm method was used to measure the specific surface area (SSA) of biochars. The biochars were characterized by a range of properties, such as heavy metal content. The surface morphology was characterized using Scanning Electron Microscope and Energy Dispersive X-ray Spectroscopy (SEM-EDX), while the surface functional groups were determined using Fourier Transform Infrared Spectroscopy (FTIR). Additionally, Solid-state 13C Nuclear Magnetic Resonance (NMR) and X-ray Photoelectron Spectroscopy (XPS) were given as important scope in this characterization. The details of these NMR and XPS characterizations of the biochar were described by Srinvasan et al.[19].
Batch adsorption studies
The batch adsorption studies followed the experimental protocol developed earlier by Srinivasan et al.[19]. Briefly, a soil sample of 2 g was added to 35 mL-glass centrifuge tubes. The samples of soil and biochar (mass ratio: 2:35) in the centrifuge tubes sealed by screw caps lined with Teflon were vortexed for 30 s to homogenize the mixture. Soils were amended with the different types of biochars (sieved to < 2 mm) at 1.0% of soil weight based on biochar application rates of 10 t·ha-1, with assumed soil bulk density.
A 1000 mg·L-1 stock solution of 2,4-D (98% purity, Sigma Aldrich) was prepared in methanol. To prevent the photodegradation of 2,4-D, the bottle of stock solution was covered with aluminum foil and stored at
Extraction and analysis
After equilibration, for all biochar amended soils (except PSD), centrifugation at centrifuged at 1,750 × g for 10 min was done. Then, the supernatant was filtered using 0.45 μm syringe filters. The filtered samples were placed in amber glass vials for HPLC analysis. For the PSD biochar amended soil with high adsorption capacity, the final aqueous concentrations for some samples were below the detection limit of the instrument. Thus, the solvent extraction method was developed. Pure solvents, namely methanol, acetonitrile ( ≥ 99.8% purity), deionized water (HPLC grade), hexane, ethyl acetate ( ≥ 99.8% purity) and dichloromethane (DCM), were used as extraction solvents. The use of ethyl acetate instead of other solvents resulted in higher recoveries > 92% for the chemical. Twenty millilitres of supernatant was extracted with 10 mL ethyl acetate. Then, 1 mL of the extracted ethyl acetate was placed into an HPLC vial and evaporated for dryness under N2 gas. The remaining contents were reconstituted in 0.5 mL methanol and were immediately analyzed using HPLC.
Analysis of the adsorption affinity of 2,4-D herbicide to biochar amended soils was performed on a Dionex HPLC system comprising an automated sampler, a pump and a UV detector (240 nm wavelength). Chromatography was performed on a 150mm × 4.6 mm Luna (5 μm packing) RP-C18 column. The mobile phase consisted of acetonitrile and water at a ratio of 60:40. A flow rate of 0.8 mL/min and an injection volume of 20 μL were used for 2,4-D analysis with a retention time of 4.5 min.
All samples were analyzed at a wavelength of 240 nm. Using a signal-to-noise ratio of 3 to 1, the limits of detection (LOD) were determined. The LOD and limits of quantification (LOQ) for 2,4-D were 0.05 μg·mL-1 and 0.025 μg·mL-1, respectively. Calibration standards were developed simultaneously with experimental runs, and an R2 value of 0.99 was obtained. Blank matrix runs and mobile phase runs were regularly conducted for quality assurance. Soil blank runs carried out indicated no interference from co-eluting peaks, which indicated that the extraction steps performed in this study were sufficiently robust.
The following equation was used to determine the sorbed amount Cs [mg·kg-1]:
where Ci (mg·L-1) is the initial solute concentration, Cw (mg·L-1) is the concentration of solute at equilibrium, V (L) is the volume of solution, and Ms (kg) is the mass of soil, respectively.
Adsorption modelling
Adsorption of organic compounds in soil organic matter (SOM) rich soils generally follows the typical Freundlich isotherm[29]. This is also based on the assumption that Freundlich data assumes cations and anions are adsorbed onto the surface simultaneously. The model is further transformed by assuming N = 1 and termed as linear Freundlich model, and this is based on the assumption that adsorption occurs exclusively to the organic matter component of the soil[29].
Adsorption isotherms were modelled using the Freundlich model as below:
where Cs (mg·kg-1) is the sorbed concentration equilibrium; Cw (mg·L-1) is the concentration of aqueous phase; N is the empirical constant related to the adsorption intensity to the heterogeneity of the material; KF (mg1-N·LN·kg-1) is the Freundlich adsorption constant.
The amount of herbicide sorbed per unit of soil organic carbon, Koc, was calculated using the equation Koc = KF/OC%, where OC is the organic carbon content of the soil and Koc is defined as the soil organic carbon normalized partition coefficient (L·kg-1). To compare adsorption across different treatments, the concentration-dependent effective distribution coefficient (Kdeff = Kf CwN-1) was calculated where a concentration of 0.5 mg·L-1 was used. Similarly, the Koc for each biochar amended soil was calculated for
RESULTS AND DISCUSSION
Biochar and soil characterization
The chemical properties of biochars including elemental analysis, pH, surface area, and exchangeable ions, were summarized in Table 1, while soil’s physical and chemical properties were presented in Table 2. The PSD biochar was found to be having the highest carbon percentage of 90.9%, while PG biochar has the lowest of 64.79% fixed carbon. Moreover, PSD has the lowest H/C ratio (0.01) among the biochars, which indicates it is highly aromatized. On the contrary, PG has the highest H/C ratio (0.035), which indicates it has the lowest degree of carbonization and aromaticity. PSD biochar had the highest SSA value of 795 m2·g-1 as compared with other biochars. The exchangeable cation capacity for the biochars in terms of Ca2+, Mg2+, K+ and Na+ were presented in Table 2. The PSD biochar exhibited the highest exchangeable cation capacity, followed by PG and SS biochars. The trend in the increasing cation exchange capacity can be indicative of the number of exchangeable ions between adsorbent and adsorbate, which is further discussed in the mechanism section. The metal concentrations present in the biochar were analyzed by ICPMS and presented in Supplementary Table 1 along with threshold limits for soil amendment given by the International Biochar Initiative[30]. The results indicate that all the metal concentrations (Hg, Cu, Pb, Co, Cu, As) were below the range except for Zn2+ concentrations which were above the threshold limits
Surface morphology by SEM-EDX
SEM micrographs presented in Figure 1 show that the PSD, PG and SS biochars maintain the surface morphology of their biomass precursor. The results were expected as the biochar structure is a function of feedstock material. The morphology of the PSD from the SEM shows that the material has a smooth, glassy, elongated appearance and a non-porous surface. At the same time, porous structures were evident for the other biochars from the SEM imaging. The EDX point scans were analyzed; compositions are also presented in Figure 1. The EDX results were consistent with the elemental analysis findings, with the highest carbon of 93.5% found in PSD.
Surface functional groups by FTIR analysis
The surface functional groups present on the biochars were investigated by FTIR analysis, and corresponding spectrum as a function of wavenumber are presented in Figure 2. Overall, very similar functional groups were present on all biochars. A plausible explanation for this could be due to the production of the biochars at the same temperature. The results also indicate that the biochars were highly aromatized. Various functional groups of the biochar can affect a number of processes during the biochar-complex media (such as soil) interaction. The FTIR spectra corresponding to 3,440 cm-1 could be ascribed to the vibration of O−H stretching. The peaks at 2,920 and 2,850 cm-1 corresponded to symmetric and asymmetric C−H stretching vibrations. The peaks at 1,730 cm-1 are attributed to the stretching vibration of the C=O bond, while the peaks at 1,620 cm-1 can be correlated with C=O and C=C stretching vibrations from ketones and aldehydes groups[31]. Overall, oxygen-containing functional groups were a major part and could play a role in the adsorption process.
X-ray Photoelectron Spectroscopy analysis
Table 3 presents the C1s and O1s bonding state and its relative percentage on the surface of the biochar, while Figure 3 shows the peak deconvolution for the C1s and O1s region scan. Additionally, chemical composition was found and presented in
C1s and O1s bonding state and its relative atomic percentage on biochar surfaces
Binding energy (eV) | Structure | PSD | PG | SS |
284.8 | C−C | 62.4 | 27.2 | 78.6 |
~ 286.0 | C−O | 37.6 | 68.7 | 21.4 |
~ 288.0 | COOH | - | - | - |
289.2 | C−O | - | 4.1 | - |
~ 532.5-533 | C=O | 100 | 100 | 100 |
Table 2. The results were consistent with CHN and EDX analyses. At higher resolution, the XPS spectrum of the C1s peaks shows the main peak at 284.8 eV. This could be attributed to the aromatic carbon (C−C), while the presence of other peaks could be associated with oxygen-containing functional groups, namely carbonyl (C−O at 285.7 eV) and carboxylic and/or ester (O−C=O in at 289.2 eV). For all biochars, O1s region scan was deconvoluted into a single peak with a binding energy of 533.4 eV, which is associated with O=C–O.
Nuclear magnetic resonance analysis
The NMR spectra for the biochar are shown in Figure 4, and the corresponding properties are presented in Table 4. The spectra of all biochars were dominated by the aromatic C resonance centred at ~130 ppm, which is a characteristic of aromatic carbon. The shoulder peak in the 165-185 ppm range may be contributed by carboxylic acid (COOH) and esters (COOR)[32]. The signals at ~55 ppm can be attributed to carbohydrates[33], while the peaks at ~15 and ~35 ppm could be attributed to alkyl. The PSD, PG and SS biochars were predominantly aromatic, with the presence of alkyl C at a small amount. The negative chemical shift (Δδ), which denotes the degree of aromatic condensations of the biochars, is shown in Table 4. Although all biochars were produced at the same temperature, PSD biochar (-9.1) had the highest degree of aromatic condensation, followed by SS and PG. This trend is consistent with the amount of carbon present in the biochar found in the elemental analysis. The PSD biochar has low CP observability [Table 4], and this is evident in the poor spectrum of PSD with an evident poor signal-to-noise ratio [Figure 4].
Figure 4. The NMR spectra of paunch grass, sewage sludge and pine sawdust biochar. NMR: Nuclear magnetic resonance.
NMR observabilities for biochars determined from spin-counting and aromatic condensation (Δδ)
Biochar | C(obs) | δ13C-benzene-CP | Δδ-CP |
(%) | (ppm) | (ppm) | |
PSD | 3.0 | 119.6 | -9.1 |
PG | 18.2 | 125.8 | -2.9 |
SS | 7.8 | 124.9 | -3.8 |
Adsorption isotherms
Adsorption isotherms (linear adsorption model and Freundlich model) for 2,4-D in the different biochar amended soils (Te Kowhai and Matawhero) are shown in Figure 5. The Freundlich isotherm adsorption parameters, Kd and Kdeff were obtained and presented in Table 5. Higher linear determination coefficients
Figure 5. Freundlich isotherm plots for control soil and biochar amended soils. The measured data were represented by symbols, while the model fits were in solid lines.
The isotherm parameters for 2, 4-D on Matawhero and Te Kowhai Soil amended with biochars
Treatment | Kd | R2 | Kf | N | R2 | Kdeff | Log Koc | |
L·kg-1 | mg1-N·LN·kg-1 | L·kg-1 | ||||||
Control | 1.27 | 0.94 | 2.06 | 0.83 | 0.98 | 2.32 | 1.67 | |
Te Kowhai | PSD | 530.35 | 0.82 | 566.11 | 0.83 | 0.95 | 638.23 | 4.11 |
PG | 1.13 | 0.67 | 3.65 | 0.52 | 0.98 | 5.10 | 2.01 | |
SS | 1.40 | 0.85 | 2.75 | 0.74 | 0.98 | 3.28 | 1.82 | |
Control | 0.44 | 0.44 | 1.93 | 0.37 | 0.99 | 2.98 | 2.15 | |
Matawhero | PSD | 314.87 | 0.61 | 360.58 | 0.61 | 0.95 | 474.01 | 4.35 |
PG | 1.59 | 0.98 | 1.70 | 0.94 | 0.98 | 1.77 | 1.93 | |
SS | 1.46 | 0.95 | 2.06 | 1.06 | 0.90 | 2.43 | 2.06 |
Overall, PSD biochar amended soils exhibited higher adsorption of 2,4-D than control and other biochar amended soils. There is minimal effect on the adsorption capacity of the soils amended with SS and PG. The Kd values for PSD amended soils were 530 and 315 L·kg-1, which is significantly higher than the remaining soils, with the second highest of 1.59 L·kg-1 for PG biochar amended Matawhero soils. The adsorption capacity, as represented by Freundlich adsorption coefficient (KF), followed an order: PSD > PG > SS for the Te Kowhai soils and PSD > SS> PG for the Matawhero soils. For Te Kowhai soils, the addition of biochar decreased the linearity based on N values that range from 0.52 to 0.83. In contrast, its addition to Matawhero soil increased its linearity with N values ranging from 0.37 to 1.06 [Table 5]. The Kdeff values for 2,4-D at the lowest equilibrium concentration (Cw = 0.5 mg·L-1) ranged from 2.32 to 638 L·kg-1 in biochar amended soils. PSD-amended soils showed 20-30 times greater adsorption potential than the control soil.
Comparative data showing the various adsorption parameters such as Kd, Kf, and dominant adsorption mechanisms for removal of 2,4-D are shown in Table 6 under carbon-based and soil-based adsorbents. The Kd and Kf values for the PSD amended with soil were higher than most of the recent literature-reported data. The high Kd value in our case for PSD biochar amended soil could be attributed to its extremely high SSA of 795 m2·g-1 and aromatic carbon structure. The high log Koc values of soil with PSD also further demonstrate the ability of PSD biochar to immobilize the 2,4-D herbicide due to the strong affinity of 2,4-D to PSD biochar.
Comparative adsorption parameters for the carbon-based and soil sorbents from the recent literature
Author | Adsorbent | Max Capacity | Kd | Kf | Mechanism |
Zhu et al., 2018[35] | Amino activated corn stalk Biochar | 22.84 mg·g-1 | - | 0.919 | π-π interaction, chemisorption and hydrogen bonding |
Essandoh et al., 2017[36] | Switchgrass biochar | 134 mg·g-1 | - | 2.675 mg·g-1 | van der Waals, dipole-dipole, and π-π interactions and hydrogen bonding |
Doczekalska et al., 2018[37] | Flax, hemp shives and miscanthus based activates carbon | 2.682 m.mol·g-1 | - | 2.41 | Dipole-dipole, H-bonding, covalent bonding |
Binh & Nguyen, 2020[38] | Corn cob biochar | 37.40 mg·g-1 | - | 13.8 (mg·g-1) (mg·L-1)-n | Pore-filling, electrostatic, H- bonding, hydrophobic bonding, and π-π EDA interactions |
Li et al., 2018[31] | Nitric Acid Modified Activated Carbon Fiber | 555 mg·g-1 | - | 235.017 (mg·g-1) (mg·L-1)-n | Surface/Physical adsorption |
Alikhani et al., 2021[8] | TiO2/BiOBr/Bi2S3 nanostructure stabilized on the activated carbon | 87.61 mg·g-1 | - | 23.3 L/g | - |
Salomón et al., 2021[39] | Activated carbon derived from Queen palm fruit | 367 mg·g-1 | - | Hydrogen bond, π-π, or electrostatic interactions | |
Soil systems | |||||
Meftaul et al., 2020[34] | Loam, sandy loam, silt loam, sand, loamy sand, and clay- Australian soils | 92 mg·kg-1 | 4.68 L·Kg-1 | Surface sorption and partition | |
Fernandez et al., 2021[40] | Soil and wheat stubble mixture | 155.7 mg·kg-1 | - | 0.64 (mg·kg-1)(L·mg-1) | Electrostatic interactions and non-polar interactions |
Cotillas et al., 2018[41] | Clay soil washing and adsorption onto activated carbon | 216 mg·g-1 | - | 9.01 | - |
Buerge et al., 2020[42] | Agriculture/railway track | - | Kf-0.83 mL·g-1 | Sorption to organic matter | |
Ololade et al., 2015[43] | soil without soil organic matter | 333 mg·g-1 | Oxic-1.40 Anoxic- 1L·kg-1 | 1.148 | Electrostatic interaction |
Ozbay et al., 2018[44] | Calcareous soil | - | 0.66 L·kg-1 | 2.37 | Soil organic matter |
Mon et al., 2009[45] | Volcanic ash soil | - | 41.1 L·kg-1 | 52.5 | Soil organic matter |
Werner et al., 2013[46] | Below mentioned soils/sorbents | 1.44 L·kg-1 | |||
This study | PSD amended soils | 224.12 mg·kg-1 | 530.3 L·kg-1 | 566 (mg/Kg)/(mg·L)n | Hydrogen bond, π-π EDA, and pore intrusion |
Plausible adsorption mechanisms
Adsorption of 2, 4-D onto soil
Between the two soil controls, the adsorption of 2,4-D was higher onto Te Kowhai soil (Kf = 2.06) than Matawhero soils (Kf = 1.93). The Te Kowhai and Matwhero soils possessed organic carbon content of 5% and 2%, respectively, and have been earlier attributed to the high adsorption of 2,4-D onto soils[43]. Additionally, electrostatic attraction interaction of positive Fe and Al ions present in the soil with the 2,4-D anions can likely enhance the adsorption of 2,4-D (Ololade et al., 2015)[43]. The high clay percentage in the Te Kowhai soils could also be an explanation for the higher adsorption than the Matawhero soils. Overall, surface hydroxyls derived from metal hydroxides and metal-SOM complexes and clay mineralogy were postulated to be the plausible adsorption mechanisms[47].
Adsorption of 2,4-D onto biochar amended soils
The plausible adsorption mechanism of 2,4-D onto biochar amended soils is represented in Figure 6, and it can be broadly explained by three different mechanisms: (i) pore intrusion/filling; (ii) hydrogen bonding; and (iii) π-π EDA interactions. The size of 2,4 D molecular structure is around 1.54 nm × 0.56 nm × 0.22 nm, and SSA for the biochars used as summarized in Table 2 reveals some interesting observations. By comparing the adsorption of 2,4-D onto different adsorbents, it can be surmised that SSA is directly related to the removal of 2,4-D. For instance, PSD has the highest SSA of 795 m2·g-1 and the highest Kf values of 530 and 315 for the two soils. However, the remaining biochar’s SSA values were found to be in the range of approximately 2.0-8.0 m2·g-1 and showed a similar level of adsorption with Kf values of 1.7-3.65. In addition, the biochar particles’ pore size was in the range of micro- and meso-size [Figure 1], which is greater than the nano size of the 2,4-D, implying the possibility of pore intrusion mechanisms.
Figure 6. Plausible adsorption mechanisms between PSD biochar and 2,4-D: (A) hydrogen bonding; (B) π- π EDA interactions. PSD: pine sawdust.
Hydrogen bonding as the adsorption mechanism can be explained in two ways: (i) interaction of H+ ions in the 2,4-D (alcoholic group) with oxygen ions from the functional groups such as hydroxyl, phenolic, present on the biochar; (ii) oxygen ions from the ketonic group or hydroxyl group present in the biochar to the hydrogen ion from the 2,4-D. Based on the FTIR and NMR analysis, the biochar has functional groups: OH− stretching, C=O stretching, COOR, and carboxylic (-COOH). The presence of hydrogen and oxygen ions in the functional groups and 2-4, D can interact to form potential hydrogen bonds and result in the adsorption of 2,4-D onto biochar. An example of such possible hydrogen bonding is schematized in Figure 6A, where the hydroxylic group is present in the biochar, forming potential hydrogen bonding with 2,4-D.
The third possible adsorption mechanism presented in Figure 6B could be associated with the π electrons in the aromatic rings of 2,4-D and biochar[38]. The biochar is highly aromatic (confirmed by NMR and elemental analysis) and contains functional groups such as −OH, C=C, C-H and C=O. The O−H group acts as an electron donor and creates a π- in the cyclic ring of biochar. Then, the halogen (Cl) group in the 2,4-D can withdraw electrons, thus forming π+ density in the nucleus of 2,4-D[38]. This resulting interaction could potentially enable 2,4-D adsorption onto biochar. On comparing different biochars, given that the PSD has a high carbon percentage (90.9%) and H/C = 0.01, it is conceivable that it has a high degree of aromaticity and indeed demonstrated a higher adsorption behaviour than the other biochar due to potential dominating effect of π-π EDA mechanisms.
ENVIRONMENTAL SIGNIFICANCE
We investigated the utilization of waste-derived biochars (PSD, PG and SS) as an effective adsorbent for a widely used herbicide, 2,4-D, also termed as an emerging contaminant in soil systems. The findings from the study demonstrated that waste-derived biochars have the potential not only to retain and/or reduce the mobility of the compound that can potentially contaminate the surface water and groundwater, but could further decrease the ecological and human health risks. Due to the use of waste as feedstocks to produce biochar, there is no commercial value and competition with other potential uses of these feedstocks, thus demonstrating the potential of minimization or conversion of waste to adsorbent or as soil enhancer that can be beneficial for agricultural sectors. Therefore, the two main environmental significance of this study can be: (i) the retention of herbicide to reduce its mobility and contamination of aquatic environment; and (ii) waste minimization or management of such organic waste stream.
CONCLUSIONS
The following conclusions are drawn from the study.
Amending soil with biochar may increase adsorption capacity for 2,4-D; however, it is dependent on the characteristics of the biochar. The adsorption affinity for biochar amended soils followed an order: PSD > > PG > SS.
PSD biochar ammended soil resulted in increased 2,4-D uptake, suggesting that the mobility potential for the herbicide was decreased. Thus, this could potentially lead to reduction of toxicity and less availability to soil organisms such as microbes, plants and invertebrates. However, caution is required as high adsorption could also lead non-availability of nutrients for the plants.
PSD biochar exhibited a very high adsorption affinity for 2,4 D compared to other biochars due to its significantly higher degree of aromaticity and SSA.
The adsorption affinity of 2,4-D for biochar was not affected by surface functional groups.
Based on the findings, biochars produced from biomass of lignocellulosic origin could potentially be an excellent adsorbent to remediate soils contaminated with similar organic compounds.
DECLARATIONS
Authors’ contributionsReview, editing, revision of the manuscript: Reguyal F
Review, editing, plotting, revision of the manuscript: Praneeth S
Conceptualisation, writing original draft, data analysis, modelling, revision, correspondence: Sarmah AK
Availability of data and materialsData and materials used in this manuscript are confidential.
Financial support and sponsorshipNone.
Conflicts of interestAll authors declared that there are no conflicts of interest.
Ethical approval and consent to participateNot applicable.
Consent for publicationNot applicable.
Copyright© The Author(s) 2023.
Supplementary MaterialsREFERENCES
1. Trivedi NS, Kharkar RA, Mandavgane SA. Utilization of cotton plant ash and char for removal of 2, 4-dichlorophenoxyacetic acid. Resour-Effic Technol 2016;2:S39-46.
2. Li L, Zou D, Xiao Z, et al. Biochar as a sorbent for emerging contaminants enables improvements in waste management and sustainable resource use. J Clean Prod 2019;210:1324-42.
3. Baloochi SJ, Solaimany Nazar AR, Farhadian M. 2,4-Dichlorophenoxyacetic acid herbicide photocatalytic degradation by zero-valent iron/titanium dioxide based on activated carbon. Environ Nanotechnol Monit Manage 2018;10:212-22.
4. Bahrami M, Amiri MJ, Beigzadeh B. Adsorption of 2,4-dichlorophenoxyacetic acid using rice husk biochar, granular activated carbon, and multi-walled carbon nanotubes in a fixed bed column system. Water Sci Technol 2018;78:1812-21.
5. Coelho ERC, Brito GM, Frasson Loureiro L, Schettino MA Jr, Freitas JCC. 2,4-dichlorophenoxyacetic acid (2,4-D) micropollutant herbicide removing from water using granular and powdered activated carbons: a comparison applied for water treatment and health safety. J Environ Sci Health B 2020;55:361-75.
6. Chen M, Zheng J, Dai R, Wu Z, Wang Z. Preferential removal of 2,4-dichlorophenoxyacetic acid from contaminated waters using an electrocatalytic ceramic membrane filtration system: mechanisms and implications. Chem Eng J 2020;387:124132.
7. Xu X, Cai J, Zhou M, Du X, Zhang Y. Photoelectrochemical degradation of 2,4-dichlorophenoxyacetic acid using electrochemically self-doped Blue TiO2 nanotube arrays with formic acid as electrolyte. J Hazard Mater 2020;382:121096.
8. Alikhani N, Farhadian M, Goshadrou A, Tangestaninejad S, Eskandari P. Photocatalytic degradation and adsorption of herbicide 2,4-dichlorophenoxyacetic acid from aqueous solution using TiO2/BiOBr/Bi2S3 nanostructure stabilized on the activated carbon under visible light. Environ Nanotechnol Monit Manage 2021;15:100415.
9. Lewis KA, Green A, Tzilivakis J, Warner D. The Pesticide Properties DataBase (PPDB) developed by the Agriculture & Environment Research Unit (AERU), University of Hertfordshire, 2006-2015. Available from: https://www.researchgate.net/publication/302465822_The_Pesticide_Properties_DataBase_PPDB_developed_by_the_Agriculture_Environment_Research_Unit_AERU [Last accessed on 17 Aug 2023].
10. Wu H, Gong L, Zhang X, He F, Li Z. Bifunctional porous polyethyleneimine-grafted lignin microspheres for efficient adsorption of 2,4-dichlorophenoxyacetic acid over a wide pH range and controlled release. Chem Eng J 2021;411:128539.
11. US EPA. 2012 Edition of the drinking water standards and health advisories (EPA 822-S-12-001); 2012. p 1-12. Available from: https://nepis.epa.gov/Exe/ZyNET.exe/P100N01H.txt?ZyActionD=ZyDocument&Client=EPA&Index=2011%20Thru%202015&Docs=&Query=&Time=&EndTime=&SearchMethod=1&TocRestrict=n&Toc=&TocEntry=&QField=&QFieldYear=&QFieldMonth=&QFieldDay=&UseQField=&IntQFieldOp=0&ExtQFieldOp=0&XmlQuery=&File=D%3A%5CZYFILES%5CINDEX%20DATA%5C11THRU15%5CTXT%5C00000016%5CP100N01H.txt&User=ANONYMOUS&Password=anonymous&SortMethod=h%7C-&MaximumDocuments=1&FuzzyDegree=0&ImageQuality=r75g8/r75g8/x150y150g16/i425&Display=hpfr&DefSeekPage=x&SearchBack=ZyActionL&Back=ZyActionS&BackDesc=Results%20page&MaximumPages=1&ZyEntry=1. [Last accessed on 15 Aug 2023].
12. Sarmah AK, Müller K, Ahmad R. Fate and behaviour of pesticides in the agroecosystem - a review with a New Zealand perspective. Aust J Soil Res 2004;42:125-54.
13. Kanissery R, Gairhe B, Mcavoy C, Sims G. Herbicide bioavailability determinant processes in the soil. J Bioremediat Biodegrad 2019;10:458.
14. Sarmah AK, Srinivasan P, Smernik RJ, et al. Retention capacity of biochar-amended New Zealand dairy farm soil for an estrogenic steroid hormone and its primary metabolite. Aust J Soil Res 2010;48:648-58.
15. Luo Z, Yao B, Yang X, et al. Novel insights into the adsorption of organic contaminants by biochar: A review. Chemosphere 2022;287:132113.
16. Qiu B, Tao X, Wang H, Li W, Ding X, Chu H. Biochar as a low-cost adsorbent for aqueous heavy metal removal: a review. J Anal Appl Pyrol 2021;155:105081.
17. Zhou Y, Qin S, Verma S, et al. Production and beneficial impact of biochar for environmental application: a comprehensive review. Bioresour Technol 2021;337:125451.
18. Sun K, Keiluweit M, Kleber M, Pan Z, Xing B. Sorption of fluorinated herbicides to plant biomass-derived biochars as a function of molecular structure. Bioresour Technol 2011;102:9897-903.
19. Srinivasan P, Sarmah AK, Smernik R, Das O, Farid M, Gao W. A feasibility study of agricultural and sewage biomass as biochar, bioenergy and biocomposite feedstock: production, characterization and potential applications. Sci Total Environ 2015;512-3:495-505.
20. An X, Wu Z, Shi W, et al. Biochar for simultaneously enhancing the slow-release performance of fertilizers and minimizing the pollution of pesticides. J Hazard Mater 2021;407:124865.
21. Suo F, You X, Ma Y, Li Y. Rapid removal of triazine pesticides by P doped biochar and the adsorption mechanism. Chemosphere 2019;235:918-25.
22. Kearns JP, Shimabuku KK, Knappe DR, Summers RS. High temperature co-pyrolysis thermal air activation enhances biochar adsorption of herbicides from surface water. Environ Eng Sci 2019;36:710-23.
23. Khorram MS, Sarmah AK, Yu Y. The Effects of biochar properties on fomesafen adsorption-desorption capacity of biochar-amended soil. Water Air Soil Pollut 2018;229:60.
24. Kookana RS, Sarmah AK, Van Zwieten L, Krull E, Singh B. Biochar application to soil: agronomic and environmental benefits and unintended consequences. Adv Agron 2011;112:103-43.
25. Jin J, Kang M, Sun K, Pan Z, Wu F, Xing B. Properties of biochar-amended soils and their sorption of imidacloprid, isoproturon, and atrazine. Sci Total Environ 2016;550:504-13.
26. Chen B, Yuan M. Enhanced sorption of polycyclic aromatic hydrocarbons by soil amended with biochar. J Soils Sediments 2011;11:62-71.
27. Kırbıyık Ç, Pütün AE, Pütün E. Equilibrium, kinetic, and thermodynamic studies of the adsorption of Fe(III) metal ions and 2,4-dichlorophenoxyacetic acid onto biomass-based activated carbon by ZnCl 2 activation. Surf Interfaces 2017;8:182-92.
28. Kazak O, Eker YR, Akin I, Bingol H, Tor A. Green preparation of a novel red mud@carbon composite and its application for adsorption of 2,4-dichlorophenoxyacetic acid from aqueous solution. Environ Sci Pollut Res 2017;24:23057-68.
29. Xing B, Pignatello J. Sorption | Organic Chemicals. In:Encyclopedia of Soils in the Environment. Elsevier; 2005. p. 537-48.
30. Environmental Science. Standardized product definition and product testing guidelines for biochar that is used in soil. 2015.Available from: https://www.semanticscholar.org/paper/Standardized-Product-Definition-and-Product-Testing-Ibi/d7f179afe9080d86b27be014109d4ebbd4b46a1b. [Last accessed on 15 Aug 2023].
31. Li Q, Sun J, Ren T, et al. Adsorption mechanism of 2,4-dichlorophenoxyacetic acid onto nitric-acid-modified activated carbon fiber. Environ Technol 2018;39:895-906.
32. Haeldermans T, Claesen J, Maggen J, et al. Microwave assisted and conventional pyrolysis of MDF-characterization of the produced biochars. J Anal Appl Pyrol 2019;138:218-30.
33. Mcbeath AV, Smernik RJ, Krull ES, Lehmann J. The influence of feedstock and production temperature on biochar carbon chemistry: a solid-state 13C NMR study. Biomass Bioenerg 2014;60:121-9.
34. Meftaul IM, Venkateswarlu K, Dharmarajan R, Annamalai P, Megharaj M. Movement and fate of 2,4-D in urban soils: a potential environmental health concern. ACS Omega 2020;5:13287-95.
35. Zhu L, Zhao N, Tong L, Lv Y, Li G. Characterization and evaluation of surface modified materials based on porous biochar and its adsorption properties for 2,4-dichlorophenoxyacetic acid. Chemosphere 2018;210:734-44.
36. Essandoh M, Wolgemuth D, Pittman CU Jr, Mohan D, Mlsna T. Phenoxy herbicide removal from aqueous solutions using fast pyrolysis switchgrass biochar. Chemosphere 2017;174:49-57.
37. Doczekalska B, Kuśmierek K, Świątkowski A, Bartkowiak M. Adsorption of 2,4-dichlorophenoxyacetic acid and 4-chloro-2-metylphenoxyacetic acid onto activated carbons derived from various lignocellulosic materials. J Environ Sci Health B 2018;53:290-7.
38. Binh QA, Nguyen HH. Investigation the isotherm and kinetics of adsorption mechanism of herbicide 2,4-dichlorophenoxyacetic acid (2,4-D) on corn cob biochar. Bioresour Technol Rep 2020;11:100520.
39. Salomón YLDO, Georgin J, Franco DS, et al. High-performance removal of 2,4-dichlorophenoxyacetic acid herbicide in water using activated carbon derived from Queen palm fruit endocarp (Syagrus romanzoffiana). J Environ Chem Eng 2021;9:104911.
40. Fernandez ME, Morel MDR, Clebot AC, Zalazar CS, Ballari MDLM. Effectiveness of a simple biomixture for the adsorption and elimination of 2,4-dichlorophenoxyacetic acid (2,4-D) herbicide and its metabolite, 2,4-dichlorophenol (2,4-DCP), for a biobed system. J Environ Chem Eng 2022;10:106877.
41. Cotillas S, Sáez C, Cañizares P, Cretescu I, Rodrigo MA. Removal of 2,4-D herbicide in soils using a combined process based on washing and adsorption electrochemically assisted. Sep Purif Technol 2018;194:19-25.
42. Buerge IJ, Pavlova P, Hanke I, Bächli A, Poiger T. Degradation and sorption of the herbicides 2,4-D and quizalofop-P-ethyl and their metabolites in soils from railway tracks. Environ Sci Eur 2020;32:150.
43. Ololade IA, Alomaja F, Oladoja NA, Ololade OO, Oloye FF. Kinetics and isotherm analysis of 2,4-dichlorophenoxyl acetic acid adsorption onto soil components under oxic and anoxic conditions. J Environ Sci Health B 2015;50:492-503.
44. Ozbay B, Akyol NH, Akyol G, Ozbay I. Sorption and desorption behaviours of 2,4-D and glyphosate in calcareous soil from Antalya, Turkey. Water Environ J 2018;32:141-8.
45. Mon EE, Hirata T, Kawamoto K, Hiradate S, Komatsu T, Moldrup P. Adsorption of 2,4-dichlorophenoxyacetic acid onto volcanic ash soils: effects of pH and soil organic matter. Environment Asia 2009;1:1-9. Available from: http://www.tshe.org/ea/pdf/EA2(1)_01.pdf. [Last accessed on 17 Aug 2023]
46. Werner D, Garratt JA, Pigott G. Sorption of 2,4-D and other phenoxy herbicides to soil, organic matter, and minerals. J Soils Sediments 2013;13:129-39.
Cite This Article
Export citation file: BibTeX | RIS
OAE Style
Reguyal F, Praneeth S, Sarmah AK. Modelling and spectroscopic investigation of 2,4-D adsorption in soil amended with pine sawdust, paunch grass and sewage sludge biochars. Water Emerg Contam Nanoplastics 2023;2:16. http://dx.doi.org/10.20517/wecn.2023.35
AMA Style
Reguyal F, Praneeth S, Sarmah AK. Modelling and spectroscopic investigation of 2,4-D adsorption in soil amended with pine sawdust, paunch grass and sewage sludge biochars. Water Emerging Contaminants & Nanoplastics. 2023; 2(3): 16. http://dx.doi.org/10.20517/wecn.2023.35
Chicago/Turabian Style
Reguyal, Febelyn, Sai Praneeth, Ajit K. Sarmah. 2023. "Modelling and spectroscopic investigation of 2,4-D adsorption in soil amended with pine sawdust, paunch grass and sewage sludge biochars" Water Emerging Contaminants & Nanoplastics. 2, no.3: 16. http://dx.doi.org/10.20517/wecn.2023.35
ACS Style
Reguyal, F.; Praneeth S.; Sarmah AK. Modelling and spectroscopic investigation of 2,4-D adsorption in soil amended with pine sawdust, paunch grass and sewage sludge biochars. Water. Emerg. Contam. Nanoplastics. 2023, 2, 16. http://dx.doi.org/10.20517/wecn.2023.35
About This Article
Special Issue
Copyright
Data & Comments
Data
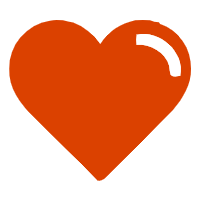

Comments
Comments must be written in English. Spam, offensive content, impersonation, and private information will not be permitted. If any comment is reported and identified as inappropriate content by OAE staff, the comment will be removed without notice. If you have any queries or need any help, please contact us at support@oaepublish.com.