Tungsten tailings issues and reprocessing solutions
Abstract
This paper aims to provide an overview of tungsten (W) tailings properties, detrimental impacts of these tailings, approaches to mitigate these impacts, and a presentation of methods to reprocess them to capture their economic value. Since W is widely used in a variety of industries, it has been extensively mined since the 19th century, and the mining continues to generate significant volumes of tailings. Recent data show that global W production stands at 84 kt per year, and more than 100 Mt of W tailings exist containing over 100 kt of WO3. The tailings contain variable amounts of valuable products and deleterious environmental substances. Some of the contained metals are in great demand for the energy transition. However, these tailings usually contain FeS2/Pyrrhotite and FeAsS minerals, which, when exposed to air and water, can produce acid mine drainage. As such, W tailings may pose environmental and human health risks. Globally, the reprocessing of W tailings presents a potential resource that can be regarded as a paradigm of sustainability and circular economy. Flotation, enhanced gravity separation, and wet high-intensity magnetic separation have been reported to be the common approaches to reprocessing W tailings. However, W processing presents particular difficulties owing to complex material properties, such as fine particle size, surface weathering, similarity in surface properties exhibited by gangue materials (fluorite, apatite, calcite), low concentrations of the elements of interest, and poor mineral liberation.
Keywords
INTRODUCTION
Following an increasing global population, digitalization, or technological evolution (industry 4.0 revolution, including the internet of things and artificial intelligence), industrialization, and growing demand from developing countries, pressure on resources is increasing[1-9]. Tungsten (W), a specialty metal with exceptional properties, is utilized in aerospace, construction, mining, automotive, military, ammunition, electronics, and many other industries. The latter properties make the replacement of W by other metals difficult for many industrial applications[10-12]. Concerns about W supply security have been discussed by different studies[13]. According to Graedel et al., a critical raw material (CRM) is defined as a material in which its supply shortage would result in a large negative economic impact[14]. The element is categorized as a CRM in the European Union (EU) and other countries, including the United States of America (US) and Japan. Tungsten has an economic importance (EI) of almost 9 out of a 10-index scale, which has increased from 8.1 in 2020[13,15]. Thus, W is a CRM due to its EI and the potential for supply disruption[16].
In 2021, the worldwide W production was around 83.8 kt; this figure changed by 0.24% in 2022 as the production reached 84 kt. The later production is expected to rise in 2023 due to the reopening of mines in the United Kingdom, South Korea, and Australia[1]. In 2022, the consumption of W in China by vehicle and other manufacturing industries was adversely affected by COVID-19 pandemic health measures such as lockdowns. In the US, a recovery of the aerospace industry and the expansion of oil and gas drilling led to an estimated increase in W consumption. Electric vehicle (EV) and smartphone industries are also interested in tungsten; for instance, the iPhone 12 is an Apple product that uses recycled tungsten[17]. Future growth in W consumption encompasses new industrial applications, such as the use of W in Li-ion batteries (LIBs) and tungsten powders for additive manufacturing.
China remains the largest tungsten producer and plays a pivotal role in the global W market[18-21]. It accounts for more than 84% of the world’s production (~71 kt)[1]. Various investigators predict worldwide tungsten consumption will increase with the reopening of the global economy and industrial production following the stagnation in 2020 as a result of the global COVID-19 pandemic[22]. In 2021, the prices of tungsten concentrates, downstream tungsten materials, and scrap shifted upward due to low inventory levels, reduced scrap availability, strong demand, and constrained spot supplies of ammonium paratungstate and concentrates[23]. The global market supply for tungsten is forecasted to reach a revised quantity of 113.2 kt by 2026, growing at a compound annual growth rate (CAGR) of 3.9% over the analysis period[24]. Technavio, a market search and advisory company, has predicted growth of 28.14 million tonnes per annum (MTPA) for 2022-2026, growing at a CAGR of 4.44% during the analysis or forecast period[25]. As per the 2022 USGS commodity report, the March 2022 monthly average price for ammonium paratungstate in the EU market was 3% higher than the one in February 2022 and 30% higher than that in March 2021. This W price spike could be linked to the rise in demand for tungsten and the post-pandemic outcome[23]. The future global demand for tungsten will be high as the innovation in technology develops and the fact that there is a growth of emerging economies, which involve manufacturing industries that depend on W[26,27]. Overall, there is a growing demand for tungsten and a dependence on China, which has raised concerns among academia, industries, and governments[2,21].
As tungsten mine tailings have extended storage requirements, they may have substantial environmental footprints affecting nearby rivers, the environment, and ground waters. Exposure of these tailings to both oxygen and water facilitates the oxidative dissolution of the contained sulfide minerals (arsenopyrite, pyrrhotite, and pyrite), generating acidic wastewaters that may contain appreciable concentrations of dissolved metals and sulfate[27]. Acid mine drainage (AMD) is a widely reported polluting mechanism through drainage of low-pH effluents with potentially high concentrations of hazardous and toxic elements[28].
W tailings from mining sites must be appropriately managed or treated in order to ensure the extended stability of disposal facilities and to prevent or mitigate any water and soil pollution arising from AMD and leaching of metals[29]. Unlike other mine tailings, studies on the possible utilization of W tailings at an industrial level are scarce. Recent work shows that W tailings could be used as raw materials by vitrification technology[30]. Provided that the major components of W tailings are SiO2 and Al, tailings could be used in glass-ceramics, cement (as a raw material for cement clinker), bioceramics, and mineral polymeric materials[31]. Castro-Gomes et al. highlighted the reuse of W mining waste rock in technical-related artistic value-added products[32]. Other researchers have found that modified W tailings could be used as fillers in the manufacture of high polymer rubber[33]. Figure 1 illustrates the possible utilization of mine tailings as raw materials in different industrial sectors. Industrial sectors where W tailings could be used are also included.
Enhanced physicochemical and separation processes are required to ensure that the tailings can be considered in a circular economy, securing critical or valuable metals through reprocessing while reducing the deleterious impacts on the environment[35]. Efforts to find feasible reprocessing methods include the REMinE project (Improve Resource Efficiency and Minimize Environmental Footprint). This is an ERA-Min project which involved Lulea University of Technology (Sweden), University of Porto (Portugal), and the Research and Development National Institute for Metals & Radioactive Resources (INCDMRR) in Romania and three mines as case studies, Sasca Romania (Cu, Au, Ag), Panasqueira in Portugal (W), and Yxsjöberg in Sweden (W, Cu, and Fluorite). The study investigated the feasibility of reprocessing historical tailings to recover valuable parts and produce compatible wastes in the nearby environment. The results that included historical W tailings are described in the reprocessing part[29]. It could also pave the way to a self-sufficiency strategy to reduce dependency on lead-producing countries[36,37]. Reprocessing of tailings aligns with the United Nations Sustainable Development Goals (SDGs) of 2022[38]. Increasingly strict environmental standards and legal measures with respect to the disposal of tailings are ensuring that mining companies control tailings meticulously.
In this review paper, we will mainly focus on W tailings and their management. The structure of our paper consists of an overview of W tailings, properties of W tailings, detrimental impacts of W tailings, approaches to mitigate these impacts, and reprocessing methods. Concomitant information was collected from the literature, including scientific reports, journal manuscripts, books, and patents. A literature search was performed through several sources, including the Web of Science, an online database of peer-reviewed scientific publications, the Theses Fr website, conference proceedings, textbooks, the USGS website, and the EU Commission’s website. The keywords used in the bibliographic search included “tungsten tailings”, “W tailings recycling techniques”, “W tailings reprocessing”, “management of W tailings”, “W recovery from tailings”, and “detrimental/adverse effects of W tailings”. An emphasis was placed on searching for reprocessing tungsten tailings approaches and challenges thereof.
GLOBAL TUNGSTEN TAILINGS OVERVIEW
As per the 2022 report of USGS, tungsten resources are widely distributed globally in both active and abandoned tungsten mines. W resources have been identified on all continents except Antarctica[1]. Most of the non-producing tungsten mines ceased their operations concurrent with the fall of tungsten prices[39,40]. Processing technology has advanced since the construction of many of the mills, and reprocessing might extract more value from the older tailings[10,11,13]. Cantung, Salau, and Yxsjöberg mines are examples of the mines that ceased operations due to low commodity prices and have abundant tailings containing relatively elevated tungsten values[13,39,41].
The rise in tungsten demand, particularly in the manufacturing industries (e.g., EV industry), may pave the way for various companies to consider W tailings as a source. As per the study of Suárez Sánchez et al., there were five W mining projects prepared for production, including two from Europe, namely the Barruecopardo open-cut project in Spain and Hemerdon open-cut project in the UK (Wolf Minerals)[42]. Other mines were the Kilba-open cut project in Australia, the Sangdong Underground project in South Korea, and the King Island Scheelite project in Tasmania. The number of prospective projects directly indicates the quantity of W tailings that can be expected in the future. As tailing accumulation is an ongoing process, recycling or reprocessing is required for an operation to align with the SDGs.
Based on the available data from the literature, for every one ton of produced tungsten concentrate, around 7-10 tons of W tailings are produced, depending mainly on the tungsten ore grade and the dressing/beneficiation level[43]. Studies have demonstrated that the remaining tungsten minerals in tailings are in fine or very fine fractions, which are challenging for conventional beneficiation methods. This issue is linked to the brittle mineral character of minerals such as scheelite (CaWO4), which generates fine and ultrafine particles during the size reduction (crushing or grinding) process. CaWO4 and Wolframite [(Fe, Mn)WO4] are primary tungsten minerals of EI. The remaining tungsten-bearing minerals are mostly present in trace amounts[12,20,44]. Secondary tungsten minerals include ferritungstite [(W, Fe)(O, OH)3], elsmoreite
CaWO4 is the most abundant tungsten mineral and is present in approximately 0.67-fold of known W deposits, but (Fe, Mn) WO4 is also commonly mined[12]. Individual deposits may have quite diverse origins. Scheelite mineralization is predominantly associated with skarnization, whilst wolframite mostly occurs in veins[12]. Generally, the beneficiation approach for scheelite and wolframite ores consists of pre-concentration after crushing and grinding, followed by roughing, cleaning, and final purification stages to produce a concentrate with 65%-75% WO3 to meet international trading requirements[47,48]. Scheelite is diamagnetic, and therefore, magnetic separation is usually unsuitable for scheelite ores, which also generally display finer liberation sizes[20,49]. Scheelite is readily amenable to gravity concentration since it is very dense (specific gravity of around 6.1), but it is also amenable to flotation. Wolframite, in contrast to scheelite, is paramagnetic. Thus, beneficiation techniques of gravity concentration and flotation are applied to scheelite ore, and gravity and/or magnetic separation are applied to wolframite ore. Both primary tungsten minerals are brittle; therefore, the comminution stage must be carefully designed to avoid overgrinding (i.e., to minimize the formation of fines and subsequent loss of W). It is recommended to use appropriate size classification techniques at each comminution stage. Screens and hydrocyclones are generally used for hydro-classification purposes[20]. Overall, tungsten ores are processed via a combination of grinding, flotation, gravity separation, magnetic separation, and electrostatic separation[20,47,48]. Other challenges include the association of scheelite with calcium-bearing minerals that have similar properties and impede the flotation chemistry of CaWO4[10].
Overall, research data about W tailings are scarce[13]. However, some available statistics on W tailings on a global scale show that more than 100 Mt of known W tailings containing above 100 kt of WO3 exist [Table 1].
Some global repositories for W tailings and known examples of their reuse
W tailings deposit | Amount (Mt) | Category | Grade (WO3), % | WO3 (kt) | Familiar reuse examples | Ref. |
Bom-Gorhon (Russia) | 0.1 | - | 0.225 | 0.23 | - | [50] |
La Parrila (Spain) | 1.13 | - | 0.28 | 3.16 | Tailing reprocessing plans | [51] |
Mount Carbine (Australia) | 2 | Sn-W | 0.1 | 2.00 | Reprocessing for W recovery | [52] |
Cantung (Canada) | 3.9 | W-Cu | 0.32 | 12.48 | Reprocessing plan for W recovery | [53] |
Yxsjöberg (Sweden) | 5.2 | W-Cu-F | 0.08 | 4.16 | Nil | [54,55] |
Panasqueira (Portugal) | 8 | W-Sn | 0.12 | 9.60 | Reprocessing trials for W recovery | [36,56-58] |
Sangdong (South Korea) | 12 | W-Mo | 0.1 | 12 | Feasibility study for reuse in cement production | [59] |
Kaitashskoe (Uzbekistan) | 12 | W-Mo | - | - | Flotation tailings reuse trials for ceramic tile production | [60] |
Luanchuan (China) | 20 | W-Mo | 0.14 | 28 | Reprocessing for W recovery | [57] |
Dzhidinsky (Russia) Salau (France) | 40 0.25 | Mo-W - | 0.1 0.19 | 40 0.48 | Nil - | [61] [39] |
Aggregate | 104.58 | 112.11 |
Evidently, from the figures [Table 1], taking Dzhidinsky (Russia) as an example, the implementation of a circular economy with acceptable reprocessing practices will be needed to encourage the extraction of economic value while simultaneously achieving eco-friendly environmental goals. The most convenient way of dealing with tailings is to capitalize on reprocessing to recover valuable parts and determine if reprocessing tailings can be used as a raw material in the construction industry, such as aggregates and railway ballast or as a backfill material in the mining industry[7,34].
TUNGSTEN TAILINGS PROPERTIES
Ore geology and W tailings properties
W tailings are the residual products of W mining activities from both open pit and underground mining approaches. There are two main types of W tailings reflecting the two geological sources of W deposits, which together account for 76% of W deposits, namely skarn (41%) and stockwork or vein deposits (35%), each requiring a distinct processing route[16]. Tungsten deposits are almost exclusively associated with granitoid rock intrusions[12]. The main settings are wolframite quartz veins and stockworks, associated with highly evolved leucogranites and spatially associated with cassiterite, or scheelite skarns, formed by contact metamorphism of a granite in a limestone bedrock, in association with molybdenum porphyries, or as scheelite stratiform-type deposits (regional metamorphism). Tungsten may also be a by-product of molybdenum porphyries[40,62]. Table 2 presents the global geological distribution of major tungsten deposit classes, size, and grades of major tungsten deposit types, respectively, and each will have associated tailings even if they are not described in the literature[10,12,63].
Global geological distribution of major tungsten deposit classes with their size and grades
Deposit type | Deposit size, t | Exploited mineral | Associated economic metals | Typical grade, WO3% | Estimated W content, 103 tonnes | % of total | Some mines (Active & Abandoned) |
Skarn | < 104-5 × 107 | Scheelite | Cu, Zn, Mo, and Bi | 0.3-1.4 | 1,764 | 41 | Vostok-2, Salau, Cantung, Sang Dong |
Vein/stockwork/Breccia | < 105-108 | Wolframite | Mo, Sn, Cu, Au, and Bi | Variable | 1,475 | 35 | Panasqueira, Negra, Chollja |
Porphyry | < 107-108 | Wolframite or/and scheelite | Sn, Bi, and Mo | 0.1-0.4 | 679 | 16 | Climax, Yangchuling, Xingluokeng |
Disseminated | < 107-108 | Scheelite and Wolframite | Sn, Mo, and Bi | 0.1-0.5 | 217 | 5 | Shizhuyuan, Lultin |
Stratabound | < 106-107 | Scheelite | Mo | 0.2-1.0 | 118 | 3 | Mount Mulgine, Mittersill |
Total | 4,253 | 100 |
W tailings are the repository of the crushed rock and the water or chemicals used during beneficiation. These tailings differ in their physical and chemical properties depending on the nature of the ore being processed. These properties include geochemical and mineralogical composition, specific gravity of tailings, permeability, viscosity, strength properties, settling behavior, leaching behavior, and pore water chemistry. The properties can be affected by the addition of reagents in mineral processing, the overall process of beneficiation, and subsequent disposal, including surface weathering. Generally, tailings are heterogeneous owing to different mineralogical compositions being processed and separation processes during the tailings discharge and deposition and alteration processes following exposure to the atmosphere[64,65].
Acosta et al. demonstrated the spatial heterogeneity of various factors, including total nitrogen, pH, organic carbon, electrical conductivity, cation exchange capacity, silt, clay, sand, and total and extractable Pb, Cd, Cu, and Zn on a tailing surface[66]. The differences could be linked to various factors, including the type of process streams, the composition of the primary ore, the mechanism of deposition (systematically or randomly), and the extent of weathering that could have occurred since their deposition[29].
The study of Kazamel et al. points out that the geochemical behavior of tungsten is not well studied, hindering the prediction of W leaching from ongoing and former mining operations[41]. Limited studies have examined the behavior of W in mine wastes[67]. Kazamel et al. concluded that the mobility of tungsten at low temperatures is primarily controlled by pH and its adsorption behavior[41].
An examination of the factors controlling tungsten mobility in W-Cu skarn tailings has been done by Kazamel et al.[68]. They point out that tungsten is transported as a dissolved species but is also adsorbed to suspended Fe-oxyhydroxide minerals under neutral pH conditions. Their research explains the limited solubility of CaWO4 in neutral or acidic mine waters, and the mobility of the small amount of W released is governed by the presence of Fe-oxyhydroxide minerals, the stability of the depositional environment, and the transport of colloidal material in surface water. Few studies have addressed the mobility of W in mine waste[67].
W tailings may contain the following mineral phases, depending mainly on the type of primary processed ore and the degree of oxidation or reduction as a result of weathering. The mineral phases may include scheelite, wolframite, quartz, plagioclase, micas, chlorite-serpentinite, kaolinite, amphibole, calcite, dolomite, pyrrhotite, chalcopyrite, gypsum, pyrite, arsenopyrite, siderite, goethite, magnetite, jarosite, hubnerite, molybdenite, galena, fluorite, etc. The chemical formulas of the mentioned mineral phases are presented in Table 3[2,11,29,35,55]. Out of the mentioned mineral phases, the sulfide-bearing mineral phases are linked to the AMD[69]. The sulfide mineral phases include pyrite, arsenopyrite, and pyrrhotite.
Rough composition of tungsten tailings
Mineral | Chemical formula |
Scheelite | CaWO4 |
Wolframite | (Fe, Mn)WO4 |
Quartz | SiO2 |
Plagioclase | Na(K)AlSi3O8·CaAl2Si2O8 |
Micas | (K, H3O)·(Al, Mg, Fe)2·(Si, Al)4O10[(OH)2, (H2O)] |
Chlorite-serpentinite | (Fe, Mg, Al)6·(Si, Al)4·O10·(OH)8 |
Amphibole | Ca2[Mg4(Al, Fe)]Si7AlO22(OH)2 |
Calcite | CaCO3 |
Dolomite | CaMg·(CO3)2 |
Pyrrhotite | Fe1-xS (x < 0.2) |
Chalcopyrite | CuFeS2 |
Gypsum | CaSO4·2H2O |
Pyrite | FeS2 |
Arsenopyrite | FeAsS |
Siderite | FeCO3 |
Goethite | FeOOH |
Magnetite | Fe3O4 |
Jarosite | KFe3(SO4)2(OH)6 |
Hubnerite | MnWO4 |
Molybdenite | MoS2 |
Fluorite | CaF2 |
Galena | PbS |
Kaolinite | Al2O3·(SiO2)2·(H2O)2 |
Much of the available literature on tungsten minerals is focused on W processing, with little attention on the mobility and weathering of W in tailings. More data about the geochemical behavior of tungsten mobility in historical tailings is needed since its geochemical mobility is still not fully understood[70]. Not only is there a need to better understand tungsten, but the interrelation with other elements is also lacking. Most W tailings are rich in arsenopyrite, and thus, arsenic mobility should also be considered[68,71].
Arsenic is stable under reducing conditions; hence, tungsten mine tailings with arsenopyrite might remain chemically stable provided they are kept water-saturated. The oxidation of arsenopyrite by both Fe3+ and oxygen is easily promoted by microorganisms, such as acidophilic-Fe and S-oxidizing bacteria[72]. Scorodite (FeAsO4·2H2O), Pharmacosiderite [KFe4(AsO4)3(OH)4·6-7H2O], amorphous ferric arsenate (AFA), arsenolite (As2O3), and arseniosiderite [Ca2Fe3(AsO4)3O2·3H2O] are common products of arsenopyrite weathering, with scorodite being the most abundant[71,73,74]. The formation of scorodite is presented in Equation 1, and its dissolution is presented in Equations 2 and 3. A research study conducted by Murciego et al. on the determination of secondary phases formed under natural weathering of arsenopyrite from abandoned tungsten and tin exploitations in Spain (25 samples of weathered arsenopyrite) showed that AFA, scorodite, and pharmacosiderite with Fe/As molar ratios in the range of 1.2-2.5 were present as secondary arsenic products[71].
A review study conducted by Koutsospyros et al. showed that weathering of tungsten-rich rocks and soils is a reason for its mobility[75]. Incomplete dissolution of tungsten minerals, especially scheelite, releases some W to mine drainage[55,69,75]. The weathering process is supposed to be due to anion exchange with carbonate ions on the scheelite surfaces in unoxidized conditions where pH is above 7. The weathering process of scheelite in the tailings is presented in Equations 4 and 5.
DETRIMENTAL IMPACTS AND ECONOMIC VALUE OF W TAILINGS
Detrimental impacts of W tailings
Social and health impacts
Since a number of W mining companies have been in operation since the 19th century, the generated tailings from the mining sites or processing factories may have an immense impact on human beings and biota. To ensure a sustainable society, responsible production, consumption, and recycling are essential. W was treated as an immobile element in the environment and considered to be a suitable substitute for Pb and U for the manufacture of “green bullets” in military and munition applications. Studies have suggested that detection of W in soil and potable water sources may represent mobility, which could result in the risk of human exposure. The pH and other factors, such as the type of minerals present, may also contribute to the solubility in soils[76]. Occupational tungsten exposure can also occur through inhalation of dust and during the preparation of tungsten carbide products that are significantly employed in making mining and cutting tools[77]. Population can be exposed via inhalation of W dust in the contaminated water or air. Water contamination comes from dissolved rocks or via effluent from mining sites and hard metal industry waste.
Several studies have been conducted to determine the extent of the impacts of W tailings on human health. Reutova et al. investigated a 170-hectare tailing pond of the Tyrnyauz tungsten-molybdenum mining, which contains over 113.4 million tonnes of waste with W, As, Mo, and other metals[78]. The factory has been in operation for more than six decades. The investigators examined the content of metal content in drinking water and in the hair of children. They also identified cytotoxic and genotoxic effects in children residing near the tailing ponds, which gave rise to an elevation of the number of children affiliated with the medium and high-risk groups. They concluded that despite the broad applications of tungsten in manufacturing and other industries, the tailings may pose some potential social effects.
Another concrete example is that in the early 2000s, discussions about the toxicity of tungsten arose. This was noted when groups of childhood leukemia cases in the western part of the US were provisionally linked to elevated concentrations of W in groundwater that was in a range from 0.27 to 742 µg·L-1[79] and drinking water that was in a range from 0.25 to 337 µg·L-1[75]. The level of W was elevated but not causally related; i.e., there was a relationship, but it was not a direct causation.
Overall, scientific research has focused on the toxicity of tungsten metal dust as a result of anthropogenic activities[80-83]. Few studies investigate the toxicity of W tailings.
Environmental impacts
Environmental impacts related to W mining may include contamination, dam failure, AMD landslides, land occupation, water pollution, and dust lifting.
Arsenic (As), Copper (Cu), and Cadmium (Cd) are regarded as the main contaminants of serious concern in wolframite tailings[84]. Reports about the Panasqueira wolframite mine in Portugal show that AMD mobilized and released Cu, As, and Cd into the nearby ecosystem[84]. Skarn tailings can present various elements of potential concern, such as Be, Bi, Cu, W, and Zn, together with Fe-sulfides and carbonates[62]. The contained sulfides can promote the metal mobility in the tailings through the sulfide oxidation, reducing the pH[85], which may be buffered by available carbonates that can neutralize mine drainage to the extent that there is sufficient carbonate quantity to immobilize the metals.
Mining tailings of tungsten need vigilant management and handling practices to warrant long-term stability of disposal and storage facilities and prevent pollution to the surrounding (air, soil, and water). Poor management of wastes or tailings resulting from mining operations antagonizes the general public, local communities, and NGOs and contributes to a pessimistic public attitude.
Some W-producing countries are enacting stringent measures for environmental protection; a concrete example is that China initiated an environmental tax of $140 in 2016 per ton of residues. Moreover, they closed many smelters and APT plants due to environmental pollution despite the ongoing high global consumption. Research studies in the treatment of W tailings are strategic necessities to fulfill the sustainable development of the tungsten industry[86].
Economic value of W tailings
Since W tailings contain valuable elements in appreciable concentrations, there is a need to unlock the economic value contained in the tailings, including gold and silver. W tailings often contain notable quantities of potentially valuable metals, specifically in historical operations, where processing technologies employed to beneficiate target minerals were not as efficient as those presently available[11,13]. However, in some cases, treatment and valorization of the tailings could also be combined with an efficient ground depollution, and such tailings could be upgraded as secondary mines of strategic metals, with easier access to the ore compared to primary mines and lower energy consumption since already milled.
REMEDIATION AND REPROCESSING FOR MITIGATING DETRIMENTAL IMPACTS AND HARNESSING ITS ECONOMIC VALUE
Phytoremediation
Generally, phytoremediation involves the use of green plants to clean up, sequester, or extract pollutants from soil and groundwater. The development of a vegetal cover in the mine waste deposits and mining areas is known as phytocap or phytocapping. The most salient characteristics that plants suitable for phytoremediation must have include the ability to adopt metals in large-scale shoots and the ability to swiftly create large biomass[87]. The primary or first step is the effective growing of species of plants while ensuring the sensitivity of plants to the behavior of mine tailings, climatic conditions, and topography[88,89]. It is a socially accepted technology to remediate polluted soils, effective, inexpensive, non-intrusive, and aesthetically pleasing[90-92]. As per Lamb et al., phytocaps prevent infiltration of rainwater and, therefore, do not allow leaching of metals[88]. Phytocapping reduces the penetration of rainwater by the following mechanisms: canopy interception of rainfall, evapotranspiration of interstitial water, and storage of moisture in the soil layers. Phytoremediation is solar-powered; hence, as far as operating costs are concerned, it is an economical & environmentally friendly route for remediation. The treatment of soils by phytoremediation involves the interaction of microflora in the rhizosphere (the layer of soil occupied by the roots), contaminants, and roots[93-96].
Despite the fact that the phytoremediation route is categorized as environmentally friendly, it has some drawbacks or limitations, as presented in Table 4[94,97,98].
Advantages and limitations of phytoremediation
Advantages | Drawbacks/Limitations |
In situ and passive technique | Limited to shallow soils or where contamination is localized to the surface (< 5 m) |
Highly accepted by the public | Metal concentrations in the soil can be lethal and toxic to plants |
Reduction in the dispersal of dust and contaminants by wind | Slower treatment than the traditional physicochemical techniques |
Low cost (uses solar energy, i.e., photosynthesis) | Still under development and, therefore, not accepted by many regulatory agencies |
Provides shelter/habitat for animal life | Mostly, plants are selective in metal remediation |
Reduction of leaching and mobilization of contaminants in soil | Efficient phytoremediation plants may not adapt to climatic and environmental conditions at contaminated sites |
Reduction of surface runoff | Contamination may spread through the food chain if accumulator plants are ingested by animals |
The harvested biomass can be economically valuable (may result in the formation of biogas and biochar) | The area to be decontaminated must be large enough to allow application of cultivation techniques |
Plant processes are more easily controlled than those of microorganisms | Toxicity and bioavailability of degradation products remain largely unknown |
Has reduced environmental impact and contributes to the landscape improvement | There is little knowledge of farming, genetics, reproduction, and diseases of phytoremediation plants |
Harvesting of the plants or organs that have accumulated metals is easy to accomplish with existing technology | If the plants release compounds to increase the mobility of the metals, these can be leached into groundwater |
Limited studies have been conducted to investigate the phytoremediation of soils affected by the W tailings. In the study conducted by Favas et al., investigating the phytoremediation of soils contaminated with metals and metalloids at Sarzedas and Vale das Gatas mining areas, it was identified that some tungsten hyperaccumulating plants were present[99]. The areas examined included abandoned Sn/W mines (Tarouca mine, Sarzedas mine, Fragas do Cavalo mine, Adoria mine, Vale das Gatas mine, Ervedosa mine, Regoufe mine, and Rio de Frades mine). Tungsten-related results obtained from Sarzedas (Central Portugal) and Vale das Gatas mines (Northern Portugal) are presented in Table 5[99]. They found that elevated levels of sulfides, especially arsenopyrite and pyrite, favored the dissolution of deleterious elements, allowing elevated bioavailability and dispersion.
Accumulation of W in different plant species of the Saredas and Vale das Gatas mine areas
Plant species with W @ Sarzedas mine area | Plant species with W @ Vale das Gatas mine area |
Digitalis purpurea subsp. purpurea | Pteridium aquilinum |
Cistus ladanifer subsp. ladanifer | Agrostis Castellana |
Pinus pinaster | Holcus lanatus |
Calluna vulgaris | Pinus pinaster |
Helichrysum | Juncus effusus |
Chamaespartinum tridentatum | |
Quercus ilex subsp. ballota | |
Erica umbellata | |
Eucalyptus globulus | |
Quercus suber | |
Genista triacanthos | |
Agrostis curtisii | |
Lavandula stoechas subsp. Stoechas | |
Halimium ocymoides |
Reprocessing W tailings
Reprocessing of abandoned W tailings can offer value for both the neighboring communities and the mining company as valuable minerals are recovered from legacy tailing storage facilities, and the reprocessed tailings are placed in engineered and modern facilities amenable to today’s environmental regulations.
In addition to tungsten, tailings may contain valuable metals, such as copper, gold, silver, molybdenum, bismuth, cobalt, and others, depending on the composition of the deposit, the metal of interest that was extracted, and the processing route that was employed. Details about the tailings’ material and even the deposits are frequently limited[11,13,27]. For the case of tungsten, the type of extraction technology employed during historical operations can also contribute to concentrations in old W tailings. Technologies for the metal recovery from W mining tailings include but are not limited to gravity separation, magnetic separation, leaching, bioleaching, and flotation[100,101]. The amount/content of metals and or minerals present in tailings depends mainly on the processing efficiencies and separation and extraction methods employed. According to a study done by Petruk[102] and Tungpalan et al.[103], in the selection of processing methods, the following mineralogical characteristics of ores must be considered as they have a link to the metallurgical performance of process flowsheets to be developed[104,105]: (i) Compositions of minerals that bear on the process; (ii) Identification of major, minor, and trace minerals; (iii) surface coatings on minerals; (iv) Quantities of minerals; (v) Mineral liberations; (vi) Particle and grain size distributions and textures of the minerals. Normally, tungsten recovery rates from ores commonly range between 60% and 90%[47]; i.e., about 0.1-0.4-fold of tungsten content of the ore is lost in the beneficiation.
Flotation is a physicochemical separation route for minerals. It utilizes surface properties to separate gangue minerals from valuable minerals[101]. Collectors, depressants, selective flocculants, and dispersants are reagents usually employed during flotation tests. Solution chemistry, mineral surface wettability, surface electrical properties, and surface lattice ion dissolution are primary controlling factors for flotation of tungsten minerals, particularly scheelite[49]. However, scheelite’s flotation presents challenges due to the similarity of surface properties exhibited by gangue minerals to those of scheelite[10,16,21]. The gangue section of scheelite ore includes calcium silicates and calcium semi-soluble salts: fluorite (CaF2), apatite
Magnetic separation is based on the use of magnetic forces to concentrate the particles that are susceptible to magnetism. It is commonly employed in wolframite beneficiation in a high-intensity magnetic separation system. In the magnetic separation process, the particle size of wolframite plays an important role, similar to gravity separation. The effect of magnetic forces acting on wolframite particles drops sharply with the decrease in wolframite size. This, unfortunately, results in an accumulation of the fine particle fraction of wolframite that is sent to tailings. A magnetic separation process is a semi-continuous procedure that involves low energy consumption; however, it offers low enrichment ratios[6].
Gravity separation is an approach that has been in application for more than 2,000 years[106]. A variety of minerals can be treated by gravity concentration, including scheelite and wolframite. The method separates different minerals and metals based on their specific gravity and relative motion under drag and gravity forces. The minerals commonly contained in tungsten skarns present a broad range of specific gravities (SGs): 3.2 for fluorite and apatite, 2.7 for calcite, and 6.1 for scheelite while silicates have SGs between 2.6 and 3.7 based on the geological context. Thus, it allows the use of enhanced gravity separation approaches to remove the minerals that are troublesome in flotation with fatty acids, mainly the calcium-bearing semi-soluble salts (fluorite, calcite, and apatite)[6]. The specific gravity of the particle, shape, weight, and size are important factors that determine gravity separation efficiency[10,106].
Since gravity concentration has been in applications for centuries in mineral processing, a large range of equipment is available. However, they do not share the same costs, throughputs, or performance measures, nor do they work with the same particle size[10,107]. Due to extended settling times, a lot of the classical gravity separation devices (spirals, jigs, shaking tables, etc.) exhibit a remarkable decrease in the separation performances as the particle size decreases[107]. Instead, centrifuge instruments are more promising and appropriate for fine particles. They can also be employed for moderately coarse particles[106,108]. Table 6 presents the working particle size range (mm) of various gravity concentrators[10,106,109-111]. From the table, we find that W tailings, with their property of fine dissemination, may be appropriately concentrated by centrifuge devices (Knelson concentrator, Falcon concentrator, and Falcon ultrafine concentrator) as they have a broad particle size range (0.001-10 mm). In contrast to flotation and magnetic separation, gravity separation has some advantages, including its low operation and investment costs, less potential pollution to the environment, and high separation efficiency[110,111]. W tailings reprocessing can be difficult, depending on the abundances of garnet, topaz, iron oxide, and sulfides that may affect the gravity concentration step as they dilute the concentrate, presence of calcium-bearing salts that affect the scheelite flotation due to similarity in chemical/surface properties, and fragility making the comminution step problematic as slime production is greater.
Working particle size range of different gravity concentrators
Working particle size range (mm) | |||||||
Concentrating device | Category | 0.001 to 0.01 | 0.01 to 0.1 | 0.1 to 1 | 1 to 10 | > 10 | Remark |
Static | Density | * | * | ||||
Dynamic | * | * | * | ||||
Water only | * | * | * | ||||
Jigging | Stratification | * | * | * | * | ||
Sluice box | Flowing film | * | * | * | * | ||
Reichert cone | * | * | * | ||||
Pinched sluice | * | * | * | ||||
Spiral | * | * | * | ||||
Shaking table | Shaking | * | * | * | |||
Bartles-mozley | * | * | |||||
Crossbelt | * | * | |||||
Pneumatic jig | Air | * | * | * | |||
Air table | * | * | |||||
Centrifugal | Miscellaneous | * | * | ||||
Knelson concentrator | Centrifuge | * | * | * | * | Promising |
The comprehensive size range to which gravity concentration is employed is larger than with any other concentration process since it can separate particles as coarse as one meter. However, 500 µm is generally accepted as the largest size of material to be separated[108]. Practically, the lower cut-off size for fine gravity concentration is about 6 µm[106,108].
Khavari[29] studied the feasibility of tungsten reprocessing from historical Swedish W tailings obtained from Yxsjöberg (0.22% WO3). The main tungsten mineral was scheelite; however, other minerals included quartz, chalcopyrite, fluorspar, pyrrhotite, cassiterite, and magnetite. Given that scheelite was their mineral of interest, a Knelson-enhanced gravity separator was used for separation tests, as CaWO4 is a good candidate for specific gravity-based tests. Magnetic separation tests were done to remove pyrrhotite and magnetite to produce an environmentally stable tailing.
Das et al. studied the potential use of enhanced falcon gravity separators in recovering fine and ultrafine CaWO4 values available in the Hutti gold mine tailings in India[112]. Several authors have reported W values in the Hutti gold mine tailings, mainly in the form of CaWO4. The study aimed to investigate the parameters that could influence concentrator efficiency. Using feed material sampled from the site, tests, including segregation tests, separation tests, and saturation tests, were performed. ICP-OES tests demonstrated ~0.03% CaWO4 (~300 ppm) in the feed. The feed material had a d80 = 37 µm and a d50 = 18 µm and was found to be richer in quartz (44.7%) and Al2O3 by 14.3%. Overall, based on the X-ray Powder Diffraction (XRD) pattern of the feed sample, the major identified minerals were quartz and plagioclase feldspar (mainly albite). A low concentration of W in the feed material meant that XRD peaks of W minerals could not be detected. An optimization study using Central Composite Design (CDD) aimed at maximizing yield, recovery, and an enrichment ratio by variating feed rate (kg·min-1), bowl speed (Hz or G-force), and wash water rate (L·min-1) was completed. The CDD consisted of 20 experiments with three variables and six replicates. The model data showed that the wash water rate and bowl speed were of paramount significance. The order of significance of the main variables on the recovery of CaWO4 follows wash water rate > bowl speed > feed rate. They found that under optimum conditions such as a wash water rate of 4 L·min-1 and a rotational speed of 70 Hz with an actual feed rate of 1 kg·min-1, a recovery of ~68%, a yield of ~17% with a ~4.7% enrichment ratio was achieved demonstrating promise for tailings reprocessing.
Clemente et al. investigated the reprocessing of slime tailings from a tungsten mine[56]. They described the development of new process routes for ultrafine wolframite recovery to enhance the efficient reprocessing of historical mine tailings and plant slime tailings. In their sample, much of the wolframite was < 25 µm, associated with a complex mixture of Zn, Cu, tourmaline, and siderite, in addition to other sulfides, ferromagnetic materials, and other strongly magnetic materials. The test work consisted of magnetic separation, flotation, and gravity concentration conducted at both the pilot scale and in the laboratory on the slime tailings of the plant and on the historical mine tailings. The results showed that a three-stage gravity separation process with intermediate sulfide flotation could produce a wolframite concentrate of 50%-55% WO3, which is a reasonable recovery. Single froth flotation provided inconsistent results.
Rosario[35] applied the electrodialytic process for W recovery and arsenic removal from Panasqueira W mine tailings (Covilhã, Portugal) using cells with 2C and 3C compartments in the presence of anion and cation exchange membranes. The results showed that the 2C cell unit with an anion exchange membrane presented the highest tungsten recovery (0.15%) and Arsenic removal (23.51% ± 21.33%). Additional preliminary tests were carried out by adding three adjuvants to the sample. A recovery of 0.64% tungsten was achieved with the removal of 9.48% As when the adjuvant B was added. The lower recovery of tungsten compared to arsenic was due to the availability of sulfates in the sample, which promoted the formation of W complexes.
Figueiredo et al. investigated approaches to remine old W tailings from the Panasqueira Mine in Portugal[100]. With an aggregate of 33 demarcated points, samples were collected for this investigation. In their scheme, they targeted recovering Zn, obtaining Zn liquor, recovering W, and eliminating sulfides. Tests, including stirred-tank leaching, flotation, and pressure leaching, were employed. The objective of the stirred tank leaching was to extract Zn from the tailings into a soluble liquor. The one for flotation was to recover arsenic as a sulfide reduction stage for the material to be sent to the pressure leaching, while the objective of pressure leaching was to extract W from the tailings into a soluble liquor. Experimental data analysis and modeling were used to evaluate the response of experimental conditions in various experiments. Using a hydrometallurgical route to reprocess the tailings satisfied the two conditions of environmental risk and metal demand, and the project represented a profitable investment.
Biohydrometallurgy has been reported to be the best technology for mine waste reprocessing. Unlike W tailings, current/implemented operations are focused on the recovery of one or two metals. Some examples of industrial case studies around the world include the Kasese (former copper mine in Uganda), Production of Co from 2000 to 2014 and Vuonos (active talc mine in Finland), Production of nickel and cobalt from high-grade sulfide flotation tail[113]. Ye et al. showed a successful application of a biohydrometallurgy approach to remove heavy metals from lead-zinc mine tailings using bioleaching followed by sulfide precipitation[114]. With the use of bacteria in a bioleaching reactor, metals were dissolved from the tailings. More than 99% of the Zn and 75% of the Fe were precipitated using 25 g·L-1 Na2S in the bioleaching leachate. Extreme thermoacidophile Metallosphaera sedula was demonstrated to grow on and directly extract W from scheelite, a potential new approach for recovering W from tailings[115]. One bioleaching experiment on W tailings was to remove Mn and As from W tailings. Using mixed cultures of Acidithiobacillus thiooxidans and Acidithiobacillus ferrooxidans, recovery of Mn could almost reach 100% while the one for As could reach about 97%[116]. Other researchers have shown that wolframite tailings could be processed by acidophilic bioleaching[69].
Thus, this literature search confirms that studies on W tailings reprocessing by mineral processing approaches could address bottlenecks in traditional processing, including the fineness of the particles. Due to the fineness of tungsten materials, mineral processing methods may not always be efficient; therefore, the material may proceed directly to the extractive metallurgy step. Table 7 shows the global reprocessing trials for W recovery from tailings with their recovery rate and concentration grades obtained.
Global list of tailing reprocessing tests for tungsten recovery from tailings
Deposit | Country | Major tungsten mineral | Tungsten tailing type | Reprocessing methods | Tailings grade, WO3% | WO3 concentrate % | WO3 recovery rate | Ref. |
Tyrnyauz | Russia | Scheelite | Old molybdenum mine tailings | Flotation | 0.05 | 54-55 | ~62 | [117] |
Hutti gold dumps | India | Scheelite | Old Au tailings dumps with scheelite | Enhanced gravity separation (Falcon concentrator) | 0.024 | 17.16 | 67.5 | [112] |
Kolar dumps | India | Scheelite | Old Au tailings dumps with scheelite | Tabling, flotation, and magnetic separation | 0.01-0.53 | 65 | - | [118] |
Guangdong | China | Scheelite | Cu-S tailings | Gravity separation, flotation | 0.11 | 32.16 | 30.29 | [119] |
Shizhuyuan | China | Scheelite | Scheelite concentrate tailings | Gravity process-shaking tables | - | 25.45 | 44.83 | [120] |
Gansu | China | Scheelite | Scheelite flotation tailings | Flotation | - | 56.86 | 51.93 | [121] |
Panasqueira | Portugal | Wolframite | Historical W mine tailings | Flotation, magnetic separation, and gravity concentration | 0.1 | 50-55 | - | [56] |
Potosi | Bolivia | Wolframite | Tin mine tailings | Chlorination segregation, flotation, high-intensity magnetic separation, and gravity separation | 0.64 | 60.22 | ~64 | [122] |
Dajishan | China | Wolframite | Fine tungsten tailings | Flotation | 0.45 | 30.18 | 80 | [123] |
Rajasthan | India | Wolframite | High-intensity magnetic separation tungsten ore slime | Polymeric dispersant with magnetic separation | 2.87 and 5.30 | 5.4-11 | - | [124] |
Jiangxi | China | Wolframite | Tungsten mine tailings | Shaking table-flotation process | 0.32 | 55.64 | 75.28 | [101] |
Bom-Gohron | Russia | Wolframite | Tungsten mine tailings | Enhanced magnetic separation using dispersant | 0.1-0.35 | - | - | [50] |
CONCLUSIONS
Tungsten, with one of the highest melting points among elements, is categorized as a critical element in many countries due to its broad applications across numerous industries. Due to its critical status and EI, W tailings, as a secondary source, have been increasingly receiving attention to ensure the stability of the supply chain. The reprocessing of W tailings does prove challenging due to the fineness of tungsten particles and the presence of gangue minerals that interfere with the physicochemical separation routes. Different research clusters are endeavoring to find suitable reprocessing approaches that could optimally recover W from their tailings and diminish deleterious environmental footprints that are formed because of W tailing accumulation. Lack of transparency and data availability across the supply chain of tungsten hinders the potential development of R&D. The challenge of tungsten data availability was evidenced by a study of mass flow analysis (MFA) regarding 21 raw materials that recognized tungsten as one of the five elements that have the least data in the literature[125].
W tailings, particularly old tailings, have an economic value as they contain other metals, such as Au, Ag, Co, Ni, Mo, Zn, etc., depending on the nature of the original mineralization and the ore processing route. Reprocessing tailings are practical as they contain relatively high metal content due to less efficient extraction or obsolete methods. The lives of biota and human beings could be vulnerable should these tailings remain abandoned; therefore, proper management of the latter tailings is a must-do thing to ensure that the United Nations SDGs are implemented exhaustively. W tailings may have adverse impacts on both the environment (air, water, soil) and human beings, mainly depending on the level of exposure. Out of all pollutants, most W tailings are associated with scorodite (FeAsO4·2H2O) at significant levels. Improper management of such tailings could result in a well-known AMD problem and other serious issues related to the direct contact of heavy elements with air, food, or water.
Proper management of W tailings is highly encouraged, with two primary goals. The first one is to extract valuable elements, particularly tungsten, and the second one is the conservation of the environment. Should proper mineral processing routes be implemented for W tailings, taking into consideration their primary and present geologies, acceptable values of tungsten could be obtained, and therefore, the production from secondary sources would be enhanced[126]. This would be an important step as the demand for this metal in the aforementioned industries is skyrocketing. Provided that most of tungsten is secured from China, as it accounts for more than 0.84 of the world’s production (about 71 kt) and ~0.50 of the world’s reserves, focusing on this secondary resource could automatically contribute to the reduction of dependency[1]. As for the second goal of ensuring the safety of the ecosystem, proper disposal of W tailings and the application of recycling techniques can help reduce toxic elements such as arsenic and other related substances to tolerable levels as per WHO standards and other health regulatory bodies. Besides, effective management of tailings could also contribute to the reduction of the “Not in My Backyard” (NIMBY) Syndrome, as tailings are one of the contributing factors.
It is, therefore, recommended to apply advanced technologies such as enhanced gravity separation systems, flotation reagents, or a combination of physical separators to old tailings with economic value to ensure the maximum recovery of targeted elements from tailings. Besides, it would be important to consider life cycle assessment (LCA) and life cycle inventory (LCI) to ensure the management of the environment while thinking of such a reprocessing project on an industrial scale. Current and/or future R&D works should consider using modeling tools at each step, whether it is in processing or life cycle analysis, to better understand the operating costs and profitability of the overall process. While considering reprocessing tailings, an ethical and responsible recycling approach, such as Environmental, Social and Governance (ESG), should be implemented to embrace the circular economy.
DECLARATIONS
Authors’ contributions
Conceptualization, design of the study, data analysis, interpretation, original draft, editing: Msumange DA
Editing: Msumange JA
Conceptualization, design of the study, data analysis, interpretation, review, editing: Bru K, Bourgeois F
Availability of data and materials
Not applicable.
Financial support and sponsorship
The authors acknowledge the financial support of the French National Research Agency ANR through the ANR-21-CE04-0017 VARTA project (https://anr.fr/Projet-ANR-21-CE04-0017).
Conflicts of interest
All authors declared that there are no conflicts of interest.
Ethical approval and consent to participate
Not applicable.
Consent for publication
Not applicable.
Copyright
© The Author(s) 2023.
REFERENCES
1. USGS. Mineral commodity summaries 2023. Available from: https://pubs.er.usgs.gov/publication/mcs2023. [Last accessed on 6 Nov 2023].
2. Han Z, Golev A, Edraki M. A review of tungsten resources and potential extraction from mine waste. Minerals 2021;11:701.
3. Leal-ayala DR, Allwood JM, Petavratzi E, Brown TJ, Gunn G. Mapping the global flow of tungsten to identify key material efficiency and supply security opportunities. Resour Conserv Recycl 2015;103:19-28.
4. Balatsky AV, Balatsky GI, Borysov SS. Resource demand growth and sustainability due to increased world consumption. Sustainability 2015;7:3430-40.
5. European Commission. Study on the EU’s list of critical raw materials. Available from: https://op.europa.eu/en/publication-detail/-/publication/c0d5292a-ee54-11ea-991b-01aa75ed71a1/language-en. [Last accessed on 6 Nov 2023].
6. Akçil A, Erüst Ünal C, Okudan MD. Gaining critical raw materials to circular economy by recycling. Bilimsel Madencilik Dergisi 2022;61:168-78. (in Turkish). Available from: https://www.researchgate.net/publication/359502178_Gaining_Critical_Raw_Materials_to_Circular_Economy_by_Recycling. [Last accessed on 6 Nov 2023]
7. Yilmaz E, Koohestani B, Cao S. Chapter 13 - Recent practices in mine tailings’ recycling and reuse. In: Managing mining and minerals processing wastes. Elsevier; 2023. p. 271-304.
8. Msumange D, Yazici EY, Celep O, Deveci H, Kritskii A, Karimov K. Recovery of Au and Ag from the roasted calcine of a copper-rich pyritic refractory gold ore using ion exchange resins. Miner Eng 2023;195:108017.
9. Kang J, Hu Y, Sun W, et al. A significant improvement of scheelite flotation efficiency with etidronic acid. J Clean Prod 2018;180:858-65.
10. Foucaud Y. Tungsten recovery from a skarn with a low separation contrast: contribution of molecular modelling in the flotation of calcium minerals. (in French). Available from: http://docnum.univ-lorraine.fr/public/DDOC_T_2019_0149_FOUCAUD.pdf. [Last accessed on 6 Nov 2023].
11. Mulenshi J, Khavari P, Chehreh Chelgani S, Rosenkranz J. Characterization and beneficiation options for tungsten recovery from yxsjöberg historical ore tailings. Processes 2019;7:895.
12. British Geological Survey (BGS). Tungsten. Available from: https://nora.nerc.ac.uk/id/eprint/17445/1/tungstenProfile[1].pdf. [Last accessed on 6 Nov 2023].
13. Mulenshi J. Reprocessing historical tailings for possible remediation and recovery of critical metals and minerals - The Yxsjöberg case. Available from: https://ltu.diva-portal.org/smash/get/diva2:1507097/FULLTEXT01.pdf. [Last accessed on 6 Nov 2023]
14. Graedel TE, Barr R, Chandler C, et al. Methodology of metal criticality determination. Environ Sci Technol 2012;46:1063-70.
15. European Commission. Critical raw materials resilience: charting a path towards greater security and sustainability. Available from: https://ec.europa.eu/docsroom/documents/42849. [Last accessed on 6 Nov 2020].
16. Foucaud Y, Filippov L, Filippova I, Badawi M. The challenge of tungsten skarn processing by froth flotation: a review. Front Chem 2020;8:230.
17. Tungsten market size 2022 global comprehensive research study, trends, share, development status, opportunities, future plans, competitive landscape and growth by forecast 2026. Available from: https://www.digitaljournal.com/pr/tungsten-market-size-2022-global-comprehensive-research-study-trends-share-development-status-opportunities-future-plans-competitive-landscape-and-growth-by-forecast-2026. [Last accessed on 6 Nov 2023].
18. Wang X, Qin W, Jiao F, et al. Review of tungsten resource reserves, tungsten concentrate production and tungsten beneficiation technology in China. T Nonferr Metal Soc 2022;32:2318-38.
19. Liu H, Liu H, Nie C, Zhang J, Steenari BM, Ekberg C. Comprehensive treatments of tungsten slags in China: a critical review. J Environ Manage 2020;270:110927.
21. Foucaud Y, Filippova I, Dehaine Q, Hubert P, Filippov L. Integrated approach for the processing of a complex tungsten Skarn ore (Tabuaço, Portugal). Miner Eng 2019;143:105896.
22. Huang J, Ding Q, Wang Y, Hong H, Zhang H. The evolution and influencing factors of international tungsten competition from the industrial chain perspective. Resour Policy 2021;73:102185.
23. USGS. Mineral Commodity Summaries 2022. Available from: https://pubs.er.usgs.gov/publication/mcs2022. [Last accessed on 6 Nov 2023].
24. Global tungsten market to reach 115 thousand metric tons by 2026. Available from: https://www.globenewswire.com/en/news-release/2022/05/03/2434786/0/en/Global%20Tungsten%20Market-to-Reach-115-Thousand-Metric-Tons-by-2026.html. [Last accessed on 6 Nov 2023].
25. Global tungsten market 2024-2028. Available from: https://www.researchandmarkets.com/reports/5568234/global-tungsten-market-2024-2028. [Last accessed on 6 Nov 2023].
26. European Commission. Report on critical raw materials and the circular economy. Available from: https://op.europa.eu/en/publication-detail/-/publication/d1be1b43-e18f-11e8-b690-01aa75ed71a1/language-en/format-PDF/source-80004733. [Last accessed on 6 Nov 2023].
27. Mulenshi J, Gilbricht S, Chelgani SC, Rosenkranz J. Systematic characterization of historical tailings for possible remediation and recovery of critical metals and minerals - The Yxsjöberg case. J Geochem Explor 2021;226:106777.
28. Akcil A, Koldas S. Acid mine drainage (AMD): causes, treatment and case studies. J Clean Prod 2006;14:1139-45.
29. Khavari P. Characterization of historical tungsten ore tailings for pre-selection of feasible reprocessing methods. Available from: https://www.diva-portal.org/smash/get/diva2:1248295/FULLTEXT01.pdf. [Last accessed on 6 Nov 2023]
30. Alfonso P, Tomasa O, Garcia-valles M, Tarragó M, Martínez S, Esteves H. Potential of tungsten tailings as glass raw materials. Mater Lett 2018;228:456-8.
31. JXSC. W tailings. Available from: https://www.jxscmachine.com/new/tungsten-tailingsutilization/#:~:text=What%20is%20tungsten%20tailings%3F,%2C%20mica%2C%20calcite%2C%20etc. [Last accessed on 6 Nov 2023].
32. Castro-Gomes JP, Silva AP, Cano RP, Durán Suarez J, Albuquerque A. Potential for reuse of tungsten mining waste-rock in technical-artistic value added products. J Clean Prod 2012;25:34-41.
33. Li Y, Zhang Y. Surface modification of tungsten tailings. In: Proceedings of the 2015 6th International Conference on Manufacturing Science and Engineering. 2015.
34. Yim G, Ji S, Cheong Y, Cho DW. Feasibility evaluation of utilizing mine tailings as a paste backfill material for mine filling. In: the 25th EGU General Assembly; 2023 Apr 24-28; Vienna, Austria; 2023.
35. Rosario ARA. Application of the electrodialytic process for tungsten recovery and arsenic removal from mine tailings. Available from: https://run.unl.pt/bitstream/10362/48731/1/Rosario_2018.pdf. [Last accessed on 6 Nov 2023]
36. Figueiredo J, Vila MC, Matos K, et al. Tailings reprocessing from Cabeço do Pião dam in Central Portugal: a kinetic approach of experimental data. J Sustain Min 2018;17:139-44.
37. Wang X, Qin W, Jiao F, et al. Review on development of low-grade scheelite recovery from molybdenum tailings in Luanchuan, China: a case study of Luoyang Yulu Mining Company. T Nonferr Metal Soc 2022;32:980-98.
38. United Nations. The sustainable development goals report 2022. Available from: https://unstats.un.org/sdgs/report/2022/. [Last accessed on 6 Nov 2023].
39. Tomas H. Le traitement des minerais de tungstène à Salau. Industrie minérale - Les techniques 1985;67:243-7. (in French).
40. Audion AS, Labbé JF. Panorama mondial 2011 du marché du tungstène. Rapport public BRGM/RP-61341-FR, Orléans, 2012. Available from: https://infoterre.brgm.fr/rapports/RP-61341-FR.pdf. [Last accessed on 6 Nov 2023].
41. Kazamel BG, Jamieson HE, Leybourne MI, Falck H. Factors controlling tungsten mobility in W-Cu skarn tailings. Chem Geol 2023;630:121487.
42. Suárez Sánchez A, Krzemień A, Riesgo Fernández P, Iglesias Rodríguez FJ, Sánchez Lasheras F, de Cos Juez FJ. Investment in new tungsten mining projects. Resour Policy 2015;46:177-90.
43. Peng K, Yang H, Ouyang J. Tungsten tailing powders activated for use as cementitious material. Powder Technol 2015;286:678-83.
45. Grey IE, Birch WD, Bougerol C, Mills SJ. Unit-cell intergrowth of pyrochlore and hexagonal tungsten bronze structures in secondary tungsten minerals. J Solid State Chem 2006;179:3860-9.
46. Sahama TG. The secondary tungsten minerals, a review. Mineral Rec 1981;12:81-7. Available from: https://rruff-2.geo.arizona.edu/uploads/MR12_81.pdf. [Last accessed on 6 Nov 2023]
47. Lassner E, Schubert WD. Tungsten. Kluwer Academic/Plenum Publishers, New York, USA. 1999. Available from: https://chemistlibrary.files.wordpress.com/2015/05/tungsten-1999-lassner-schubert.pdf. [Last accessed on 6 Nov 2023].
49. Kim S, Baek SH, Han Y, Jeon HS. Laboratory testing of scheelite flotation from raw ore in Sangdong mine for process development. Minerals 2020;10:971.
50. Frolova IV, Tikhonov VV, Nalesnik OI, Streltsova AA. The enrichment of stale tailings of bom-gorhon tungsten ore deposits. Procedia Chem 2014;10:364-8.
51. Galera JM, de la Fuente F, García J, Calleja M, Pozo V. Design and construction of a tailings dam over an ancient tailings facility at La Parrilla mine. Mine Water Environ 2021;40:63-73.
52. Pascoe A. Collingwood plant for tungsten icon. Aust Paydirt 2009;1:69. Available from: https://search.informit.org/doi/epdf/10.3316/informit.033950255246461. [Last accessed on 6 Nov 2023]
53. North American tungsten investigates tailings reprocessing potential at the cantung mine site. Available from: https://theprospectornews.com/north-american-tungsten-investigates-tailings-reprocessing-potential-at-the-cantung-mine-site/. [Last accessed on 6 Nov 2023].
54. Hallberg A, Reginiussen H. Critical raw materials in ores, waste rock and tailings in Bergslagen. Geological Survey of Sweden; Uppsala, Sweden; 2020. Available from: https://www.sgu.se/globalassets/mineralnaring/mineralinformation/pdac-2021/critical-raw-materials-in-ores-waste-rock-and-tailings-in-bergslagen.pdf. [Last accessed on 6 Nov 2023].
55. Hällström LPB, Alakangas L, Martinsson O. Scheelite weathering and tungsten (W) mobility in historical oxidic-sulfidic skarn tailings at Yxsjöberg, Sweden. Environ Sci Pollut Res Int 2020;27:6180-92.
56. Clemente D, Newling P, Botelho de Sousa A, Lejeune G, Barber SP, Tucker P. Reprocessing slimes tailings from a tungsten mine. Miner Eng 1993;6:831-9.
57. Barros SCLR, Ulla-Maija M, Willersinn S, Yang XS. Mapping the secondary resources in the EU (mine tailings, industrial waste). ICCRAM; Burgos, Spain. 2016. Available from: https://2020.prometia.eu/wp-content/uploads/2020/12/MSP-REFRAM-D3.1-Mapping-the-secondary-resources-in-the-eu-mine-tailings_industrial-waste.pdf. [Last accessed on 6 Nov 2023].
58. Chung AP, Coimbra C, Farias P, et al. Tailings microbial community profile and prediction of its functionality in basins of tungsten mine. Sci Rep 2019;9:19596.
59. Choi YW, Kim YJ, Choi O, Lee KM, Lachemi M. Utilization of tailings from tungsten mine waste as a substitution material for cement. Constr Build Mater 2009;23:2481-6.
60. Ikramova ZO, Mukhamedzhanova MT, Tukhtaeva GG. Tungsten-molybdenum ore flotation tailings for ceramic tile production. Glass Ceram 2009;66:102-3.
61. Doroshkevich SG, Bardamova IV. Phytotoxicity of tailings dam of the dzhidinsky tungsten-molybdenum combine (Western Transbaikalia). In: Frank-Kamenetskaya O, Panova E, Vlasov D, editors. Biogenic - Abiogenic Interactions in Natural and Anthropogenic Systems. Cham: Springer International Publishing; 2016. p. 277-87.
62. Meinert LD, Dipple GM, Nicolescu S. World skarn deposits. In: Hedenquist JW, Thompson JFH, Goldfarb RJ, Richards JP, editors. One Hundredth Anniversary Volume. Society of Economic Geologists; 2005.
63. Schmidt S, Geo P, Berbau W. From deposit to concentrate: the basics of tungsten mining. Part 1: project generation and project development. In: Proceedings of the ITIA’s 25th anniversary annual general meeting; 2012. Available from: https://www.itia.info/assets/files/newsletters/Newsletter_2012_06.pdf. [Last accessed on 6 Nov 2023]
64. Petrunic BM, Al TA. Mineral/water interactions in tailings from a tungsten mine, Mount Pleasant, New Brunswick. Geochim Cosmochim Acta 2005;69:2469-83.
65. Bhanbhro R. Mechanical properties of tailings: basic description of a tailings material from Sweden. Available from: https://www.diva-portal.org/smash/get/diva2:989943/FULLTEXT01.pdf. [Last accessed on 6 Nov 2023]
66. Acosta JA, Faz A, Martínez-Martínez S, Zornoza R, Carmona DM, Kabas S. Multivariate statistical and GIS-based approach to evaluate heavy metals behavior in mine sites for future reclamation. J Geochem Explor 2011;109:8-17.
67. Petrunic BM, Al TA, Weaver L. A transmission electron microscopy analysis of secondary minerals formed in tungsten-mine tailings with an emphasis on arsenopyrite oxidation. Appl Geochem 2006;21:1259-73.
68. Kazamel BG, Jamieson HE, Leybourne MI, Falck H, Johannesson KH. Aqueous geochemistry and mineralogy of tungsten with emphasis on mine wastes. Econ Geol 2023;118:659-74.
69. Han Z, Levett A, Edraki M, Jones MWM, Howard D, Southam G. Microbially influenced tungsten mobilization and formation of secondary minerals in wolframite tailings. J Hazard Mater 2023;445:130508.
70. Kreissl S, Bolanz R, Göttlicher J, Steininger R, Tarassov M, Markl G. Structural incorporation of W6+ into hematite and goethite: a combined study of natural and synthetic iron oxides developed from precursor ferrihydrite and the preservation of ancient fluid compositions in hematite. Am Mineral 2016;101:2701-15.
71. Murciego A, Alvarez-Ayuso E, Pellitero E, et al. Study of arsenopyrite weathering products in mine wastes from abandoned tungsten and tin exploitations. J Hazard Mater 2011;186:590-601.
74. Flemming RL, Salzsauler KA, Sherriff BL, Sidenko NV. Identification of scorodite in fine-grained, high-sulfide, arsenopyrite mine-waste using micro X-ray diffraction (μXRD). Can Mineral 2005;43:1243-54.
75. Koutsospyros A, Braida W, Christodoulatos C, Dermatas D, Strigul N. A review of tungsten: from environmental obscurity to scrutiny. J Hazard Mater 2006;136:1-19.
76. Wasel O, Freeman JL. Comparative assessment of tungsten toxicity in the absence or presence of other metals. Toxics 2018;6:66.
77. Toxicological Profile for Tungsten. Available from: https://www.atsdr.cdc.gov/toxprofiles/tp186.pdf. [Last accessed on 3 Nov 2023].
78. Reutova NV, Reutova TV, Dreeva FR, Shevchenko AA. Long-term impact of the Tyrnyauz tungsten-molybdenum mining and processing factory waste on environmental pollution and children’s population. Environ Geochem Health 2022;44:4557-68.
79. Seiler RL, Stollenwerk KG, Garbarino JR. Factors controlling tungsten concentrations in ground water, Carson Desert, Nevada. Appl Geochem 2005;20:423-41.
80. Sheppard PR, Ridenour G, Speakman RJ, Witten ML. Elevated tungsten and cobalt in airborne particulates in Fallon, Nevada: possible implications for the childhood leukemia cluster. Appl Geochem 2006;21:152-65.
81. Sheppard PR, Witten ML. Dendrochemistry of urban trees in an environmental exposure analysis of childhood leukemia cluster areas. EOS Trans AGU 2023;84. Available from: https://www.researchgate.net/publication/253790587_Dendrochemistry_of_Urban_Trees_in_an_Environmental_Exposure_Analysis_of_Childhood_Leukemia_Cluster_Areas. [Last accessed on 3 Nov 2023]
82. Kraus T, Schramel P, Schaller KH, Zöbelein P, Weber A, Angerer J. Exposure assessment in the hard metal manufacturing industry with special regard to tungsten and its compounds. Occup Environ Med 2001;58:631-4.
83. Sahle W, Krantz S, Christensson B, Laszlo I. Preliminary data on hard metal workers exposure to tungsten oxide fibres. Sci Total Environ 1996;191:153-67.
84. Candeias C, Ávila PF, da Silva EF, Ferreira A, Durães N, Teixeira JP. Water-rock interaction and geochemical processes in surface waters influenced by tailings impoundments: impact and threats to the ecosystems and human health in rural communities (Panasqueira Mine, Central Portugal). Water Air Soil Pollut 2015;226:23.
85. Blowes DW, Ptacek CJ, Jambor JL, Weisener CG. 9.05 - The geochemistry of acid mine drainage. Treatise Geochem 2003;9:149-204.
86. State Council. Environmental protection law of the PRC. Available from: http://www.npc.gov.cn/npc/c1773/c2518/c27694/c27698/201905/t20190521_208293.html. (in Chinese). [Last accessed on 3 Nov 2023].
87. Lekic M, Crnogorac L, Pantelic U, Nikic Z. Possibility of application of phytoremediation in mining. In: 6th International Symposium on Mining and Environmental Protection; Vrdnik, Serbia. 2017. Available from: https://www.researchgate.net/publication/319902007_POSSIBILITY_OF_APPLICATION_OF_PHYTOREMEDIATION_IN_MINING. [Last accessed on 3 Nov 2023]
88. Lamb DT, Venkatraman K, Bolan N, Ashwath N, Choppala G, Naidu R. Phytocapping: an alternative technology for the sustainable management of landfill sites. Crit Rev Environ Sci Technol 2014;44:561-637.
89. Wong MH. Ecological restoration of mine degraded soils, with emphasis on metal contaminated soils. Chemosphere 2003;50:775-80.
90. Tordoff GM, Baker AJM, Willis AJ. Current approaches to the revegetation and reclamation of metalliferous mine wastes. Chemosphere 2000;41:219-28.
91. Sharma HD, Reddy KR. Geoenvironmental engineering: site remediation, waste containment, and emerging waste management technologies. 2004. Available from: https://www.wiley.com/en-us/Geoenvironmental+Engineering%3A+Site+Remediation%2C+Waste+Containment%2C+and+Emerging+Waste+Management+Technologies-p-9780471215998. [Last accessed on 3 Nov 2023].
92. Ghosh M. A review on phytoremediation of heavy metals and utilization of its byproducts. Appl Ecol Env Res 2005;3:1-18.
93. Karaca O, Cameselle C, Reddy KR. Mine tailing disposal sites: contamination problems, remedial options and phytocaps for sustainable remediation. Rev Environ Sci Biotechnol 2018;17:205-28.
94. Mendez MO, Maier RM. Phytoremediation of mine tailings in temperate and arid environments. Rev Environ Sci Biotechnol 2008;7:47-59.
95. USEPA. Introduction to phytoremediation. Available from: https://cfpub.epa.gov/si/si_public_record_report.cfm?Lab=NRMRL&dirEntryId=63433. [Last accessed on 3 Nov 2023].
96. Reddy KR, Adams JA. Sustainable remediation of contaminated sites. Momentum Press; 2015. Available from: https://www.researchgate.net/publication/312433183_Sustainable_Remediation_of_Contaminated_Sites. [Last accessed on 3 Nov 2023].
97. Tamás J, Kovács A. Vegetation pattern and heavy metal accumulation at a mine tailing at Gyöngyösoroszi, hungary. Z Naturforsch C J Biosci 2005;60:362-7.
98. Prasad MNV. Phytoremediation of metals and radionuclides in the environment: the case for natural hyperaccumulators, metal transporters, soil-amending chelators and transgenic plants. In: Prasad MNV, editor. Heavy metal stress in plants. Berlin: Springer Berlin Heidelberg; 2004. p. 345-91.
99. Favas PJC, Pratas J, Varun M, D’souza R, Paul MS. Phytoremediation of soils contaminated with metals and metalloids at mining areas: potential of native flora. In: Hernandez-Soriano MC, editor. Environmental risk assessment of soil contamination. 2014. p. 486-510.
100. Figueiredo J, Vila MC, Fiúza A, et al. A holistic approach in re-mining old tailings deposits for the supply of critical-metals: a portuguese case study. Minerals 2019;9:638.
101. Huang Z, Zhang S, Wang H, et al. Recovery of wolframite from tungsten mine tailings by the combination of shaking table and flotation with a novel “crab” structure sebacoyl hydroxamic acid. J Environ Manage 2022;317:115372.
102. Petruk W. Chapter 7 - Applied mineralogy: porphyry copper deposits. In: Applied Mineralogy in the Mining Industry. Elsevier; 2000. p. 135-47.
103. Tungpalan K, Wightman E, Manlapig E. Relating mineralogical and textural characteristics to flotation behaviour. Miner Eng 2015;82:136-40.
104. Lotter NO, Oliveira JF, Hannaford AL, Amos SR. Flowsheet development for the Kamoa project - A case study. Miner Eng 2013;52:8-20.
105. Whiteman E, Lotter NO, Amos SR. Process mineralogy as a predictive tool for flowsheet design to advance the Kamoa project. Miner Eng 2016;96-7:185-93.
106. Burt RO. Gravity concentration methods. In: Yarar B, Dogan ZM, editors. Mineral processing design. Dordrecht: Springer Netherlands; 1987. p. 106-37.
107. Das A, Sarkar B. Advanced gravity concentration of fine particles: a review. Miner Process Extr Metall Rev 2018;39:359-94.
108. Burt RO, Mills C. Gravity concentration technology. Elsevier; 1984. Available from: https://www.osti.gov/biblio/7181338. [Last accessed on 3 Nov 2023]
109. Abols JA, Grady PM. Maximizing gravity recovery through the application of multiple gravity devices. Available from: https://citeseerx.ist.psu.edu/viewdoc/download;jsessionid=A6D569026D2BFE861C3B4CA9AE20F638?doi=10.1.1.492.1408&rep=rep1&type=pdf. [Last accessed on 3 Nov 2023].
110. Meng Q, Feng Q, Ou L. Recovery enhancement of ultrafine wolframite through hydrophobic flocs magnetic separation. Miner Process Extr Metall Rev 2017;38:298-303.
111. Yue T, Han H, Hu Y, et al. Beneficiation and purification of tungsten and cassiterite minerals using Pb-BHA complexes flotation and centrifugal separation. Minerals 2018;8:566.
112. Das SK, Kundu T, Dash N, Angadi SI. Separation behavior of Falcon concentrator for the recovery of ultrafine scheelite particles from the gold mine tailings. Sep Purif Technol 2023;309:123065.
113. Dehaine Q, Tijsseling LT, Glass HJ, Törmänen T, Butcher AR. Geometallurgy of cobalt ores: a review. Miner Eng 2021;160:106656.
114. Ye M, Li G, Yan P, et al. Removal of metals from lead-zinc mine tailings using bioleaching and followed by sulfide precipitation. Chemosphere 2017;185:1189-96.
115. Blazevic A, Albu M, Mitsche S, Rittmann SKR, Habler G, Milojevic T. Biotransformation of scheelite CaWO4 by the extreme thermoacidophile metallosphaera sedula: tungsten-microbial interface. Front Microbiol 2019;10:1492.
116. Lee E, Han Y, Park J, et al. Bioleaching of arsenic from highly contaminated mine tailings using Acidithiobacillus thiooxidans. J Environ Manage 2015;147:124-31.
117. Evdokimov SI, Evdokimov VS. Flotation of wolfram-molybdenum sand tailings at Tyrnyauz processing plant. Gorn Zhurnal 2015:63-8.
118. Rao GM, Subrahmanyam NN. Beneficiation of tungsten ores in India - problems, processes, applications and demands in general on a global scene. Fizykochemiczne Problemy Mineralurgii 1986;18:23-37. Available from: https://www.journalssystem.com/ppmp/pdf-80262-16167?filename=Beneficiation%20of%20tungsten.pdf. [Last accessed on 6 Nov 2023]
119. Zhong G, Yu L, Liu L, Zhong S, Zhang C. Experiment on recovering scheelite from the Cu-S tailings by gravity separation. Chin Tungsten Ind 2016;252:17-21. Available from: http://caod.oriprobe.com/articles/48370725/Experiment_on_Recovering_Scheelite_from_the_Cu_S_Tailings_by_Gravity_S.htm. [Last accessed on 6 Nov 2023]
120. Xiao W, Xie J, Chen Z. New gravity separation technology for recovering tungsten and cassiterite from the tailings of scheelite concentrate. Chin Tungsten Ind 2015;247:14-7. Available from: http://caod.oriprobe.com/articles/45503795/New_Gravity_Separation_Technology_for_Recovering_Tungsten_and_Cassiter.htm. [Last accessed on 6 Nov 2023]
121. Li M, Wang X. Experimental research on recycling flotation tailings in a scheelite mine in Gansu. Chin Tungsten Ind 2015;246:17-20. Available from: http://caod.oriprobe.com/articles/44928374/Experimental_Research_on_Recycling_Flotation_Tailings_in_a_Scheelite_M.htm. [Last accessed on 6 Nov 2023]
122. Xiao JH, Feng QM, Fan SP, Xu LH, Wang Z. Comprehensive utilization of copper, tungsten and tin polymetallic tailings in Bolivia. Chin J Nonferrous Met 2013;23:2949-61. (in Chinese).
123. Lv J, Qu Z, Li M. Industrialized flotation experiments on the fine mud discharge of a wolframite concentrator. Chin Tungsten Ind 2015;30:36-40. Available from: https://caod.oriprobe.com/articles/44928375/Industrialized_Flotation_Experiments_on_the_Fine_Mud_Discharge_of_a_Wo.htm. [Last accessed on 6 Nov 2023]
124. Bhagat RP, Pathak PN. The effect of polymeric dispersant on magnetic separation of tungsten ore slimes. Int J Miner Process 1996;47:213-7.
125. RPA. Study on data needs for a full raw materials flow analysis. Available from: https://op.europa.eu/en/publication-detail/-/publication/10ed5c27-edec-43de-8e6f-9676e9308af5. [Last accessed on 6 Nov 2023].
Cite This Article
Export citation file: BibTeX | RIS
OAE Style
Msumange DA, Msumange JA, Bru K, Bourgeois F. Tungsten tailings issues and reprocessing solutions. Miner Miner Mater 2023;2:14. http://dx.doi.org/10.20517/mmm.2023.21
AMA Style
Msumange DA, Msumange JA, Bru K, Bourgeois F. Tungsten tailings issues and reprocessing solutions. Minerals and Mineral Materials. 2023; 2(4): 14. http://dx.doi.org/10.20517/mmm.2023.21
Chicago/Turabian Style
Msumange, Deus Albert, Joshua Albert Msumange, Kathy Bru, Florent Bourgeois. 2023. "Tungsten tailings issues and reprocessing solutions" Minerals and Mineral Materials. 2, no.4: 14. http://dx.doi.org/10.20517/mmm.2023.21
ACS Style
Msumange, DA.; Msumange JA.; Bru K.; Bourgeois F. Tungsten tailings issues and reprocessing solutions. Miner. Miner. Mater. 2023, 2, 14. http://dx.doi.org/10.20517/mmm.2023.21
About This Article
Special Issue
Copyright
Data & Comments
Data
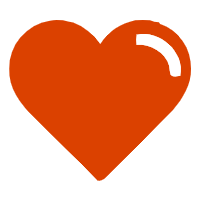

Comments
Comments must be written in English. Spam, offensive content, impersonation, and private information will not be permitted. If any comment is reported and identified as inappropriate content by OAE staff, the comment will be removed without notice. If you have any queries or need any help, please contact us at support@oaepublish.com.