Linking environmental exposure and effects of pharmaceuticals on aquatic biota: state of knowledge in Latin America
Abstract
The presence of pharmaceutically active compounds (PhACs) in the environment has been confirmed in different parts of the world, but mainly in North America and Europe. In Latin America, progress on this issue has not been as advanced, so we decided to compile the available information. The aim was to relate the environmental exposure of PhACs detected in fresh surface waters of Latin America, their bioaccumulation and lethal or sublethal effects. Most of the reported concentrations are in the same order of magnitude (113 PhACs in fresh surface water: 0.04 ng/L-227,000 ng/L, 57 PhACs in aquatic biota: 0.02-652 ng/g d.w.) as those previously reported worldwide. The groups with the highest concentrations were analgesics/anti-inflammatories in water and antibiotics in biofilm. For effects, we found 67 no observed effect concentration (NOEC) values for 37 PhACs, 36 lowest observed effect concentration (LOEC) values for 26 PhACs and 45 lethal concentration (LC50) values for 31 PhACs. The only compound whose concentration in water exceeded the lowest LC50 value was acetaminophen (32,000 ng/L), which is an alarm signal for the need for further monitoring of this PhAC. In addition, acetaminophen, caffeine, carbamazepine, propranolol, 17α-ethinylestradiol and 17β-estradiol were the only PhACs with NOEC and LOEC values below the concentration in water. At the regional level, the information available in Latin America is scarce and becomes even scarcer when bioaccumulation in aquatic biota is considered. At the global level, little ecotoxicological information is available. This review identifies knowledge gaps and serves as a guide and reference for further decision-making.
Keywords
INTRODUCTION
Today’s world is characterized by a growing and accelerated development, accompanied by a great technological capacity and the accumulation of wealth, which can lead to the irretrievable destruction of natural resources[1]. In this context, environment and development come into conflict, leading to one of the fundamental problems of humanity: trying to satisfy basic needs without simultaneously destroying the environment from which resources and services are extracted. As stated in the Brundtland Report[2], environment and development are not separate challenges but interrelated. Thus, development cannot be sustained if the environmental resource base deteriorates, and at the same time, the environment cannot be protected if growth does not take into account the consequences of environmental problems. Current environmental problems due to anthropogenic activities include habitat loss and pollution from emerging contaminants[1].
The definition of emerging contaminants has changed over time as new compounds have been added to this category[3,4]. The new approach focuses on contaminants that have been present in the environment for some time, but for which concerns have only recently arisen or for which new facts or information have provided a new perspective on concerns about these known pollutants. Consequently, a clear definition of emerging contaminants should consider them as naturally occurring, manufactured, or crafted chemicals or materials that are now discovered or suspected to be present in various environmental compartments and whose toxicity or persistence is likely to significantly alter the metabolism of any organism[4]. We have adopted the latter definition here and will, therefore, henceforth use the term “contaminants of emerging concern” (CECs). Among the various CECs, the available literature lists the following under this category: flame retardants, polar pesticides, perfluorinated compounds, cyanotoxins, pharmaceuticals, personal care products, illicit drugs, stimulants/lifestyle compounds and nanoparticles[4,5]. Another important issue regarding potential CECs is that the lack of relevant environmental fate, ecotoxicological or toxicological data prevents an adequate assessment of the associated risks[4]. The challenge is to be proactive rather than reactive in gaining knowledge about potential CECs.
The presence of pharmaceuticals has been confirmed in surface water, raw and treated wastewater and groundwater in different parts of the world, especially in North America and Western Europe[6-10]. Their bioaccumulation in aquatic biota has also been studied, but the number of reports is fewer and more recent worldwide[11,12]. Although both the beneficial and adverse effects of their direct administration are known, their side effects on humans due to indirect uptake through pharmaceutically contaminated water are unknown. However, it may be possible to determine whether a small amount of pharmaceuticals in the environment poses a threat to human and environmental health by analyzing the vast amount of data from mammalian and clinical studies, and applying advanced methods to predict long-term and low-level effects[13].
Pharmaceuticals are designed to target specific metabolic and molecular pathways in humans and nonhuman animals. Therefore, they may affect similar metabolic pathways in animals with comparable target organs, tissues, cells, or biomolecules. Because many pharmaceuticals have an unclear specific mode of action, it is challenging to design and conduct toxicity experiments that target specific drug targets in nonhuman animals. This approach can help mitigate the potential ecological effects of pharmaceuticals on aquatic biota and other organisms in the environment[14]. The effects of pharmaceuticals on aquatic biota can vary depending on the active ingredient, level of exposure, interactions between contaminants or environmental matrices and species studied. Some pharmaceuticals have been shown to disrupt the endocrine system of fish causing changes in reproduction, feeding behavior and predator avoidance[15]. Others have been shown to cause development and growth inhibition, abnormalities and mortality in different aquatic organisms[14,16,17]. Overall, the effects of pharmaceuticals on aquatic biota highlight the importance of proper disposal and management of these compounds. It is important to consider the potential ecological impacts of pharmaceuticals when designing wastewater treatment systems and developing new drugs. Ecotoxicological data can assist in decision-making and ensure the safe and sustainable use of pharmaceuticals in the environment. Although there are still contradictory results, most assessments are conducted under controlled laboratory conditions[6,11,14]. Thus, there is a need to evaluate the effects of environmental exposure and the possible synergistic effects of the different pharmaceuticals.
Considering that progress on this issue is still in its infancy in Latin America and that knowledge is not well-organized, we decided to make an overview of the existing information and organized it to be able to envision future studies that might be needed.
The general aim of this review was to summarize the existing information on human pharmaceutical contamination in Latin America and the Caribbean (hereafter, we refer to the entire region as “Latin America”) in order to identify gaps in this region, which is often underrepresented in environmental studies. The specific aim was to relate environmental exposure in fresh surface water from Latin America, bioaccumulation in native aquatic biota and lethal and sublethal effects. This review will provide information on the current state of knowledge of pharmaceutical contamination in Latin America and serve as a guide and reference for subsequent decision-making.
HUMAN PHARMACEUTICALLY ACTIVE COMPOUNDS: ENVIRONMENTAL EXPOSURE AND EFFECTS
Among CECs, pharmaceuticals and active ingredients in personal care products represent a large class of chemicals that are used in large quantities worldwide and have received minor attention. The increasing introduction of new products into the market is leading to an exponential increase in the already large number of classes of chemicals, each of which has distinct and poorly understood biochemical modes of action in mammals, and/or has not even been studied in most organisms. Most of these products are continuously disposed of through domestic and/or industrial wastewater systems, runoff or directly discharged into the environment[6,16]. In this review, we focused mainly on human pharmaceutically active compounds (PhACs), which are chemical substances used to diagnose, treat, or prevent diseases, but we have also included caffeine because it is used in combination with other compounds (e.g., stimulants, analgesics, diuretics, weight control products)[18]; cocaine and its metabolite (benzoylecgonine) as they are good indicators of urbanization and deficiency of sewage treatment systems; and natural estrogens that have been listed as important endocrine disruptors for aquatic biota[19]. In order to simplify the terminology, the term PhACs will refer throughout this work to the compounds detailed above. They are all a broad group of organic compounds used in large amounts and variations that, after administration, are absorbed and metabolized by the organism and eventually excreted in the feces and urine, either as unchanged compounds or as metabolites. These excretions, if present, become part of the wastewater system and enter surface waters either untreated or with conventional treatment methods that do not effectively eliminate them[20]. Although they generally have a high rate of degradation and their concentrations in the environment are relatively low (μg/L or ng/L) compared to other pollutants, their continuous input into the environment via wastewater has led to their classification as pseudo-persistent compounds[13]. However, given that within the term PhACs, there are a large number of compounds with different physicochemical characteristics, we consider that it would be more appropriate to refer to them as “high input rate contaminants”, regardless of their half-life and degradation capacity. In any case, there is a clear need to develop alternative and sustainable technologies to eliminate or mitigate the potential adverse effects of these compounds on the environment. In recent years, some studies have shown that additional processes such as adsorption by powdered activated carbon or even other technologies such as biodiscs and artificial wetlands could be promising tools to improve the removal of these contaminants from conventional wastewater treatment plants[21-24].
The life cycle of high input rate contaminants extends from production (preclinical and clinical research, monitoring and commercialization) to distribution, consumption and waste disposal, all of which are potential pathways into the environment. Management and use practices in different regions of the world may also vary, so that an important exposure pathway in one geographic area may be a less important pathway in another[6]. In addition, most pharmaceuticals were developed to relieve symptoms rather than to cure disease, so many are taken for long periods of time. They are currently of great importance to the main agencies concerned with the protection of public health and the environment, such as the World Health Organization (WHO), the United States Environmental Protection Agency (US EPA) or the European Commission[5]. As they are so diverse, Boxall et al.[6] pointed out the need to prioritize the compounds that are likely to pose the greatest risk because it is impossible to experimentally evaluate the hazards and risks of all available ones. The next sections address human PhACs that enter fresh surface waters and bioaccumulate in aquatic biota from Latin America, and the final section links environmental exposure to biological effects.
Data search criteria: pharmaceuticals reported in fresh surface water and aquatic biota
The search for reported PhACs concentrations in fresh surface water and freshwater biota from Latin America was conducted in the Scopus® and Scielo® databases between November and December 2022. These databases collect peer-reviewed articles from different scientific disciplines. All articles reporting PhACs concentrations in fresh surface waters and aquatic biota were considered, having discarded the following: 1: articles in which sampling sites could not be determined directly (using coordinates) or indirectly (estimated from maps); 2: articles in which PhACs were detected but not quantified in water or aquatic biota; 3: articles that reported PhACs concentrations directly measured in influents and effluents from wastewater treatment plants; and 4: articles that reported bioaccumulation of PhACs under laboratory conditions (we only considered bioaccumulation in field-collected aquatic biota naturally exposed to these contaminants). The search strategy consisted of using keywords in English, Portuguese, and Spanish
Once the search was completed, each article was read in detail and the data of interest (e.g., coordinates, concentration, unit of measure, tissue) were extracted. At the end of this phase, the references of each article were checked to detect studies that had not been included in the previous search. Then, the above criteria were applied to these newfound papers. Every effort was made to ensure that the search was as inclusive as possible. Also, unpublished information from the research group was included [Supplementary Table 2]. The cut-off date for this review was 31st December 2022. Statistical analyses and figure design were performed in the R 4.2.1 environment[25] using the tidyverse[26], lattice[27] and ggmap[28] packages.
Human PhACs in fresh surface water
Based on the research conducted [n = 44 articles; Supplementary Table 2], most of the reported concentrations (= 99%) were in the same order of magnitude as those previously reported for fresh surface waters worldwide (< 40,000 ng/L)[10,14]. Specifically, 113 PhACs belonging to 26 different therapeutic groups have been reported in fresh surface water from Latin America. Among these therapeutic groups, 21 PhACs belong to psychiatric drugs, 17 to analgesics/anti-inflammatories and 16 to antibiotics [Table 1, Supplementary Table 2]. The group with the highest observed concentrations (0.1-227,000 ng/L) were analgesics/anti-inflammatories [Figures 1 and 2], as previously reported in North America, Europe, South Africa, and Australia[9,14,29]. This therapeutic group includes non-steroidal compounds (e.g., acetylsalicylic acid, acetaminophen, ibuprofen), which are mostly used as painkillers and sold in large quantities, usually without prescription. Specifically in Latin America, the highest concentrations of analgesics/anti-inflammatories belong to acetaminophen and have been reported in Brazil (30,421 ng/L)[30] and Bolivia (227,000 ng/L)[10]. However, this last report from Bolivia appears to be an outlier, as it is three orders of magnitude higher than the maximum concentration reported for North America (291 ng/L) and Europe (394 ng/L)[10], and it is the only value of this magnitude in Latin America [Supplementary Table 2]. Although the octanol-water partition coefficient of acetaminophen indicates low lipophilicity (log Kow = 0.9), as with most analgesics, its ionizability depends on the pH of the environment, which can greatly alter this value[11]. According to the authors' descriptions, the site in Bolivia (Seke River) is suspected of draining septic tanks and the site in Brazil (Água Quente stream) is the main receptor of untreated wastewater. In addition, the authors who reported the high concentration in Brazil linked it to nonprescription sales and widespread consumption of analgesics. However, the problem of nonprescription sales is not unique to this country but is widespread throughout Latin America. Thus, the characteristics of each location could be the reasons for the higher concentrations. In this sense, comparisons between measurements performed in different countries should be made with caution to avoid false conclusions. In other words: in this review, we could compare each concentration with poverty level and access to health care, age structure, degree of urbanization or even per capita income. However, the measurements were made using different analytical methods, in different environments (rivers, streams, ponds) and at different times of the year. Therefore, this review aims to provide an overview of PhACs contamination of fresh surface water from Latin America through general comparisons at the regional level and by weighing the knowledge gaps.
Figure 1. Maximum concentrations of human pharmaceutically active compounds reported in fresh surface water from Latin America.
Figure 2. Total concentrations of human pharmaceutically active compounds, classified by therapeutic group, reported in fresh surface waters from different Latin American countries.
Summary of the number of pharmaceutically active compounds, classified by therapeutic group, that has been reported (√) in water or aquatic biota or has been tested in a laboratory* to evaluate its lethal or sublethal effects on aquatic organisms (algae, crustaceans, amphibians, and fish)
Therapeutic group | Compound | Water | Biota | Effects |
Analgesics/anti-inflammatories | Acetaminophen | √ | √ | √ |
Codeine | √ | √ | ||
Diclofenac | √ | √ | ||
Hydrocodone | √ | |||
Ibuprofen | √ | √ | √ | |
Indomethacin | √ | √ | ||
Ketoprofen | √ | √ | ||
Lidocaine | √ | |||
Meloxicam | √ | |||
Naproxen | √ | √ | ||
Nimesulide | √ | √ | ||
Orphenadrine | √ | |||
Oxycodone | √ | √ | ||
Phenazone | √ | √ | ||
Piroxicam | √ | √ | ||
Propyphenazone | √ | √ | ||
Salicylic acid | √ | √ | ||
Tramadol | √ | |||
17 | 8 | 8 | ||
Androgens | 5α-dihydrotestosterone | √ | √ | |
Testosterone | √ | √ | ||
2 | 0 | 2 | ||
Antibiotics | Azithromycin | √ | √ | |
Cephalexin | √ | |||
Cinoxacin | √ | |||
Ciprofloxacin | √ | √ | √ | |
Clarithromycin | √ | √ | √ | |
Clindamycin | √ | |||
Erythromycin | √ | √ | √ | |
Lincomycin | √ | √ | ||
Metronidazole | √ | √ | ||
N-Acetyl-Sulfamethoxazole | √ | |||
Norfloxacin | √ | √ | √ | |
Ofloxacin | √ | √ | √ | |
Oxacillin | √ | |||
Penicillin G | √ | |||
Penicillin V | √ | |||
Sulfamethoxazole | √ | √ | √ | |
Sulfathiazole | √ | √ | √ | |
Trimethoprim | √ | √ | √ | |
16 | 10 | 11 | ||
Anticoagulant | Warfarin | √ | √ | |
1 | 0 | 1 | ||
Anticonvulsants | Pregabalin | √ | ||
1 | 0 | 0 | ||
Antidiabetic | Glibenclamide | √ | √ | |
Metformin | √ | √ | ||
Sitagliptin | √ | |||
3 | 0 | 2 | ||
Antiepileptics | Gabapentin | √ | √ | |
Primidone | √ | |||
2 | 0 | 1 | ||
Antifungic | Fluconazole | √ | √ | |
1 | 0 | 1 | ||
Antihelmintics | Albendazole | √ | √ | |
Levamisole | √ | √ | ||
Mebendazole | √ | √ | ||
3 | 1 | 2 | ||
Antihypertensives | Amlodipine | √ | √ | |
Enalapril | √ | √ | ||
Irbesartan | √ | |||
Losartan | √ | √ | ||
Valsartan | √ | √ | ||
5 | 4 | 0 | ||
Antiplatelet agent | Clopidogrel | √ | √ | |
1 | 1 | 0 | ||
Antiviral | Nevirapine | √ | ||
1 | 0 | 0 | ||
Calcium channel blockers | Diltiazem | √ | √ | |
Norverapamil | √ | |||
Verapamil | √ | √ | ||
2 | 1 | 2 | ||
Diuretic | Chlorthalidone | √ | √ | |
Furosemide | √ | √ | ||
Hydrochlorothiazide | √ | √ | ||
Triamterene | √ | |||
4 | 1 | 2 | ||
Erectile dysfunction | Sildenafil | √ | √ | |
1 | 1 | 0 | ||
Estrogens | 17α-ethinylestradiol | √ | √ | |
17β-estradiol | √ | √ | ||
Estradiol-3-sulfate | √ | |||
Estriol | √ | √ | ||
Estrone | √ | √ | ||
5 | 0 | 4 | ||
Histamine H1 and H2 receptor | Cetirizine | √ | ||
antagonists | Cimetidine | √ | √ | |
Desloratadine | √ | |||
Diphenhydramine | √ | √ | ||
Famotidine | √ | |||
Fexofenadine | √ | |||
Loratadine | √ | √ | ||
Pseudoephedrine | √ | |||
Ranitidine | √ | |||
8 | 2 | 2 | ||
Illicit drugs | Benzoylecgonine | √ | √ | |
Cocaine | √ | √ | ||
2 | 1 | 1 | ||
Lipid regulators and cholesterol | Atorvastatin | √ | √ | |
lowering stating drugs | Bezafibrate | √ | √ | |
Fluvastatin | √ | |||
Gemfibrozil | √ | √ | ||
Pravastatin | √ | |||
3 | 3 | 2 | ||
Progestogens | 17-hydroxyprogesterone | √ | ||
Levonorgestrel | √ | √ | ||
Progesterone | √ | √ | ||
3 | 0 | 2 | ||
Prostatic hyperplasia | Tamsulosin | √ | √ | |
1 | 1 | 0 | ||
Psychiatric drugs | 10,11-dihydro-10,11-dihydroxycarbamazepine | √ | ||
10-hydroxy-10,11-dihydroxycarbamazepine | √ | |||
2-hydroxy-Carbamazepine | √ | √ | ||
Acridone | √ | √ | ||
Alprazolam | √ | √ | ||
Amitriptyline | √ | √ | ||
Bupropion | √ | |||
Carbamazepine | √ | √ | √ | |
Citalopram | √ | √ | ||
Desvenlafaxine | √ | |||
Diazepam | √ | √ | √ | |
Epoxy-carbamazpine | √ | √ | ||
Escitalopram | √ | |||
Fluoxetine | √ | √ | ||
Lorazepam | √ | √ | ||
Norfluoxetine | √ | √ | ||
O-desmethyl-Venlafaxine | √ | |||
Olanzapine | √ | |||
Oxcarbamazepine | √ | |||
Sertraline | √ | √ | √ | |
Trazodone | √ | √ | ||
Venlafaxine | √ | √ | √ | |
21 | 13 | 6 | ||
Stimulants/lifestyle | 1,7-dimethylxanthine | √ | ||
Caffeine | √ | √ | √ | |
2 | 1 | 1 | ||
Synthetic glucocorticoid | Dexamethasone | √ | √ | |
0 | 1 | 1 | ||
To treat asthma | Salbutamol | √ | √ | |
Terbutaline | √ | |||
2 | 1 | 0 | ||
To treat cancer | Tamoxifen | √ | √ | |
1 | 0 | 1 | ||
Tranquilizer | Azaperone | √ | ||
0 | 1 | 0 | ||
β-Blocking agents | Atenolol | √ | √ | √ |
Carazolol | √ | |||
Metoprolol | √ | √ | √ | |
Nadolol | √ | √ | ||
Propranolol | √ | √ | √ | |
Sotalol | √ | √ | ||
5 | 6 | 3 | ||
Total | 113 | 57 | 55 |
Another therapeutic group with high concentrations was stimulants/lifestyle [Figure 2], consisting mainly of caffeine (2.0-129,585 ng/L). This result makes sense considering that caffeine is used in combination with other groups that also showed high concentrations in the environment. Besides, its uptake with drinks and foods caused its inclusion on the U.S. EPA list of High Production Volume Chemicals. For all these reasons, it is usually considered as an anthropogenic marker in aquatic systems, but mainly because it is ubiquitous in surface water, seawater and also groundwater[14,31]. Here, we demonstrated that there is a significant association between the mean concentration of PhACs in fresh surface waters and the mean concentration of caffeine at different sites in Latin America [Supplementary Figure 1]. The range of concentrations of this compound in rivers and lakes from North America (3-1440 ng/L) and Europe (50-2,400 ng/L) is similar to the range reported for Latin America, except for two outliers from Brazil (129,585 ng/L[30] and 127,092 ng/L[32]) [Figure 2]. Antidiabetics (1.50-64,100 ng/L) and psychiatric drugs (0.04 ng/L-36,920 ng/L) are the other two therapeutic groups that showed high concentrations [Figure 2], with metformin and carbamazepine leading in each of these groups. Metformin is a compound that appears to act directly or indirectly on the insulin receptor. The maximum concentration measured in surface waters in the United States was 150 ng/L[33], two orders of magnitude lower than in Latin America. Carbamazepine is used primarily for the treatment of epilepsy but also as a psychotropic drug. The range of concentrations in surface waters from North America, Europe, South Africa, and China (0.2-11.600 ng/L)[14] is similar to the range reported for Latin America, with the exception of an outlier from Colombia (36,920 ng/L)[34].
Another therapeutic group that deserves special mention is that of antibiotics, which includes all natural or synthetic compounds that have a selective toxic effect on bacteria or other unicellular microorganisms[11]. It consists of a very large group of compounds with different physicochemical properties that have been detected in surface waters from Latin America [0.6-25,000 ng/L, Figures 1 and 2] at lower concentrations than the previous therapeutic groups, as found in North America (30-1,900 ng/L)[33,35], Europe (0.5-29,000 ng/L)[9,36,37] and Africa (34-21,000)[9]. Among this therapeutic group, the higher concentrations for Latin America belong to clarithromycin (3-25,000 ng/L)[38,39], sulfamethoxazole (5-6,550 ng/L)[10,40], and trimethoprim (2-3,580 ng/L)[10,34]. The maximum concentration of clarithromycin is one or two orders of magnitude higher than those reported for North America (857 ng/L), Europe (186 ng/L), Asia (177 ng/L), and Africa (241 ng/L)[10]. The same is true when comparing the maximum concentration of trimethoprim reported for Latin America with the values reported in North America (61 ng/L), Europe (36 ng/L), Asia (44 ng/L), and Africa (287 ng/L)[10]. For sulfamethoxazole, the maximum concentration is an order of magnitude higher than the levels reported for North America (111 ng/L) and Asia (200 ng/L)[10]. The estimated supply of over-the-counter antibiotics is 62% worldwide, which corresponds to 65% for Asia, 53% for Southern and Eastern Europe, 57% for Africa, and 78% for South America[41]. However, it would be inappropriate to associate the high concentrations of antibiotics in Latin America with better access to over-the-counter antibiotics for two reasons: 1: the range of antibiotic concentrations found in the region is similar to other regions of the world, as mentioned earlier, 2: the article from which this percentage was estimated for Latin America is based on data from only 3 countries (representing less than 1% of the region). Therefore, more variables need to be evaluated to determine the reasons why some PhACs are detected at higher concentrations than others.
Looking at the 33 countries in Latin America, concentrations of PhACs in fresh surface water were registered in less than 40% of them, with Brazil (24 papers quantifying 47 different compounds), Argentina (10 papers quantifying 56 different compounds and 20 unpublished new compounds) and Mexico (5 papers quantifying 24 different compounds) topping the list [Figures 1 and 2]. Concentrations greater than 2500 ng/L were detected in all countries where PhACs were measured (n = 12), but only seven of them had concentrations greater than 5000 ng/L. Although investment in research and development in this region has steadily increased in recent years, only three countries account for 88% (Brazil = 64%, Mexico = 17%, and Argentina = 7%)[42]. Thus, the lack of studies in the region seems to be related to the lack of government support.
The largest number of PhACs was reported in Argentina (n = 76). However, it should be noted that this number includes 20 new PhACs recently reported in an unpublished research article[43] by one of the authors of this review. However, ignoring this article, Argentina still tops the list (n = 56), although the number of PhACs reported in Brazil is not so different (n = 47). In other words, Argentina and Brazil are the countries with the most reported PhACs. However, when considering the number of articles published in each country, the number in Brazil is two times higher than that in Argentina. This is probably due not only to the analytical capabilities of each country, but also to the objectives of each study. It should also be noted that in Argentina, all measurements were made in the central region of the country, whereas in Brazil, measurements were scattered throughout the national territory. Both patterns are consistent with the concentration of urban areas in each of these countries. Therefore, it is necessary to increase the number of sampling sites to perform measurements in different regions of each country.
Human PhACs accumulated in aquatic biota
Based on the research conducted [n = 8 articles; Supplementary Table 2], all reported concentrations were in the same order of magnitude as most of the previously reported concentrations for aquatic biota worldwide (< 1,000 ng/g d.w.)[44]. Although the highest concentrations are generally found in waters adjacent to areas with direct discharge of treated municipal water or areas with significant water runoff from highly urbanized and populated areas, bioaccumulation has been found in freshwater biota from areas distant from the source of contamination[44,45]. This makes sense because concentrations in aquatic biota are the result of daily and historical exposure to bioavailable PhACs in surface water. In this sense, bioaccumulation is determined by the absorption, distribution, metabolism and excretion capacity of aquatic organisms, in addition to their migration capacity. This raises questions about transportation pathways and persistence of pharmaceuticals in terms of global environmental health risk[44].
In Latin America, 57 PhACs belonging to 18 different therapeutic groups have been reported in aquatic biota. Of these therapeutic groups, 13 PhACs belong to psychiatric drugs, 10 to antibiotics and 8 to analgesics/anti-inflammatories [Table 1, Supplementary Table 2]. The highest reported concentrations belong to antibiotics bioaccumulated in biofilm (5-652 ng/g d.w.)[39,46] and vascular plants (20-112 ng/g d.w.)[46] [Figures 3 and 4]. The antibiotic with the highest concentration was ciprofloxacin (400-652 ng/g d.w. in biofilm)[39] (log Kow = 0.28), as previously reported for cultured and wild organisms[11]. However, the highest concentrations reported in China (3-4 ng/g d.w. in fish muscle and decapods)[44] are two orders of magnitude lower than those reported in Latin America. Thus, the fate and distribution of antibiotics in the environment may depend on other factors and not only on the Kow value, which is considered a good predictor of the bioaccumulative capacity of most compounds. That is, the Kow value is only a chemical property that does not take into account biological and environmental factors such as bioavailability and biotransformation, and it is expected to give many false positives for ionizable polar organics[47]. Another issue to consider is the definition of bioaccumulation, as most studies consider chemical concentrations in an organism that exceed those found in the respiratory medium and the diet[47], whereas some studies refer to the concentration of a compound within cells and in the surrounding matrix[39]. The latter definition has been used to describe the bioaccumulation of antibiotics in biofilm, which may explain the higher concentrations reported.
Figure 3. Maximum concentrations of human pharmaceutically active compounds bioaccumulated in aquatic biota from Latin America.
Figure 4. Total concentrations of human pharmaceutically active compounds, classified by therapeutic group, reported in aquatic biota from different Latin American countries.
Another therapeutic group with high concentrations were analgesics/anti-inflammatories [0.1 ng/g - 163 ng/g d.w., Figure 4], with codeine (163 ng/g d.w. in whole fish)[48], acetaminophen (~80 ng/g d.w. in anuran muscle)[43] and ibuprofen (63 ng/g d.w. in biofilm)[46] leading the group. Of these three PhACs, acetaminophen was the only one whose concentration was two orders of magnitude higher in North America (4,660 ng/g d.w. in fish plasma)[49] than in Latin America. However, the fact that the report was made in plasma could explain the difference in magnitude in terms of tissue accumulation.
Psychiatric drugs (1-90.8 ng/g d.w.) and β-Blocking agents (1-85 ng/g d.w.) were the other two therapeutic groups that showed high concentrations [Figure 4]. For each of these groups, carbamazepine (90.8 ng/g d.w. in fish muscle)[50] and propranolol (85 ng/g d.w. in whole fish)[48] showed the highest concentrations. Notably, psychiatric drugs were the only group reported in all Latin American countries where bioaccumulation studies were conducted. However, as with fresh surface waters, we hypothesize that the lack of reports is related to the lack of government support for conducting studies rather than the actual presence/absence of a particular PhAC in the environment. Among β-Blocking agents, the concentration of propranolol reported for Latin America is two orders of magnitude higher than that reported for Canada (8.3 ng/g w.w. in bivalves)[51].
At the regional level, only 12% (n = 4) of countries recorded concentrations of bioaccumulated PhACs in aquatic biota, with fish being the most studied taxonomic group. Besides, analgesics/anti-inflammatories were reported only in Argentina and Uruguay, and most of the reports of other therapeutic groups were made in Argentina, showing the lack of field studies with wildlife [Figure 3]. That is, Argentina is the Latin American country with the most reported PhACs in aquatic biota, both considering (n = 55) and not considering (n = 34) the previously mentioned unpublished work[43]. Therefore, future studies should be conducted to better understand the range of concentrations of each PhAC and therapeutic group in the region.
Data search criteria: effects of pharmaceuticals on aquatic biota
Data on the lethal and sublethal effects of PhACs on native and non-native aquatic biota were retrieved from the USEPA database (https://cfpub.epa.gov/ecotox/), one of the most important ecotoxicological databases in the world. Only PhACs quantified in fresh surface waters and aquatic biota from Latin America were searched using the keywords listed in Supplementary Table 1. Once the search was completed, each article was read in detail and data from laboratory tests with algae, crustaceans, amphibians and fish were extracted (e.g., lethal and sublethal endpoints, concentration, biological organization level). For each taxonomic group, the lowest concentration of each PhAC that induced lethal and sublethal effects was selected. The cut-off date for this review was 31st December 2022.
Effects of PhACs on aquatic biota
Based on the research conducted, we found 67 no observed effect concentration (NOEC) values for 37 PhACs, 36 lowest observed effect concentration (LOEC) values for 26 PhACs and 45 lethal concentration (LC50) values for 31 PhACs [Supplementary Table 3]. Considering the PhACs for which we found reports in fresh surface water, less than half (50 out of 113) were tested in the laboratory for lethal or sublethal effects on an aquatic organism. Lethal and sublethal effects of most PhACs were evaluated in fish (n = 45) and crustaceans (n = 32), with amphibians (n = 15) and algae (n = 12) being the groups with the least available information [Figure 5]. Looking at the targets for prioritization of compounds, based on the available information, those compounds whose highest environmental concentrations exceed the lowest reported LC50 should be prioritized (high prioritization), followed by those whose highest environmental concentrations exceed the lowest reported LOEC (medium prioritization), and finally, those whose highest environmental concentrations exceed the lowest reported NOEC values (low prioritization). That is, the order of prioritization would be from right to left in the analysis of Figure 5. Specifically, the only compound whose concentration in fresh surface water exceeded the minimum lethal concentration was acetaminophen (lowest LC50 in crustaceans = 32,000 ng/L[52]). Regardless of whether this concentration in water is an outlier value, this is an alarm signal for the need to continue monitoring concentrations of this PhAC in water because of its potential to cause lethal effects. Also, there are several other PhACs for which the reported maximum concentration in fresh surface water exceeds the NOEC (n = 13 out of 33) and/or LOEC (n = 11 out of 20). Specifically, acetaminophen (analgesics/anti-inflammatories), caffeine (stimulants/lifestyle), carbamazepine (psychiatric drugs), propranolol (β-blocking agents), 17α-ethinylestradiol and 17β-estradiol (Estrogens) were the only six PhACs whose NOEC and also their LOEC values were lower than the concentration in fresh surface water [Figure 5]. Therefore, special attention should also be paid to these PhACs. In view of the above, the information available worldwide on the ecotoxicology of PhACs is still scarce [Table 1].
Figure 5. Comparison of the maximum concentration of different pharmaceutically active compounds (PhACs) reported in fresh surface waters from Latin America with the lowest concentrations of each PhAC that induced no effects (NOEC) or sublethal/lethal effects (LOEC, LC50) in laboratory tests with algae, crustaceans, amphibians or fish. W > E: water concentration exceeding at least one NOEC, LOEC, or LC50
CONCLUSIONS
Compared to other parts of the world, there is little information on PhACs contamination of fresh surface waters in Latin America. This situation is exacerbated when considering the available information on the bioaccumulation of PhACs in aquatic biota. In addition, the available information is not systematized, which makes it even more difficult to assess the current situation. At the regional level, Argentina is the country with the largest amount of available information on aquatic contamination with PhACs. Although there is a moderate number of published articles on the subject in this country, a large amount of PhACs has been quantified in both water and biota. However, there is a need to expand sampling sites to areas of the country that have not yet been studied. Brazil is also the country with the most quantity of articles on this topic, but the number of reports of PhACs in water is lower than in Argentina. In addition, there are no reports of bioaccumulation of PhACs in aquatic biota in this country. Thus, as in the other Latin American countries, the focus should be on filling these knowledge gaps.
Based on the research conducted, most of the reported concentrations are in the same order of magnitude as most of the previously reported concentrations in fresh surface water and aquatic biota worldwide. The group with the highest concentrations in fresh surface waters were analgesics/anti-inflammatories, with acetaminophen leading the group, whereas the group with the highest concentrations in aquatic biota were antibiotics that bioaccumulate in biofilm, with ciprofloxacin leading the group. Thus, the fate and distribution of PhACs in the environment may depend on physicochemical and biological factors of individual sites, which may control their bioavailability. The latter may also control the effective environmental exposure of organisms to PhACs, which may bioaccumulate differently depending on the mobility of the organism, lipid content, size and life stage.
Considering all the ecotoxicological information collected, fish are the most studied taxonomic group. Moreover, of all the PhACs for which we have found reports in fresh surface water, less than half have been tested in the laboratory for lethal or sublethal effects on aquatic organisms. Therefore, it is not only necessary to know the bioaccumulation of PhACs in other taxonomic groups to better understand the absorption, distribution, metabolism and excretion capacity of aquatic organisms, but also to know the potential effects of all bioavailable PhACs.
Considering all PhACs that have been reported in water or tested in the laboratory to evaluate lethal or sublethal effects on aquatic organisms, 13 of them could be considered as low priority compounds, 11 as medium priority compounds and one as a high priority compound. In general, there is an urgent need for advanced treatment methods to remove PhACs to ensure the safe disposal of effluents into the environment.
DECLARATIONS
Authors’ contributionsMade substantial contributions to conceptualization, design, and writing of the original draft: Salgado Costa C
Performed data acquisition and writing-reviewing support: Rimoldi F
Performed data acquisition and design of all tables: Peluso L
Performed data acquisition, data analysis, interpretation, and design of all figures: Demetrio P
Availability of data and materialsNot applicable.
Financial support and sponsorshipPart of this research was funded by PICT 2019–1177. All the authors acknowledge the support of the National Council of Scientific and Technical Research (CONICET) and the National University of La Plata (UNLP).
Conflicts of interestAll authors declared that there are no conflicts of interest.
Ethical approval and consent to participateNot applicable.
Consent for publicationNot applicable.
Copyright© The Author(s) 2023.
REFERENCES
1. WWF, World Wildlife Fund. Living Planet Report 2020. Bending the curve of biodiversity loss. 2020: World Wildlife Fund. Available from: https://wwf.panda.org/es/campanas_ambientales/ipv2020/ [Last accessed on 28 Apr 2023].
2. Brundtland, Report of the World Commission on environment and development:"our common future". 1987: United Nations. Available from: http://www.un-documents.net/wced-ocf.htm [Last accessed on 28 Apr 2023].
3. Daughton CG. Non-regulated water contaminants: emerging research. Environmental Impact Assessment Review 2004;24:711-32.
5. Barceló, D., M.J. López de Alda. Contaminación y calidad química del agua: el problema de los contaminantes emergentes. Available from:http://elaguapotable.com/Contaminaci%C3%B3n%20y%20calidad%20qu%C3%ADm%20del%20agua-los%20contaminantes%20emergentes.xlsx [Last accessed on 28 Apr 2023].
6. Boxall AB, Rudd MA, Brooks BW, et al. Pharmaceuticals and personal care products in the environment: what are the big questions? Environ Health Perspect 2012;120:1221-9.
7. Blair BD, Crago JP, Hedman CJ, Klaper RD. Pharmaceuticals and personal care products found in the Great Lakes above concentrations of environmental concern. Chemosphere 2013;93:2116-23.
8. Sui Q, Cao X, Lu S, et al. Occurrence, sources and fate of pharmaceuticals and personal care products in the groundwater: a review. Emerging Contaminants 2015;1:14-24.
9. aus der Beek T, Weber FA, Bergmann A, et al. Pharmaceuticals in the environment--global occurrences and perspectives. Environ Toxicol Chem 2016;35:823-35.
10. Wilkinson JL, Boxall ABA, Kolpin DW, et al. Pharmaceutical pollution of the world's rivers. Proc Natl Acad Sci USA 2022:119.
11. Puckowski A, Mioduszewska K, Łukaszewicz P, et al. Bioaccumulation and analytics of pharmaceutical residues in the environment: a review. J Pharm Biomed Anal 2016;127:232-55.
12. Huerta B, Rodriguez-Mozaz S, Lazorchak J, et al. Presence of pharmaceuticals in fish collected from urban rivers in the U.S. EPA 2008-2009 national rivers and streams assessment. Sci Total Environ 2018;634:542-9.
13. Ankley GT, Brooks BW, Huggett DB, Sumpter JP. Repeating history: pharmaceuticals in the environment. Environ Sci Technol 2007;41:8211-7.
14. Fent K, Weston AA, Caminada D. Ecotoxicology of human pharmaceuticals. Aquat Toxicol 2006;76:122-59.
15. Weinberger J 2nd, Klaper R. Environmental concentrations of the selective serotonin reuptake inhibitor fluoxetine impact specific behaviors involved in reproduction, feeding and predator avoidance in the fish Pimephales promelas (fathead minnow). Aquat Toxicol 2014;151:77-83.
16. Halling-Sørensen B, Nors Nielsen S, Lanzky PF, Ingerslev F, Holten Lützhøft HC, Jørgensen SE. Occurrence, fate and effects of pharmaceutical substances in the environment--a review. Chemosphere 1998;36:357-93.
17. Flaherty CM, Dodson SI. Effects of pharmaceuticals on Daphnia survival, growth, and reproduction. Chemosphere 2005;61:200-7.
18. Arnaud MJ. The pharmacology of caffeine. In: Jucker E, Meyer U, editors. Progress in drug research/fortschritte der Arzneimittelforschung/Progrès des recherches pharmaceutiques. Basel: Birkhäuser; 1987. pp. 273-313.
19. Vethaak AD, Lahr J, Schrap SM, et al. An integrated assessment of estrogenic contamination and biological effects in the aquatic environment of The Netherlands. Chemosphere 2005;59:511-24.
20. Daughton CG, Ternes TA. Pharmaceuticals and personal care products in the environment: agents of subtle change? Environ Health Perspect 1999;107 Suppl 6:907-38.
21. Delgado N, Capparelli A, Navarro A, Marino D. Pharmaceutical emerging pollutants removal from water using powdered activated carbon: Study of kinetics and adsorption equilibrium. J Environ Manage 2019;236:301-8.
22. Delgado N, Navarro A, Marino D, Peñuela GA, Ronco A. Removal of pharmaceuticals and personal care products from domestic wastewater using rotating biological contactors. Int J Environ Sci Technol 2019;16:1-10.
23. Delgado N, Bermeo L, Hoyos DA, et al. Occurrence and removal of pharmaceutical and personal care products using subsurface horizontal flow constructed wetlands. Water Res 2020;187:116448.
24. Delgado N, Marino D, Capparelli A, Casas-zapata JC, Navarro A. Pharmaceutical compound removal using down-flow fixed bed filters with powder activated carbon: a novel configuration. Journal of Environmental Chemical Engineering 2022;10:107706.
25. R Core Team. R: a language and environment for statistical computing. Viena; 2022. Available from: http://www.R-project.org/ Available from: http://www.R-project.org/[Last accessed on 28 Apr 2023].
28. Kahle, D.J, H. Wickham. ggmap: Spatial visualization with ggplot2. The R Journal; 2013. 5: p. 144-161. Available from: https://journal.r-project.org/archive/2013-1/kahle-wickham.xlsx [Last accessed on 28 Apr 2023].
29. Batucan NSP, Tremblay LA, Northcott GL, Matthaei CD. Medicating the environment? Environmental Advances 2022;7:100164.
30. Campanha MB, Awan AT, de Sousa DN, et al. A 3-year study on occurrence of emerging contaminants in an urban stream of São Paulo State of Southeast Brazil. Environ Sci Pollut Res Int 2015;22:7936-47.
31. Buerge II, Poiger T, Müller MD, Buser HR. Caffeine, an anthropogenic marker for wastewater comtamination of surface waters. Environ Sci Technol 2003;37:691-700.
32. Montagner CC, Jardim WF. Spatial and seasonal variations of pharmaceuticals and endocrine disruptors in the Atibaia River, São Paulo State (Brazil). J Braz Chem Soc 2011;22:1452-62.
33. Kolpin DW, Furlong ET, Meyer MT, et al. Pharmaceuticals, hormones, and other organic wastewater contaminants in U.S. streams, 1999-2000: a national reconnaissance. Environ Sci Technol 2002;36:1202-11.
34. Bedoya-Ríos DF, Lara-Borrero JA, Duque-Pardo V, Madera-Parra CA, Jimenez EM, Toro AF. Study of the occurrence and ecosystem danger of selected endocrine disruptors in the urban water cycle of the city of Bogotá, Colombia. J Environ Sci Health A Tox Hazard Subst Environ Eng 2018;53:317-25.
35. Batt AL, Kincaid TM, Kostich MS, Lazorchak JM, Olsen AR. Evaluating the extent of pharmaceuticals in surface waters of the United States using a national-scale rivers and streams assessment survey. Environ Toxicol Chem 2016;35:874-81.
36. Burns EE, Thomas-Oates J, Kolpin DW, Furlong ET, Boxall ABA. Are exposure predictions, used for the prioritization of pharmaceuticals in the environment, fit for purpose? Environ Toxicol Chem 2017;36:2823-32.
37. Gros M, Rodríguez-Mozaz S, Barceló D. Fast and comprehensive multi-residue analysis of a broad range of human and veterinary pharmaceuticals and some of their metabolites in surface and treated waters by ultra-high-performance liquid chromatography coupled to quadrupole-linear ion trap tandem mass spectrometry. J Chromatogr A 2012;1248:104-21.
38. Elorriaga Y, Marino DJ, Carriquiriborde P, Ronco AE. Human pharmaceuticals in wastewaters from urbanized areas of Argentina. Bull Environ Contam Toxicol 2013;90:397-400.
39. Valdés ME, Santos LHMLM, Rodríguez Castro MC, et al. Distribution of antibiotics in water, sediments and biofilm in an urban river (Córdoba, Argentina, LA). Environ Pollut 2021;269:116133.
40. Archundia D, Duwig C, Lehembre F, et al. Antibiotic pollution in the Katari subcatchment of the Titicaca Lake: major transformation products and occurrence of resistance genes. Sci Total Environ 2017;576:671-82.
41. Auta A, Hadi MA, Oga E, et al. Global access to antibiotics without prescription in community pharmacies: a systematic review and meta-analysis. J Infect 2019;78:8-18.
42. Enrique LE LE, Núñez Jover JR, Hernández Alfonso EA EA, et al. Pensamiento latinoamericano en ciencia, tecnología e innovación: políticas, determinantes y prácticas. Desde el Sur 2022;14:e0008.
43. Salgado Costa, C, et al. First evidence of environmental bioaccumulation of pharmaceuticals on adult native anurans (Rhinella arenarum) from Argentina. Forthcoming 2023.
44. Świacka K, Maculewicz J, Kowalska D, Caban M, Smolarz K, Świeżak J. Presence of pharmaceuticals and their metabolites in wild-living aquatic organisms - current state of knowledge. J Hazard Mater 2022;424:127350.
45. Ondarza PM, Haddad SP, Avigliano E, Miglioranza KSB, Brooks BW. Pharmaceuticals, illicit drugs and their metabolites in fish from Argentina: implications for protected areas influenced by urbanization. Sci Total Environ 2019;649:1029-37.
46. Mastrángelo MM, Valdés ME, Eissa B, et al. Occurrence and accumulation of pharmaceutical products in water and biota of urban lowland rivers. Sci Total Environ 2022;828:154303.
47. Gobas FA, de Wolf W, Burkhard LP, Verbruggen E, Plotzke K. Revisiting bioaccumulation criteria for POPs and PBT assessments. Integr Environ Assess Manag 2009;5:624-37.
48. Valdés ME, Huerta B, Wunderlin DA, Bistoni MA, Barceló D, Rodriguez-Mozaz S. Bioaccumulation and bioconcentration of carbamazepine and other pharmaceuticals in fish under field and controlled laboratory experiments. evidences of carbamazepine metabolization by fish. Sci Total Environ 2016;557-558:58-67.
49. Bean TG, Rattner BA, Lazarus RS, et al. Pharmaceuticals in water, fish and osprey nestlings in delaware river and bay. Environ Pollut 2018;232:533-45.
50. Cacua-Ortiz SM, Aguirre NJ, Peñuela GA. Methyl paraben and carbamazepine in water and striped catfish (Pseudoplatystoma magdaleniatum) in the cauca and magdalena rivers. Bull Environ Contam Toxicol 2020;105:819-26.
51. de Solla SR, Gilroy ÈA, Klinck JS, et al. Bioaccumulation of pharmaceuticals and personal care products in the unionid mussel Lasmigona costata in a river receiving wastewater effluent. Chemosphere 2016;146:486-96.
Cite This Article

How to Cite
Download Citation
Export Citation File:
Type of Import
Tips on Downloading Citation
Citation Manager File Format
Type of Import
Direct Import: When the Direct Import option is selected (the default state), a dialogue box will give you the option to Save or Open the downloaded citation data. Choosing Open will either launch your citation manager or give you a choice of applications with which to use the metadata. The Save option saves the file locally for later use.
Indirect Import: When the Indirect Import option is selected, the metadata is displayed and may be copied and pasted as needed.
About This Article
Special Issue
Copyright
Data & Comments
Data
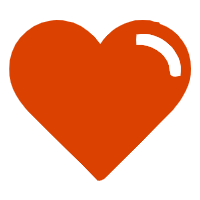
Comments
Comments must be written in English. Spam, offensive content, impersonation, and private information will not be permitted. If any comment is reported and identified as inappropriate content by OAE staff, the comment will be removed without notice. If you have any queries or need any help, please contact us at [email protected].