35 years of lessons learned designing, building, and monitoring passive treatment systems for mining influenced water (part 1)
Abstract
While natural processes have been remediating acidic water exhibiting elevated dissolved metals for eons, engineers and scientists have been trying to mimic nature in the treatment of mining influenced water for only about 50 years. The technical community has adopted the term “passive treatment” to describe the technology. The design of passive treatment systems is based on the application of multidisciplinary engineering sciences including biogeochemistry, hydraulics, physics, microbiology, and agronomics to name a few. Consequently, there are many opportunities to not quite “get it right” in passive treatment system design, construction, and operation. Fortunately, Mother Nature can be quite forgiving and sometimes a design or operational hiccup can reveal new insights. The case histories presented in this review reflect the learning experiences of a mining engineer whose technical education barely touched on the fundamentals of passive treatment system design. Early system designs were often based on the findings of other practitioners who were gracious enough to share their knowledge at
Key takeaways from the lessons learned by this author are:
● Biochemical reactors (BCRs) can be overloaded, but only for a short time to avoid permanent harm to the microbial community.
● Other metal removal processes can substitute for sulfate reduction in overloading situations but the beneficial effects may be reversible.
● Media material substitution risk in full scale construction can be lessened if bench or pilot data is available.
● BCRs are feasible in cold climates.
● The age of woody material (fresh vs. moldy) in BCR media is inconsequential.
● Allow plants to grow on BCR surfaces with caution.
● Excessive aluminum concentrations do not cause BCR media to plug.
● Metal adsorption to manganese oxide coatings may be a viable alternative to sulfide precipitation in BCRs.
● Mixing bypassed mining influenced water with BCR effluent may offer benefits in a freshet.
● A test failure may be due to an improper design and should not condemn the passive treatment concept.
● In final design and construction, avoid deviating from the bench- or pilot-scale test conditions.
Keywords
INTRODUCTION
A core of pioneering passive treatment practitioners comprising engineers, scientists, and academia recently published a comprehensive history of passive treatment from a North American perspective[1]. The combined bibliography of these pioneers would be a volume in itself. Consequently, the reader would be well served to review this publication prior to proceeding much further as it contains a storied timeline of progress in the development of passive treatment processes as a mature technology that is basically well understood about 50 years on.
This review delves deeper into the last 35 years of passive treatment development from the perspective of a mining engineer whose career took an unexpected path toward mined land reclamation and remediation in the late 1980s. Since then, a series of bench-, pilot-, demonstration-, and full scale passive treatment projects have yielded lessons learned that will be discussed in this review via a series of mini-case histories. The author assumes that the reader is already very familiar with passive treatment processes; it is not intended to be an introductory primer as there are numerous publications and online information that satisfy that knowledge gap. For example, the biochemical reactor (BCR) technology, which is used in most of the forthcoming mini-case histories, is addressed by the Interstate Technology Regulatory Council[2]. This reference, to which this author contributed, provides advantages and disadvantages of the BCR technology and other details.
These selected projects have been the topic of technical papers or presentations, and the reader is directed to individual references for more information on a given site. Unfortunately, some of these references were published pre-internet and may only be available in paper copy and do not possess DOIs or URLs; sharing scans of these paper documents electronically may violate copyrights.
The common thread among the mini-case histories discussed is that most are from bench- and pilot-scale testing programs, which reinforces the benefits of small-scale testing as an economical way to accumulate valuable lessons learned experience with minimum risk.
The act of learning a given lesson does not necessarily suggest system failure which was previously addressed by Gusek[3], who discussed four major reasons why some passive treatment systems do not function as intended:
● No design. E.g., “Just build a swamp here, fill that pond over there with manure and call it good”.
● Inadequate design. Undersized for contaminant mass loads, applying the wrong geochemical approach, phased design lacking, complex geochemistry, improper startup and operational procedures.
● Inadequate maintenance. (Low maintenance does not mean no maintenance).
● Last minute design changes. Departure from well-conceived construction specifications in response to field conditions can affect system performance - experience and bench-scale testing of alternative materials helps.
Problems related to the first bullet are rare due to the sharing of data and information on the internet and in conference proceedings. Project examples related to the last three bullets follow.
Passive treatment process review
Before delving into lessons learned, a baseline definition of passive treatment should be considered. To paraphrase Gusek[4], “Passive treatment is a process of sequentially removing contaminants and/or acidity from mining influenced water (MIW) in a natural-looking, man-made bio-system that capitalizes on ecological, and/or geochemical reactions coupled with physical sequestration. The process does not require power or chemicals after construction, and lasts for decades with minimal human help”.
While practitioners have developed many different names for passive treatment process steps, the processes generally fall into three categories that coincide with the oxidation-reduction potential (ORP) conditions or if the process involves a biological component.
● Aerobic processes that introduce oxygen or capitalize on oxygen already present in the MIW are typically applicable when iron and manganese and mild acidity are problematic and/or only trace concentrations of dissolved metals occur. Aerobic process units may contain cattails, other plants, and algae. This process can also polish total organic carbon from anaerobic processes and foster the adsorption of trace metals onto iron or manganese oxides[5]. Aerobic processes can also be used as a pre-treatment step for very acidic MIWs[6].
● Anaerobic processes typically involve sulfate reducing bacteria symbiotically functioning with cellulose degrading bacteria and/or fungi to produce sulfide ion that reacts with dissolved metals to form insoluble sulfides that are retained within the organic media. Bicarbonate ion, which can buffer the MIW, is also produced in this process. Under certain conditions, sulfate ion is removed as elemental sulfur; nitrate can be removed with denitrifying microbes.
● Abiotic processes can involve simple neutralization with alkalinity sources such as limestone or industrial by-products such as steel slag. In some situations[7], the limestone can function as an ion exchange media.
Most passive treatment systems employ one or more of these processes, typically in sequence.
CASE HISTORIES/PROJECTS
Details of lessons learned in these thumbnail case histories are provided in the cited reference(s) associated with the project; a summary paragraph of the project is provided. With some exceptions, most of the projects involved BCRs as the primary treatment process.
Big 5 Tunnel pilot BCRs cells B & E[8]
Location/Elevation: Colorado, USA, 2,230 m.
Date window: 1987 to 1991.
MIW chemistry: 3.0 pH; Fe, 40 mg/L; Zn, ~9 mg/L; Cu, 0.9 mg/L Mn, ~30 mg/L.
Flow: 0.85 to 3.8 L/min per process unit.
Site/Project summary: This was a research project by the Colorado School of Mines funded under a USEPA grant. As shown in Figure 1, the pilot-scale system was comprised of four BCRs that received MIW from an abandoned metal mine tunnel. Cell D was configured as an aerobic polishing zone; it received the discharge from Cell A. MIW drained by gravity from the tunnel portal to the left, out of view in Figure 1, and then distributed to each of the four BCR cells. Cell A was horizontal (plug) flow; Cell B (originally configured as plug flow) was retrofitted to vertical flow (upwards and downwards); Cell C was plug flow, and Cell E was vertical flow (downwards). This was the earliest BCR system built; data supporting the benchmark value of 0.3 moles/day/m3 of media for metals and sulfate removal was collected here.
Figure 1. Big five tunnel pilot passive treatment system and system schematic[8].
Lessons learned:
● MIW tends to flow over the top of a horizontal BCR. Vertical flow (downward) BCRs are easier to build and operate than horizontal plug flow BCRs as the MIW has more intimate contact with the media.
● BCR Cell E was filled with media that had previously been placed in Cell B. Despite being disturbed in the move from Cell B to E and being allowed to partially dry, the functionality of the media was not compromised and Cell E outperformed the retrofitted Cell B which had been subdivided into upflow and downflow zones.
Burleigh Tunnel pilot BCRs[3,9]
Location/Elevation: Colorado, USA, 2,800 m.
Date window: 1994 to 1995.
MIW chemistry: 7.1 pH; Zn, 45 to 65 mg/L; Fe, 0.3 mg/L; Cd, 0.09 mg/L.
Flow: 26.5 L/min per process unit.
Site summary: This early 20th century mine drainage tunnel is connected to multiple near-vertical vein structures and other mine workings [Figure 2]. The BCR design included two identical BCRs: one upflow and the other downflow. It was believed the flows and chemistry were relatively constant but the system was exposed to an unusual freshet overloading event for four months while the flow tripled and the zinc concentration increased to 100 mg/L (~6X design metal load). The media was primarily fresh mushroom compost (96% by weight) and hay (4%).
Lessons learned:
● If the MIW is circumneutral, the media thickness can be increased and the area footprint can be decreased for a given metal load; BCR design surface area is a function of MIW acidity. Substantiated by A. Rose[10] in 2005.
● The addition of limestone to BCR media should be considered for “insurance” even in circumneutral situations due to the potential of acidity overloading. It also suppresses fermenting bacteria which can lower pH.
● When a BCR is consistently overloaded, it should be allowed to re-incubate and/or be re-inoculated with sulfate reducing bacteria before full design flow is restored.
● Mushroom compost lost permeability over time due to humification; a woody component to the organic media might overcome this physical limitation to media longevity.
Gold mine waste rock dump leachate pilot BCR[11]
Location/Elevation: Western USA, 1,937 m.
Date window: 1993 to 1994.
MIW chemistry: 2.4 pH; Fe, 200 mg/L; As, 2.7 mg/L; Cu, 7.4 mg/L; Mn 6.7 mg/L; Zn, 1.8 mg/L; Sulfate, 2,160 mg/L.
Flow: 23 L/min.
Site summary: This now closed open pit gold mine discharged acid rock drainage (ARD) from a fully revegetated waste rock disposal area containing sulphidic rock. The site was developed by one of the major mining companies. Static Proof of Concept testing results indicated that a BCR could address the Constituents of Concern (COCs) from a biogeochemical testing perspective. Despite recommendations to conduct follow-on bench-scale testing, the company elected to skip bench testing and proceed to a pilot test. While initial performance results were promising, the BCR media contained a significant amount of fines, as suggested by the dust evident in the construction photo [Figure 3]. When the media permeability decreased to the point that the BCR could not accept the design flow, the BCR was abandoned in place and the BCR technology was declared a failure by the mining company. For years, the mining company decision makers continued to believe that “passive treatment systems don’t work” based on this single project.
Lessons learned:
● Skipping bench-scale testing of BCR media introduces a high level of design risk unless the media mixture has been shown to work at other sites.
● Project failure can be unfairly attributed to process failure instead of design failure that could have been averted with bench testing.
● A BCR medium may provide all the necessary ingredients to support a robust microbial community but the grain size distribution of those ingredients needs to be considered in the design phase.
Brewer Mine leach pad pilot BCR[12]
Location/Elevation: South Carolina, USA, 176 m.
Date window: 1993 to 1995 (18 months).
MIW chemistry: Classic ARD; pH, 2.0 to 4.7 s.u.; Fe, 25 to 3,950 mg/L; Cu, 2 to 103 mg/L; Al, 3.6 to
Flow: 3.8 L/min.
Site summary: This open pit gold mine (now a Superfund site) discharged ARD from a spent cyanide heap leach pad containing sulphidic ore. The Pad 5 BCR was filled with a mixture of composted turkey manure, sawdust, phosphate rock reject (limestone), and cow manure inoculum with a top dressing of insulating hay. The pad had been rinsed to reduce cyanide concentrations but its effluent had turned acidic. During the pilot test, two things happened: seeds in hay germinated, as seen in Figure 4, and the site received a
Lessons learned:
● Heavy rainfall on the surface of a downflow configured BCR can dilute sulfate concentrations enough to cause stress to the sulfate reducing bacteria (SRB) microbial community.
● Plants growing on top of a downflow BCR can introduce enough oxygen into the media to form aerobic conditions that suppress bacterial sulfate reduction.
● Temporarily covering the BCR with geomembrane to suppress the plants will induce the plant matter to decay and become a labile nutrient source for the SRB microbial community.
West Fork Mine pilot and full scale BCRs[13,14]
Location/Elevation: Missouri, USA, 275 m.
Date window: 1996 to 2000.
MIW chemistry: Neutral pH; Pb, 0.4 mg/L; Zn, 0.36 mg/L; the Pb discharge limit was 23 ppb.
Flow: Pilot, 95 L/min; Full, 4.54 m3/min.
Site summary: A BCR system at this active underground lead mine operated for about 19 years before being decommissioned due to a mine subsidence event nearby. Initially built in 1995, the design was based on a pilot BCR and bench-scale test results. The media, comprised of a mixture of sawdust, inert limestone sand, alfalfa hay, and cow manure, was about two meters thick and separated into three zones in the final design. To allow maximum operational flexibility especially during hot summer months, each zone was fitted with a perforated pipe network that could function to deliver untreated MIW or to collect treated mine water in either upflow or downflow configurations. Each pipe network zone was underlain by a layer of white geotextile and geonet, as shown in the construction photo [Figure 5]. The interior zone plumbing design was driven by regulatory concerns; it was not part of the pilot BCR that operated for two years.
Figure 5. BCR media placement showing white geotextile that impeded MIW percolation due to gas lock phenomenon and an aerial view of the system. BCR: Biochemical reactor; MIW: mining influenced water.
Lessons learned:
● Within six weeks of startup, the apparent media permeability had decreased to unacceptable levels for steady-state operation. While the last-minute substitution of alfalfa pellets instead of baled alfalfa (that was used in the pilot) in the media mixture was likely a contributing factor, H2S gas buildup underneath the geotextile layers appeared to be causing a “gas-lock” condition that impeded downward percolation of MIW through the media. The condition was remedied with a trencher that breached the two uppermost geotextile/geonet horizons.
● A robust stand of cattail plants established itself on the pilot BCR. Unlike the Brewer BCR, this stand of vegetation did not appear to affect ORP or pilot cell performance. Vegetation did not populate the full scale BCRs immediately after construction and an algal mat that formed on the BCR surface. The mat impeded downward flow, especially toward the end of the summer. Until a stand of willows established itself on the BCRs, the upper surface was rototilled annually to break up the algal mat and restore media permeability.
● The primary lesson learned is to avoid straying too far from bench or pilot design when scaling up BCRs. For example, bench BCR tests should include diverse media component sources (various hay, straw, or wood chip/sawdust vendors) so the risks of construction substitutions are low. If summertime heating is a concern, consider a reflective floating cover that could also protect the BCR during the winter.
● In retrospect, an aerobic wetland designed to remove manganese as an oxide coating on inert gravel to which the lead would adsorb might have been considered as an alternative passive process. A similar system was constructed at the nearby Buick Mine tailings facility in about 1970 and it appears to still be functioning over 50 years later. However, manganese levels in the West Fork mine effluent may have been too low to make a manganese removal bed feasible as the sole treatment process. However, experience at other nearby sites suggests that a phytochelating[15] process involving algal uptake of metals could enhance metal adsorption to manganese oxide.
Ferris Haggarty Copper Mine bench and pilot BCRs[16]
Location/Elevation: Wyoming, USA, 2,900 m.
Date window: 1998 to 2000.
MIW chemistry: At portal pond, neutral pH; Cu @3 to 6 mg/L @0.1 to 4 °C; sulfate 40 to 100 mg/L.
Bench flow: 3.8 L/min.
Site summary: This high-elevation abandoned underground copper mine has neutral pH, and its discharge is affecting a nearby creek. The mine is accessible only by a 32-km snowmobile trek for nine months out of the year. Flow from the portal varied from 57 to 114 L/min in the winter months to about 102 m3/h or more during the freshet. Copper concentration observed at the portal was fairly constant all year long, somewhat independent of flow rate. A pilot-scale downflow BCR [Figure 6, left] was fabricated using an off-the-shelf backyard swimming pool about 5 m in diameter and 1.2 m deep. The sides of the pilot BCR were insulated with hay bales. Before the onset of winter, the entire unit was protected with a built-from-scratch enclosure equipped with a “snorkel” for winter access [Figure 6, right].
Lessons learned:
● Low temperature (< 10 °C) MIW can be treated in a BCR if the MIW is heated during the incubation process to allow the bacterial community to mature.
● Exposed BCR sidewalls can freeze during the winter but the center of the media mass does not, allowing the BCR to continue functioning. The media is pushed inward by the ice around the perimeter. When the ice zone melts in the spring, a gap between the media and the BCR wall opens, causing a short-circuiting condition. To prevent short-circuiting, BCRs in wintry situations should be either buried or the perimeter sloped to avoid the expanding ice - media compression phenomenon.
● During the investigation that revealed the perimeter ice zone above, a small test hole (150 mm × 150 mm) was excavated in the BCR media. Below a depth of about 300 mm, what appeared to be fresh green hay was observed in the media. This suggested that in a downflow BCR, much of the biological activity (sulfate reduction) is likely occurring in the uppermost zone of the media rather than throughout the entire media mass. Other researchers confirmed this about a decade later[17].
Judy 14 Mine demonstration BCR[18]
Location/Elevation: Pennsylvania, USA, 343 m.
Date window: 2001.
MIW chemistry: Classic Coal Mine ARD; Fe, 20 to 55 mg/L; Al, 24 to 58 mg/L; Zn, 0.86 mg/L; Cu,
Flow: 38 to 207 L/min.
Site summary: The Judy 14 underground coal mine in Indiana County, Pennsylvania, about 75 km east of Pittsburgh, was active from the 1940’s to about 1963. Collapsed portals drain acidic MIW. A demonstration BCR was constructed in 2002 [Figure 7]. During its operation, it was intentionally overloaded by a factor of six and was typically operated at flow rates 50% above design. When metal and sulfate removal performance dropped, timothy hay was added to the surface of the BCR and mixed in. The apparent water depth in Figure 7 may be misleading; it is only about 10 cm deep, which facilitated the placement of the hay.
Lessons learned:
● BCR can sustain overloading by a factor of six. While performance suffers, it can rebound if the overloading event is followed by design loading or less to allow the bacterial community to recover.
● BCR media can be rejuvenated with the addition of organic matter into the upper 10 cm of the media layer.
Dixon Run demonstration BCR[19]
Location/Elevation: Pennsylvania, USA, 343 m.
Date window: 2000-2002.
MIW chemistry: Classic Coal Mine ARD; Fe, 130 mg/L; Al, 30 mg/L; Mn, 2 mg/L, pH 2.8.
Demo flow: 6.5 L/min.
Site summary: Discharge originates in an underground coal mine in Western Pennsylvania which closed in 1985. The mine pool that developed was relatively small and its level was controlled by mine pumps that fed a lime dosing treatment system. The pumping rate varied with local precipitation from about 42 to over
Lessons learned:
● Acidic MIW exhibiting mildly elevated aluminum concentrations (~30 mg/L) will not plug up with aluminum hydroxide precipitates. This assertion was validated by Thomas and Romanek[17].
● Introducing acidic MIW into a BCR lacking a standing water cover (i.e., into the media) can induce
Fran Coal Mine pilot BCR[20,21]
Location/Elevation: Pennsylvania, USA, 473 m.
Date window: 2001-2002.
MIW chemistry: Extreme Coal Mine ARD; Fe, 185 to 792 mg/L; Al, 221 to 343 mg/L; Cu, 0.6 to 1.8 mg/L; Zn, 2 to
Pilot flow: 3.8 L/min.
Site summary: Until the 15ha backfilled and reclaimed surface coal mine was remediated in 2019 at a cost of about $US 7 million[22], the seepage from this site was considered to exhibit the worst acidic chemistry in the State of Pennsylvania [Figure 9, left]. Its effluent destroyed nearly 10 km of three otherwise pristine streams which are tributaries of the Susquehanna River. Bench- and pilot-scale BCR studies were conducted in 2001 and 2002-2003, respectively. The pilot BCR, which was buried to protect it from winter temperatures, is shown under construction in [Figure 9, right].
Figure 9. Fran Mine ARD and pilot BCR under construction prior to burial. BCR: Biochemical reactor; ARD: acid rock drainage.
Lessons learned:
● Very acidic MIW exhibiting elevated aluminum concentrations (~340 mg/L) will not plug up with aluminum precipitates. Again, validated by Thomas and Romanek[15].
● Unless adequate ditching and/or drainage is provided, even with a buried BCR, a BCR can capture acidic surface water runoff that can result in 250% overloading during rainy intervals.
● A BCR can recover after an overloading event if the flow and loading are reduced to less than the design values.
Lutrelle Repository bench BCR[7,23]
Location/Elevation: Montana, USA, 2,320 m.
Date window: 2002.
MIW chemistry: Classic ARD; Fe, 185 to 792 mg/L; Al, 221 to 343 mg/L; Cu, 0.6 to 1.8 mg/L; Zn, 2 to 6.4 mg/L; acidity, 2,000 to 4,400 mg/L; sulfate, 2,140 to 5,000 mg/L; pH 2.3.
Bench flow: 5.2 L/day per barrel initially, then decreased to 3.5 L/day.
Site summary: Leachate from a backfilled open pit filled with mine waste collected from multiple mine sites in the Basin Creek Superfund Site was being treated with reverse osmosis and barium for sulfate removal. The EPA wished to minimize costs and commission a demonstration BCR. A series of four bench BCRs in 132-liter plastic barrels was constructed and monitored on site for 13 weeks [Figure 10, left]. Initial MIW chemistry data was outdated, and laboratory lag time prevented receiving true influent chemistry for six weeks. At that time, it was determined that the bench BCRs were being overloaded and the flow was decreased to 67% of the initial rate in the hope that the cells would recover. As they did, the BCR effluents exhibited metals concentrations that were greater than the influent concentrations. The test identified two preferred media mixtures that performed almost identically well; the recipe used in constructing the demonstration BCR [Figure 10, right] was selected by flipping a coin but then changed to the other mixture when some media components were found to be in short supply.
Lessons learned:
● BCR bench tests that yield similar performance results can provide design flexibility during construction because the risks of material substitution in shortage situations are lowered.
● BCRs can recover from overloading, but when they do, they will release metals that may have been sequestered as carbonates (Zn, Fe) until sulfate reduction rates recover to sequester them as sulfides.
Golinsky Mine pilot BCR[24,25]
Location/Elevation: California, USA, 480 m.
Date window: 2004.
MIW chemistry: Classic ARD; the mine pool behind a draining bulkhead is typical acidic MIW exhibiting a pH of 2.5 to 4 containing metals including iron, 104 mg/L; aluminum, 25 mg/L; copper, 9 mg/L; zinc,
Pilot flow: 3.4 up to 7.2 L/min.
Site summary: The Golinsky site is a small underground copper mine complex consisting of abandoned mine workings and remnants of smelter operations located on a steep hillside above Little Backbone Creek, a tributary to Lake Shasta. Bench- and pilot-scale testing programs were conducted to demonstrate that the BCR technology would work at the remote site and reduce metal loading (primarily copper) on the lake. A pilot-scale BCR [Figure 11, left] was constructed in 2004 and decommissioned in September 2006 after
Lessons learned:
● Unlike previous tests where only the flow increased during an overloading event, both flow and metals concentrations increased. The flow doubled and the metals concentrations increased by 50%, causing a tripling of the contaminant loading for about a month. Metal removal efficiency dropped from a nominal 95+% to about 85% during this time. Flow was subsequently adjusted to the design flow and contaminant removal efficiency improved to pre-overloading levels. It was theorized that the overloading event was brief enough to avoid permanently affecting the sulfate reducing bacteria community.
● The results of the titration test suggested that BCR-treated MIW has geochemical benefits beyond the expected straight dilution effects. That is, the elevated alkalinity and sulfide concentrations of BCR effluent appear to be capable of providing additional treatment of raw MIW in a simple mixing and settling process.
Smolnik Mine bench BCR[26]
Location/Elevation: Slovakia, 582 m.
Date window: 1999.
MIW chemistry: Metal Mine ARD; Fe, 570 mg/L; Al, 120 mg/L; Cu, 3 mg/L; Zn, 16 mg/L; Mg, 400 mg/L; sulfate, 3,500 mg/L; pH 3.9.
Bench flow: 1 to 5 L/day.
Site summary: The Smolnik Mine is one of many sources of ARD from inactive mines and mineral processing facilities located in the Ruzin Basin of Slovakia. In 1999, bench-scale tests were conducted to evaluate the feasibility of applying passive treatment technology to the Smolnik ARD or other similar local mine water discharges. The test work was conducted under a grant from Ecolinks, an arm of the US Agency for International Development (USAID). Four bench-scale test cells [Figure 12, left] were constructed at the Smolnik mine and operated for two months to provide baseline information [Figure 12, right] to design a larger pilot-scale treatment cell.
Lessons learned:
● Two cells had identical media recipes except one used aged sawdust (#2) while the other (#4) used fresh sawdust. Fresh pine was believed to contain excess phenol which would make it difficult for cellulose degrading bacteria to break down. Aged pine was believed to contain more white rot fungi that would facilitate the breakdown of lignin. After the BCR test cells were fully incubated, there was a measurable difference in performance between the two [Figure 12, right]: the fresh sawdust (#4) media slightly
● Interestingly, all four bench BCRs removed magnesium and released calcium, creating dolomite, suggesting that the limestone component of the media was functioning in an ion exchange capacity.
CONCLUSIONS
From the initial BCR research conducted at the Big 5 Tunnel in 1987 to the Golinsky pilot in 2004, a span of 17 years elapsed. The title of the article (Part 1) rightfully infers that a Part 2 covering 2005 to the present may be appropriate. The lessons learned discussed herein in the dozen mini-case studies can be summarized as follows:
● BCRs can be overloaded, but only for a short time to avoid permanent harm to the microbial community which is typically found in the upper 15 to 30 cm in a downflow configured BCR.
● Other metal removal processes can substitute for sulfate reduction in overloading situations, but the beneficial effects may be reversible as metals may be temporarily remobilized as the BCR recovers to anoxic conditions.
● Media material substitution risk in full scale construction can be lessened if bench or pilot data that quantifies the probable effects of proposed substitutions is available.
● BCRs are feasible in cold climates as long as they are incubated in temperate months or the initial incubation MIW is gently heated. Exposed vertical sidewalls should avoided in the final design and surface insulation is recommended, perhaps by burial.
● The age of woody material (fresh vs. moldy) does not appear to affect BCR sulfate or metal removal kinetics, at least at one site.
● Allow plants to grow on BCR surfaces with caution; maintain a complete water cover over BCR media to prevent short-circuiting.
● In contrast to limestone-dominated passive treatment processes, excessive aluminum concentrations do not cause media to plug.
● Metal adsorption to manganese oxide coatings may provide metal removal efficiencies equivalent to those in BCRs at lesser capital and operating costs.
● Mixing bypassed MIW with BCR effluent should be considered in dealing with freshet situations.
● A test failure may be due to an improper design (e.g., overloading or poor media material selection). A failure should not condemn the passive treatment concept.
● In final design and construction, avoid deviating from the bench- or pilot-scale test conditions at all costs.
To quote Louis Pasteur: “In the fields of observation, chance favors only the prepared mind”. The author hopes that passive treatment practitioners will have become better prepared as a result of the information provided and that they may be inspired to share their similar or perhaps even contradictory experiences with their peers.
DECLARATIONS
Authors’ contributions
The author contributed solely to the article.
Availability of data and materials
Unfortunately, some of these references were published pre-internet and may only be available in paper copy and do not possess DOIs or URLs; sharing scans of these paper documents electronically may violate copyrights. Many of the references cited have been uploaded to https://www.researchgate.net/profile/James-Gusek.
Financial support and sponsorship
None.
Conflicts of interest
The author declared that there are no conflicts of interest.
Ethical approval and consent to participate
Not applicable.
Consent for publication
Not applicable.
Copyright
© The Author(s) 2024.
REFERENCES
1. Kleinmann B, Skousen J, Wildeman T, Hedin B, Nairn B, Gusek J. The early development of passive treatment systems for mining-influenced water: a North American perspective. Mine Water Environ 2021;40:818-30.
2. Interstate Technology Regulatory Council. Biochemical reactors for treating mining influenced water. 2013. Available from: https://projects.itrcweb.org/bcr-1/. [Last accessed on 24 Jan 2024].
3. Gusek J, Wildeman T. Why do some passive treatment systems fail while others work? [Presented at the 2002 Annual Meeting of the Society of Mining, Metallurgy, and Exploration (SME) in Salt Lake City, Utah, USA. 2002]. Available from: https://bc-mlard.ca/files/presentations/2002-20-GUSEK-WILDERMAN-why-some-passive-systems-fail.pdf. [Last accessed on 24 Jan 2024].
4. Gusek J. Sulfate-reducing bioreactor design and operating issues: is this the passive treatment technology for your mine drainage? (Presented at the National Association of Abandoned Mine Land Programs, Park City, UT. September 15-18, 2002). Available from: https://www.researchgate.net/publication/228505464_Sulfate-reducing_bioreactor_design_and_operating_issues_is_this_the_passive_treatment_technology_for_your_mine_drainage. [Last accessed on 24 Jan 2024].
5. Tebo BM, Bargar JR, Clement BG, et al. Biogenic manganese oxides: properties and mechanisms of formation. Annu Rev Earth Planet Sci 2004;32:287-328.
6. Gusek J, Josselyn L, Wolaver E. Advancements in iron terrace design for metal mine sites. (Presented at the 2017 National Meeting of the American Society of Mining and Reclamation, What’s Next for Reclamation? 2017). Available from: https://wvmdtaskforce.files.wordpress.com/2017/05/2017-gusek-tues-930-salon-e.pdf. [Last accessed on 24 Jan 2024].
7. Reisman DJ, Gusek JJ, Bishop M. A pre-treatability study to provide data for construction of a demonstration bioreactor. In: International Conference on tailings and mine waste; 2003 Oct 12-15; Vail, CO, USA. 2003. pp. 304-15. Available from: https://pascal-francis.inist.fr/vibad/index.php?action=getRecordDetail&idt=15901263. [Last accessed on 29 Jan 2024]
8. Wildeman T, Brodie G, Gusek J. Wetland design for mining operations. Bi-Tech; 1993. Available from: https://www.amazon.com/Wetland-Design-Mining-Operations-Wildeman/dp/0921095279. [Last accessed on 24 Jan 2024].
9. Anaerobic compost constructed wetlands system (CWS) technology. 2002. Available from: https://www.loc.gov/item/2003373406. [Last accessed on 24 Jan 2024].
10. Arthur AW. Vertical flow systems - effects of time and acidity relations. 2004. Available from: https://www.asrs.us/Publications/Conference-Proceedings/2004/1595-Rose.pdf. [Last accessed on 24 Jan 2024]
11. Wildeman T, Cevaal J, Whiting K, Gusek J, Scheuering J. Laboratory and pilot-scale studies on the treatment of acid rock drainage at a closed gold-mining operation in California. 1994. Available from: https://www.asrs.us/Publications/Conference-Proceedings/1994-Volume-2/0379-Wilderman.pdf. [Last accessed on 24 Jan 2024]
12. Gusek JJ. Three case histories of passive treatment of metal mine drainage. 1998. Available from: https://wvmdtaskforce.files.wordpress.com/2016/01/98-gusek.pdf. [Last accessed on 24 Jan 2024].
13. Gusek J, Wildeman T, Miller A, Fricke J. The challenges of designing, permitting and building a 1,200 GPM passive bioreactor for metal mine drainage, West Fork Mine, Missouri. 1998. Available from: https://www.asrs.us/Publications/Conference-Proceedings/1998/0203-Gusek.pdf. [Last accessed on 24 Jan 2024]
14. Gusek J, Wildeman T, Mann C, Murphy D. Operational results of a 1,200-gpm passive bioreactor for metal mine drainage, West Fork, Missouri. 2000.
15. Cobbett CS. Phytochelatins and their roles in heavy metal detoxification. Plant Physiol 2000;123:825-32.
16. Reisinger RW, Gusek J. Mitigation of water contamination at the historic Ferris-Haggarty Mine, Wyoming. Available from: https://www.researchgate.net/publication/294362754_Mitigation_of_water_contamination_at_the_historic_Ferris-Haggarty_Mine_Wyoming. [Last accessed on 24 Jan 2024].
17. Thomas RC, Romanek CS. Passive treatment of low-pH, ferric iron-dominated acid rock drainage in a vertical flow wetland II: metal removal. 2002. Available from: https://www.asrs.us/Publications/Conference-Proceedings/2002/0752-Thomas.pdf. [Last accessed on 24 Jan 2024]
18. Eppley RL. Sulfate reducing bioreactor treating acidic coal mine influenced water (MIW) in Western Pennsylvania. 2010. Available from: https://www.onemine.org/documents/sulfate-reducing-bioreactor-treating-acidic-coal-mine-influenced-water-miw-in-western-pennsylvania. [Last accessed on 29 Jan 2024]
19. Gusek JJ. Design challenges for large scale sulfate reducing bioreactors. In: Calabrese EJ, Kostecki PT, Dragun J, editors. Contaminated soils, sediments and water. Boston, MA, USA: Springer; 2005.
20. Gusek JJ. Bench and pilot scale test results passive treatment of acid mine drainage (AMD) at the Fran Coal Mine, PA. 2004. Available from: https://gsa.confex.com/gsa/2004AM/webprogram/Paper79792.html. [Last accessed on 24 Jan 2024].
21. Gusek J, Wildeman TR. Passive treatment of aluminum-bearing acid rock drainage. 2002. Available from: https://www.yumpu.com/en/document/read/51930757/passive-treatment-of-aluminum-bearing-acid-rock-drainage. [Last accessed on 24 Jan 2024].
22. Pennsylvania Department of Environmental Protection, Office of Active and Abandoned Mine Operations. Fran Contracting Camp Run No. 2 Acid Mine Drainage Abatement Project Contract No. AMD 18(0817)103.1. Available from: https://files.dep.state.pa.us/Mining/Abandoned%20Mine%20Reclamation/AbandonedMinePortalFiles/Accomplishments/AMD18_0817_103.1_Fran_Contracting_Camp_Run_No._2.pdf. [Last accessed on 24 Jan 2024].
23. Reisman D, Gusek J, Torgerson D, Bishop M. Case studies demonstration sulfate reducing bioreactors at the Luttrell Repository & Peerless Jennie King Mine 10-Mile Superfund Site, Montana. In: EPA Hardrock Conference in Phoenix AZ in 2006.
24. Gusek J, Shipley B, Lindsay D. Overcoming access issues at a remote passive treatment site near Lake Shasta, CA. 2005. Available from: https://www.asrs.us/Publications/Conference-Proceedings/2005/0443-Gusek.pdf. [Last accessed on 24 Jan 2024]
25. Gusek J, Kelsey J, Schipper R, Shipley B. Biochemical reactor construction and mine pool chemistry changes, Golinsky Mine, CA. 2011. Available from: https://www.researchgate.net/publication/300368895_BIOCHEMICAL_REACTOR_CONSTRUCTION_AND_MINE_POOL_CHEMISTRY_CHANGES_GOLINSKY_MINE_CALIFORNIA. [Last accessed on 24 Jan 2024].
26. Gusek JJ, Wildeman TR, Jaško V. Bench-scale passive treatment of heavy metals, Smolnik Mine, Slovakia. 2000. Available from: https://www.asrs.us/Publications/Conference-Proceedings/2000/0275-Gusek.pdf. [Last accessed on 24 Jan 2024]
Cite This Article

How to Cite
Gusek, J. J. 35 years of lessons learned designing, building, and monitoring passive treatment systems for mining influenced water (part 1). Miner. Miner. Mater. 2024, 3, 2. http://dx.doi.org/10.20517/mmm.2023.29
Download Citation
Export Citation File:
Type of Import
Tips on Downloading Citation
Citation Manager File Format
Type of Import
Direct Import: When the Direct Import option is selected (the default state), a dialogue box will give you the option to Save or Open the downloaded citation data. Choosing Open will either launch your citation manager or give you a choice of applications with which to use the metadata. The Save option saves the file locally for later use.
Indirect Import: When the Indirect Import option is selected, the metadata is displayed and may be copied and pasted as needed.
About This Article
Special Issue
Copyright
Data & Comments
Data
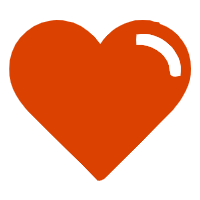
Comments
Comments must be written in English. Spam, offensive content, impersonation, and private information will not be permitted. If any comment is reported and identified as inappropriate content by OAE staff, the comment will be removed without notice. If you have any queries or need any help, please contact us at support@oaepublish.com.