Single-phase grounding line selection method for closed-loop distribution network adapted to medium voltage microgrid access
Abstract
Aim: The access of the medium-voltage (MV) microgrid changes the radial structure of traditional distribution networks, causing the distribution network to operate in a closed-loop manner. The closed-loop operation complicates the fault characteristics of the distribution network, and the traditional single-phase grounding (SPG) line selection method is no longer applicable. Therefore, it is necessary to propose a new targeted SPG line selection method.
Methods: This paper analyzes the network structure of MV microgrids and MV distribution networks and establishes a multi-level closed-loop operation distribution network model with MV microgrid access. Then, the SPG fault characteristics of the closed-loop operation distribution system under the small current grounding mode are analyzed from the perspective of two-level bus nodes. Furthermore, based on the analysis of fault characteristics, a closed-loop operation distribution network line selection method suitable for microgrid access is proposed, and a detailed design is carried out in terms of equipment configuration and communication schemes, forming a relatively complete automatic line selection system. At the same time, the proposed line selection scheme is based on the two-level bus nodes, which can be analogously applied to the multi-level closed-loop operation distribution network.
Results: The simulation results show that the proposed line selection scheme can be effectively applied to the closed-loop operation distribution network with MV microgrid access.
Conclusion: The traditional SPG line selection method will have misjudgment problems after the MV microgrid is integrated into the distribution network, but the line selection method proposed in this paper has good adaptability.
Keywords
INTRODUCTION
Microgrids play a key role in improving the reliability of distribution networks, supporting the high penetration rate of renewable energy, and improving the efficiency of power generation[1-3]. With the development of power electronics technology and the optimization of micro-power control technology, the microgrid with large capacity has gradually developed into the form of access to the distribution network through medium-voltage (MV) levels, such as 10 kV or 20 kV[4,5]. It cooperates with the large power grid to provide effective power support for all loads in distribution networks.
Single-phase grounding (SPG) fault is a frequent fault type in the distribution system. In small current grounding systems, the fault line is often allowed to run for a period of time when the SPG fault occurs. However, in order to prevent further deterioration of the fault and ensure the safety of both personnel and equipment, it is important to detect the fault line in time and carry out isolation maintenance. Similar to the MV distribution network, the MV microgrid also has two small current grounding modes: the neutral point ungrounded and the neutral point grounded by arc suppression coil (ASC)[6]. Adapted to the MV distribution network, two small-current grounding systems will be formed after the MV microgrid access to the distribution network. On the other hand, the distribution network in China usually adopts the “closed-loop design, open-loop operation” power supply mode, which is essentially a radial structure. The access of the MV microgrid changes the traditional topology of the distribution network, causing it to operate in a closed-loop manner. The closed-loop distribution network can further improve the power supply reliability of the system, but it also complicates its fault characteristics and further increases the difficulty of fault line detection[7,8].
At present, most research on SPG fault line selection of distribution networks is based on the radial network topology. There are few studies on SPG fault line selection for microgrids and for closed-loop operation networks formed after microgrids are incorporated into the distribution network. In engineering, the SPG fault line selection for the traditional radial distribution network usually adopts the method of group amplitude comparison and phase comparison[9] (neutral point ungrounded system) and the method of zero-sequence current (ZSC) active component[10] (neutral point grounded by ASC system). In addition, there are various line selection methods, such as the traveling wave method[11], injection method[12], and information fusion method[13]. However, these methods are all based on the radial network, which is difficult to apply to the closed-loop operation network formed after the MV microgrid access to the distribution network. In order to meet the increasing demand for power supply, Singapore and some developed areas in China have established petal-shaped distribution networks with closed-loop operation. However, the petal-shaped distribution network adopts the small resistance grounding method, and differential protection is usually used to cut off the fault line instantaneously when a single-phase ground fault occurs[14-16]. Therefore, the petal-shaped distribution network under closed-loop operation actually has no specific grounding fault line selection problem. In[17], it is envisaged to transform the existing distribution network into closed-loop operation, and an SPG comprehensive line selection method based on complex distribution network structures is proposed, but it can only be applied to the proposed specific grid structure.
The microgrid usually has multiple micro-power sources, so it is often in the state of closed-loop operation, and it may even form a multi-level closed-loop operation system structure after its access to the distribution network. In the research of SPG fault protection in microgrids, based on the idea of combining control and protection, a distance protection scheme with a high ability to resist transition resistance is proposed for adapting to island scenarios under SPG faults, as outlined in[18]. A zero-sequence non-power frequency component injection microgrid grounding fault protection scheme is proposed in[19], which can be applied to both grid-connected and islanded operation modes of microgrids. In fact, SPG faults are usually instantaneous, and the fast-tripping method is not conducive to improving the reliability of the microgrid operation. The microgrid should first choose the small current grounding method. In[20], it is found that the islanded microgrid with the neutral point ungrounded mode and ring network topology has a virtual terminal point (ZSC is zero) when an SPG fault occurs, and a fault line selection method based on the skewness signs of high-frequency zero-sequence voltage and zero-sequence current is proposed. However, this method is only applicable to microgrids with islanded operation and ring network topology and cannot be applied to multi-microgrid clusters and the microgrid-distribution network coupling system under grid-connected conditions.
In this paper, a complete line selection scheme is proposed for the closed-loop operation network under the small current grounding mode formed after the MV microgrid access to the distribution network. Firstly, a closed-loop operation distribution network model with MV microgrid access is established based on the grid structure of the MV microgrid and distribution network. The SPG fault characteristics of the closed-loop operation distribution network are analyzed by taking the fault occurring in the connecting feeder and non-connecting feeder, respectively. On this basis, a closed-loop operation distribution network line selection scheme suitable for MV microgrid access is designed. Finally, the fault simulation model is built by Matlab/Simulink, and the effectiveness of the proposed scheme is verified.
SYSTEM DESCRIPTION
Topology of MV microgrid
The capacity of the microgrid connected at the voltage level of 10 kV and above is mostly at the megawatt level. In terms of the connection form of the power generation unit, unlike the direct integration of multiple distributed power sources that are complementary in the low-voltage microgrid, the MV microgrid has higher voltage and capacity, and the power generation unit usually needs to be connected to the microgrid bus through the boost transformer[21]. The common connection forms of photovoltaic power generation and wind power generation in medium voltage microgrids are shown in Figure 1. Limited by the capacity of the inverter, the megawatt photovoltaic power station usually designs the photovoltaic module into multiple
Figure 1. Common distributed power supply wiring forms. (A) Photovoltaic power generation unit; (B) wind power unit.
The microgrid can operate in both grid-connected mode and island mode and access to the large power grid through the public coupling point (PCC) switch. In addition, the distributed power supply in the microgrid has various forms, such as wind turbines, photovoltaic cells, diesel generators, and micro gas turbines. It can usually be divided into two types: inverter interfaced micro-distributed generation (IIDG) and rotating motor micro-distributed generation (RMDG)[22]. According to the microgrid structure proposed by the Consortium for Electric Reliability Technology Solutions and the actual wiring form of the MV microgrid in Dongao Island of China[23,24], the typical topology of a generally representative MV microgrid is shown in Figure 2.
Figure 2. Wiring diagram of medium voltage microgrid. IIDG: Inverter interfaced micro-distributed generation; PCC: public coupling point; RMDG: rotating motor micro-distributed generation.
In Figure 2, the line where the PCC is located is the connecting line for the energy interaction between the microgrid and the distribution network. The microgrid mainly contains three feeders: feeder L1 access to the RMDG, feeder L2 access to the non-sensitive load, and feeder L3 access to the IIDG and equipped with a sensitive load.
Topology of MV distribution network
According to the power grid structure recommended by China’s Technical Guidelines for Distribution Network Planning and Design, MV overhead lines mostly adopt multi-segment and multi-connection, multi-segment and single-connection, and multi-segment and single-radiation grids. MV cable lines generally adopt ring network structures, including single-ring networks and double-ring networks. Some common MV distribution network wiring is shown in Figure 3.
Figure 3. Common medium voltage distribution network wiring. (A) Multi-section single-connection wiring; (B) Single-ring network wiring.
In fact, although the MV distribution network adopts a closed-loop structure, the connecting switch is disconnected during normal operation; that is, the distribution network operates in an open-loop mode. Therefore, the MV distribution network in normal operation is often a single-source radial structure.
Closed-loop operation distribution network with multi-level nodes
Based on the topology of the MV microgrid and MV distribution network analyzed in Sections 2.1 and 2.2, the closed-loop operation distribution network after microgrid access is established, as shown in Figure 4. The closed-loop operation distribution network is composed of the original open-loop operation distribution network and two connected microgrids. The 10 kV busbar of the original open-loop operation distribution network has three feeders: L1 and L2 are normal load feeders, and L3 is a connecting feeder with the three-section two-connection structure. In addition to connecting the two microgrids, L3 also undertakes certain loads. It can be seen that after the microgrid access to the traditional open-loop distribution network, the original single-power radial structure of the distribution network is changed; that is, the distribution network becomes a closed-loop operation network powered by multi-terminal power supplies.
Figure 4. Closed-loop operation distribution network with medium voltage microgrid access. IIDG: Inverter interfaced micro-distributed generation; PCC: public coupling point; RMDG: rotating motor micro-distributed generation.
In fact, the microgrid itself has multiple power supplies, so it is in a closed-loop operation state, and the closed-loop operation structure of the system after the microgrid access to the distribution network will be more complicated. As shown in Figure 4, the system will have multi-level bus nodes after the MV microgrid access to the distribution network. If the bus node M1 of the distribution network is the first-level node, the two microgrid bus nodes M2 and M3 connected by the feeder L3 are the second-level nodes. Obviously, M2-1 and M3-1 are the next-level nodes of M2 and M3, respectively, and the more complex structures can be deduced by analogy. Due to the nesting relationship between different busbar nodes, it is only necessary to analyze the fault problem of the closed-loop operation network from the perspective of two-level busbar nodes. For example, M2-1 and M3-1 can be regarded as loaded feeders to analyze the fault characteristics of the closed-loop operation between the distribution network and the two microgrids. It should be noted that in order to simplify the analysis, the influence of the power winding (transformer winding) and its connecting line with the 10kV busbar on the ground capacitance will not be considered in the following. Therefore, there are a total of 9 feeder lines in the closed-loop operation system shown in Figure 4, L1~L9, among which L3, L4, and L9 are connecting feeders. Under normal operation, the PCC switch is closed, and the system is in a closed-loop operation state. Hereinafter, the system after the MV microgrid access to the distribution network shown in Figure 4 is called the closed-loop operation distribution network.
METHODS
Single-phase grounding fault characteristics of closed-loop distribution network
Neutral point ungrounded system
Neutral point ungrounded is a common small current grounding method in distribution networks. Adapting to the grounding mode of the connected distribution network, the MV microgrid also adopts the neutral point ungrounded mode, thus forming a neutral point ungrounded closed-loop operation network. Considering the same production model and working environment of each phase conductor used in each outgoing line, the three-phase conductors have the same capacitance Ci to the ground. Under normal operation, each phase has a capacitive current to the ground, and the phase is ahead of the phase voltage. At the same time, because the three-phase voltage and line parameters are symmetrical, the sum of the three-phase capacitance current is 0; that is, there is no ZSC. It is stipulated that the direction of the ZSC flowing from the busbar to the feeder is positive. The fault characteristics of the distribution network with neutral point ungrounded closed-loop operation are analyzed by taking the SPG faults occurring in the non-connecting feeder (L2 fault as an example) and in the connecting feeder (L3 fault as an example), respectively.
Non-connecting feeder fault, taking L2 as an example
When the fault occurs in L2, the ZSC distribution of each line of the system is shown in Figure 5. Among them, the zero-sequence current of all non-fault non-connecting feeders (L1, L5, L6, L7, L8) is the capacitive current of the line itself to the ground, and the direction is from the bus to the line.
Figure 5. ZSC distribution diagram of closed-loop distribution network under L2 fault. ZSC: Zero-sequence current.
For the connecting feeder, if it does not belong to the same busbar as the fault line, (L4, L9), its ZSC is the sum of the non-connecting feeders at the same bus node, and the direction is from the feeders to the busbar.
If it and the fault line belong to the same busbar (L3), its ZSC is the sum of the ZSC of this line and the ZSC of the downstream line, and the direction is from the busbar to the feeder.
The ZSC of the fault line L2 is the sum of the ZSC of all non-fault lines in the system, and the direction flows from the feeders to the busbar.
From the above analysis, when the fault occurs in non-connecting feeder L2, the ZSC of the fault line L2 is the largest, and the current direction is opposite to other feeders at the same bus node M1. Therefore, the traditional amplitude and phase comparison method can be used to select the fault line in the node feeder. However, for the connecting feeders of other bus nodes (M2, M3), the current direction is the same as the ZSC direction of the fault line, and the amplitude is large, which will be misjudged as the fault line.
Connecting feeder fault, taking L3 as an example
When the fault occurs in L3, the ZSC flow of each line of the system is shown in Figure 6. At this time, the ZSC of all non-fault non-connecting feeders (L1, L2, L5, L6, L7, L8) is still the capacitance current of the line itself to the ground, and the direction is from the busbar to the feeder. For the non-fault connecting feeders (L4, L9), the ZSC is still the sum of the non-connecting feeders in the same bus, and the direction is from the feeder to the busbar. However, the magnitude of the ZSC of the fault connecting feeder L3 becomes the sum of the ZSC of the non-connecting feeder of the busbar where it is located, and the direction flows from the feeder to the busbar.
Figure 6. ZSC distribution diagram of closed-loop distribution network under L3 fault. ZSC: Zero-sequence current.
Therefore, when the fault occurs in the connecting feeder, the fault condition is the same as that when the bus nodes are not connected to each other and operate in an independent radial manner and the faults occur in the connecting feeders (L3, L4, L9) at the same time. At this time, the traditional amplitude and phase comparison method will mistake all the connecting feeders as fault feeders.
The simulation results of the above two cases are shown in Figure 7A and B, respectively. In the above two figures, the connecting lines L3, L4, and L9 are respectively represented by imaginary lines. It can be seen that the current directions of connecting lines L4 and L9 are the same as those of fault lines, which is easy to cause misjudgment of line selection.
Neutral point grounded by arc suppression coil system
When the grounding current of the distribution system is too large and the arc cannot be extinguished by itself, the method of installing ASC at the neutral point is often used to compensate for the capacitive current of the power grid to greatly reduce the grounding current. In China, 110 kV/10 kV or 220 kV/10 kV transformers use the YNd11 connection, so there is no grounded neutral point for 10 kV lines. Generally, the grounded transformer with a Z-type connection method is used to form the artificial neutral point, and then the grounded system through ASC is formed by arc suppression devices. The capacity of the MV microgrid is larger, the line is longer, and the capacitance current to ground is also larger. At the same time, to adapt to the grounding method of the connected distribution network, the MV microgrid should also adopt the grounding method through the ASC, thus forming a closed-loop operation network whose neutral point is grounded through the ASC. The traditional ASC grounding system usually adopts the zero-sequence active component method to select the line. Therefore, this section discusses the zero-sequence active current distribution of the system in the closed-loop operation. In the same way, the fault characteristics of the distribution network with the neutral point grounded by ASC and closed-loop operation are analyzed by taking the SPG faults occurring in the non-connecting feeder (L2 fault as an example) and in the connecting feeder (L3 fault as an example), respectively.
Non-connecting feeder fault, taking L2 as an example
The zero-sequence active current distribution is shown in Figure 8. The zero-sequence active current flowing into the fault point is composed of three parts, which are respectively the zero-sequence active current formed by the ASCs connected to the bus nodes M1, M2, and M3.
Figure 8. Zero-sequence active current distribution diagram of closed-loop distribution network under L2 fault.
Due to the closed-loop operation of the system, zero-sequence active current will also appear on the connecting feeder. If the connecting feeder and the fault line do not belong to the same bus node, that is, L4 and L9, the zero-sequence active current is the active current to the ground of the bus node where it is located.
If the connecting feeder and the fault line belong to the same bus node, namely L3, its zero-sequence active current is the sum of the zero-sequence active current of the downstream line.
Based on the above analysis, when the non-connecting feeder has an SPG fault, in addition to the fault line, the zero-sequence active current will also appear on the connecting feeder, and the zero-sequence active current of the connecting feeder at the same bus node is the sum of the zero-sequence active current of the downstream line. At this time, the line selection scheme based on the zero-sequence active component method will be misjudged. At this time, in addition to the faulted feeder, the zero-sequence active component will also appear on the three connecting feeders. The zero-sequence active component generated by the connecting feeders (L4, L9) of the two non-fault bus nodes is large and will be mistaken for the fault line.
Connecting feeder fault, taking L3 as an example
The zero-sequence active current distribution at this time is shown in Figure 9. The zero-sequence active current of the fault line is the active current generated by the zero-sequence voltage (ZSV) of the bus to the ground through the ASC. Since the system is connected by the connecting line, zero-sequence active current will also be generated on other connecting feeders, and the magnitude is the active current generated by the ZSV of the bus through the ASC. At this time, zero-sequence active current components appear on all connecting feeders, and the large amplitude can trigger the zero-sequence active component method line selection device of each node. According to the principle of maximum amplitude of zero-sequence active component, all connecting lines will be judged as fault lines. Therefore, it is impossible to select the line by the zero-sequence active component method.
Figure 9. Zero-sequence active current distribution diagram of closed-loop distribution network under L3 fault.
The simulation results of the above two cases are shown in Figure 10A and B, respectively. It can be seen that, except for the fault line, all connecting lines will be judged as fault lines.
Single-phase grounding line selection method for closed-loop distribution network
In the previous analysis, various line selection schemes applied to the traditional distribution network cannot show good adaptability when applied in the closed-loop operation system. Therefore, based on the analysis of Section 3, this section proposes corresponding reliable line selection schemes for the closed-loop distribution network under two small current grounding modes.
Line selection scheme for neutral point ungrounded system
Since the traditional group amplitude and phase comparison method will misjudge the connecting line, it is proposed to use the zero-sequence current direction at both ends of the connecting line to preliminarily judge the fault location of each bus node to lock the fault area.
In Figure 11, the red part represents the distribution automation feeder terminal unit (FTU) installed on the line, which is used to judge the occurrence of faults and measure ZSV and ZSC. FTUs are installed at the head of each feeder and the ends of the connecting line. It is stipulated that the direction of the ZSC of the feeder flowing from the busbar to the feeder is positive, and the direction of the ZSC on the connecting line flowing from the busbar or feeder to the connecting line is positive. The following gives the direction of zero-sequence steady-state current at both ends of the connecting line when an SPG fault occurs in each line.
From the Figure 12, general conclusions can be drawn: (1) If the direction of the ZSC at terminal b of the connecting line is positive, there must be an SPG fault on the feeder at node M2; (2) If the direction of the ZSC at terminal d of the connecting line is positive, there must be an SPG fault on the feeder at node M3; (3) Only when the direction of ZSC at the terminal a and terminal c of the connecting line is positive at the same time can it be judged that an SPG fault on the feeder at node M1; (4) When the ZSC at the terminal a and terminal b of the connecting line is negative at the same time, there must be a SPG fault on the connecting line L4. In the same way, when the ZSC at terminal c and terminal d of the connecting line is negative at the same time, there must be an SPG fault on connecting line L9. The above conclusions also constitute the logical basis for our preliminary determination of the fault location.
Figure 12. The zero-sequence steady-state current direction table at both ends of the connecting line when faults occur on different lines.
The specific line selection scheme is as follows:
(1) For each busbar node, set up a microcomputer line selection device (MLSD), set up a master station (M1), and two slave stations (M2, M3). Each MLSD operates independently during the line selection work; the slave station only selects the feeder line of its own node, and the master station is not only responsible for the feeder line of its own node but also selects the connecting line.
(2) Set the sampling frequency and the ZSV setting value Uset of FTU at each node. The setting value should be greater than the unbalanced voltage under normal conditions. When the two U0 sampling values are greater than the set value, it can be determined that the SPG fault has occurred on the line, and the fault data will be sent to the corresponding MLSD.
(3) The FTU measurement data of each node feeder is directly transmitted to the node MLSD, and the zero-sequence current direction of each feeder is judged by it. The FTU measurement data at both ends of each connecting line are transmitted to the corresponding MLSD, and the MLSD judges the direction of the ZSC at each end of the connecting line. For the slave, only the signal from the FTU at the head of the connecting line connected to the node is received, and the master receives the signal from the FTU at both ends of all the connecting lines, as shown in Figure 11.
(4) The MLSD of each node judges the node where the fault occurs according to the above logic judgment information or directly obtains the connecting line where the fault occurs by the master station.
(5) If it is determined that the fault occurs at a certain node, the fault feeder or node bus is further determined by the amplitude-phase comparison method.
Combined with the above description, the line selection process of a closed-loop distribution network with neutral point ungrounded mode is shown in Figure 13.
Line selection scheme for neutral point grounded by arc suppression coil system
Due to the influence of ASC compensation, the direction of the fault line may become the same as that of the normal line, and the amplitude is uncertain according to the change of compensation degree. In addition, considering the misjudgment of zero-sequence active components in the closed-loop distribution network and the complexity of zero-sequence active component extraction, a ZSC difference method line selection scheme based on ASC tuning is proposed.
Different from Section 3.1, the MLSD of each node in Figure 14 needs to communicate to cooperate with the line selection, and the slave station no longer receives the connecting line signal. Figure 15 shows the change of the zero-sequence steady-state current at both ends of the connecting line after adjusting the tuning degree of the ASC at node M1 of the master station when an SPG fault occurs on each line. In order to prevent the influence of errors, it is stipulated that the change of ZSC of each line is greater than 5% of the ZSC of the neutral point of the bus node to the ground fault, which can be considered as a significant change in the ZSC.
Figure 15. Table of zero-sequence steady-state current changes at both ends of the connecting line when faults occur on different lines.
Where ΔI0.iis the variation of the ZSC amplitude of the i feeder or at some end of the connecting line, and
The general conclusions can be drawn from Figure 15: (1) If the ZSC at terminal b of the connecting line changes significantly, it must be an SPG fault in the feeder of node M2; (2) If the ZSC at terminal d of the connecting line changes significantly, it must be an SPG fault in the feeder of node M3; (3) Only when the ZSC at the terminal a and terminal c of the connecting line does not change at the same time can it be judged that an SPG fault has occurred in the feeder of node M1; (4) Only when the ZSC at end a of the connecting line changes and the ZSC at end b does not change can it be judged that a SPG fault has occurred on connecting line L4. Similarly, only when the ZSC at terminal c of the connecting line changes and the ZSC at terminal d does not change can it be judged that an SPG fault has occurred on connecting line L9. The above conclusions also constitute the logical basis for our preliminary determination of the fault location.
The specific line selection scheme is as follows:
(1) For each busbar node, set up an MLSD, set up a master station (M1), and two slave stations (M2, M3). Each MLSD operates independently during the line selection work; the slave station only selects the feeder line of its own node, and the master station is not only responsible for the feeder line of its own node but also selects the connecting line.
(2) Set the sampling frequency and the ZSV setting value Uset of FTU at each node. The setting value should be greater than the unbalanced voltage under normal conditions. When the two U0 sampling values are greater than the set value, it can be determined that the SPG fault has occurred on the line, and the fault data will be sent to the corresponding MLSD.
(3) The FTU measurement data of each node feeder is directly transmitted to the node MLSD, and the FTU measurement data at both ends of each connecting line is transmitted to the MLSD of the master station. The master station node MLSD obtains the steady-state current amplitude of the master station node feeder and each connecting line under a 10% system overcompensation degree. At the same time, the steady-state current amplitude of the feeder of the slave station node is obtained by the MLSD of the slave station node.
(4) The master station MLSD controls the master station ASC overcompensation to 15% of the whole system after a period of fault. The FTU device returns the measured signal. The master station and the slave station obtain the second ZSC measurement amplitude and make a difference between the two measurement data to obtain the current amplitude variation.
(5) The master station MLSD determines the node where the fault occurs according to the above logic judgment information or directly obtains the connecting line where the fault occurs from the master station. If it is judged that the fault occurs on the connecting line, the master station will send a no-fault signal to each slave station, and the line selection will end. If it is judged that the fault occurred in the node feeder of the master station, the master station will send a no-fault signal to each slave station. Afterward, the master station selects the line with the largest difference as the faulted feeder according to the current amplitude change of the feeder it is responsible for, or if there is no obvious change in the feeder, it is a busbar fault. And the line selection ends.
(6) If it is judged that the fault occurs on the feeder of the non-master station node, the master station sends the fault signal to the fault slave station and sends the no-fault signal to other slave stations. The slave station selects the line with the largest difference as the faulted feeder according to the current amplitude change of the feeder it is responsible for, or if there is no obvious change in the feeder, it is a busbar fault. After the line selection is completed, each ASC returns to the 10% overcompensation state.
Combined with the above description, the line selection process of the closed-loop operation distribution network with the neutral point grounded through the arc-suppression coil is shown in Figure 16.
RESULTS
Line selection scheme verification for neutral point ungrounded system
Aiming at the neutral point ungrounded closed-loop operation distribution network, this paper proposes an improved amplitude and phase comparison method based on the identification of the ZSC direction of the connecting line in Section 4.1. This section uses the Matlab/Simulink platform to verify the scheme. The parameters of the simulation test system are shown in Table 1 (the parameters are the same as the simulation model in Section 4.2).
Table of Simulation test system parameter
Parameter | Value | Parameter | Value |
Positive sequence resistance | 0.01273 Ω/km | Zero-sequence resistance | 0.3864 Ω/km |
Positive sequence inductance | 0.9337 mH/km | Zero-sequence inductance | 4.1264 mH/km |
Positive sequence capacitance | 12.74 pF/km | Negative sequence capacitance | 7.751 pF/km |
Length of L1 | 20 km | Length of L2 | 62 km |
Length of L3-1 | 6 km | Length of L3-2 | 6 km |
Length of L3-3 | 6 km | Length of L4 | 12 km |
Length of L5 | 20 km | Length of L6 | 2 km |
Length of L7 | 30 km | Length of L8 | 23 km |
Length of L9 | 12 km | Power capacity | Infinite |
Taking a single-phase ground fault on the connecting line L4 as an example, Figure 17 shows the steady-state waveforms of the corresponding FTU data received by each MLSD. The magenta dotted line represents the reduced ZSV waveform so that we can analyze the direction of each ZSC. It is stipulated that the direction of ZSC leading ZSV is positive, and the direction of lagging ZSV is negative. The MLSD of each node works independently. In the waveform of the master station M1, the direction of La is negative, while the direction of Lc is positive, but not positive at the same time. Therefore, node M1 has no fault. Further judging whether the connecting line has a fault, it is found that the La and Lb directions are negative at the same time, so it is concluded that the SPG fault occurs on connecting line L4. For slave M2, the direction of Lb is negative, so node M2 has no fault. For slave M3, the direction of Ld is negative, so node M3 has no fault. After the above judgment, the microcomputer device obtains that the SPG fault occurs on the connecting line L4, and the line selection is correct.
Line selection scheme verification for neutral point grounded by arc suppression coil system
In the fault simulation model of the neutral point grounded by the ASC system, the ASC parameters are set according to the capacitance current of all feeders of the compensation node. If the condition of complete compensation is satisfied; that is,
When an SPG fault occurs, the ASC of the master station node M1 is tuned for the first time, and the over-compensation degree is increased to 15% of the entire closed-loop operation system. At this time, the inductance value of the ASC of the master station should be
The series resistance in the ASC is 10% of the inductance.
Figure 18 shows the change of ZSC of each line obtained according to the line selection strategy in Section 4.2 when an SPG fault occurs on feeder L5. When the system detects an SPG fault, the overcompensation degree of the ASC of the master station is adjusted to 15% of the system. The master station first judges the amplitude change of the ZSC at each end of the connecting line. The ZSC at the a and b ends of the connecting line changes significantly, as shown in Figure 18B, so it is determined that the feeder of node M2 has an SPG fault. The master station judges that there is no fault in the responsible area, sends a fault signal to the M2 slave station, and sends a no-fault signal to the M3 slave station. The slave station M2 receives the fault signal and obtains the ZSC amplitude difference of each feeder, as shown in Figure 18D. Because the fault line is first locked in the M2 node area, it is judged that the SPG fault occurs in the feeder L5 according to the maximum difference, and the line selection is correct.
Figure 18. The zero-sequence current of each line changes when the grounding fault occurs in L5. (A) Change of zero-sequence current amplitude at each end of the connecting line; (B) The difference of zero-sequence current at each end of the connecting line; (C) Change of zero-sequence current amplitude of each feeder; (D) Zero-sequence current difference of each feeder.
CONCLUSIONS
This paper proposes a complete line selection scheme for the closed-loop operation network formed by the MV microgrid connected to the MV distribution network under the low-current grounding mode and draws the following conclusions:
(1) After the medium voltage microgrid access to the distribution network, the radiation grid structure of the traditional distribution network is changed, resulting in its closed-loop operation. The formed closed-loop operation network has multi-level bus nodes and different bus nodes have nested relationships.
(2) The SPG fault characteristics of the closed-loop distribution network are more complicated. The traditional SPG line selection method represented by the method of group amplitude comparison and phase comparison (neutral point ungrounded) and the zero-sequence active component method (neutral point grounded by ASC) has the problem of line selection misjudgment when applied in the closed-loop distribution network.
(3) Based on the analysis of the fault characteristics of the closed-loop operation distribution network, the grounding fault line selection method is proposed for the closed-loop operation network with the neutral point ungrounded and the neutral point grounded by ASC. At the same time, the equipment configuration and communication scheme are designed in detail, which constitutes a complete set of automatic line selection systems. The simulation results show that the proposed line selection scheme can be effectively applied to the closed-loop operation distribution network with MV microgrid access. At the same time, the proposed line selection scheme is based on the two-level bus nodes, which can be analogously applied to the multi-level closed-loop operation distribution network.
In the future, the line selection scheme proposed in this paper can be further improved in the experiment to meet the needs of engineering applications.
DECLARATIONS
Authors’ contributionsFunding acquisition, project administration, provision of research resources, and supervision: Yin XG
Methodology, software, and writing-review editing: Lu Q, Liu Z
Data processing, investigation, methodology, software, visualization, and writing-original draft: Qiao J, Zhu L, Liu Z
Conceptualization, data acquisition, project supervision, and validation: Qiao J, Yin X
Writing-review editing: Yin X, Lu Q, Yin XG
Availability of data and materialsNot applicable.
Financial support and sponsorshipThis work was supported by the National Youth Foundation of China (No. 52007010).
Conflicts of interestAll authors declared that there are no conflicts of interest.
Ethical approval and consent to participateNot applicable.
Consent for publicationNot applicable.
Copyright© The Author(s) 2023.
REFERENCES
1. Cortes CA, Contreras SF, Shahidehpour M. Microgrid topology planning for enhancing the reliability of active distribution networks. IEEE Trans Smart Grid 2018;9:6369-77.
2. Yu X, Li H, Yang L, Li Q. Bi-level programming method for optimal sizing of grid-connected DC microgrid system based on economic efficiency of enterprises and customer electricity experience. J Electr Power Sci Technol 2020;35:38-45. (in Chinese). Available from: http://www.alljournals.cn/view_abstract.aspx?pcid=5B3AB970F71A803DEACDC0559115BFCF0A068CD97DD29835&cid=49C0C02D7521563C154D9F5E3C92CCDE&jid=8F04FF55B0ED2F735608831F572764EC&aid=C2816A21AE91BA8FE19EC1D84ECFA0CB&yid=0D1D160AB8016934. [Last accessed on 13 Sep 2023]
3. Planas E, Andreu J, Gárate JI, Martínez de Alegría I, Ibarra E. AC and DC technology in microgrids: a review. Renew Sust Energ Rev 2015;43:726-49.
4. Quan H, Utkarsh K, Srinivasan D. A distributed dual-optimization framework for ancillary-service coordination between MV microgrids and LV distribution networks. IEEE Syst J 2023;17:212-23.
5. Ghazanfari A, Hamzeh M, Mokhtari H, Karimi H. Active power management of multihybrid fuel cell/supercapacitor power conversion system in a medium voltage microgrid. IEEE Trans Smart Grid 2012;3:1903-10.
6. Mohammadi J, Badrkhani Ajaei F, Stevens G. Grounding the AC microgrid. IEEE Trans Ind Appl 2019;55:98-105.
7. Zhang Z, Xu B, Crossley P, Li L. Positive-sequence-fault-component-based blocking pilot protection for closed-loop distribution network with underground cable. Int J Elec Power 2018;94:57-66.
8. Gan G, Wang Z, Zhou Y, Li L, Li X. Reliability and economy based closed-loop operation modes for medium voltage distribution networks. Autom Electric Power Syst 2015;39:144-50.
9. Shen W, Jiang X. Development status of fault line selection and location in distribution network. Electronic Test 2022;3:129-31. (in Chinese).
10. Du D, Xu Y. Faulted line detecting with active power in an auto-compensated distribution net work. Relay 2002;30:33-6. (in Chinese). Available from: https://kns.cnki.net/kcms/detail/detail.aspx?FileName=JDQW200205009&DbName=CJFQ2002. [Last accessed on 13 Sep 2023].
11. Li Z, Wang W, Zhuang L, Zhou P, Li S. Dynamic test of traveling wave line selecting device based on RTDS small time-step simulation. Power Syst Protection Control 2019;47:108-14. (in Chinese).
12. Liu B, Zeng X, Zhang H, Ma H. Distribution model of injection current in feeder and its application in single phase to ground fault detection. Power Syst Technol 2021;45:2731-40. (in Chinese).
13. Wei X, Yang D, Wang X, Wang B, Gao J, Wei K. Faulty feeder detection based on fundamental component shift and multiple-transient-feature fusion in distribution networks. IEEE Trans Smart Grid 2021;12:1699-711.
14. Cai Y, Zhang S, Yang Y, Hua H. Analysis on relay protection setting and operation of 20 kV loop network. Guangdong Electric Power 2016;29:64-9.
15. Papaspiliotopoulos VA, Korres GN, Kleftakis VA, Hatziargyriou ND. Hardware-in-the-loop design and optimal setting of adaptive protection schemes for distribution systems with distributed generation. IEEE Trans Power Delivery 2017;32:393-400.
16. Chen X, Yuan S, Li Y, Li Z, Wang Q, Geng S. Analysis of single-phase grounding fault characteristics in petal-shaped distribution network with inverter-interfaced distributed generator. Electric Power Autom Equip 2022;42:129-37. (in Chinese).
17. Liu X. Research on line selection method for single-phase grounding fault in complex distribution network. China Univ Mining Technol 2019. (in Chinese). Available from: https://kns.cnki.net/kcms/detail/detail.aspx?FileName=1019854670.nh&DbName=CMFD2019. [Last accessed on 13 Sep 2023].
18. Li Z, Wu Q, Zheng Y, et al. Distance protection scheme for island grid based on control combined with protection under single-phase ground fault. Proceedings of the CSEE 2022. (in Chinese). Available from: https://kns.cnki.net/kcms/detail/11.2107.TM.20221020.1500.007.html. [Last accessed on 13 Sep 2023]
19. Zhao H, Mu L, Fang C. Grounding fault protection scheme based on zero-sequence off-nominal frequency component injection for microgrid. Electric Power Autom Equip 2022;42:25-31. (in Chinese).
20. He L, Li Y, Chu X, Shuai Z, Peng Y, Shen ZJ. Single-phase to ground fault line identification for medium voltage islanded microgrids with neutral ineffectively grounded modes. IEEE Trans Smart Grid 2022;13:4312-26.
21. Jia Z. Research on Micro-grid’s grounding method and protection configuration. North China Electric Power University 2016. (in Chinese). Available from: https://kns.cnki.net/kcms2/article/abstract?v=BaYza4XbGEIrWkmfeBORNvY_gVVpg6VWdC1fojNgfTFk-fZKiRQx0i_IRePJmfG7sbV7-aDIpDGpAAr4OvGyNII6uhRNVRyNTNpN3-CxnNNPsM2F3CYFCn5PU97qFPOI9YEn2yIt7go=&uniplatform=NZKPT&language=CHS. [Last accessed on 13 Sep 2023].
22. Yang X, Su J, LÜ Z, Liu H, Li R. Overview on micro-grid technology. Proceedings of the CSEE 2014;34:57-70.
23. Zhao Z, Yang P, Cai Z, Zhou S, Green TC. Cooperative control of transient voltage stability for islanded medium-voltage microgrid with wind power. Electric Power Autom Equip 2015;35:1-9. (in Chinese).
24. 1547-2003 - IEEE standard for interconnecting distributed resources with electric power systems. Available from: https://ieeexplore.ieee.org/document/1225051. [Last accessed on 13 Sep 2023]
Cite This Article
How to Cite
Download Citation
Export Citation File:
Type of Import
Tips on Downloading Citation
Citation Manager File Format
Type of Import
Direct Import: When the Direct Import option is selected (the default state), a dialogue box will give you the option to Save or Open the downloaded citation data. Choosing Open will either launch your citation manager or give you a choice of applications with which to use the metadata. The Save option saves the file locally for later use.
Indirect Import: When the Indirect Import option is selected, the metadata is displayed and may be copied and pasted as needed.
About This Article
Copyright
Data & Comments
Data
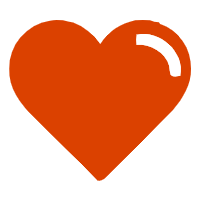
Comments
Comments must be written in English. Spam, offensive content, impersonation, and private information will not be permitted. If any comment is reported and identified as inappropriate content by OAE staff, the comment will be removed without notice. If you have any queries or need any help, please contact us at [email protected].