Towards an understanding of the aetiology, genomic landscape and management of Moebius syndrome
Abstract
Moebius Syndrome (MBS) is a rare neurodevelopmental disorder characterised by facial paralysis and ocular motility defects. Its origins trace back to the 19th century, with its clinical delineation attributed to German neurologist Paul Möbius. The syndrome presents with a spectrum of variable systemic clinical features, necessitating a multidisciplinary approach to diagnosis and management. The prevalence of MBS has been estimated to range between 1 in 50,000 to 1 in 500,000 individuals, with a universal distribution across ethnicities and genders. The aetiology of MBS is poorly understood but is likely multifactorial, with developmental, genetic, and environmental factors playing roles. Recent research has identified potential genetic contributors, REV3L and PLXND1, but further work is needed to elucidate the genetic landscape of this rare neurodevelopmental disorder. Here we describe the current understanding of the clinical features, aetiology, genetic landscape, and management of MBS, emphasising the importance of early diagnosis and a holistic approach to patient care. We also propose a set of criteria aimed at standardising MBS reporting to enhance information sharing and bolster MBS research initiatives. Collaborative research efforts in the future hold the potential to offer transformative insights and improved outcomes for affected individuals and their families.
Keywords
INTRODUCTION
Moebius Syndrome (MBS) serves as a paradigmatic example in the field of neurodevelopmental disorders, highlighting the intricate and often elusive interplay between developmental biology, genetic predispositions, and environmental determinants in disease aetiology. The syndrome’s initial clinical delineation can be traced back to the observations of German ophthalmologist Alfred Von Gräfe in 1880[1]. However, it was subsequently named after Paul Möbius, a German neurologist who, in 1888[2], published a more comprehensive clinical characterisation of the disorder.
MBS is a rare congenital disorder characterised by facial paralysis and ocular abduction defects in the presence of full vertical gaze[3]. Neonates with MBS often present with distinctive clinical markers, such as asymmetrical or “expressionless” facies, feeding difficulties, and an inability to achieve complete eyelid closure. Cases with unilateral manifestations, which are occasionally attributed to perinatal trauma[4], can present diagnostic ambiguities, necessitating a more nuanced clinical evaluation. Beyond these primary manifestations, MBS exhibits a much broader clinical spectrum, encompassing a range of associated congenital anomalies. These can range from limb and cardiac abnormalities to craniofacial dysmorphisms, adding layers of complexity to its clinical and diagnostic profile[5].
Current epidemiological data on MBS prevalence remain somewhat nebulous, with estimates suggesting a range from 1 in 50,000 to 1 in 500,000 individuals[6]. It is noteworthy that this non-progressive syndrome exhibits a pan-ethnic and pan-gender distribution, emphasising its universal clinical relevance[3].
The pathogenesis and underlying molecular mechanisms of MBS remain poorly understood[7]. While a handful of familial cases provide tantalising hints towards potential genetic underpinnings[8], the broader landscape of genetic and environmental interactions, especially in the context of the cranial nerve developmental anomalies characteristic of MBS, remains to be fully delineated[9].
Historically, the relative rarity and inherent diagnostic complexities associated with MBS have led to it being somewhat marginalised in the broader context of neurodevelopmental research. This historical oversight underscores the pressing need for more rigorous, multidisciplinary research endeavours. As the scientific and medical communities deepen their exploration into the intricacies of Moebius Syndrome, it becomes increasingly evident that a holistic, integrative approach is essential. Delving into the genetic basis of MBS not only offers insights into its pathogenesis but also holds the potential to revolutionise therapeutic interventions[4]. This paves the way for precision medicine tailored to the individual’s genetic makeup[10]. Such an approach should not only aim to unravel the molecular, genetic, and clinical characteristics of the syndrome but also to develop and refine management strategies and support frameworks for affected individuals and their families. By doing so, we can significantly enhance patient outcomes[11], improve quality of life, and broaden our understanding of this enigmatic neurodevelopmental condition.
CLINICAL FEATURES OF MBS
Moebius Syndrome (MBS) is a complex neurodevelopmental disorder with a spectrum of clinical manifestations[5]. At its core, MBS is characterised by congenital, non-progressive facial weakness and limited abduction of one or both eyes[3]. The diagnostic criteria have evolved over time, with the current minimum being congenital, non-progressive, bilateral or unilateral, symmetrical or asymmetrical cranial nerve VI (CNVI), and CNVII palsies, accompanied by a full vertical gaze[8,12].
The hallmark clinical features of MBS stem from CN VI and VII dysfunction, leading to the distinctive loss of facial expression and compromised ocular motility. However, the syndrome’s clinical landscape is vast and often extends beyond these primary manifestations[5] [Figure 1]. Other cranial neuropathies, particularly involving CN V, IX, X, and XII, have been documented[9,13]. These result in oro-motor disorders, manifesting as impaired sucking and swallowing, which can lead to failure to thrive in infancy and dysphagia[3,14]. Feeding difficulties in infants with MBS are further exacerbated by the presence of a high-arched or cleft palate, observed in approximately 70% of patients[5]. Speech delay, dysarthria, poor intelligibility, and other speech problems are frequently encountered in MBS, further complicating the clinical picture[3,9,14]. Historically, children with MBS were believed to have a much higher prevalence of autism and autistic-like behaviours compared to the general population[4]. However, the exact prevalence is not clear due to the known challenges of making a diagnosis of autism in the context of impaired social and communication skills secondary to a physical/structural condition, such as MBS.
Figure 1. Clinical features of MBS. All MBS patients exhibit both CN VI and VII palsy in the presence of a full vertical gaze (as per the minimal diagnostic criteria). The prevalence of additional features is as previously reported.
Sleep disturbances are a notable concern in Moebius Syndrome, with approximately one-third of affected children experiencing sleep-related challenges[8]. These disturbances are believed to arise from the maldevelopment of the rhombencephalon[15] with the reticular formation, which is involved in the sleep-wake cycle, in close proximity to the area of the brainstem affected by MBS[15]. Manifestations include night terrors, sleepwalking, and recurrent awakenings with subsequent difficulties in resuming sleep. Such sleep disruptions not only impact the child but also pose a significant strain on their families. For some individuals with MBS, these sleep issues can persist, extending into their adult years and necessitating ongoing management and support[5,8].
The syndrome’s diverse clinical features also encompass lingual hypoplasia, sensorineural hearing loss, and craniofacial dysmorphisms such as epicanthic folds, micrognathia, and dysplastic or low-set ears[13,16]. Limb abnormalities, including syndactyly, pes planus, and talipes, are not uncommon[13,16]. Notably, over a third of MBS patients exhibit an association with Poland anomaly[5]. Cardiac anomalies, such as dextrocardia, atrial or ventricular septal defects, and transposition of the great vessels, further complicate the clinical picture[5,17]. While the majority of MBS patients possess normal cognitive abilities, about 10% may experience varying degrees of intellectual disability[16]. Developmental delays and motor coordination challenges are frequently associated with MBS[5]. Neuroimaging often reveals abnormalities consistent with the clinical symptoms of CN VI and VII dysfunction, including an absent facial colliculus and pontine hypoplasia[18]. Additionally, lower brainstem hypoplasia has been linked to the occasional primary respiratory dysfunction observed in MBS cases[16].
The physical manifestations, coupled with the psychosocial challenges stemming from facial paralysis and other symptoms, can significantly impact the quality of life of affected individuals. This underscores the importance of a comprehensive approach to care, addressing both the medical and emotional needs of MBS patients[3,14,19].
Two clinical subgroups of MBS
A recent systematic review and statistical cluster analysis have shed light on the heterogeneity within the MBS diagnosis, suggesting the existence of two distinct subgroups[5] [Figure 2]. Both subtypes of MBS are defined by the presence of CN VI and VII palsy in the presence of a full vertical gaze. Type 1 MBS is deemed the “less severe” form and is marked by clustering of micrognathia, limb anomalies, and swallowing difficulties[5]. Nearly two-thirds of patients exhibiting any one of these features had all three[5]. In contrast, Type 2 MBS, considered the "more severe" subtype, displays a broader phenotypic diversity, with abnormal neuroimaging findings and developmental delays being more prevalent in this subgroup[5] [Figure 2]. It is important to note that the number of patients included in this cluster analysis was small and that many of these cases were identified before the advent of modern genetic testing methods such as chromosome microarray testing or whole exome or genome sequencing (WGS)[5].
Figure 2. Two subgroups of MBS. Clinical features of MBS cluster to form two subgroups of MBS. Type 1 MBS tends to exhibit limb abnormalities, micrognathia, and swallowing difficulties. Nearly two-thirds of patients exhibiting any one of the marked features (*) had all three of those features. Type 2 MBS is more likely to include developmental delay, additional cranial nerve palsies, and palatal and brain imaging abnormalities, but the clustering is weaker.
The heterogeneity and rarity of MBS have historically led to significant diagnostic uncertainties, compounded by the lack of consistent diagnostic criteria in the past. This inconsistency is evident in the literature, where many cases previously labelled as "Moebius" might not align with the current minimum diagnostic criteria. For instance, reports associating MBS with hypogonadotropic hypogonadism are now believed to represent congenital fibrosis of the extraocular muscles (CFEOM)[20] due to TUBB3 mutations. Similarly, the chromosome 3 locus initially linked to Moebius syndrome in cases of hereditary congenital facial paresis (HCFP)[21] was later found to involve patients without any eye movement abnormalities, a key feature of MBS. Almost all reports of familial MBS associations fail to meet the current minimal MBS criteria. These examples highlight the complexities and misinterpretations arising from the previous lack of standardised diagnostic approaches. Such inconsistencies in diagnosis have impeded efforts to share knowledge and understand the natural history and aetiology of MBS. Recognising these challenges, we propose a set of diagnostic criteria for typical and isolated MBS based on our experience in running a national multidisciplinary MBS clinic [Table 1], which we hope will facilitate more consistent reporting of MBS across the medical and research communities. There is clearly a need for detailed phenotypic analysis of larger MBS cohorts in the future, as only systematic reporting of features (and their absence) will provide high-quality data to develop and validate more refined diagnostic MBS criteria in the future.
Proposed diagnostic criteria for MBS utilising a combination of essential and supporting features to facilitate standardised MBS diagnosis and reporting
Diagnosis | Criteria | Supporting features |
Typical MBS | Essential features: •Bilateral congenital, non-progressive CNVI palsy •Bilateral congenital, non-progressive CNVII palsy •Presence of a full vertical gaze Presence of all three essential features (bilateral or unilateral) plus ≥ 2 supporting features in the absence of chromosome abnormalities | Neurological: •Involvement of other cranial nerves •Hypotonia •Dysarthria •Abnormal motor coordination •Brain imaging abnormalities (e.g., volumetric reduction in key brainstem areas) Musculoskeletal: •Lower limb anomalies (e.g., talipes) •Upper limb anomalies (e.g., Poland anomaly, symbrachydactyly) Craniofacial: •Craniofacial dysmorphism (e.g., low set/dysplastic ears, hypertelorism, epicanthic folds) •High or cleft palate •Micrognathia •Hypoplastic/atrophic tongue Gastrointestinal/Nutritional: •Swallowing/feeding difficulties •Failure to thrive Cardiovascular: •Cardiac anomalies (e.g., dextrocardia, atrial/ventricular septal defects, transposition of the great vessels) Developmental: •Developmental delay •Sleep problems •Behavioural and/or social communication difficulties |
Isolated MBS | Presence of all three essential features (bilateral or unilateral) plus ≤ 1 supporting features in the absence of chromosome abnormalities | |
Alternative diagnosis likely | •Presence of only two essential features with or without supporting features ·Presence of three essential features and one of: atypical additional features, such as profound intellectual disability or atypical dysmorphic features ·Family history |
AETIOLOGY OF MBS
Moebius Syndrome (MBS) demonstrates the many challenges of elucidating the interplay between embryonic development, genetic determinants, and environmental influences in deciphering the aetiology of complex neurodevelopmental disorders.
Historically, the structural anomalies associated with MBS have been attributed to aberrations during the embryonic development of the mesencephalon-rhombencephalon, specifically between the 4th and 5th weeks of embryonic development[22]. This period is critical for dorsoventral patterning, and disruptions during this phase can lead to the characteristic manifestations of MBS. The primary histopathological hallmark of MBS is observed in the facial colliculus, situated in the pontine tegmentum. This region houses the nuclei for the intra-axial fibres of CNVI and CNVII[23]. The prevailing developmental model posits that an initial insult during this embryonic period triggers a cascade of secondary events, culminating in the clinical presentation of MBS[14]. This primary insult is believed to be localised ischaemia in the dorso-medial aspect of the brain stem. This region is particularly vulnerable to hypoxic-ischemic injury because of the distribution pattern of the embryonic basilar artery branches[18,24].
Several environmental factors have been implicated in the pathogenesis of MBS. The theory of vascular disruption, particularly in the territory of the subclavian artery during the sixth week of embryogenesis, has gained traction[4]. This disruption could arise from foetal vascular events, including thrombus formation, embolism, or haemorrhage. Additionally, vasoconstrictive agents, such as cocaine[25], ergotamine[5], or misoprostol-induced uterine contractions[26,27], can attenuate blood flow during critical developmental windows, potentially precipitating MBS[23,28]. However, it is worth noting that the involvement of the subclavian artery does not fully account for the clinical manifestations of MBS, necessitating further exploration.
The aetiology of MBS is likely multifactorial [Figure 3], with a combination of genetic, environmental, and perhaps stochastic factors converging to manifest the syndrome[4]. This complexity necessitates a multidimensional approach to research, integrating genetic studies with environmental and epidemiological data to holistically understand MBS’s origins. In particular, the role of genetic factors in the aetiology of MBS cannot be overlooked[4]. The inherent complexity of MBS suggests that disruptions in various cellular and developmental processes might converge on a common outcome[29]. Consequently, multiple genes, each involved in distinct pathways, might collectively contribute to MBS’s pathophysiology. This notion of genetic heterogeneity is further supported by the observation that different genes might be implicated in MBS across different families[4,12,29]. The interplay between genetic predispositions and environmental factors [Figure 3] might render certain individuals more susceptible to the environmental insults that precipitate MBS.
Figure 3. Multifactorial aetiology of MBS. (A) Represents an axial section of the brainstem at the level of the facial colliculus, illustrating the abducent (CNVI) and facial (CNVII) nuclei and motor neurons, as present in typical development. (B) Illustrates how multifactorial insults in early embryonic development can result in hypoplastic CNVI and CNVII nuclei and/or reduced number of CNVI and CNVII motor neurons, resulting in the key clinical manifestations of MBS.
The aetiological landscape of Moebius Syndrome is intricate, with multiple layers of complexity. While significant strides have been made in understanding its embryonic, environmental, and genetic facets, many questions remain unanswered. The potential involvement of multiple genes, each contributing to a shared clinical outcome, underscores the need for comprehensive genetic analyses. It is hoped that a better understanding of the genetic factors in MBS will help devise aetiology-based disease classifications and offer opportunities for personalised medical management of MBS patients.
GENETIC LANDSCAPE OF MBS
Recent advances in genetic testing, particularly whole-exome and genome sequencing, have ushered in a new era of understanding for neurodevelopmental disorders. However, the genetic basis of MBS is still not fully understood. The majority of MBS cases are sporadic, presenting a notably low recurrence risk among siblings (which is often quoted to be around 2%-3%[8]). Such a pattern could align with several genetic scenarios: dominant de novo mutations, the interplay of environmental factors, or polygenic inheritance mechanisms. Another possibility is that causative genetic alterations exhibit pronounced reduced penetrance and variable expressivity. This makes the task of characterising the genetic factors integral to MBS particularly daunting, especially given the syndrome’s rarity and its phenotypic pleiotropy.
Nevertheless, establishing genotype-phenotype correlations in Moebius syndrome is of paramount importance. Such correlations would not only shed light on the disorder’s pathogenesis but also hold significant implications for its diagnosis, prognosis, and management. In this evolving landscape, recent research has spotlighted two promising candidate genes that could be instrumental in the pathogenesis of MBS.
REV3L: a key contender in MBS pathogenesis
Tomas-Roca et al. described three different pathogenic de novo mutations in REV3L among MBS patients[29]. The REV3L gene, encoding a catalytic subunit of DNA polymerase zeta (Pol zeta), is instrumental in DNA repair, particularly through the process of translesion synthesis[30,31]. This mechanism ensures the replication of damaged DNA while preserving genome integrity and stability. In mice at midgestation, REV3L is highly expressed in the developing embryonic brain, including areas that form the rhombencephalon[29]. Biallelic inactivation of Rev3l in mice leads to embryonic lethality associated with pleiotropic morphological abnormalities and extensive apoptosis, especially of the brain[29]. Furthermore, REV3L heterozygous mice showed significantly reduced hindbrain volumes with a strong decrease in the number of motoneurons in the facial motor nucleus. This situation is reminiscent of the anomalous neuroimaging findings in MBS patients[29].
Beyond its role in DNA repair, recent research has demonstrated that REV3L localises to mitochondria in human cells and that its inactivation results in mitochondrial dysfunction[32]. The same study reports that REV3L is associated with regions of mitochondrial DNA that are particularly susceptible to DNA damage after exposure to UV light. Finally, experiments with Rev3L knockout cells indicate that REV3L likely plays an important role in the maintenance of mitochondrial DNA[32]. In the context of MBS, loss of mitochondrial DNA stability due to alterations in REV3L could result in mitochondrial depletion, rendering cells more sensitive to ischemic insults.
Notably, the influence of REV3L extends beyond MBS. Another study investigating chromosomal imbalances in Poland Syndrome (PS) patients highlighted REV3L’s association with this condition[33]. PS, which usually presents with pectoralis muscle agenesis and upper limb malformation - a feature also observed in MBS - revealed that REV3L might act as a PS risk factor. Adding another layer to REV3L’s significance, a child, without classical MBS but exhibiting developmental delay, was identified with a rare homozygous missense REV3L variant with residual protein function[34]. Given that developmental delay often coexists with MBS, this discovery emphasises the intertwined nature of these conditions.
Given the association of REV3L with intersecting phenotypes, a model emerges: abnormal REV3L function during early embryonic development could lead to stochastic cell loss due to unrepaired DNA damage. This could result in a spectrum of overlapping neurodevelopmental disorders. Moreover, given the hypothesis that vascular disruptions are a primary cause of MBS, it is also possible that abnormal REV3L function increases neuronal cell sensitivity to ischemic events during early development due to its role in maintaining mitochondrial genome stability. Of note, unpublished work (Lylcheva-Bennett et al., personal communication, unpublished work 2023) has identified two unrelated patients in the 100,000 Genomes[35] cohort with phenotypic features of “typical MBS” (see Table 1) and likely pathogenic REV3L variants. Intriguingly, one of the patients showed evidence of mitochondrial depletion in the absence of a mitochondrial disorder (personal communication).
In summary, while REV3L was initially associated with Moebius Syndrome, its broader role in various overlapping neurodevelopmental disorders has become evident. Its central function in DNA repair and mitochondrial genome stability positions it as a cornerstone in understanding the genetic foundations of these conditions. Continued exploration of REV3L promises to offer invaluable insights, potentially paving the way for stratified therapeutic strategies for those affected.
PLXND1: another potential contributor to MBS
PLXND1 is another MBS candidate gene reported by Tomas-Roca et al. Through exome sequencing of MBS patients and their parents, de novo mutations in PLXND1 were identified in several patients[29]. The PLXND1 gene is a member of the plexin family that is expressed in various regions of the central nervous system, including the cranial and spinal ganglia and cortical plate. Plexins are known to bind to semaphorins, a large family of proteins that play a role in various developmental processes, including the guidance of axons and the migration of cells[29,36].
Notably, mice with mutations in Plxnd1 exhibited disruptions in neural pathways, particularly at the facial branchiomotor nucleus[29]. This disruption affected either the migration or proliferation of motoneurons. The facial nerve phenotype observed in these mutant mice mirrored the facial nerve weakness seen in MBS patients[29]. Furthermore, PLXND1’s involvement in neural migration was highlighted by the observation that deficiency in this gene affected the structures of neural fibres in the brain. In particular, there was consistent hypoplasia of certain brain structures that are essential for neural communication[29].
Another study by Glass et al. identified a PLXND1 variant in a child with Moebius syndrome and concurrent Poland anomaly[37]. Although the variant was inherited from an unaffected parent, the authors hypothesise that this may be the consequence of reduced penetrance or due to other variables modifying the phenotypic expression in genetically predisposed individuals.
Interestingly, although PLXND1 had previously been considered a candidate gene for Hereditary Congenital Facial Paresis (HCFP)[29], recent work by Tenney et al. demonstrated that HCFP is caused by noncoding variants affecting GATA2 expression[38]. While PLXND1 has been shown to play an important role in cardiac development, with biallelic variants in PLXND1 notably associated with congenital heart defects[39], its likely pleiotropic role remains to be fully elucidated.
Future genomic studies
The genetic landscape of Moebius Syndrome is as intricate as its clinical presentation. The heterogeneous clinical manifestations of MBS may represent the outcome of multiple distinct yet converging pathological processes. While significant progress has been made in deciphering its genetic aetiology, myriad questions remain. The inherent complexity of MBS suggests that disruptions in various developmental processes might converge on a shared outcome. Consequently, multiple genes, each involved in distinct pathways, might collectively contribute to MBS’s pathophysiology. Only a handful of patients with features of MBS have been found to have de novo mutations in REV3L and PLXND1 and these findings have so far not been replicated in other cohorts of MBS patients. It is possible that the interplay between genetic predispositions and environmental factors might render certain individuals more susceptible to the environmental insults that precipitate MBS. The potential involvement of multiple genes, each contributing to a shared clinical outcome, underscores the need for further genetic analyses.
Despite recent advances in genomic medicine, it is estimated that over 6,000 monogenic disorders remain unidentified[40]. Therefore, there is a clear need to target research efforts towards candidate disease gene discovery. One promising approach may be a reanalysis of existing genomic data from recent initiatives such as the 100,000 Genomes Project, which aims to revolutionise the diagnostic landscape for rare disorders. As the world’s most ambitious national sequencing initiative, it sought to sequence genomes from 85,000 patients, prioritising those with unresolved diagnostic needs[35].
However, the sheer volume of variants in each genome necessitated a focused approach. Analysis was limited to panels of known disease genes, based on the patient’s phenotype. This method, while efficient, had its drawbacks and is not suitable for the discovery of novel disease genes.
To delve deeper into MBS’s genetic landscape, we advocate for a meticulous review of the existing genomic and phenotypic data from projects such as the 100,000 Genomes Project. By scrutinising data from parent-offspring trios with phenotypic features of MBS, it may be possible to identify novel potential disease-causing variants. Participation of specialist centres with extensive MBS disease registries would further bolster such initiatives and other genomic studies. Upon identifying potential candidate genes, rigorous functional analyses and case series studies will be paramount. Collaboration with research partners to enable functional characterisation of any candidate genes/variants will further enhance such endeavours.
The analysis and reanalysis of genomic data, especially from initiatives like the 100,000 Genomes Project and diagnostic Whole Genome or Exome Sequencing, presents a golden opportunity to unravel the genetic intricacies of rare disorders, including MBS. Future efforts to elucidate the genetic determinants of MBS should ultimately yield transformative discoveries that can enhance diagnostic capabilities and patient care.
A MULTIDISCIPLINARY APPROACH TO THE MOEBIUS PATIENT
Each patient presents a unique clinical picture, with variations in the challenges they face. As we advance our understanding of the genomic landscape of MBS, we aspire to harness this knowledge for more precise prognostication. This will enable us to tailor interventions, ensuring that treatments are promptly initiated for those who stand to benefit the most.
The importance of early diagnosis
The importance of early diagnosis cannot be overstated, as it paves the way for timely multidisciplinary interventions and support[3]. Given the rarity of the condition, access to a specialist multidisciplinary team (MDT) familiar with MBS is crucial. While a specialist centre might not be near every patient, having a dedicated MDT can help coordinate care for patients locally, ensuring they receive the best possible support. Participation in collaborative rare disease networks, where applicable, can further improve patient experience.
Genetic considerations in MBS
As discussed above, the genetic basis of MBS is potentially extensive. Genetic evaluation plays a pivotal role in the management of MBS. The potential involvement of multiple genetic factors and their complex interplay with environmental determinants underscore the importance of not only ruling out alternative diagnoses but also establishing a comprehensive family pedigree and examining any pertinent features in the family history. Such evaluations are crucial in providing parents of affected children with accurate recurrence risks and offering them tailored counselling [Table 2]. All patients with suspected MBS and multiple congenital anomalies should be offered a chromosome microarray (CMA) as part of a genetic assessment. With the increased accessibility of Whole Genome Sequencing (WGS)[41], patients in some countries can now also be offered WGS as part of routine clinical care. In the UK, as well as access to WGS, affected families are also offered the opportunity to participate in research initiatives, such as the National Genomic Research Library, aiming to further our understanding of rare disorders, such as MBS.
Management strategies for Moebius syndrome (MBS)
Management area | Strategy | Outcome/Goal |
Genetic considerations | •Genetic evaluation •Family pedigree analysis •Whole Genome Sequencing (WGS) | •Rule out alternative diagnoses, recurrence risk assessment and tailored counselling, potential identification of causative genes |
Feeding and communication | •Evaluation of palate, tongue, and swallowing •Consideration of parenteral nutrition •Specialist speech and language input | •Optimal feeding strategies, effective speech development, and improved communication |
Respiratory concerns | •Early specialist input for airway protection •Management strategies for respiratory complications | •Prevention of respiratory complications and improved respiratory function |
Ophthalmological issues | •Early ophthalmological assessments •Botulinum toxin injections •Strabismus surgery | •Prevention of corneal exposure and visual loss; good alignment in primary position |
Facial and physical features | •Plastic surgery consultations and psychological support •Consider facial reanimation procedures •Assessment and management of any hand abnormalities | •Improved facial expression and social interaction •Improved hand function |
Development and behaviour | •Care under a community paediatrician •Integrated Clinical Psychology provision as part of specialist MDT | •Identify and address any developmental delays, motor coordination challenges, and behavioural difficulties |
Emotional and psychological | •Regular psychological screening and assessments •Psychological therapies and tailored interventions for patients and their families •Systemic working with healthcare and educational settings | •Normalise emotional responses, reduce psychological distress and promote resilience. •Tailored interventions to support decision-making and address feelings of isolation, anxiety, low self-esteem, and improve social wellbeing •Psychoeducation, promote awareness of (peer-) support organisations |
Education & specialist MDT | •Liaise with educators •Tailored learning plans •Physical adaptations in school environment | •Foster an inclusive and supportive learning environment to help achieve academic and social success |
Feeding and communication challenges
Feeding difficulties, commonly associated with MBS and present from birth, often lead to failure to thrive in affected infants. Neonates with MBS often cannot effectively achieve a suck and may have difficulties in coordinating their swallow, putting them at risk of aspiration and its consequences[42]. An early and expert evaluation of oral motor skills, tongue function, and palatal structure is essential, as is an early feeding assessment, assessing the safety of the swallow[3,39]. This ensures safe and optimal feeding strategies are in place and sets the foundation for effective feeding in the future.
Specialist speech and language input is also crucial to assess and treat the communication difficulties frequently seen in MBS[8,42] [Table 2]. These can vary from affected children developing normal language but presenting with articulatory difficulties related to their facial palsy to more complex children relying on nonverbal communication as speech is not achievable.
Respiratory concerns
Respiratory complications, including increased respiratory effort and feeding-related apnoea, have been reported in a significant number of MBS cases[8,42]. Early specialist input is essential for airway protection in affected neonates. Management strategies might encompass positioning, the use of airway adjuncts, and prolonged enteral feeding for those with respiratory complications [Table 2].
Ophthalmological interventions
Ophthalmological manifestations in MBS, such as abnormal ocular motility, necessitate early intervention[3]. The unopposed action of the medial rectus can lead to contracture, which can be mitigated with botulinum toxin injections[43]. These injections also facilitate subsequent strabismus surgery. Furthermore, relative weakness in the orbicularis oculi muscle can result in incomplete eye closure, potentially leading to corneal exposure and visual loss. Thus, early ophthalmological assessments are essential for corneal protection and timely diagnosis and correction of gaze palsies[8,16] [Table 2].
Addressing facial expression and physical abnormalities
The hallmark of MBS is the lack or impairment of facial expression, often leading to impaired social interaction and stigmatisation[44]. Often, a psychological approach to supporting young people and their families in managing their facial differences and lack of facial expression is employed with great success (A. O’Connor personal communication, 2023). For patients wishing to have surgical intervention, specialist plastic surgery involvement is key to addressing this challenge. Comprehensive pre-surgical assessment to support decision making and manage expectations is very important. Facial reanimation is technically challenging, and success is limited by the inherent absence of both facial nerve and facial musculature in MBS patients, so a single muscle is used to reconstruct the numerous muscles responsible for the subtle movements of the face[45]. In addition to facial reanimation, plastic surgery plays a pivotal role in the assessment and management of any hand abnormalities encountered in MBS [Table 2].
Developmental and behavioural aspects
While most MBS patients, and in particular those with Type 1 MBS, generally possess normal intelligence, the syndrome is often associated with developmental delay[5,42]. This includes motor delay due to hypotonia and poor coordination stemming from structural deficiencies observed in the brainstem of MBS patients, as well as structural defects impacting motor development. Speech delay, resulting from wider cranial nerve involvement, is also a common occurrence[42]. The increased prevalence of autistic features[46] and challenges in social communication are exacerbated by the reduced or absent facial expressions in affected individuals. Sleep disturbances, affecting approximately one-third of children with MBS, can be persistent and disruptive, sometimes continuing into adulthood[8]. Given the developmental, communication, and sleep challenges faced by children with MBS, it is recommended that most affected children be under the care of a community paediatrician.
Furthermore, the prevalence of psychological distress (e.g., anxiety, depression) is much higher in individuals with long-term health conditions compared to the general population[47]. Where significant behavioural difficulties arise, specialist psychological assessment and tailored support are indicated, highlighting the importance of integrated psychology provision within specialist MDTs [Table 2].
Emotional and psychological support
Beyond the physical and developmental challenges faced by individuals with MBS, the emotional and psychological toll on both patients and their families cannot be overlooked[3,14,19]. Psychosocial screening, comprehensive assessments, and tailored screenings are essential to address feelings of isolation, anxiety, and low self-esteem that may arise due to the condition. Families, too, benefit from support groups and counselling to better understand and cope with the challenges of raising a child with MBS.
Education and specialist MDT team
Education plays a pivotal role in the holistic management of MBS. It is essential that the affected child’s educational setting is well-informed about the condition, its implications, and the specific needs of the child. The specialist MDT team should liaise closely with educators to ensure that necessary accommodations are made in the school environment. This includes tailored learning plans, physical adaptations, and ensuring that peers and educators are sensitised to the condition, promoting an inclusive and supportive learning environment. Such collaborative efforts between healthcare professionals and educational institutions ensure that the child receives consistent support, both medically and academically, fostering an environment where they can thrive and reach their full potential.
The management of Moebius syndrome is a multifaceted endeavour that requires a holistic approach[8,14] [Table 2]. The emphasis on early diagnosis, multidisciplinary input, and access to specialist teams familiar with this rare condition is paramount for achieving the best outcomes for patients.
CONCLUSION
Moebius syndrome embodies many challenges within the varied landscape of neurodevelopmental disorders. This review provides an overview of the current understanding of MBS and highlights future approaches to further elucidate disease aetiology to enhance medical understanding and improve patient care.
Our current understanding of Moebius syndrome is that it is a consequence of multiple pathological processes that impact a common developmental pathway. Moebius remains a clinical diagnosis for a heterogeneous group of patients with a constellation of clinical features, where other conditions have been excluded. Despite the challenges associated with Moebius syndrome, advances in genetic research have led to the identification of several genes associated with the disorder, and ongoing research continues to shed light on the complex interplay between genetic and environmental factors in the development of Moebius syndrome. Careful genomic analysis, combined with deep phenotyping and standardised reporting of clinical diagnoses, will facilitate future research towards a better understanding of this enigmatic neurodevelopmental syndrome.
However, many questions remain. For example, why do some individuals with mutations in the same gene develop Moebius syndrome while others do not? What are the specific environmental factors that contribute to the development of the disorder, in addition to the known effects of certain drugs such as misoprostol and various vasoconstrictive agents? And how can we use this knowledge to develop effective treatments for Moebius syndrome?
Collaboration among researchers, clinicians, and stakeholders is paramount for advancing our understanding of Moebius syndrome. By pooling expertise and resources, there is potential to accelerate the pace of discovery and innovation. While answering the intricate questions surrounding the syndrome will necessitate further research, the insights garnered will deepen our comprehension of Moebius syndrome and delineate the relationships between genotypic variations and phenotypic manifestations. This will set the foundation for individualised management plans and strengthen clinicians’ capacity for accurate diagnosis, prognostication, targeted screening, and optimisation of patient and familial outcomes. Ultimately, with such collaborative efforts, the lives of those affected by Moebius syndrome stand to be significantly transformed, underscoring the importance of this collective goal.
DECLARATIONS
Authors’ contributions
Conceptualisation, writing original draft: Lyulcheva-Bennett E
Writing, review, and editing: All authors
Visualisation: Lyulcheva-Bennett E, Bennett D
Availability of data and materials
Not applicable.
Financial support and sponsorship
None.
Conflicts of interest
All authors declared that there are no conflicts of interest.
Ethical approval and consent to participate
Not applicable.
Consent for publication
Not applicable.
Copyright
© The Author(s) 2023.
REFERENCES
1. Graefe A. Diagnostik der Augenmuskellähmungen and Aetiologie und Pathogenese der Augenmuskellähmungen. In: Carl Ferdinand von Arlt, editor. Handbuch der gesammten Augenheilkunde. Leipzig: Verlag Von Wilhelm Engelmann; 1880. pp. 60-7.
2. Mobius PJ. Ueber angeborene doppelseitige Abducens-Facialis-Lahmung. Munch Med Wochenschr 1888;35:91-4. Available from: https://www.europeana.eu/de/item/03924/_0011_oai_biusante_parisdescartes_fr_medica_epo0687 [Last accessed on 14 Dec 2023].
3. Picciolini O, Porro M, Cattaneo E, et al. Moebius syndrome: clinical features, diagnosis, management and early intervention. Ital J Pediatr 2016;42:56.
4. Kadakia S, Helman SN, Schwedhelm T, Saman M, Azizzadeh B. Examining the genetics of congenital facial paralysis - a closer look at Moebius syndrome. Oral Maxillofac Surg 2015;19:109-16.
5. Bell C, Nevitt S, McKay VH, Fattah AY. Will the real Moebius syndrome please stand up? A systematic review of the literature and statistical cluster analysis of clinical features. Am J Med Genet A 2019;179:257-65.
7. Zaidi SMH, Syed IN, Tahir U, Noor T, Choudhry MS. Moebius syndrome: what we know so far. Cureus 2023;15:e35187.
8. McKay VH, Touil LL, Jenkins D, Fattah AY. Managing the child with a diagnosis of Moebius syndrome: more than meets the eye. Arch Dis Child 2016;101:843-6.
9. Renault F, Flores-guevara R, Sergent B, et al. Pathogenesis of cranial neuropathies in Moebius syndrome: electrodiagnostic orofacial studies. Muscle Nerve 2018;58:79-83.
11. Vicente AM, Ballensiefen W, Jönsson JI. How personalised medicine will transform healthcare by 2030: the ICPerMed vision. J Transl Med 2020;18:180.
12. MacKinnon S, Oystreck DT, Andrews C, Chan WM, Hunter DG, Engle EC. Diagnostic distinctions and genetic analysis of patients diagnosed with moebius syndrome. Ophthalmology 2014;121:1461-8.
13. Terzis JK, Noah EM. Möbius and Möbius-like patients: etiology, diagnosis, and treatment options. Clin Plast Surg 2002;29:497-514.
14. Pedersen LK, Maimburg RD, Hertz JM, et al. Moebius sequence - a multidisciplinary clinical approach. Orphanet J Rare Dis 2017;12:4.
15. Sadeghi N, Hutchinson E, Van Ryzin C, et al. Moebius Syndrome Research Consortium. Brain phenotyping in Moebius syndrome and other congenital facial weakness disorders by diffusion MRI morphometry. Brain Commun 2020;2:fcaa014.
16. Verzijl HT, van der Zwaag B, Cruysberg JR, Padberg GW. Möbius syndrome redefined: a syndrome of rhombencephalic maldevelopment. Neurology 2003;61:327-33.
17. Raroque HG Jr, Hershewe GL, Snyder RD. Möbius syndrome and transposition of the great vessels. Neurology 1988;38:1894-5.
18. Herrera DA, Ruge NO, Florez MM, Vargas SA, Ochoa-Escudero M, Castillo M. Neuroimaging findings in moebius sequence. AJNR Am J Neuroradiol 2019;40:862-5.
20. Whitman MC, Jurgens JA, Hunter DG, Engle EC. Congenital fibrosis of the extraocular muscles overview. In: Adam MP, Feldman J, Mirzaa GM, Pagon RA, Wallace SE, Bean LJH, et al., editors. GeneReviews®. Seattle: Seattle (WA); 1993.
21. Webb BD, Frempong T, Naidich TP, Gaspar H, Jabs EW, Rucker JC. Mirror movements identified in patients with moebius syndrome. Tremor Other Hyperkinet Mov 2014;4:256.
22. Jissendi-Tchofo P, Severino M, Nguema-Edzang B, Toure C, Soto Ares G, Barkovich AJ. Update on neuroimaging phenotypes of mid-hindbrain malformations. Neuroradiology 2015;57:113-38.
23. Marques-Dias MJ, Gonzalez CH, Rosemberg S. Möbius sequence in children exposed in utero to misoprostol: neuropathological study of three cases. Birth Defects Res A Clin Mol Teratol 2003;67:1002-7.
24. Sarnat HB. Watershed infarcts in the fetal and neonatal brainstem. An aetiology of central hypoventilation, dysphagia, Möibius syndrome and micrognathia. Eur J Paediatr Neurol 2004;8:71-87.
25. Puvabanditsin S, Garrow E, Augustin G, Titapiwatanakul R, Kuniyoshi KM. Poland-Möbius syndrome and cocaine abuse: a relook at vascular etiology. Pediatr Neurol 2005;32:285-7.
26. Auffret M, Bernard-Phalippon N, Dekemp J, et al. Misoprostol exposure during the first trimester of pregnancy: is the malformation risk varying depending on the indication? Eur J Obstet Gynecol Reprod Biol 2016;207:188-92.
27. Vauzelle C, Beghin D, Cournot MP, Elefant E. Birth defects after exposure to misoprostol in the first trimester of pregnancy: prospective follow-up study. Reprod Toxicol 2013;36:98-103.
28. Terzis JK, Anesti K. Developmental facial paralysis: a review. J Plast Reconstr Aesthet Surg 2011;64:1318-33.
29. Tomas-Roca L, Tsaalbi-Shtylik A, Jansen JG, et al. De novo mutations in PLXND1 and REV3L cause Möbius syndrome. Nat Commun 2015;6:7199.
30. Lin W, Wu X, Wang Z. A full-length cDNA of hREV3 is predicted to encode DNA polymerase zeta for damage-induced mutagenesis in humans. Mutat Res 1999;433:89-98.
31. Van Sloun PP, Varlet I, Sonneveld E, et al. Involvement of mouse Rev3 in tolerance of endogenous and exogenous DNA damage. Mol Cell Biol 2002;22:2159-69.
32. Singh B, Li X, Owens KM, Vanniarajan A, Liang P, Singh KK. Human REV3 DNA polymerase zeta localizes to mitochondria and protects the mitochondrial genome. PLoS One 2015;10:e0140409.
33. Vaccari CM, Tassano E, Torre M, et al. Assessment of copy number variations in 120 patients with Poland syndrome. BMC Med Genet 2016;17:89.
34. Halas A, Fijak-Moskal J, Kuberska R, et al. Developmental delay with hypotrophy associated with homozygous functionally relevant REV3L variant. J Mol Med 2021;99:415-23.
35. Smedley D, Smith KR, Martin A, et al. 100,000 Genomes Project Pilot Investigators. 100,000 genomes pilot on rare-disease diagnosis in health care - preliminary report. N Engl J Med 2021;385:1868-80.
36. van der Zwaag B, Hellemons AJ, Leenders WP, et al. PLEXIN-D1, a novel plexin family member, is expressed in vascular endothelium and the central nervous system during mouse embryogenesis. Dev Dyn 2002;225:336-43.
37. Glass GE, Mohammedali S, Sivakumar B, et al. Poland-Möbius syndrome: a case report implicating a novel mutation of the PLXND1 gene and literature review. BMC Pediatr 2022;22:745.
38. Tenney AP, Di Gioia SA, Webb BD, et al. Noncoding variants alter GATA2 expression in rhombomere 4 motor neurons and cause dominant hereditary congenital facial paresis. Nat Genet 2023;55:1149-63.
39. Guimier A, de Pontual L, Braddock SR, et al. Biallelic alterations in PLXND1 cause common arterial trunk and other cardiac malformations in humans. Hum Mol Genet 2023;32:353-6.
40. Seaby EG, Rehm HL, O’Donnell-Luria A. Strategies to uplift novel mendelian gene discovery for improved clinical outcomes. Front Genet 2021;12:674295.
41. Stark Z, Dolman L, Manolio TA, et al. Integrating genomics into healthcare: a global responsibility. Am J Hum Genet 2019;104:13-20.
42. Renault F, Flores-Guevara R, Baudon JJ, et al. Orofacial motor dysfunction in Moebius syndrome. Dev Med Child Neurol 2020;62:521-7.
43. Bains RD, Elbaz U, Zuker RM, Ali A, Borschel GH. Corneal neurotization from the supratrochlear nerve with sural nerve grafts: a minimally invasive approach. Plast Reconstr Surg 2015;135:397e-400e.
44. Bogart KR. “People are all about appearances”: a focus group of teenagers with Moebius syndrome. J Health Psychol 2015;20:1579-88.
45. Fattah A, Borschel GH, Manktelow RT, Bezuhly M, Zuker RM. Facial palsy and reconstruction. Plast Reconstr Surg 2012;129:340e-52e.
46. Johansson M, Wentz E, Fernell E, Strömland K, Miller MT, Gillberg C. Autistic spectrum disorders in Möbius sequence: a comprehensive study of 25 individuals. Develop Med Child Neuro 2001;43:338-45.
Cite This Article
Export citation file: BibTeX | EndNote | RIS
OAE Style
Lyulcheva-Bennett E, Blumenow W, O’Connor A, Kelly M, Bennett D, Fattah A. Towards an understanding of the aetiology, genomic landscape and management of Moebius syndrome. J Transl Genet Genom 2023;7:259-73. http://dx.doi.org/10.20517/jtgg.2023.33
AMA Style
Lyulcheva-Bennett E, Blumenow W, O’Connor A, Kelly M, Bennett D, Fattah A. Towards an understanding of the aetiology, genomic landscape and management of Moebius syndrome. Journal of Translational Genetics and Genomics. 2023; 7(4): 259-73. http://dx.doi.org/10.20517/jtgg.2023.33
Chicago/Turabian Style
Ekaterina Lyulcheva-Bennett, Wendy Blumenow, Adelene O’Connor, Maria Kelly, Daimark Bennett, Adel Fattah. 2023. "Towards an understanding of the aetiology, genomic landscape and management of Moebius syndrome" Journal of Translational Genetics and Genomics. 7, no.4: 259-73. http://dx.doi.org/10.20517/jtgg.2023.33
ACS Style
Lyulcheva-Bennett, E.; Blumenow W.; O’Connor A.; Kelly M.; Bennett D.; Fattah A. Towards an understanding of the aetiology, genomic landscape and management of Moebius syndrome. J. Transl. Genet. Genom. 2023, 7, 259-73. http://dx.doi.org/10.20517/jtgg.2023.33
About This Article
Special Issue
Copyright
Data & Comments
Data
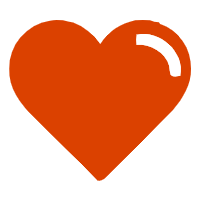
Comments
Comments must be written in English. Spam, offensive content, impersonation, and private information will not be permitted. If any comment is reported and identified as inappropriate content by OAE staff, the comment will be removed without notice. If you have any queries or need any help, please contact us at support@oaepublish.com.