Inborn errors of immunity present with neuropsychiatric symptoms overlapping with autistic behavioral symptoms
Abstract
Autism spectrum disorder (ASD) is a behaviorally defined syndrome affected by multiple genetic and environmental factors. A wide variety of risk factors for ASD have been identified and many of these affect immune functions. This may not be surprising, since the immune system and the nervous system share common signaling mechanisms and affect each other as a part of the neuroimmune network. The ever-expanding scope of inborn errors of immunity (IEIs) has revealed multiple pathogenic gene variants that manifest overlapping clinical features of common neuropsychiatric diseases, including ASD. These IEIs often cause dysregulated immune activation and resultant chronic inflammation affecting multiple organs. Some IEIs also cause changes in morphogenesis and plasticity of the central nervous system. Such patients often present with a puzzling array of clinical features and some of them may be diagnosed with ASD or other neuropsychiatric conditions. The progress of our understanding of disease mechanisms for IEIs at the molecular levels has led to gene-specific treatment measures in some diseases. In addition, some ASD patients are found to have laboratory findings of neuroinflammation that resemble those seen in IEI patients. This may pave the way for applying specific treatment measures used for IEI patients in such ASD patients. This review focuses on describing IEIs that have overlapping features of ASD. Emphasis is also on IEIs that can be treated by targeting identified disease mechanisms. Such information may be helpful for clinicians who are considering genetic/metabolic workup in ASD patients.
Keywords
INTRODUCTION
Various genetic analysis has indicated that there are multiple ASD risk variants. These variants are reported in genes associated with both brain morphogenesis and neuronal plasticity affecting the signaling pathways, including chromatin remodeling, and Wnt/Notch pathways[1]. Genes involving regulating oxidative stress and the associated metabolic pathways also affect neuronal growth. Therefore, variants of these genes are implicated in ASD pathogenesis as well[1]. Recent studies of gene-protein interactions and other regulatory factors of transcription and translation, like microRNA (miRNA), are also implicated in the epigenetic basis of ASD development[2]. In such changes, the immune system is thought to play a crucial role in the onset and progress of ASD, partly through the generation of immune-mediated neuroinflammation[2]. The role of neuroinflammation in ASD pathogenesis is best documented in the animal model of ASD called maternal immune activation (MIA), in which maternal sterile inflammation induced by injection of endotoxin during the 2nd trimester has been shown to cause impaired neuropsychiatric development in offspring[3,4].
Candidate genes for ASD risk are often those involved in the regulation of gene expression in epigenetic pathways[2]. Likewise, environmental factors that affect epigenetic regulation are also implicated in ASD risk. For example, valproate, an anti-seizure medication and a well-known risk factor of ASD through fetal exposure, is known to affect a histone modification enzyme and folic acid metabolism[2,5]. The resultant epigenetic changes last for a prolonged time, as shown in animal models of MIA[6]. In the MIA model, maternal inflammation was triggered by endotoxin, causing sterile inflammation. Therefore, lasting effects on the immune system and the brain are thought to be mediated by its effects on innate immunity. Apart from the study of ASD pathogenesis, lasting changes in innate immunity following potent immune stimuli are known to involve epigenetic changes and are often referred to as innate immune memory[7,8]. In summary, there may be underlying mechanisms involving gene variants that may cause immune activation and resultant chronic inflammation in some ASD subjects, manifesting multi-organ phenotypes. In that regard, research on IEIs provides valuable information.
The study of IEIs has revealed a genetic basis for autoinflammatory and immunodysregulatory conditions, which often involve the central nervous system (CNS): this is partly due to the onset of inflammation during the early stage of brain development[9]. Mechanisms of neuroinflammation associated with IEIs often involve dysregulated innate immune responses. Understanding such mechanisms has shed light on the role of neuroinflammation in the pathogenesis of common neuropsychiatric conditions, including ASD. In this review, IEIs that could present with ASD-like behavioral symptoms will be summarized along with currently available treatment measures for each IEI condition. Such information may be helpful when considering exploring advanced genetic testing for ASD subjects. In addition, treatment measures used for specific IEI may be applicable for ASD subjects if they carry gene variants that can affect their clinical manifestations.
IEI ASSOCIATED WITH NEURODEVELOPMENTAL CONDITIONS
IEI causing thymic defect and multiple pathways associated with the neuroimmune network
Syndromes that affect the morphogenesis of multiple organs and chromatin remodeling often present with a puzzling array of clinical features. Both the immune and nervous systems are frequently affected. In addition, these two systems have an intricate network of cells that are essential for both morphogenesis and organ functions, by utilizing overlapping mechanisms of actions. In this section, we describe well-recognized syndromes that could develop ASD-like clinical features and could be initially diagnosed with ASD as a primary diagnosis. Since these patients are vulnerable to recurrent infection and
CATCH-22 syndrome
In 1993, based on studies of 44 subjects with the above-described clinical features, Wilson et al. proposed the acronym of CATCH-22 (Cardiac disease, Abnormal facies, Thymic hypoplasia, Cleft palate, and Hypocalcemia resulting from 22q11 deletions)[10]. Prior to our understanding of the mechanisms of 22q11.2 microdeletion and CHARGE syndrome, these two conditions with overlapping clinical features were included in CATCH-22 syndrome. These two syndromes often present with overlapping neuropsychiatric features of ASD and will be discussed in this section.
(1) 22q11.2 microdeletion syndrome
The frequency of DiGeorge syndrome (DGS) is reported to be 1 in 3,000 to 1 in 6,000, making DGS one of the most common chromosomal microdeletion syndromes[11]. However, its clinical manifestations are markedly variable, affected by a range of genetic and environmental modifiers[12]. The degree of thymic hypoplasia varies considerably from aplasia of a thymus, which presents as the complete absence of T cells, to partial or almost intact thymus. The only curative measure for complete DGS is thymic transplant[11]. Partial defect of the thymus may be compensated by oligoclonal expansion of T cells in the later years, as shown in the improvement in responses to vaccinations with age. However, oligoclonal expansion is associated with a decrease in T cell diversity that may increase the risk of autoimmune complications[11]. Some DGS patients with partial thymic hypoplasia may present with predominant features of developmental delay and neurocognitive and psychiatric disorders, making DGS diagnosis challenging to clinicians. We have experienced a few DGS patients who were initially diagnosed with ASD and later found to have the 22q11.2 microdeletion through chromosome microarray analysis. 22q11.2 region contains a cluster of low copy repeats (LCRs) that mediate meiotic non-allelic homologous recombination between LCRs and are susceptible to deletion or duplication[12]. This region also codes long non-coding RNAs (lncRNAs) and miRNAs that affect gene expression[12]. Which part of this region is deleted also affects the clinical features of DGS[12]. For example, deletion of TBX1, which is essential for normal thymus formation, leads to thymic aplasia or hypoplasia, greatly affecting T cell immunity, but not all DGS patients have deletion of TBX1[11,12]. Low T cell numbers may be detected by newborn screening (NBS) of T lymphopenia. However, many DGS patients with mild to moderate T lymphopenia will be missed. When thymus formation is impaired but not completely absent, T cell tolerance is affected partly due to decreased expression of AIRE (autoimmune regulator), and reduced generation of central regulatory T cells[13]. In older patients, the development of autoimmune complications may also cause puzzling clinical features. Apart from the TBX1 gene, this 22q11.2 region contains genes coding proteins that affect mitochondrial metabolism, such as DGCR8 and TXNRD2[14]. Deletion of these genes leads to an increase in the production of reactive oxygen species (ROS), further affecting immune functions and neuronal development. Deletion of these genes is thought to contribute to impaired morphogenesis of the CNS and neuronal functioning in DGS patients[14,15]. The deleted lesion of 22q11.2 also contains genes implicated with the pathogenesis of schizophrenia or other neurodevelopmental conditions. For example, COMT hemizygosity and the resultant reduction of enzymatic activity of COMT (catechol-o-methyltransferase) are implicated with psychosis risk in 22q11.2 deletion syndrome[16] and ASD-like psychiatric phenotypes[17]. It was proposed that the 22q11.2 microdeletion syndrome is a genetic model of neurodevelopmental phenotypes[15]. The 22q11.2 microdeletion is easily detectable with the use of chromosomal microarray analysis; thus, clinicians seeing ASD subjects must keep this syndrome in the differential diagnoses when ASD subjects present with clinical symptoms indicating DGS.
(2) CHARGE syndrome
Impaired regulation of gene expression through chromatin remodeling affects numerous genes, resulting in variable clinical manifestations involving multiple organs. CHARGE (coloboma, heart defect, atresia choanae, retardation of growth and development, genital hypoplasia, and ear anomalies/deafness) syndrome is one of such syndromes occurring 1:15,000 newborns and characterized by overlapping features of 22q11.2 microdeletion syndrome[11]. Autosomal dominant pathogenic variants of CHD7 coding for the chromodomain helicase DNA binding domain protein 7 (CHD7) cause this syndrome in up to 70% of patients diagnosed with CHARGE syndrome[11]. Effects of CHD7 on gene expression during neural crest development may cause overlapping features of 22q11.2 microdeletion, including impaired thymic development[18]. Patients with CHARGE syndrome often initially present with marked hypotonia and resultant severe gastroesophageal reflux disease (GERD) accompanied by impaired growth[11,19,20]. They often require gastric tube feeding and Nissen fundoplication for controlling GERD. Developmental delay is also highly prevalent, along with ASD-like behavioral symptoms in patients with CHARGE syndrome[21,22]. Therefore, they are frequently diagnosed with ASD[21]. Secondary to thymic hypoplasia, T cell anomalies resembling those seen in DGS patients are also found at high frequency in CHARGE syndrome patients[23]. Clinicians are generally aware of feeding difficulty and developmental delay/behavioral symptoms in patients with CHARGE syndrome. Aspects of possible immunodeficiency in CHARGE syndrome may be overlooked. However, impaired T cell immunity and subsequent recurrent infection will further aggravate behavioral symptoms in patients with CHARGE syndrome. An increase in autoimmune complications, as seen in DGS patients, will also make clinical management challenging. Addressing a component of impaired T cell immunity in CHARGE syndrome patients will be important for the best clinical outcomes.
Down syndrome (trisomy 21)
Down syndrome (DS) is the most common chromosomal abnormality, occurring in approximately 1:700 live births[24]. DS patients are well known for their characteristic dysmorphism, developmental delay, and other complications affecting multiple organs. DS patients present with ASD-like behavioral symptoms; diagnosis of ASD in DS patients is reported to be 16%-41%[25]. DS patients are also characterized by abnormal thymic epithelium development, resulting in progressive thymic hypoplasia with age[13], and further thymic abnormalities found in DGS patients. Their clinical features and progressive decline in immune functions are mostly attributed to the presence of the 3rd chromosome 21. Excessive genes due to trisomy 21 are associated with impaired regulation of redox state and a resultant increase in oxidative stress, subsequently affecting the development of multiple organs[26]. Such changes also contribute to premature senescence and increased oxidative stress in thymus and other lymphoid organs, worsening progressively with age[26,27]. As a result, in DS patients, impaired T cell immunity gets worse with age, often manifested as a decline in antibody titers generated by childhood vaccinations: in infants or young DS children, DS patients generally exhibit normal lymphocyte count and reveal decent responses to infant vaccines, but such responses progressively get worse with age in some DS patients[27,28]. ikewise, cognitive functions may decline with age in DS patients, indicating their need for more supportive measures for oxidative stress[14].
Kabuki syndrome
Kabuki syndrome (KS) is another complex syndrome with overlapping features of CATCH-22 syndrome, occurring approximately at 1 in 32,000[31]. It is characterized by facial dysmorphism resembling kabuki makeup, psychomotor developmental delay, growth retardation, and other highly variable clinical features[32]. KS was first found to be associated with pathogenic variants of KMT2D, which codes histone lysine methyltransferase that regulates chromatin remodeling and subsequently affects the expression of numerous genes[32]. Up to 75% of KS patients express KMT2D gene variants, while about 5% of KS patients reveal variants of KDM6A, which codes histone demethylase[32,33]. In addition to variable developmental issues, KS patients manifest difficult behavioral symptoms with overlapping features of ASD[32,33]. In addition, KS patients often present with functional neurological abnormalities including muscular hypotonia, and dysarthria[33]. Partial seizures are also found at high frequency (around 17%) in KS patients[34]. KS patients are also characterized by immunodeficiency (usually antibody deficiency) and autoimmune complications. Hypogammaglobulinemia is reported to be seen in up to 58% of KS patients and may become more apparent with age, as seen in patients with common variable immunodeficiency (CVID) and so are autoimmune complications[33]. Neuropsychiatric symptoms overlapping features of ASD may lead to a diagnosis of ASD in KS patients, despite characteristic facial dysmorphism and other clinical features. In our clinic, we encountered two KS patients who were originally diagnosed with ASD, but other clinical manifestations led to Dx of KS with identified pathogenic variants of KMT2D. In both patients, recurrent infection was a major trigger of worsening behavioral symptoms and seizure cluster: starting supplemental Ig treatment dramatically improved recurrent respiratory infection and subsequent seizure controls. Thus, clinicians are encouraged to be familiar with characteristic facial dysmorphism of KS, since genetic diagnosis is relatively easy through target gene sequencing offered by commercial laboratories.
The syndromes described above often develop impaired humoral immunity over time, as typically seen in DS patients. Because of thymic hypoplasia, these patients may not show hypogammaglobulinemia[9], but specific antibody production may become more apparent with age, as typically shown by loss of response to vaccine antigens, especially less immunocompetent polysaccharide antigens: PPV23 was typically used for assessing such responses[35]. Anti-microbial therapy is important for the above-described syndrome, given impaired T cell immunity. Typically, prophylaxis antibiosis is aimed to cover intracellular organisms that are not well covered by supplemental immunoglobulin (Ig) treatment. Trimethoprim-sulfamethoxazole (TMP-SMX) has been typically used for patients with impaired T cell immunity to cover PJP (pneumocystis jirovecii pneumonia) and other intracellular organisms in patients with predominant T cell defects[36]. Partial DGS patients may only require TMP-SMX prophylaxis for the 1st few of life, while other patients described in this section may continue to require such prophylaxis measures. Over time, many of these patients with the above-described syndromes, especially KS patients, require supplemental Ig treatment[37]. When these patients also suffer from difficult behavioral symptoms, it may be challenging to administer Ig intravenously. It is our experience that many patients with underlying primary immunodeficiency have difficulty in tolerating a high dose of Ig for presumed autoimmune conditions, which may be attributed to prior antigen load. In our clinic, one KS patient had a severe adverse reaction to high-dose (HD) intravenous immunoglobulin (IVIg) administered by other providers who initially diagnosed the individual with autoimmune diseases before confirming the KS diagnosis. This patient tolerates supplemental Ig administered subcutaneously with improvement in recurrent respiratory infection and infection-induced seizure clusters. The dose and route of administration may require consideration in these patients.
IEIs causing autoinflammatory conditions affecting the brain
Recent progress in clinical and molecular immunology has revealed that there are many genetic variants causing monogenic autoinflammatory diseases. Primary autoinflammatory diseases are characterized by dysregulated innate immune responses caused by variants of genes associated with the regulation of non-specific inflammatory responses exerted by innate immunity as the 1st line of immune defense. Since such genes are also often closely associated with morphogenesis and plasticity of the nervous system[38], it is not surprising that patients with monogenic autoinflammatory diseases often exhibit neuropsychiatric symptoms and developmental delay. It is beyond the scope of this review to describe each group of monogenic autoinflammatory diseases. Therefore, in this review, we describe representative autoinflammatory diseases that are relatively common and often present with predominant neuropsychiatric symptoms that may mimic ASD behavioral symptoms or, if they co-exist, will be challenging for clinical management.
Variants of genes regulating activation of inflammasomes
In this category, we will mainly discuss autoinflammatory diseases caused by MEFV variants that cause familial Mediterranean fever (FMF) and NLRP3 variants that cause cryopyrinopathies.
(1) FMF: FMF is one of the most common systemic autoinflammatory diseases and its gene (MEFV) was initially cloned in 1997[39]. FMF affects populations of Mediterranean descent and its prevalence is reported to be 1:500 to 1:1,000 in endemic countries[40]. It was known to cause intermittent fever with serositis and subsequent progressive amyloidosis, provided sterile inflammation is not managed properly[41]. Self-limited fever attacks are thought to be a hallmark of FMF. However, it became more evident that some FMF patients with pathogenic MEFV gene variants do not present with classical fever attacks and can present with clinical features mimicking vasculitis and Behçet disease[42]. An atypical manifestation of FMF is now well recognized and effects of genotypes and epigenetic regulations are implicated in its variable clinical manifestations[43]. One case report describes two heterozygous mutations of FMF in a high-functioning ASD subject, and these genes may be contributing to muscle rigidity and other symptoms of the musculoskeletal system in this patient[44]. The pathogenesis of FMF is attributed to a gain of function (GOF) variant of MEFV, which causes persistent activation of pyrin. Severe phenotypes are associated with autosomal recessive (AR) homozygous MEFV variants and/or compound heterozygous AR mutations of MEFV[45]. Autosomal dominant (AD) GOF MEFV variants manifest more distinct clinical phenotypes[46]. It is now recognized that the presence of pathogenic variants of the MEFV genes aggravates autoimmune conditions[47]. We have encountered one patient who was diagnosed with autoimmune encephalitis with the presence of ovarian teratoma, but this patient’s neuropsychiatric symptoms appeared to be affected by severe musculoskeletal (MSK) pain symptoms. This patient was later found to have an AD, GOF MEFV pathogenic variant: her MSK pain was brought under control with daily intake of colchicine and resultant improvement of her neuropsychiatric symptoms.
(2) Cryopyrinopathyies: Activation of the NLRP3 inflammasome is triggered by signaling from non-specific triggers of innate immunity, including danger-associated molecular patterns (DAMPS) and pathogen-associated molecular patterns (PAMPS). These stimuli activate NLRP3 through the NF-kB signaling pathway, resulting in the up-regulation of IL-1ß (interleukin-1ß) transcription, called priming, and subsequent NLRP3 activation follows through multiple mechanisms[45]. Activation of NLRP3 results in the release of oxidized mitochondrial DNA that can then directly activate NLRP3[48]. AD, GOF mutations in NLRP3 are known to manifest variable degrees of symptoms, called cryopyrin-associated periodic fever syndrome (CAPS). CAPS is considered to be rare, occurring 1 to 3 per 1 million[49], but patients with milder phenotypes may be present at a higher frequency. Familial cold autoinflammatory syndrome (FACS) is manifested as intermittent delayed onset cold induced urticaria, often with fever and joint ache, and patients with Muckle-Wells syndrome (MWS) present with urticaria and sensory hearing loss[41]. Neonatal-onset multisystem inflammatory disease (NOMID) is characterized by an early onset of symptoms dominated by neurological manifestations resembling serous meningitis[41,45]. Interestingly, patients with multiple sclerosis (MS) are reported to have pathogenic, low-penetrance variants of MEFV and NLRP3 genes at a high frequency[50].
Variants of NLRP and NLRC4 inflammasomes are also known to cause similar autoinflammatory diseases. As seen in NOMID patients, severely affected patients with cryopyrinopathies are expected to develop significant neurological symptoms mimicking serous meningitis that may result in significant developmental delay and seizure disorders. Some patients with cryopyrinopathies develop behavioral symptoms that overlap with ASD behavioral symptoms in our experience but respond dramatically to treatment measures for cryopyrinopathies.
(3) Inflammasome blockers: The above-described pyrin and NLRP3-inflammatory disorders have been treated with blockers of inflammasome activation with favorable clinical responses. Such treatment measures may be applicable for ASD subjects in whom variants of these genes are found to have a role in the regulation of underlying neuroinflammation. For FMF patients, colchicine has been the 1st line of treatment for the past few decades. Colchicine inhibits the polymerization of microtubules, which is crucial for chemotaxis and phagocytosis of innate immune cells[51,52]. Colchicine also inhibits NLRP3, subsequently blocking IL-1ß induced inflammasome activation, and production of TNF-α (tumor necrosis factor-α) and IL-6[53,54]. Actions of colchicine on microtubes also inhibit neutrophil-platelet interactions, preventing thrombosis triggered by neutrophilic inflammation[54,55]. These actions of colchicine indicate that colchicine will be effective for controlling neutrophilic inflammation triggered by Th17 cells and/or other innate immune cells. However, colchicine is a strong inhibitor of P450 3A4; thus, it will be necessary to evaluate drug interactions with medications that patients are already taking. As for CAPS patients, IL-1 blockers are the 1st line of treatment measures[45]. Humanized monoclonal antibodies against IL-1ß (canakinumab) or soluble IL-1ß receptor conjugated with the Fc portion of human IgG1 (rilonacept) have been used for long-acting IL-1ß blockers[56]. In contrast, anakinra, a IL-1 receptor antagonist (IL-1ra), is a recombinant protein of naturally produced human IL-1ra that acts as a counter-regulatory mediator for controlling IL-1-induced inflammation, blocking actions of both Il-1ß and IL-1[57]. Anakinra is short-acting, requiring daily subcutaneous injection. However, it can penetrate intact blood-brain barriers (BBB): this unique property of anakinra may be handy when it is required to control acute cytokine storms or CNS inflammation associated with seizures. We have reported one case of treatment-resistant seizures and the favorable effects of anakinra when used with a mTOR (mammalian target of rapamycin) blocker[58]. Recent results of randomized clinical trials of anakinra indicated attenuation of severe COVID-19 (coronavirus disease 2019) in hospitalized patients[59,60], although a recent metanalysis reported negative results of anakinra for severe COVID-19[61]. It is also of note that CAPS patients are expected to suffer from more potent adjuvant effects from vaccinations, but such effects are well attenuated with concurrent use of IL-1ß blockers[45].
As summarized, dysregulated activation of inflammasome pathways can present with a puzzling array of clinical presentations, often including neuropsychiatric symptoms. Clinicians may need to pay attention to possible genetic risk factors associated with inflammasome disorders in some ASD subjects if other clinical features indicate autoinflammatory conditions, since blockers targeting inflammasome activation are readily available and have good safety records[45].
Type 1 interferonopathies
Interferons (IFNs) are crucial for innate immune defense, interfering with viral replication and dissemination. Any cells can produce IFNs upon the invasion of microbes. However, some innate immune cells, such as plasmacytoid dendritic cells, produce large amounts of type 1 and type 3 IFNs, while type 2 IFN (IFN-γ) is mainly produced by type 1 T-helper (Th1) cells[62]. IFNs are potent activators of macrophage and monocyte lineage cells, and dysregulated signaling through IFNs can lead to macrophage activation syndrome, a potentially lethal condition affecting multiple organs[62]. The COVID-19 pandemic showed what a crucial role that IFNs can play. It is now known that severe COVID-19 disease in elderly subjects is partly attributed to the production of autoantibodies against type 1 IFNs[63]. On the other hand, uncontrolled production of type 1 IFNs or dysregulated signaling via IFNs in innate immune cells leads to autoinflammatory conditions, now referred to as type 1 interferonopathies[64].
(1) Clinical presentation: Various variants of multiple genes are now recognized to cause overproduction of IFNs or dysregulated activation of type 1 IFN signaling pathways[65]. This leads to uncontrolled activation of the downstream innate immune signaling pathways, polyclonal B cell activation, and subsequent autoinflammatory and autoimmune conditions[66]. One of the striking features of interferonopathies is neuroinflammation. Aicardi-Goutières syndrome, the prototype of interferonopathies, is known to present with symptoms caused by severe neuroinflammation in early infancy, resembling clinical features of congenital infectious encephalopathy and subsequent progressive loss of cognitive functioning[64]. Neuroinflammation in type 1 interferonopathies is, in part, attributed to uncontrolled activation of microglial cells as seen in patients with macrophage activation syndrome (MAS) or hemophagocytic lymphohistiocytosis (HLH)[66]. Type 1 IFNs also affect other neuronal cells, causing profound effects on the CNS through multiple mechanisms[66,67]. Although the number of subjects, with each of the gene variants reported to cause type 1 interferonopathies, is not large, more and more genes are being reported to be associated with interferonopathies and many of them are inherited in an AD manner[68]. Therefore, this condition may be more likely to be frequently encountered as IEIs.
(2) Treatment measures: Since interferonopathies are caused by pathogenic variants of multiple genes, therapeutic approaches will be influenced by which genes are affected and what effects are exerted by other epigenetic factors[62], as evidenced by the markedly variable clinical presentations seen. It has been reported that patients with type 1 interferonopathies are generally resistant to the first-line treatment measures typically used for autoimmune conditions like lupus; efficacies of high dose IV bolus of methylprednisolone and/or IVIg for controlling the acute phase of symptoms are suboptimal at best[66,69].
Downstream signaling of type 1 IFNs involves Janus kinases (JAKs), which act as signal transducers, and four mammalian members of JAKs are identified: JAK1, JAK2, JAK3, and TYK2[70,71]. All JAKs, except for JAK3 that is expressed only in hematopoietic and lymphoid cells, are expressed ubiquitously and play a crucial role in the JAK-STAT (signal transducers and activators of transcription) pathway[71]. The JAK-STAT pathway transduces signaling from multiple cytokine receptors. JAK3 mediates signals from type 1 cytokines and thus the deficiency of JAK3 leads to SCIDS[72]. On the other hand, dysregulated activation of the JAK-STAT pathway will lead to chronic inflammatory conditions, implicated in the pathogenesis of autoimmune, allergic, and autoinflammatory conditions[73]. In patients with type 1 interferonopathies, type 1 IFN signaling involving JAK1/JAK2 and TYK2 has been proposed as therapeutic targets for these patients[66]. In clinical trials of small numbers of monogenic type 1 interferonopathies, improvement of clinical symptoms and inflammatory markers are reported with the use of JAK inhibitors, including baricitinib and tafacitinib[66,74-76]. Other types of treatment measures targeting the production and/or actions of type 1 IFNs have been proposed, but not widely used compared to JAK inhibitors[66,77,78].
JAK inhibitors have also become increasingly popular for treating various autoimmune conditions, including rheumatoid arthritis (RA), inflammatory bowel diseases (IBD), atopic dermatitis, etc. The actions of a new generation of JAK inhibitors are more selective for specific JAKs, as opposed to the 1st generation JAK inhibitors. In ASD subjects in whom type 1 IFN-induced neuroinflammation plays a role in their behavioral symptoms or cognitive dysfunctions, orally available JAK inhibitors may provide an additional treatment option. Interestingly, multiple open-label studies have reported the beneficial effects of baricitinib for severe COVID-19[79]. With the increase in reports of the favorable effects of baricitinib, the FDA issued an EUA (emergency use authorization) for its use as an adjunct treatment, with remdesivir, for hospitalized COVID-19 patients older than 2 years of age. In ASD patients who have a risk of more significant neuroinflammation following COVID-19, baricitinib may also offer another treatment option for controlling post-COVID inflammation.
Immunodeficiency affecting PI3K/Akt/mTOR pathways
PI3k/Akt/mTOR pathways are crucial for immune functions, especially differentiation of Th17 vs. Treg cell pathways, but these pathways are also crucial for neuronal cell activation and neuronal plasticity, as shown in patients diagnosed with tuberous sclerosis (TS)[80]. Dysregulation of these signaling pathways caused by multiple pathogenic gene variants including TS complex 1/2 are now categorized as activated PI3Kinase delta syndrome with highly variable clinical features[81]. As expected, apart from the immune system, this condition affects the nervous system, frequently exhibiting neurodevelopmental delay and learning disabilities along with autoimmune and autoinflammatory complications[81-83].
Class IA PI3kinase (PI3K) molecules consist of a p110 catalytic subunit and a regulatory subunit, and catalyze phosphatidylinositol 4.5 bisphosphate to the phosphatidylinositol 2,3,4 trisphosphate (PIP3), which serves as a secondary messenger for cell proliferation, activation, and differentiation[84]. Its catalytic unit (p110) is coded by genes PIK3CA, PIK3CB, and PIK3CD. PIK3CD is predominantly expressed in leukocytes[84]. Its regulatory subunits are coded by PIK3R1, PIK3R2, and PIK3R3[84]. AD, GOF mutations of PIK3CD (Activated PI3Kinase delta syndrome (APDS) 1) or AD, LOF mutations of PIK3R1 (APDS2) can cause overactivation of PI3K and downstream Akt/mTOR pathways in immune cells and other lineage cells. This results in complex clinical features of immunodeficiency and autoimmune/autoinflammatory symptoms, illustrating the importance of balanced activation in this signaling pathway in immune cells[81,85]. These patients present with clinical features overlapping with both type 1 interferonopathies and CAPS[81,85,86]. Regulatory proteins for PI3K activation also affect this signaling pathway and pathogenic variants coding for such regulatory molecules cause clinical features resembling APDS: such conditions are often referred to as APDS-like syndrome. LOF PTEN pathogenic variants are best known for causing APDS-like syndrome[85].
(1) APDS1 and APDS2: As described above, the clinical features of APDS1/APDS2 are highly variable. However, in general, these patients present with combined immunodeficiency with evidence of impaired humoral and cellular immunity. Impaired humoral immunity is typically suspected when they suffer from recurrent sinopulmonary infection caused by community-acquired organisms covered by infant vaccines (Prevnar and Hib). Ocular infection is reported to be frequently seen in APDS patients[83]. Impaired immunity exerted by T cells and innate immune cells is also impaired, as evidenced by an increase in the frequency of viral and opportunistic infection: asymptomatic viremia of CMV and EBV is common[83], as is persistent or recurrent herpes infection[87]. Chronic viral infection may lead to benign lymphadenopathy and hepatosplenomegaly[83,86]. In addition to symptoms associated with immunodeficiency, APDS patients also manifest autoimmune conditions typically after the first decade of life: cytopenia and glomerulonephritis are common, along with an increase in the risk of malignant complications[88]. Since both APDS1/APDS2 are inherited in an AD pattern, despite the recent identification of APDS, the frequency of this condition is expected to be fairly high; a total of 243 APDS patients were reported in the systemic review published in 2020[89]. We already follow two APDS patients in our clinic and it is not unusual for these patients to be found in other immunology clinics.
PI3Kδ coded by PIK3CD is upregulated in developing brains, and developmental delay and ASD-like behavioral symptoms have been described in APDS patients, more commonly in APDS2 patients[89]. Neurodevelopmental delay may manifest as mild cognitive impairment and/or learning disabilities[90].
The variable clinical manifestations of APDS led to highly individualized treatments for controlling the affected areas. Impaired humoral and cellular immunity are treated with Ig replacement therapy and prophylactic antibiosis targeting intracellular organisms. When they develop autoimmune complications, immunosuppressive treatment is often required. In addition to the 1st line of treatment measures such as steroids, therapies targeting the PI3Kδ signaling pathway have been tried. Sirolimus, a mTOR inhibitor, has been used, because sirolimus blocks mTOR, a gatekeeper enzyme, downstream of PI3Kδ[91]. Oral agents that directly inhibit enzymatic actions of PI3Kδ are now available with promising results[92,93]. These PI3Kδ inhibitors have also been used for treating malignancies such as CLL (chronic lymphocytic leukemia). However, it remains to be seen if these oral medications are applicable for controlling the CNS inflammation associated with dysregulated activation of PI3Kδ.
(2) PTEN hamartoma tumor syndrome (PHTS): PTEN (phosphatase and tensin homolog) coded by PTEN serves as an antagonist of PI3K/Akt/mTOR pathway activation. PTEN suppresses activation of this pathway by hydrolyzing PIP3 to PIP2, thereby inhibiting secondary messenger actions of PIP3 generated by PI3Kδ[94]. With this action, PTEN serves as a tumor suppressor, and heterozygous germline mutations of PTEN are associated with cancer syndromes[95]. Although PHTS is considered to be rare, previously described under multiple names of syndromes, the development of comprehensive diagnostic guidelines will provide better information on true prevalence[96]. Inherited LOF, AD, PTEN variants that result in over-activation of the PI3K/Akt/mTOR pathway will cause clinical features resembling those seen in APDS, which is referred to as APDS-like syndrome[85,96]. As with APDS patients, impaired neurodevelopment is also expected to occur in PHTS patients. In fact, a subset of PHTS patients are known to exhibit clinical features overlapping with ASD (impaired speech/social skills, and repetitive behaviors) along with macrocephaly and a high frequency of seizures[94]. It has been reported that PTEN mutations associated with ASD-like clinical features are dominant negative mutations, causing unstable but functional protein products, indicating the importance of PTEN in the early stage of neuronal development[94]. In MIA models in which maternal inflammation is induced by a low dose of endotoxin during pregnancy, mice carrying LOF PTEN variants revealed worsening macrocephalus and ASD-like behavioral symptoms in offspring[97]. Several PTEN animal models have also been shown to exhibit ASD-like clinical features[98]. Overactivation of PI3K/Akt/mTOR pathways due to PETN mutations will cause dysregulated immune activation and subsequent neuroinflammation and autoimmune conditions[85]. Therefore, PHTS subjects will be a good candidate for blockers targeting PI3K/Akt/mTOR pathways.
Sirolimus, an inhibitor of mTORC1, has been shown to attenuate clinical symptoms of PHTS patients[98,99]. A 6 month, randomized, double-blinded, placebo-controlled clinical trial of everolimus, another mTORC1 inhibitor, was recently conducted to evaluate its effects on neurocognitive symptoms in PHTS patients. The results indicated that everolimus has modest effects, but the wide range of ages in the study subjects
Phenocopies of IEI
Recent progress in IEI research has revealed the conditions that mimic the IEIs summarized in previous sections. For example, somatic mutations in NLRP3, TNSRSF6, KRAS, NRAS, and STAT5B revealed clinical features of autoinflammatory syndromes resembling those caused by inherited or de novo germline mutations of the above-described genes[9]. In patients with late-onset autoinflammatory syndrome without identifiable germline pathogenic variants, the possibility of somatic mutation may need to be considered. Likewise, the production of autoantibodies against key mediators of inflammation, usually cytokines, may also create phenocopies of the IEIs described above[9]. In ASD subjects with late-onset deterioration, the possibility of phenocopies of IEIs with the above-described mechanisms should be considered when exploring treatment options.
VEXAS syndrome
Among autoinflammatory syndromes caused by somatic mutation, Vacuoles, E1 enzyme, X-linked, Autoinflammatory, Somatic (VEXAS) syndrome is just recently described as an adult-onset monogenic autoinflammatory disease caused by somatic mutations of the UBA1 gene. This condition is characterized by severe adult-onset chronic inflammation associated with hematological disorders, most commonly myelodysplasia syndrome[101]. Despite the fact that this syndrome was only recently described, a fair number of VEXAS syndrome patients have been described, indicating that this condition may not be uncommon[101]. UBA1 encodes one of the two E1 enzyme isoforms and E1 enzyme is crucial for ubiquitylation, which is one of the cellular functions to terminate enzymatic actions. In the immune system, this mutation results in uncontrolled activation of immune responses, resulting in autoinflammatory syndrome[101]. Multiple organs are affected, as is seen in patients with autoinflammatory syndrome caused by germline mutations. The nervous system is also affected, manifesting symptoms of headache, cerebrovascular accidents, serous meningitis symptoms, and sensory neuropathy[102]. As for treatment, biologics targeting IL-1, IL-6, and JAK have been used along with hematopoietic stem cell transplant (HSCT) in severe cases[101]. When dealing with difficult cases with negative genetic workup results in ASD subjects, the possibility of somatic mutations may need to be considered, as illustrated in VEXAS syndrome.
Anti-cytokine autoantibodies
Anti-cytokine autoantibodies have also been recognized as phenocopies of IEIs[9]. These autoantibodies are thought to differ from anti-idiotypic agonistic antibodies that regulate immune balance for the prevention of autoimmune conditions[103]. For example, the production of antagonistic autoantibodies against IL-17 and IL-22 has been implicated in the pathogenesis of chronic mucocutaneous candidiasis, since both IL-17 and IL-22 are key cytokines produced by Th17 cells, exerting major defense against fungi[104,105]. Antagonistic autoantibodies against IL-6 predispose affected subjects to pyogenic infection, with clinical features resembling STAT3 deficiency or AD hyper IgE syndrome[105]. During the era of the COVID pandemic, the production of antagonistic autoantibodies against type 1 IFNs has been shown to be associated with an increased susceptibility to severe COVID-19 in the elderly[63]. However, such anti-cytokine autoantibodies are also detectable in asymptomatic individuals and clinical manifestations are highly variable[106]. In severe COVID-19 cases, the presence of anti-IFN autoantibodies is detrimental in the absence of immune memory, which generally exerts other arms of immune defense against viral infection[106]. In addition, the presence of anti-IFN autoantibodies may cause dysregulated immune responses and could lead to long-term sequelae of COVID-19, now often referred to as long COVID. We have treated several ASD subjects with long COVID. It is our experience that pre-existing difficult ASD behaviors make it challenging to diagnose and treat long COVID symptoms in a timely manner. ASD subjects with components of IEIs described in this review are likely more prone to produce such autoantibodies following potent immune stimuli such as COVID-19. In that regard, in older ASD subjects with sudden worsening behavioral symptoms, the possibility of phenocopies of IEIs may be kept in mind as a possible pathogenesis.
CONCLUSION
In this review, we have summarized IEIs that present with predominant neuropsychiatric symptoms, secondary to neuroinflammation and impaired neuronal development caused by pathogenic variants of genes/deletion of genes as summarized in Figure 1. These conditions can be manifested by microdeletion, genes affecting chromatin remodeling, and those affecting key signaling molecules shared by both the immune and nervous systems. It should be noted that both the development of autoantibodies against key mediators and/or somatic mutations of key genes may also cause clinical symptoms similar to those seen in patients with IEIs, although these patients tend to present later in life. Some patients with IEIs may initially be diagnosed with ASD, as we have experienced in our clinic. Clinicians need to be aware of the clinical features of IEIs that may overlap with ASD clinical presentation. It is also important to make use of the various genetic tests and treatment measures that are currently available for specific gene mutations. Research in these areas is rapidly expanding, and it will be important to keep up to date on the latest information. In addition, if ASD patients present with similar immune abnormalities as found in those with IEIs, immunomodulating agents targeting specific pathways affected by such gene mutations may be applicable, expanding the scope of treatment options.
DECLARATIONS
Acknowledgments
The author thanks Dr. L. Huguenin for critically reviewing the manuscript.
Authors’ contributions
The author contributed solely to the article.
Availability of data and materials
Not applicable.
Financial support and sponsorship
This work is partly funded by the Brain Foundation, Pleasanton, CA, the Jonty Foundation, St. Paul, MN, and the O’Sullivan Foundation, Princeton, NJ.
Conflicts of interest
The author declared that there are no conflicts of interest.
Ethical approval and consent to participate
Not applicable.
Consent for publication
Not applicable.
Copyright
© The Author(s) 2023.
Supplementary Materials
REFERENCES
1. Genovese A, Butler MG. The autism spectrum: behavioral, psychiatric and genetic associations. Genes 2023;14:677.
2. Singh R, Kisku A, Kungumaraj H, et al. Autism spectrum disorders: a recent update on targeting inflammatory pathways with natural anti-inflammatory agents. Biomedicines 2023;11:115.
3. Massrali A, Adhya D, Srivastava DP, Baron-Cohen S, Kotter MR. Virus-induced maternal immune activation as an environmental factor in the etiology of autism and schizophrenia. Front Neurosci 2022;16:834058.
4. Zawadzka A, Cieślik M, Adamczyk A. The role of maternal immune activation in the pathogenesis of autism: a review of the evidence, proposed mechanisms and implications for treatment. Int J Mol Sci 2021;22:11516.
5. Frye RE, Cakir J, Rose S, et al. Mitochondria may mediate prenatal environmental influences in autism spectrum disorder. J Pers Med 2021;11:218.
6. Bucknor MC, Gururajan A, Dale RC, Hofer MJ. A comprehensive approach to modeling maternal immune activation in rodents. Front Neurosci 2022;16:1071976.
7. Bekkering S, Domínguez-Andrés J, Joosten LAB, Riksen NP, Netea MG. Trained immunity: reprogramming innate immunity in health and disease. Annu Rev Immunol 2021;39:667-93.
8. Tercan H, Riksen NP, Joosten LAB, Netea MG, Bekkering S. Trained immunity: long-term adaptation in innate immune responses. Arterioscler Thromb Vasc Biol 2021;41:55-61.
9. Tangye SG, Al-Herz W, Bousfiha A, et al. Human inborn errors of immunity: 2022 update on the classification from the international union of immunological societies expert committee. J Clin Immunol 2022;42:1473-507.
10. Wilson DI, Burn J, Scambler P, Goodship J. DiGeorge syndrome: part of CATCH 22. J Med Genet 1993;30:852-6.
11. Mustillo PJ, Sullivan KE, Chinn IK, et al. Clinical practice guidelines for the immunological management of chromosome 22q11.2 deletion syndrome and other defects in thymic development. J Clin Immunol 2023;43:247-70.
12. Szczawinska-Poplonyk A, Schwartzmann E, Chmara Z, et al. Chromosome 22q11.2 deletion syndrome: a comprehensive review of molecular genetics in the context of multidisciplinary clinical approach. Int J Mol Sci 2023;24:8317.
13. Marcovecchio GE, Bortolomai I, Ferrua F, et al. Thymic epithelium abnormalities in DiGeorge and down syndrome patients Contribute to dysregulation in T cell development. Front Immunol 2019;10:447.
14. Menghi M, Micangeli G, Tarani F, et al. Neuroinflammation and oxidative stress in individuals affected by digeorge syndrome. Int J Mol Sci 2023;24:4242.
15. Fiksinski AM, Schneider M, Zinkstok J, Baribeau D, Chawner SJRA, Vorstman JAS. Neurodevelopmental trajectories and psychiatric morbidity: lessons learned from the 22q11.2 deletion syndrome. Curr Psychiatry Rep 2021;23:13.
16. Gothelf D, Law AJ, Frisch A, et al. Biological effects of COMT haplotypes and psychosis risk in 22q11.2 deletion syndrome. Biol Psychiatry 2014;75:406-13.
17. Radoeva PD, Coman IL, Salazar CA, et al. Association between autism spectrum disorder in individuals with velocardiofacial (22q11.2 deletion) syndrome and PRODH and COMT genotypes. Psychiatr Genet 2014;24:269-72.
18. Basson MA, van Ravenswaaij-Arts C. Functional insights into chromatin remodelling from studies on CHARGE syndrome. Trends Genet 2015;31:600-11.
19. Dijk DR, Bocca G, van Ravenswaaij-Arts CM. Growth in CHARGE syndrome: optimizing care with a multidisciplinary approach. J Multidiscip Healthc 2019;12:607-20.
20. Blake KD, Hudson AS. Gastrointestinal and feeding difficulties in CHARGE syndrome: a review from head-to-toe. Am J Med Genet C Semin Med Genet 2017;175:496-506.
21. Thomas AT, Waite J, Williams CA, Kirk J, Oliver C, Richards C. Phenotypic characteristics and variability in CHARGE syndrome: a PRISMA compliant systematic review and meta-analysis. J Neurodev Disord 2022;14:49.
22. Zhang R, He H, Yuan B, et al. An intronic variant of CHD7 identified in autism patients interferes with neuronal differentiation and development. Neurosci Bull 2021;37:1091-106.
23. Collins C, Sharpe E, Silber A, Kulke S, Hsieh EWY. Congenital athymia: genetic etiologies, clinical manifestations, diagnosis, and treatment. J Clin Immunol 2021;41:881-95.
24. Mai CT, Isenburg JL, Canfield MA, et al. National Birth Defects Prevention Network. National population-based estimates for major birth defects, 2010-2014. Birth Defects Res 2019;111:1420-35.
25. Jenner L, Richards C, Howard R, Moss J. Heterogeneity of autism characteristics in genetic syndromes: key considerations for assessment and support. Curr Dev Disord Rep 2023;10:132-46.
26. Micangeli G, Menghi M, Profeta G, et al. The impact of oxidative stress on pediatrics syndromes. Antioxidants 2022;11:1983.
27. Marcovecchio GE, Ferrua F, Fontana E, et al. Premature senescence and increased oxidative stress in the thymus of down syndrome patients. Front Immunol 2021;12:669893.
28. Janoff EN, Tseng HF, Nguyen JL, et al. Incidence and clinical outcomes of pneumonia in persons with down syndrome in the United States. Vaccine 2023;41:4571-8.
29. Peeters D, Pico-Knijnenburg I, Wieringa D, et al. AKT hyperphosphorylation and T cell exhaustion in down syndrome. Front Immunol 2022;13:724436.
30. Edmister S, Ibrahim R, Kakodkar R, Kreiling JA, Creton R. A zebrafish model for calcineurin-dependent brain function. Behav Brain Res 2022;416:113544.
31. Li Y, Bögershausen N, Alanay Y, et al. A mutation screen in patients with kabuki syndrome. Hum Genet 2011;130:715-24.
32. Boniel S, Szymańska K, Śmigiel R, Szczałuba K. kabuki syndrome-clinical review with molecular aspects. Genes 2021;12:468.
33. Barry KK, Tsaparlis M, Hoffman D, et al. From genotype to phenotype-a review of kabuki syndrome. Genes 2022;13:1761.
34. Kurahashi N, Miyake N, Mizuno S, et al. Characteristics of epilepsy in patients with kabuki syndrome with KMT2D mutations. Brain Dev 2017;39:672-7.
35. Orange JS, Ballow M, Stiehm ER, et al. Use and interpretation of diagnostic vaccination in primary immunodeficiency: a working group report of the basic and clinical immunology interest section of the American academy of allergy, asthma & immunology. J Allergy Clin Immunol 2012;130:S1-24.
36. McCusker C, Upton J, Warrington R. Primary immunodeficiency. Allergy Asthma Clin Immunol 2018;14:61.
37. Paris K, Wall LA. The Treatment of primary immune deficiencies: lessons learned and future opportunities. Clin Rev Allergy Immunol 2023;65:19-30.
38. Zengeler KE, Lukens JR. Innate immunity at the crossroads of healthy brain maturation and neurodevelopmental disorders. Nat Rev Immunol 2021;21:454-68.
39. International FMF Consortium. Ancient missense mutations in a new member of the RoRet gene family are likely to cause familial Mediterranean fever. Cell 1997;90:797-807.
40. Ben-Chetrit E, Touitou I. Familial mediterranean fever in the world. Arthritis Rheum 2009;61:1447-53.
41. Georgin-Lavialle S, Ducharme-Benard S, Sarrabay G, Savey L, Grateau G, Hentgen V. Systemic autoinflammatory diseases: clinical state of the art. Best Pract Res Clin Rheumatol 2020;34:101529.
42. Maggio MC, Corsello G. FMF is not always “fever”: from clinical presentation to “treat to target”. Ital J Pediatr 2020;46:7.
43. Migita K, Fujita Y, Asano T, Sato S. The expanding spectrum of autoinflammatory diseases. Intern Med 2023;62:43-50.
44. Vanzo RJ, Prasad A, Staunch L, et al. The temple grandin genome: comprehensive analysis in a scientist with high-functioning autism. J Pers Med 2020;11:21.
45. Alehashemi S, Goldbach-Mansky R. Human autoinflammatory diseases mediated by NLRP3-, Pyrin-, NLRP1-, and NLRC4-inflammasome dysregulation updates on diagnosis, treatment, and the respective roles of IL-1 and IL-18. Front Immunol 2020;11:1840.
46. Masters SL, Lagou V, Jéru I, et al. Familial autoinflammation with neutrophilic dermatosis reveals a regulatory mechanism of pyrin activation. Sci Transl Med 2016;8:332ra45.
47. Missoum H, Adadi N, Alami M, et al. Correlation genotype-phenotype: MEFV gene mutations and Moroccan patients with rheumatoid arthritis. Pan Afr Med J 2022;41:121.
49. Cuisset L, Jeru I, Dumont B, et al. French CAPS study group. Mutations in the autoinflammatory cryopyrin-associated periodic syndrome gene: epidemiological study and lessons from eight years of genetic analysis in France. Ann Rheum Dis 2011;70:495-9.
50. Mulazzani E, Wagner D, Havla J, et al. Neurological phenotypes in patients with NLRP3-, MEFV-, and TNFRSF1A low-penetrance variants. J Neuroinflammation 2020;17:196.
51. Reyes AZ, Hu KA, Teperman J, et al. Anti-inflammatory therapy for COVID-19 infection: the case for colchicine. Ann Rheum Dis 2021;80:550-7.
52. Potere N, Del Buono MG, Caricchio R, et al. Interleukin-1 and the NLRP3 inflammasome in COVID-19: pathogenetic and therapeutic implications. EBioMedicine 2022;85:104299.
53. Martínez GJ, Celermajer DS, Patel S. The NLRP3 inflammasome and the emerging role of colchicine to inhibit atherosclerosis-associated inflammation. Atherosclerosis 2018;269:262-71.
54. Slobodnick A, Shah B, Krasnokutsky S, Pillinger MH. Update on colchicine, 2017. Rheumatology 2018;57:i4-11.
55. Shah B, Allen N, Harchandani B, et al. Effect of colchicine on platelet-platelet and platelet-leukocyte interactions: a pilot study in healthy subjects. Inflammation 2016;39:182-9.
56. Dubois EA, Rissmann R, Cohen AF. Rilonacept and canakinumab. Br J Clin Pharmacol 2011;71:639-41.
57. Baskar S, Klein AL, Zeft A. The use of IL-1 receptor antagonist (anakinra) in idiopathic recurrent pericarditis: a narrative review. Cardiol Res Pract 2016;2016:7840724.
58. Jyonouchi H, Geng L. Resolution of EEG findings and clinical improvement in a patient with encephalopathy and ESES with a combination of immunomodulating agents other than corticosteroids: a case report. Epilepsy Behav Rep 2020;14:100379.
59. Kyriazopoulou E, Huet T, Cavalli G, et al. International Collaborative Group for Anakinra in COVID-19. Effect of anakinra on mortality in patients with COVID-19: a systematic review and patient-level meta-analysis. Lancet Rheumatol 2021;3:e690-7.
60. Kyriazopoulou E, Poulakou G, Milionis H, et al. Early treatment of COVID-19 with anakinra guided by soluble urokinase plasminogen receptor plasma levels: a double-blind, randomized controlled phase 3 trial. Nat Med 2021;27:1752-60.
61. Dahms K, Mikolajewska A, Ansems K, Metzendorf MI, Benstoem C, Stegemann M. Anakinra for the treatment of COVID-19 patients: a systematic review and meta-analysis. Eur J Med Res 2023;28:100.
62. Siebeler R, de Winther MPJ, Hoeksema MA. The regulatory landscape of macrophage interferon signaling in inflammation. J Allergy Clin Immunol 2023;152:326-37.
63. Bastard P, Gervais A, Le Voyer T, et al. Autoantibodies neutralizing type I IFNs are present in ~4% of uninfected individuals over 70 years old and account for ~20% of COVID-19 deaths. Sci Immunol 2021;6:62.
64. Crow YJ, Stetson DB. The type I interferonopathies: 10 years on. Nat Rev Immunol 2022;22:471-83.
65. Manna R, Rigante D. The everchanging framework of autoinflammation. Intern Emerg Med 2021;16:1759-70.
66. d'Angelo DM, Di Filippo P, Breda L, Chiarelli F. Type I interferonopathies in children: an overview. Front Pediatr 2021;9:631329.
67. Viengkhou B, Hofer MJ. Breaking down the cellular responses to type I interferon neurotoxicity in the brain. Front Immunol 2023;14:1110593.
68. Haşlak F, Kılıç Könte E, Aslan E, Şahin S, Kasapçopur Ö. Type I interferonopathies in childhood. Balkan Med J 2023;40:165-74.
69. Volpi S, Picco P, Caorsi R, Candotti F, Gattorno M. Type I interferonopathies in pediatric rheumatology. Pediatr Rheumatol Online J 2016;14:35.
70. Jain NK, Tailang M, Jain HK, et al. Therapeutic implications of current Janus kinase inhibitors as anti-COVID agents: a review. Front Pharmacol 2023;14:1135145.
71. Mortezavi M, Martin DA, Schulze-Koops H. After 25 years of drug development, do we know JAK? RMD Open 2022;8:e002409.
72. Mella P, Schumacher RF, Cranston T, de Saint Basile G, Savoldi G, Notarangelo LD. Eleven novel JAK3 mutations in patients with severe combined immunodeficiency-including the first patients with mutations in the kinase domain. Hum Mutat 2001;18:355-6.
73. Xin P, Xu X, Deng C, et al. The role of JAK/STAT signaling pathway and its inhibitors in diseases. Int Immunopharmacol 2020;80:106210.
74. Sanchez GAM, Reinhardt A, Ramsey S, et al. JAK1/2 inhibition with baricitinib in the treatment of autoinflammatory interferonopathies. J Clin Invest 2018;128:3041-52.
75. Li W, Wang W, Wang W, et al. Janus kinase inhibitors in the treatment of type I interferonopathies: a case series from a single center in China. Front Immunol 2022;13:825367.
76. Kanazawa N, Ishii T, Takita Y, Nishikawa A, Nishikomori R. Efficacy and safety of baricitinib in Japanese patients with autoinflammatory type I interferonopathies (NNS/CANDLE, SAVI, And AGS). Pediatr Rheumatol Online J 2023;21:38.
77. Lindahl H, Bryceson YT. Neuroinflammation associated with inborn errors of immunity. Front Immunol 2021;12:827815.
78. Lin B, Goldbach-Mansky R. Pathogenic insights from genetic causes of autoinflammatory inflammasomopathies and interferonopathies. J Allergy Clin Immunol 2022;149:819-32.
79. Yuan Y, Jiao B, Qu L, Yang D, Liu R. The development of COVID-19 treatment. Front Immunol 2023;14:1125246.
80. Luo C, Ye WR, Shi W, et al. Perfect match: mTOR inhibitors and tuberous sclerosis complex. Orphanet J Rare Dis 2022;17:106.
81. Thouenon R, Moreno-Corona N, Poggi L, Durandy A, Kracker S. Activated PI3Kinase delta syndrome-a multifaceted disease. Front Pediatr 2021;9:652405.
82. Elkaim E, Neven B, Bruneau J, et al. Clinical and immunologic phenotype associated with activated phosphoinositide 3-kinase δ syndrome 2: a cohort study. J Allergy Clin Immunol 2016;138:210-8.e9.
83. Coulter TI, Chandra A, Bacon CM, et al. Clinical spectrum and features of activated phosphoinositide 3-kinase δ syndrome: a large patient cohort study. J Allergy Clin Immunol 2017;139:597-606.e4.
84. Okkenhaug K. Signaling by the phosphoinositide 3-kinase family in immune cells. Annu Rev Immunol 2013;31:675-704.
85. Redenbaugh V, Coulter T. Disorders related to PI3Kδ hyperactivation: characterizing the clinical and immunological features of activated PI3-kinase delta syndromes. Front Pediatr 2021;9:702872.
86. Tessarin G, Rossi S, Baronio M, et al. Activated phosphoinositide 3-kinase delta syndrome 1: clinical and immunological data from an Italian cohort of patients. J Clin Med 2020;9:3335.
87. Cohen JI. Herpesviruses in the Activated phosphatidylinositol-3-kinase-δ syndrome. Front Immunol 2018;9:237.
88. Maccari ME, Abolhassani H, Aghamohammadi A, et al. Disease evolution and response to rapamycin in activated phosphoinositide 3-kinase δ syndrome: the European society for immunodeficiencies-activated phosphoinositide 3-kinase δ syndrome registry. Front Immunol 2018;9:543.
89. Jamee M, Moniri S, Zaki-Dizaji M, et al. Clinical, immunological, and genetic features in patients with activated pi3kδ syndrome (APDS): a systematic review. Clin Rev Allergy Immunol 2020;59:323-33.
90. Moreno-Corona N, Chentout L, Poggi L, et al. Two monogenetic disorders, activated PI3-kinase-δ syndrome 2 and smith-magenis syndrome, in one patient: case report and a literature review of neurodevelopmental impact in primary immunodeficiencies associated with disturbed PI3K signaling. Front Pediatr 2021;9:688022.
91. Kang JM, Kim SK, Kim D, et al. Successful sirolimus treatment for korean patients with activated phosphoinositide 3-kinase δ syndrome 1: the first case series in Korea. Yonsei Med J 2020;61:542-6.
92. Rao VK, Webster S, Dalm VASH, et al. Effective “activated PI3Kδ syndrome”-targeted therapy with the PI3Kδ inhibitor leniolisib. Blood 2017;130:2307-16.
93. Diaz N, Juarez M, Cancrini C, et al. Seletalisib for activated PI3Kδ syndromes: open-label phase 1b and extension studies. J Immunol 2020;205:2979-87.
94. Rademacher S, Eickholt BJ. PTEN in autism and neurodevelopmental disorders. Cold Spring Harb Perspect Med 2019;9:a036780.
95. Spinelli L, Black FM, Berg JN, Eickholt BJ, Leslie NR. Functionally distinct groups of inherited PTEN mutations in autism and tumour syndromes. J Med Genet 2015;52:128-34.
96. Plamper M, Gohlke B, Woelfle J. PTEN hamartoma tumor syndrome in childhood and adolescence-a comprehensive review and presentation of the German pediatric guideline. Mol Cell Pediatr 2022;9:3.
97. Le Belle JE, Sperry J, Ngo A, et al. Maternal inflammation contributes to brain overgrowth and autism-associated behaviors through altered redox signaling in stem and progenitor cells. Stem Cell Reports 2014;3:725-34.
98. DeSpenza T Jr, Carlson M, Panchagnula S, et al. PTEN mutations in autism spectrum disorder and congenital hydrocephalus: developmental pleiotropy and therapeutic targets. Trends Neurosci 2021;44:961-76.
99. Yehia L, Ngeow J, Eng C. PTEN-opathies: from biological insights to evidence-based precision medicine. J Clin Invest 2019;129:452-64.
100. Srivastava S, Jo B, Zhang B, et al. Developmental Synaptopathies Consortium. A randomized controlled trial of everolimus for neurocognitive symptoms in PTEN hamartoma tumor syndrome. Hum Mol Genet 2022;31:3393-404.
101. Vitale A, Caggiano V, Bimonte A, et al. VEXAS syndrome: a new paradigm for adult-onset monogenic autoinflammatory diseases. Intern Emerg Med 2023;18:711-22.
102. Georgin-Lavialle S, Terrier B, Guedon AF, et al. Further characterization of clinical and laboratory features in VEXAS syndrome: large-scale analysis of a multicentre case series of 116 French patients. Br J Dermatol 2022;186:564-74.
103. Stanova AK, Ryabkova VA, Utekhin SV, Shoenfeld VJ, Churilov LP, Shoenfeld Y. Anti-idiotypic agonistic antibodies: candidates for the role of universal remedy. Antibodies 2020;9:19.
104. Bunte K, Beikler T. Th17 cells and the IL-23/IL-17 axis in the pathogenesis of periodontitis and immune-mediated inflammatory diseases. Int J Mol Sci 2019;20:3394.
105. Ku CL, Chi CY, von Bernuth H, Doffinger R. Autoantibodies against cytokines: phenocopies of primary immunodeficiencies? Hum Genet 2020;139:783-94.
Cite This Article

How to Cite
Download Citation
Export Citation File:
Type of Import
Tips on Downloading Citation
Citation Manager File Format
Type of Import
Direct Import: When the Direct Import option is selected (the default state), a dialogue box will give you the option to Save or Open the downloaded citation data. Choosing Open will either launch your citation manager or give you a choice of applications with which to use the metadata. The Save option saves the file locally for later use.
Indirect Import: When the Indirect Import option is selected, the metadata is displayed and may be copied and pasted as needed.
About This Article
Special Issue
Copyright
Data & Comments
Data
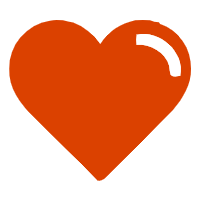
Comments
Comments must be written in English. Spam, offensive content, impersonation, and private information will not be permitted. If any comment is reported and identified as inappropriate content by OAE staff, the comment will be removed without notice. If you have any queries or need any help, please contact us at [email protected].