Use of sodium D, L-3-hydroxybutyrate as adjunct therapy in two siblings with HMG-CoA lyase deficiency
Abstract
3-hydroxy-3-methylglutaryl-coenzyme A (HMG-CoA) lyase deficiency is a rare autosomal recessive mitochondrial disease characterised by recurrent life-threatening metabolic crises generally presenting in neonates or infancy during catabolic stress triggered by prolonged fasting or intercurrent illness. Acute decompensations with lethargy, vomiting, hypoketotic hypoglycemia and metabolic acidosis may evolve into Reye-like syndrome if untreated, with acute liver failure, hyperammonemic encephalopathy, dilated cardiomyopathy, and death in 20% of cases. Long-term health complications include psychomotor retardation, white matter abnormalities, epilepsy, hepatic steatosis, pancreatitis, cardiomyopathy, and arrythmia. The mitochondrial enzyme catalyses the cleavage of HMG-CoA to acetyl-CoA and acetoacetate, the common final step of ketogenesis and leucine degradation, resulting in diagnostic urinary organic acid pattern (elevated 3-hydroxy-3-methylglutaric, 3-methylgutaconic, 3-methylglutaric, and 3-hydroxyisovaleric acids) with the absence of ketonuria, when deficient. Therapeutic interventions include dietary protein or leucine and fat restriction, carnitine supplementation, avoidance of fasting, and use of carbohydrate-based high caloric intake when unwell. We describe the clinical course and diagnostic work-up in two affected siblings, with the proband presenting with severe neonatal onset disease with metabolic acidosis, non-ketotic hypoglycaemia, hyperammonemia and white matter changes on brain MRI. High-risk screening for a younger sibling led to pre-emptive management with good outcomes. Sodium D, L-3-hydroxybutyrate (S-DL-3OHB) was used in both siblings as an adjunct therapy to prevent cerebral dysfunction and cardiomyopathy, with the rationale that decreased ketogenesis in this disorder may impact the major energy source for the brain and heart during starvation. S-DL-3OHB therapy is a well-tolerated and effective therapeutic option for this disorder.
Keywords
INTRODUCTION
3-hydroxy-3-methylglutaryl-coenzyme A (HMG-CoA) lyase deficiency (OMIM # 246450) is a rare inborn error of metabolism. A systematic literature review identified 211 worldwide cases, with a pan-ethnic distribution, although many patients originate from the Iberic peninsula, and Saudi Arabia where it is the most prevalent organic aciduria[1], with an incidence of 1:55,357[2], approximately twice the world prevalence of less than 1/100,000 live births[3]. The mitochondrial HMG-CoA lyase (HMGCL; EC 4.1.2.4) catalyses the cleavage of HMG-CoA to acetyl-CoA and acetoacetate which is the common final step of ketogenesis and leucine degradation [Figure 1][4,5]. Ketone bodies are essential alternative energy sources for extrahepatic organs, particularly the brain at times of insufficient energy supply, wherein it generates up to 60% of the brain’s energy requirement[6]. Consequently, HMGCL deficiency is characterised by recurrent episodes of life-threatening non-ketotic hypoglycemia and metabolic acidosis, usually precipitated by catabolic stress including fasting, intercurrent illness and excessive protein intake (leucine load), resulting in protein breakdown and increased ketogenesis. Greater than 95% of patients present with acute metabolic decompensations[1] manifesting with lethargy, vomiting, hypotonia, tachypnoea, and if left untreated, may progress to Reye-like syndrome with liver failure and hyperammonemia, neurological compromise with cerebral infarction or stroke-like encephalopathy[7] and even death[1]. Hyperammonemia can be severe, with ammonia levels > 1,000 μmol/L described, and peritoneal dialysis performed in one patient with levels
Figure 1. Biochemical pathway illustrating role of HMG-CoA lyase in leucine catabolism and ketogenesis. Mitochondrial HMG-CoA lyase catalyses the cleavage of HMG-CoA to acetyl-CoA and acetoacetate, the common final step of ketogenesis and leucine degradation, resulting in a diagnostic urinary organic acid pattern, (elevated 3-hydroxy-3-methylglutaric, 3-methylgutaconic,
Urine organic acid profiles include the absence of ketonuria and accumulation of characteristic leucine metabolites including 3-hydroxy-3-methylglutarate (HMG), 3-methylglutaconate (MGC),
Here, we report a 7-year-old patient who presented aged 6 days with lethargy, respiratory distress, metabolic acidosis, non-ketotic hypoglycaemia, and hyperammonemia. Dietary management and carnitine supplementation were combined with S-DL-3OHB as an effective adjunct to prevent cerebral dysfunction and cardiomyopathy. His similarly affected, 12-month-old younger sibling was pre-emptively managed through high-risk newborn screening, with a good outcome.
CASE REPORT
Patient 1
A male neonate, the first child of consanguineous Lebanese parents, was born at 38 weeks after an uncomplicated pregnancy with a birth weight of 2.945 kg (21st centile) and Apgar scores 9 at 1 and 5 min. He developed respiratory distress at 31 h of age, metabolic acidosis (pH 7.2, HCO3 9, base excess -17), and hypoglycaemia (1 mmol/L). He responded promptly to intravenous 10% dextrose correction, antibiotic coverage for 48 h, and was discharged home on day five, fully breastfed.
He represented on day six with respiratory distress, poor feeding, lethargy, approximately 10% weight loss (2.66 kg), hypothermia (33.0 °C), compensated metabolic acidosis (pH 7.38, pCO2 16 mmHg, HCO3
Upon presentation, he was clinically inactive, though his Moro’s reflex was intact, and appeared oedematous. His head circumference was 37 cm (63rd centile). Liver was palpable two cm below the right costal margin in the midclavicular line. Liver function tests showed hypoalbuminemia and transaminitis [Table 1]. Plasma amino acids were generally low, suggestive of poor oral intake which corroborated with the preceding history of decreased feeding for three to four days. Urgent urine organic acid analysis showed grossly increased HMG, MGC, MGL and 3IV, confirming a diagnosis of HMGCL deficiency. Plasma acylcarnitine analysis displayed an increase in C5-OH acylcarnitine (2.59 µmol/L, RR < 0.15). Day 2 newborn screening showed elevated C5-OH (2.8 µmol/L, RR < 1). Formal echocardiogram was normal. He commenced on a protein and fat-restricted diet, oral S-DL-3OHB 150 mg/kg/dose, QID in the acute phase and 150 mg/kg/dose BD when well (Veriton Pharma, Weybride, UK), and L-carnitine supplementation
Metabolic parameters in blood used to monitor clinical response to treatment in patient 1
Age | Day 6 of life | Day 7 of life | 2 weeks | 5 months | 12 months | 20 months | 4.5 years (admission) | 6 years |
Ammonia NH3 (umol/L) [RR 20-100 in neonates, thereafter 0-50] | 160 | 15 | 9 | NA | < 9 | NA | 25 | NA |
Blood Gasses pH | 7.38 [7.32-7.46] | 7.45 | 7.43 | NA | NA | NA | 7.39 [RR 7.34-7.43] | NA |
Base excess (mmol/L) [RR -4.0 to 3.0] | -14 | -3.6 | -0.6 | NA | NA | NA | -2.8 | NA |
Bicarbonate (mmol/L) | 9 [RR 18-24] | 18.7 | 23.1 [RR 18-24] | NA | NA | NA | 21.6 [RR 22-26] | NA |
Blood lactate (mmol/L) | 2.6 [RR 0.7-1.9] | 1.7 | 2.0 [RR0.7 1.9] | NA | NA | NA | 2.8 | NA |
Glucose (mmol/L) [RR 3.5-5.5] | 1.7 | 5.0 | 4.8 | NA | NA | NA | 5.2 | NA |
Plasma acylcarnitine- Total carnitine (umol/L) [RR 8-45] | 11 | NA | 44 | 80 [RR 21-70] | NA | 107 [RR 21-70] | 68 | 49 |
Free carnitine (umol/L) [RR 4-33] | 3 | NA | 33 | 46 [RR 13-56] | 83 [RR 13-56] | 34 | 29 | |
C5-OH (umol/L) [RR 0.01-0.15] | 2.59 | NA | 0.45 | 0.37 [RR 0.00-0.31] | NA | 0.80 [RR 0.00-0.31] | NA | 0.82 [RR 0.00-0.10] |
C6DC (umol/L) | 0.07 | NA | 0.19 | 0.23 [RR 0.02-0.06] | NA | 0.45 [RR 0.02-0.06] | NA | 0.17 [RR 0.00-0.08] |
Formal blood bicarbonate | NA | NA | NA | 24 [RR 16-29] | 22 | 23 | 20 [RR 17-30] | 28 |
Liver Function Tests | ||||||||
ALT (U/L) [RR 0-45] | 272 | NA | 95 | 33 | 30 | 30 | 14 | 21 |
AST (U/L) [RR 20-90] | 211 | NA | 35 | 68 | 59 | 66 | 49 | 81 |
ALP (U/L) [RR 120-550] | 120 | NA | 95 | 252 | 136 | 150 | 247 | 219 |
GGT (U/L) [RR 0-200] | 160 | NA | 146 | 48 | 11 | < 10 | 13 | 16 |
Albumin (g/L) [RR 28-40] | 23 | NA | 20 | 37 | 46 | 47 | 47 | 50 |
Free fatty acids (umol/L) [RR 100-550] | NA | NA | NA | NA | NA | NA | 1,512 (inpatient) | NA |
Beta-hydroxybutyrate (mmol/L) [RR 0.00-0.50] | NA | NA | NA | < 0.18 | 0.31 | < 0.18 | < 0.18 | < 0.18 |
Capillary beta-hydroxybutyrate- bedside testing | Not detected | 0.2 mmol/L | NA | 0.2 mmol/L- during admissions at 7 months | NA | NA | NA | NA |
He attained age-appropriate developmental milestones and weight gain till 12 months of age. During an interim period, with a change in clinical care after 12 months, the dose of his S-DL-3OHB had decreased from approximately 530 mg/kg/day (when well) at 12 months (8.42 kg) to 300 mg/kg/day at 15 months
His parents noted a decline in his overall well-being since approximately 15 months. He was described to be increasingly lethargic, irritable, and displayed regression of speech and language skills. He had nine presentations within 15 months to the emergency department and admissions, with the most severe at 2.5 years, when he had status epilepticus lasting 66 mins due to severe hypoglycemia (unrecordable blood glucose), hyperammonemic encephalopathy (ammonia 261 µmol/L), metabolic acidosis and lactic acidemia. The lack of dose increment of S-DL-3OHB, appropriate to his weight gain, was a potential cause for his decompensation as his dietary management had largely remained the same, and there were no triggering intercurrent illnesses or prolonged fasting preceding this event. His social and language skills further regressed after this acute decompensation. Formal developmental assessment using the Mullen Scales of Early Learning at 2 years 9 months concurred with clinical assessments of normal, age- related milestone till age 12 months, with subsequent regression thereafter. He was diagnosed with DSM-5 (Diagnostic and Statistical Manual of Mental Disorders-fifth edition by the American Psychiatric Association, 2013) criteria for autism spectrum disorder and significant global developmental delay with severe speech and language impairment.
Following this, the dose of S-DL-3OHB was increased to 500 mg/kg/day, with no associated changes in his dietary management. Within 2 to 3 days of this dose adjustment, his parents noticed an improvement in his activity and energy levels, appetite, decreased irritability and subsequently, a gain of new developmental milestones over the following months. 3-day food diary at 3 years showed restriction of both fat and protein to approximately 11.5% energy. This provided 3.1 g protein/kg/day, predominantly from cereal and vegetable sources, and he was also taking high amylopectin starch (1.5 g/kg, Glycosade®) before bed. At his last review aged 6.5 years, he interacted well with his treating team, spoke in short sentences, and had good eye contact, which corroborated with encouraging feedback from his clinical psychologist who had observed improvements in his autistic behaviour. He was well thrived, weighing 22.0 kg (60th percentile) and height of 113.6 cm (27th percentile) on the Centers for Disease Control and Prevention (CDC) growth charts.
Molecular analysis by massively parallel sequencing determined a homozygous pathogenic frameshift variant, classified as per American College of Medical Genetics and Genomics guidelines[15], c.887_888del, in the last exon of the in the HMGCL gene, which was predicted to create a premature stop codon (p.Leu296Profs*19), resulting in a null allele. Both parents are heterozygous for the variant.
Patient 2
Prenatal diagnosis for the younger sibling of patient 1 was declined by the parents; hence, high-risk management was initiated at birth, before confirmatory diagnosis was available. The female neonate was born after an uncomplicated pregnancy with normal Apgar scores and a birth weight of 3.045 kg. She had an initial hypoglycaemia (1.7 mmol/L) noted soon after birth, which normalised with IV dextrose 10% maintenance fluids. Her ammonia levels, blood gas, and lactate levels remained normal. Day 2 newborn screening showed elevated C5-OH (1.9 µmol/L, RR < 1) and normal plasma amino acids. Upon confirmation of HMG CoA lyase deficiency through urine organic acid analysis (increased HMG, MGC, MGL and 3IV) and elevated plasma C5-OH acylcarnitine
Metabolic parameters in blood used to monitor clinical response to treatment in patient 2
Age | 12 h of life-different hospital specific results not available | 3 weeks | 4 months | 16 months |
Ammonia NH3 (umol/L) [RR 20-100 in neonates, thereafter 0-50] | N | 42 | 12 | |
Blood Gasses pH | N | 7.37 | NA | NA |
Base excess (mmol/L) [-3 to 3.0] | N | -0.8 | NA | NA |
Bicarbonate (mmol/L) [RR 15-28] | N | 24.5 | NA | NA |
Blood lactate (mmol/L) [RR 0.0-1.9] | N | 2.7 | NA | 2.5 |
Glucose (mmol/L) [RR 3.5-5.4] | N | 4.9 | NA | 5.1 |
Plasma acylcarnitine- Total carnitine (umol/L) [RR 8-45] | 14 | NA | 44 | 67 [RR 21-70] |
Free carnitine (umol/L) [RR 4-33)] | 9 | NA | 33 | 55 [RR 13-56] |
C5-OH (umol/L) [RR 0.01-0.10] | 0.45 | NA | 0.45 | 0.17 |
C6DC (umol/L) [RR 0.00-0.08] | 0.17 | NA | 0.59 | 0.07 |
Formal blood bicarbonate) (mmol/L)) [RR 16-29] | N | NA | 26 | 26 |
Liver Function Tests | ||||
ALT (U/L) [RR 0-58] | N | 20 | 35 | 25 |
AST (U/L) [RR 0-97] | N | 44 | 63 | 42 |
ALP (U/L) [RR 120-650] | N | 295 | 295 | 236 |
GGT (U/L) [RR 0-45] | N | 51 | 146 | 21 |
Albumin (g/L) [RR 28-40] | N | 33 | 41 | 39 |
Beta-hydroxybutyrate (mmol/L) [RR 0.00-0.50] | NA | < 0.18 | < 0.18 | ND |
Capillary beta-hydroxybutyrate- bedside testing | NA | ND | ND | ND |
At 9 months of age, her total dietary intake provided 1.2 g protein/kg and 23% energy from fat. At all ages, prescribed unwell feeds provided 120% estimated energy requirements, with S-DL-3OHB maintained at approximately 150 mg/kg/dose TDS when well, and 150 mg/kg/dose, 4-hourly when unwell. Clinically, she has progressed well with satisfactory weight (10.48 kg, 43 percentile) and height (76 cm, 42nd percentile, CDC growth chart) gain and age-appropriate developmental milestones when last reviewed at 16 months. She is due to have a cardiac ECHO soon and has remained clinically asymptomatic.
MATERIALS AND METHODS
Urine organic acid analysis
Organic acids in urine were analysed by gas chromatography/mass spectrometry (GC/MS) (QP-2010 Ultra, Shimadzu Corp., Japan), as previously described[16,17].
Plasma acylcarnitine analysis
Underivatized acylcarnitine analysis on plasma was performed by ultra-high-performance liquid chromatography-tandem mass spectrometry (UPLC-MS/MS) (Xevo TQ-S Acquity UPLC, Waters, USA). Analysis was based on a previously published method[18].
DISCUSSION
Treatment strategies described in the comprehensive review by Grünert and Sass 2020[1] include avoidance of fasting, dietary fat and leucine/protein restriction in 54.3% of patients, low-leucine or low-protein diet in 43.8% of patients, many of whom were supplemented with a leucine-free amino acid mixture, while only one patient (1%) had a fat-restricted diet without protein restriction. 7.7% of patients had no dietary restrictions, and a few had a self-imposed diet prior to diagnosis. Additional carbohydrate supplementation with uncooked corn starch or glucose polymers was used by some patients. L-carnitine supplementation was prescribed in 78% of patients[1] as it enhances the urinary excretion of toxic metabolites, facilitates the transport of fatty acids into the mitochondria and acts as a free radical scavenger and cofactor in the oxidation of long-chain fatty acids[2].
The use of S-DL-3OHB as an alternative energy source for ATP production by circumventing the disturbed ketogenesis has previously been described in our patient in a prior abstract[19] and in an 8-month-old infant with HMGCL deficiency from our centre, with demonstrated stabilisation of declining blood glucose levels after administration via a peristaltic pump at 12 and 14 h of a fasting study[12,14]. This therapy had successfully reversed deep white matter changes on brain MRI in a 3-month-old male infant with HMGCL deficiency after presenting on day 2 with vomiting, metabolic acidosis, hypoglycaemia, and hyperammonaemia and additionally, in a 16-year-old following acute encephalopathy with cerebella tonsillar herniation, liver dysfunction and hyperammonaemia[4,13].
Long-term S-DL-3OHB treatment has been used in other inborn errors of metabolism, with improved cardiac contractility in infants and reversal of progressive leukodystrophy in children with multiple acyl-CoA dehydrogenase deficiency[20-24]. Exogenous ketone bodies have been utilised as an effective adjunct therapy in pyruvate dehydrogenase deficiency[25], hyperinsulinemic hypoglycaemia[26], carnitine-acylcarnitine translocase deficiency, very long-chain acyl-CoA dehydrogenase deficiency, glycogen storage disease type III, mitochondrial complex IV deficiency and Brown-Violetto-Van Laere syndrome[24]. Stable isotope infusion studies have demonstrated increased endogenous ketone body production in fasting healthy newborns compared with healthy adults, hence indicating higher exogenous requirements in infants when endogenous generation is insufficient[27]. Titration of S-DL-3OHB towards at least detectable concentrations in blood or urine can be a surrogate marker for dosing adequacy[24]. Reported side effects, which were difficult to discriminate from intercurrent illness, included constipation, diarrhea, abdominal pain, vomiting or nausea, and dehydration. The risk of side effects appeared to rise with dose increment of S-DL-3OHB, but could not be clearly ascertained if attributable to the higher dose or associated cation load[24]. Doses up to 900 mg/kg/day have been used for HMGCL deficiency in the acute setting[4]. Higher doses of up to 2,600 mg/kg/day, divided into one to six daily doses, have also been described in multiple acyl-CoA dehydrogenase deficiency (MADD), also known as glutaric aciduria type II, OMIM 231680)[23]. Severe alkalosis and hypernatremia have been reported with S-DL-3OHB doses above 1,400 mg/kg/day[21]; hence, appropriate hydration is recommended to prevent associated electrolyte challenges. The benefits of S-DL-3OHB appeared to outweigh the side effects.
CONCLUSION
This report highlights the need for prompt recognition of HMGCL deficiency as a differential in any neonatal presentation of non-ketotic hypoglycaemia, hyperammonemia, and metabolic acidosis. While our observations are suggestive of the benefits of S-DL-3OHB as an adjunct therapy in the management of HMGCL deficiency, future long-term studies are warranted to correlate clinical improvements with biomarkers to determine efficacy.
DECLARATIONS
Authors’ contributionsInvolving in the clinical management of the patient and outlined the focus of the manuscript: Balasubramaniam S
Overseeing the biochemical genetics studies and prepared the initial draft: Devanapalli B, Tolun AA
Overseeing the newborn screening studies: Kim WT, Wotton T
Providing oversight on the dietary management: Thompson S
All authors contributed to writing and critically revising the manuscript.
Availability of data and materialsNot applicable.
Financial support and sponsorshipNone.
Conflicts of interestAll authors declared that there are no conflicts of interest.
Ethical approval and consent to participateThis study was approved by the Sydney Children’s Hospitals Network Human Research Ethics Committee, Westmead, NSW, Australia (project # CCR2019/29).
Consent for publicationAll procedures followed were in accordance with the ethical standards of the responsible committee. Informed consent was obtained from the patients' mother for inclusion in this write-up.
Copyright© The Author(s) 2023.
REFERENCES
1. Grunert SC, Sass JO. 3-hydroxy-3-methylglutaryl-coenzyme A lyase deficiency: one disease - many faces. Orphanet J Rare Dis 2020;15:48.
2. Alfadhel M, Al Othaim A, Al Saif S, et al. Expanded newborn screening program in Saudi Arabia: incidence of screened disorders. J Paediatr Child Health 2017;53:585-91.
3. Santarelli F, Cassanello M, Enea A, et al. A neonatal case of 3-hydroxy-3-methylglutaric-coenzyme A lyase deficiency. Ital J Pediatr 2013;39:33.
4. Thompson S, Hertzog A, Selvanathan A, et al. Treatment of HMG-CoA lyase deficiency-longitudinal data on clinical and nutritional management of 10 Australian cases. Nutrients 2023;15:531.
5. Pie J, Lopez-Vinas E, Puisac B, et al. Molecular genetics of HMG-CoA lyase deficiency. Mol Genet Metab 2007;92:198-209.
7. Huemer M, Muehl A, Wandl-Vergesslich K, Strobl W, Wanders RJ, Stoeckler-Ipsiroglu S. Stroke-like encephalopathy in an infant with 3-hydroxy-3-methylglutaryl-coenzyme A lyase deficiency. Eur J Pediatr 1998;157:743-6.
8. Stacey TE, de Sousa C, Tracey BM, et al. Dizygotic twins with 3-hydroxy-3-methylglutaric aciduria; unusual presentation, family studies and dietary management. Eur J Pediatr 1985;144:177-81.
9. Alfadhel M, Abadel B, Almaghthawi H, et al. HMG-CoA lyase deficiency: a retrospective study of 62 saudi patients. Front Genet 2022;13:880464.
10. Yalcinkaya C, Dincer A, Gunduz E, Ficicioglu C, Kocer N, Aydin A. MRI and MRS in HMG-CoA lyase deficiency. Pediatr Neurol 1999;20:375-80.
11. Sass JO, Fukao T, Mitchell GA. Inborn errors of ketone body metabolism and transport: an update for the clinic and for clinical laboratories. J Inborn Errors Metabolism Scr 2018;6:2326409818771101.
12. Bhattacharya K, Matar W, Tolun AA, et al. The use of sodium DL-3-hydroxybutyrate in severe acute neuro-metabolic compromise in patients with inherited ketone body synthetic disorders. Orphanet J Rare Dis 2020;15:53.
13. Dalkeith T, Ellaway C, Thompson S. The use of 3-hydroxybutyrate in patients with fat oxidation disorders. In 12th International Congress of Inborn Errors of Metabolism. Barcelona, Spain; 2013.
14. François B, Bachmann C, Schutgens RBH. Glucose metabolism in a child with 3-hydroxy-3-methylglutaryl-coenzyme a lyase deficiency. J Inherit Metab Dis 1981;4:163-4.
15. Richards S, Aziz N, Bale S, et al. Standards and guidelines for the interpretation of sequence variants: a joint consensus recommendation of the American college of medical genetics and genomics and the association for molecular pathology. Genet Med 2015;17:405-24.
16. Chalmers RA, Lawson AM. Organic acids in man: analytical chemistry, biochemistry and diagnosis of the organic acidurias. Dordrecht: Springer; 2012. Available from: https://link.springer.com/book/10.1007/978-94-009-5778-7 [Last accessed on 16 Sep 2023].
17. Goodman SI, Markey SP. Diagnosis of organic acidemias by gas chromatography-mass spectrometry. Lab Res Methods Biol Med 1981;6:1-158.
18. Millington DS, Kodo N, Norwood DL, Roe CR. Tandem mass spectrometry: a new method for acylcarnitine profiling with potential for neonatal screening for inborn errors of metabolism. J Inherit Metab Dis 1990;13:321-4.
19. Devanapalli B, Balasubramaniam S, Carpenter K. D, L-3-hydroxybutyrate treatment of HMG-CoA lyase deficiency in a patient. In 13th International Congress of Inborn Errors of Metabolism. Rio de Janeiro, Brazil; 2017.
20. Gautschi M, Weisstanner C, Slotboom J, Nava E, Zurcher T, Nuoffer JM. Highly efficient ketone body treatment in multiple acyl-CoA dehydrogenase deficiency-related leukodystrophy. Pediatr Res 2015;77:91-8.
21. Habarou F, Bahi-Buisson N, Lebigot E, et al. Ketone bodies as a possible adjuvant to ketogenic diet in PDHc deficiency but not in GLUT1 deficiency. JIMD Rep 2018;38:53-9.
22. Van Hove JL, Grunewald S, Jaeken J, et al. D,L-3-hydroxybutyrate treatment of multiple acyl-CoA dehydrogenase deficiency (MADD). Lancet 2003;361:1433-5.
23. Van Rijt WJ, Heiner-Fokkema MR, du Marchie Sarvaas GJ, et al. Favorable outcome after physiologic dose of sodium-D,L-3-hydroxybutyrate in severe MADD. Pediatrics 2014;134:e1224-8.
24. van Rijt WJ, Jager EA, Allersma DP, et al. Efficacy and safety of D,L-3-hydroxybutyrate (D,L-3-HB) treatment in multiple acyl-CoA dehydrogenase deficiency. Genet Med 2020;22:908-16.
25. Plecko B, Stoeckler-Ipsiroglu S, Schober E, et al. Oral beta-hydroxybutyrate supplementation in two patients with hyperinsulinemic hypoglycemia: monitoring of beta-hydroxybutyrate levels in blood and cerebrospinal fluid, and in the brain by in vivo magnetic resonance spectroscopy. Pediatr Res 2002;52:301-6.
26. Fischer T, Och U, Marquardt T. Long-term ketone body therapy of severe multiple acyl-CoA dehydrogenase deficiency: a case report. Nutrition 2019;60:122-8.
Cite This Article

How to Cite
Download Citation
Export Citation File:
Type of Import
Tips on Downloading Citation
Citation Manager File Format
Type of Import
Direct Import: When the Direct Import option is selected (the default state), a dialogue box will give you the option to Save or Open the downloaded citation data. Choosing Open will either launch your citation manager or give you a choice of applications with which to use the metadata. The Save option saves the file locally for later use.
Indirect Import: When the Indirect Import option is selected, the metadata is displayed and may be copied and pasted as needed.
About This Article
Copyright
Data & Comments
Data
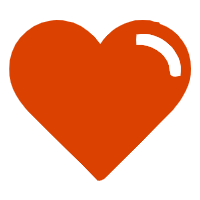
Comments
Comments must be written in English. Spam, offensive content, impersonation, and private information will not be permitted. If any comment is reported and identified as inappropriate content by OAE staff, the comment will be removed without notice. If you have any queries or need any help, please contact us at [email protected].