Climate change - mediated atmospheric 210Po and 210Pb distribution: How significant can it be for the inhalation dose to humans?
Abstract
The concentration of 210Po and 210Pb in vegetation, and their transfer from soil to vegetation and via aerosol deposited on foliage are well established. The available data show significantly higher levels of these radionuclides in ash from forest fires and aerosols downwind from industrial sites. The climate change-induced hot and dry conditions promote the spread of forest fires, which burn huge areas. On average, over 10 million hectares are reportedly lost annually. Large-scale forest fires and fossil-fuel and coal-operated Power and Desalination Plants are very likely to result in the dispersion of 210Pb and 210Po into the regional aerosol; such an effect has already been observed due to fires in Ukraine, Belarus, and Russia, which have led to the dispersion of 137Cs over large parts of Europe. We have measured elevated levels in Kuwait, and similar observations have been reported from Portugal. The higher levels of 210Po in PM2.5 raise a serious concern about an increased inhalation dose humans could receive. Our estimate shows that humans in areas affected by forest fires might receive a dose equivalent to 2 µSv d-1, which is significantly higher than 0.099 µSv d-1, the dose a person gets from smoking a packet of cigarettes daily. We propose that size-fractionated aerosol sampling should be taken up in regions affected by forest fires and industrial activities that add 210Po to the atmosphere in order to obtain a robust inhalation dose assessment and issue informed advisories to the public.
Keywords
INTRODUCTION
The ubiquitous presence of 238U and 232Th in the environment and vegetation has been reported. Polonium-210 and lead-210 are naturally occurring radioactive isotopes and are part of the uranium radioactive decay series. Polonium-210 has a 138-day half-life, while radioactive lead-210 has a much longer half-life of 22.2 years. Both 210Po and 210Pb are produced as part of the decay chain of radon gas (222Rn), which itself has a half-life of 3.8 days[1]. Radon, a noble gas, has a neutral electric charge, but its radioactive daughters, 210Po and 210Pb, are positively charged ions. These ions are highly reactive and quickly attach to airborne particles. Polonium-210 ions in the atmosphere rapidly become attached to aerosol particles within a relatively short time frame, ranging from 40 to 180 seconds after their formation from the radioactive decay of precursor radon[2]. This attachment to particles is a key factor in their atmospheric dispersion. Dry and wet atmospheric depositions continuously remove radon daughters from the atmosphere. This removal process prevents the establishment of a secular radioactive equilibrium between radon and its progeny, including
The 210Po/210Pb ratio in the aerosols is generally much lower than 0.1 due to the ongoing removal of radon daughters from the atmosphere[3,4]. Consequently, the concentration of 210Po is typically much lower than that of 210Pb in aerosol samples. This aspect highlights the dynamic behavior of these radionuclides in the atmosphere and their connection to the decay of radon gas, leading to a significant radioactive disequilibrium between 210Po and 210Pb in natural settings[5]. This disequilibrium is vital to consider when studying environmental radioactivity, including the mean residence time of aerosols (210Bi/210Pb ratios for recent air mass up to a few days[5,6], and 210Po/210Pb for older air mass of a month or more[7,8]) and their potential health effects[9,10].
While the radioactive decay of atmospheric radon is one source of 210Po and 210Pb, later research has identified additional sources, including volcanic emissions, industrial facilities, forest fires, coal burning, and nuclear weapons tests[11-17]. These sources can release significant quantities of 210Po and sometimes 210Pb into the atmosphere. Fossil fuel and coal-based Power and Desalination plants and Oil refineries are known to contribute to significantly higher concentrations of 210Po and 210Pb[18,19]. Importantly, these additional sources can significantly increase the 210Po/210Pb ratios in the atmosphere[14,17]. Both 210Po and 210Pb can be inhaled when they are adhered to ultra-fine particles in the air. This type of exposure to these radionuclides can result in internal radiation doses to human beings [Figure 1], particularly from 210Po, which is generally higher than other naturally occurring radionuclides, except for radon[20]. The activity-to-dose conversion factor is 2.2 µSv/Bq for inhaled 210Po[21], which is higher than other naturally occurring radionuclides, including 210Pb.
The redistribution of 137Cs was first noticed in Europe after the extensive forest fires in Ukraine, Belarus, and Russia during 2010[22]. However, not much attention has been given to natural radionuclides associated with vegetation that are released into the atmosphere during forest fires[14,23]. Given the potential health risks associated with exposure to 210Po and 210Pb, monitoring their presence and redistribution in the environment consequent to forest fires is crucial. With an increase in the frequency of forest fires globally, mainly due to climate change, about 9.3 × 106 hectares of tree cover were lost in 2021, and Russia alone lost 5.4 × 106 hectares of tree cover to fire in 2021[24]. The more recent statistics are even more striking; the Forest Fire Centre, Canada estimated an area of 9.5 × 106 hectares was burned in the seven months of 2023, between January and July, keeping in view the recurrence and increasing spatial scale of the forest fires, it is quite likely that the activity concentration of 210Po, 210Pb and other radionuclides could be considerably enhanced in the surface air over areas beyond the charred forests.
RESULTS AND DISCUSSION
Certain investigations have documented 210Po/210Pb ratios surpassing one. These escalated ratios are frequently linked to the release of 210Po from human-made sources involving high temperatures, such as metal smelters, ceramic kilns, incinerators, and forest fires[2,3,10,17,25-27]. Studies analyzing radionuclides in aerosols have revealed that a significant amount of polonium activity is associated with fine and ultrafine aerosol particles. In Japan, over 70% of 210Po activity in aerosols was detected in particles smaller than
Several studies have highlighted the concentration of 210Po and 210Pb in vegetation [Table 1] and aerosols [Table 2]. The concentration in vegetation samples exhibited 210Pb concentrations ranging from 0.98 to 20.27 Bq kg-1 and 210Po concentrations ranging from 0.97 to 49.4 Bq kg-1, where the aerosol-containing combusted particles showed a significant increase in 210Po and 210Pb concentrations. A significant variation in 210Po levels was observed in non-fire impacted aerosol in which the 210Po on the filter sample was
210Pb and 210Po concentration (in Bq kg-1 dry weight) in various plants, and soil
Country | Sample | 210Pb | 210Po | Reference |
Viseu region, North Portugal, (late summer 2012) | Citrus bushes | 9.90 ± 0.35 | 12.0 ± 2.4 | [16] |
Oak tree trunk wood | 3.27 ± 0.16 | 5.51 ± 0.02 | ||
Oak tree, leaves | 17.2 ± 0.4 | 30.8 ± 1.2 | ||
Eucalyptus, trunk wood | 0.98 ± 0.03 | 1.68 ± 0.05 | ||
Eucalyptus bark | 1.88 ± 0.09 | 2.60 ± 0.06 | ||
Eucalyptus leaves | 10.3 ± 0.4 | 49.4 ± 2.3 | ||
Acacia tree, trunk wood | 2.04 ± 0.05 | 4.05 ± 0.15 | ||
Acacia tree, leaves | 20.27 ± 0.47 | 8.61 ± 0.33 | ||
Pine tree trunk wood | 1.43 ± 0.13 to 1.98 ± 0.09 | 0.97 ± 0.003 to 1.53 ± 0.006 | ||
Pine tree, bark | 2.80 ± 0.08 | 2.87 ± 0.06 | ||
Pine tree, needle (leaves) | 10.36 ± 0.31 | 3.10 ± 0.08 | ||
Ashes from the ground after forest fire | 402 ± 6 | 1115 ± 66 | ||
Size fractionated aerosol (Fly ash) | 923 ± 53 to 2070 ± 88 | 3604 ± 148 to 7255 ± 285 | ||
Aerosol (without fire smoke) | 5895 ± 218 | 114 ± 7 | ||
Wyoming, USA | Soil | 0.1073 ± 0.02 to 3.108 ± 0.24 | 0.078 ± 0.009 to 2.997 ± 0.333 | [38] |
Sagebrush | 0.016 ± 0.006 to 0.051 ± 0.034 | 0.022 ± 0.003 to 0.198 ± 0.107 | ||
Mixed grasses | 0.020 ± 0.008 to 0.481 ± 0.159 | 0.020 ± 0.004 to 0.355 ± 0.112 | ||
Mixed Forbs | 0.031 ± 0.003 to 0.322 ± 0.199 | 0.008 ± 0.001 to 0.777 ± 0.249 | ||
All plants | 0.021 ± 0.003 to 0.444 ± 0.126 | 0.017 ± 0.003 to 0.577 ± 0.145 | ||
Portugal | Cabbage leaves | 0.435 | 0.044 | [16] |
Maize aerial parts | 0.304 | 0.217 | ||
Olive tree leaves | 22.381 | 2.50 | ||
Olive tree trunk wood | 10.24 | 0.333 | ||
Olive tree roots | 3.333 | 2.619 | ||
Palm tree leaves | 12.857 | 2.548 | ||
Palm Tree bark | 0.786 | 0.214 | ||
Tobacco leaves (cured) | 11.90 | 11.19 | ||
Soil (0 - 30 cm) | 100 | 100 |
210Pb and 210Po concentration (in Bq kg-1 dry weight) in aerosols
Country | Sample | 210Pb | 210Po | Reference |
Viseu region, North Portugal, (late summer 2012) | Size fractionated aerosol (Fly ash) | 923 ± 53 to 2070 ± 88 | 3604 ± 148 to 7255 ± 285 | [16] |
Aerosol (without fire smoke) | 5895 ± 218 | 114 ± 7 | ||
Kuwait, January 2018 - November 2019 | Aerosol PM ≥ 10 (R,Su) | 228 – 279 | [19, 29, 41] | |
Aerosol PM ≥ 10 (U, Su) | 252 - 288 | |||
Aerosol PM ≥ 10 (I, Su) | 370 - 406 | |||
Aerosol PM ≥ 10 (R, Au) | 199 - 210 | |||
Aerosol PM ≥ 10 (U, Au) | 207 – 245 | |||
Aerosol PM ≥ 10 (I, Au) | 311 - 344 | |||
Aerosol PM ≥ 10 (R, Wi) | 121 - 176 | |||
Aerosol PM ≥ 10 (U, Wi) | 133 - 190 | |||
Aerosol PM ≥ 10 (I, Wi) | 288 – 301 | |||
Aerosol PM ≥ 10 (R, Sp) | 155 - 193 | |||
Aerosol PM ≥ 10 (U, Sp) | 170 - 198 | |||
Aerosol PM ≥ 10 (I, Sp) | 305 - 336 | |||
Aerosol PM2.5 - 10 (R,Su) | 303 - 342 | |||
Aerosol PM2.5 - 10 (U, Su) | 288 - 326 | |||
Aerosol PM2.5 - 10 (I, Su) | 406 – 411 | |||
Aerosol PM2.5 - 10 (R, Au) | 265 – 278 | |||
Aerosol PM2.5 - 10 (U, Au) | 294 - 451 | |||
Aerosol PM2.5 - 10 (I, Au) | 349 - 387 | |||
Aerosol PM2.5 - 10 (R, Wi) | 190 - 223 | |||
Aerosol PM2.5 - 10 (U, Wi) | 252 - 284 | |||
Aerosol PM2.5 - 10 (I, Wi) | 330 – 335 | |||
Aerosol PM2.5 - 10 (R, Sp) | 220 - 251 | |||
Aerosol PM2.5 - 10 (U, Sp) | 252 - 284 | |||
Aerosol PM2.5 - 10 (I, Sp) | 352 - 403 | |||
Aerosol PM0.39 - 2.5 (R,Su) | 515 – 596 | |||
Aerosol PM0.39 - 2.5 (U, Su) | 705 - 746 | |||
Aerosol PM0.39 - 2.5 (I, Su) | 944 - 960 | |||
Aerosol PM0.39 - 2.5 (R, Au) | 481 - 502 | |||
Aerosol PM0.39 - 2.5(U, Au) | 631 - 698 | |||
Aerosol PM0.39 -2.5 (I, Au) | 903 - 913 | |||
Aerosol PM0.39 - 2.5(R, Wi) | 435 - 436 | |||
Aerosol PM0.39 - 2.5(U, Wi) | 572 - 661 | |||
Aerosol PM0.39 - 2.5 (I, Wi) | 863 - 904 | |||
Aerosol PM0.39 - 2.5 (R, Sp) | 466 - 479 | |||
Aerosol PM0.39 - 2.5 (U, Sp) | 606 - 700 | |||
Aerosol PM0.39 - 2.5 (I, Sp) | 896 - 897 | |||
Vienna, Austria* | Aerosol PM0.15 - 0.3 (23.04.1996) | 7.5 ± 0.4 | 1.5 ± 0.17 | [42] |
Aerosol PM0.3 - 0.6 (23.04.1996) | 17.4 ± 0.7 | 1.8 ± 0.17 | ||
Aerosol PM0.6 - 1.2 (23.04.1996) | 14.6 ± 1.0 | 2.2 ± 0.2 | ||
Aerosol PM1.2 - 2.4 (23.04.1996) | 3.85 ± 0.3 | 0.2 ± 0.03 | ||
Aerosol PM2.4 - 5.0 (23.04.1996) | 1.9 ± 0.2 | |||
Aerosol PM5.0 - 10 (23.04.1996) | 0.88 ± 0.1 | |||
Aerosol PM0.15 - 0.3 (12.06.1996) | 9.3 ± 0.5 | 0.55 ± 0.08 | ||
Aerosol PM0.3 - 0.6 (12.06.1996) | 15 ± 0.7 | 0.5 ± 0.08 | ||
Aerosol PM0.6 - 1.2 (12.06.1996) | 15.8 ± 0.7 | 0.52 ± 0.08 | ||
Aerosol PM1.2 - 2.4 (12.06.1996) | 3.15 ± 0.2 | |||
Aerosol PM2.4 - 5.0 (12.06.1996) | 0.95 ± 0.1 | 0.2 ± 0.04 | ||
Aerosol PM0.15 - 0.3 (12.08.1996) | 12.4 ± 0.5 | 1.33 ± 0.15 | ||
Aerosol PM0.3 - 0.6 (12.08.1996) | 13.7 ± 0.7 | 0.7 ± 0.1 | ||
Aerosol PM0.6 - 1.2 (12.08.1996) | 6.5 ± 0.4 | |||
Aerosol PM1.2 - 2.4 (12.08.1996) | 1.7 ± 0.2 | |||
Aerosol PM0.15 - 0.3 (2.10.1996) | 3.3 ± 0.40 | 0.19 ± 0.12 | ||
Aerosol PM0.3-0.6 (2.10.1996) | 5.5 ± 0.50 | 0.62 ± 0.15 | ||
Aerosol PM0.6 - 1.2 (2.10.1996) | 4.8 ± 0.50 | 0.19 ± 0.12 | ||
Aerosol PM1.2 - 2.4 (2.10.1996) | 0.7 ± 0.30 | 0.17 ± 0.13 | ||
Aerosol PM2.4 - 5.0 (2.10.1996) | 0.25 ± 0.23 | 0.24 ± 0.14 | ||
Aerosol PM0.15 - 0.3 (13.11.1996) | 7.5 ± 0.4 | 12.3 ± 0.5 | ||
Aerosol PM0.3 - 0.6 (13.11.1996) | 10.5 ± 0.6 | 2 ± 0.2 | ||
Aerosol PM0.6 - 1.2 (13.11.1996) | 10.2 ± 0.6 | 0.7 ± 0.1 | ||
Aerosol PM1.2 - 2.4 (13.11.1996) | 1.2 ± 0.1 | 0.2 ± 0.04 | ||
Aerosol PM2.4 - 5.0 (13.11.1996) | 1.9 ± 0.2 | 0.2 ± 0.04 | ||
Aerosol PM5.0 - 10 (13.11.1996) | 0.7 ± 0.1 | 0.07 ± 0.02 | ||
Aerosol PM0.15 - 0.3 (17.12.1996) | 10.8 ± 0.6 | 0.5 ± 0.2 | ||
Aerosol PM0.3 - 0.6 (17.12.1996) | 25 ± 0.9 | 0.9 ± 0.2 | ||
Aerosol PM0.6 - 1.2 (17.12.1996) | 38.8 ± 1.3 | 0.8 ± 0.2 | ||
Aerosol PM1.2 - 2.4 (17.12.1996) | 20 ± 0.9 | 0.5 ± 0.1 | ||
Aerosol PM2.4 - 5.0 (17.12.1996) | 1.2 ± 0.3 | 0.1 ± 0.1 | ||
Aerosol PM5.0 - 10 (17.12.1996) | 5.8 ± 0.4 | 0.1 ± 0.1 | ||
Aerosol PM0.15 - 0.3 (12.02.1997) | 4.8 ± 0.3 | 0.69 ± 0.1 | ||
Aerosol PM0.3 - 0.6 (12.02.1997) | 7.7 ± 0.4 | 1.45 ± 0.15 | ||
Aerosol PM0.6 - 1.2 (12.02.1997) | 5.8 ± 0.4 | 0.33 ± 0.05 | ||
Aerosol PM1.2 - 2.4 (12.02.1997) | 1.3 ± 0.1 | 0.26 ± 0.05 | ||
Aerosol PM2.4 - 5.0 (12.02.1997) | 1.1 ± 0.1 | |||
Aerosol PM0.15 - 0.3 (11.03.1997) | 6.4 ± 0.4 | 1.4 ± 0.2 | ||
Aerosol PM0.3 - 0.6 (11.03.1997) | 11.8 ± 0.6 | 1.4 ± 0.2 | ||
Aerosol PM0.6 - 1.2 (11.03.1997) | 7.2 ± 0.4 | 0.98 ± 0.18 | ||
Aerosol PM1.2 - 2.4 (11.03.1997) | 1.7 ± 0.2 | 0.3 ± 0.05 | ||
Aerosol PM2.4 - 5.0 (11.03.1997) | 0.4 ± 0.04 | |||
Aerosol PM0.15 - 0.3 (29.04.1997) | 3.6 ± 0.3 | |||
Aerosol PM0.3 - 0.6 (29.04.1997) | 5.9 ± 0.4 | 0.12 ± 0.03 | ||
Aerosol PM0.6 - 1.2 (29.04.1997) | 3.3 ± 0.3 | |||
Aerosol PM1.2-2.4 (29.04.1997) | 1.2 ± 0.1 | |||
Aerosol PM2.4 - 5.0 (29.04.1997) | 1.8 ± 0.2 | |||
Aerosol PM0.15 - 0.3 (21.05.1997) | 3.3 ± 0.25 | |||
Aerosol PM0.3 - 0.6 (21.05.1997) | 5.9 ± 0.4 | 0.14 ± 0.03 | ||
Aerosol PM0.6 - 1.2 (21.05.1997) | 4 ± 0.28 | 0.07 ± 0.02 | ||
Aerosol PM1.2 - 2.4 (21.05.1997) | 0.8 ± 0.08 | |||
Aerosol PM2.4 - 5.0 (21.05.1997) | 0.2 ± 0.02 | |||
Badgastein, Austria* | Aerosol PM0.15 - 0.3 (09.01.1999) | 9.5 ± 0.5 | 1.7 ± 0.2 | |
Aerosol PM0.3 - 0.6 (09.01.1999) | 7.9 ± 0.4 | |||
Aerosol PM0.6 - 1.2 (09.01.1999) | 4.7 ± 0.3 | |||
Aerosol PM1.2 - 2.4 (09.01.1999) | 0 | |||
Aerosol PM0.15 - 0.3 (28.05.1999) | 14.3 ± 0.6 | 0.85 ± 0.10 | ||
Aerosol PM0.3 - 0.6 (28.05.1999) | 24.9 ± 0.9 | 1.04 ± 0.20 | ||
Aerosol PM0.6 - 1.2 (28.05.1999) | 11.5 ± 0.6 | 0.76 ± 0.10 | ||
Aerosol PM1.2 - 2.4 (28.05.1999) | 1.7 ± 0.1 | |||
Stubnerkogel, Austria* | Aerosol PM0.15 - 0.3 (25.05.1999) | 22.8 ± 0.9 | 4.1 ± 0.3 | |
Aerosol PM0.3 - 0.6 (25.05.1999) | 11.3 ± 0.6 | 1.5 ± 0.2 | ||
Aerosol PM0.6 - 1.2 (25.05.1999) | 7.8 ± 0.5 | 1.2 ± 0.1 | ||
Aerosol PM1.2 - 2.4 (25.05.1999) | 1.1 ± 0.1 | 0.5 ± 0.1 | ||
Aerosol PM0.15 - 0.3 (27.05.1999) | 8.9 ± 0.5 | 1 ± 0.12 | ||
Aerosol PM0.3 - 0.6 (27.05.1999) | 10.5 ± 0.5 | 0.6 ± 0.08 | ||
Aerosol PM0.6 - 1.2 (27.05.1999) | 9 ± 0.5 | 0.6 ± 0.08 | ||
Aerosol PM1.2 - 2.4 (27.05.1999) | 0.9 ± 0.1 |
Since 210Po and 210Pb are non-essential for any growth and metabolic functions in plants, a detailed study has provided data to underpin the hypothesis that both 210Po and 210Pb are taken up by plants from soil[37]. In spite of the restrictive uptake of potentially toxic elements by plants in metalliferous soils, 210Po and 210Pb accumulation has been observed in both metal-tolerant and non-tolerant plants[38]. 210Pb and 210Po enter the vegetation through root uptake and aerial deposition on foliage[39].
The evidence of the accumulation of these radionuclides in vegetation is explicit. The edibles (fruits and vegetables) are likely to contribute to the internal radiation dose to consumers. The data are compelling that 210Po and 210Pb are significantly incorporated in these fruits and vegetables, which follow a non-linear uptake pattern mathematically. Plant concentration for 210Pb is expressed as
while for 210Po, the concentration is expressed as:
The data depict that bioaccumulation takes place at even lower substrate concentrations and 210Po accumulation exceeds 210Pb by about a factor of 2[37]. The much higher 210Po and 210Pb concentrations in aerosols emanating from forest fires, power and desalination plants, and oil installations highlight the considerable dose humans can get from inhalation. The issue is exacerbated given that frequent forest fires are linked to climate change. An extensive area is lost to forest fires year after year globally, most recently in Australia, Canada, Spain, Belarus, Russia, Portugal, and Turkiye. A very detailed spatial distribution of forest fires[24] provides an overview of approximately 10 million hectares of forest lost yearly.
It will be quite imperative to conduct aerosol sampling and define the concentration of these radionuclides in size-fractionated aerosols. We have found a six-stage cascade impactor mounted on a high-volume air sampler to be very effective in determining radioactivity in respirable and inhalable fractions[19,29,40,41]. The likelihood of volatilization of 210Po in forest fires is relatively high as temperatures above 1,000 oC have been reported from forest fires[17]. The considerable reduction of volume due to fire and the positive charge of gaseous 210Po can result in the recapture of 210Po and 210Pb on ash particles, resulting in higher concentrations of these radionuclides in ash and aerosols.
The highest concentration of 210Po in the vicinity of forest fires and downwind industrial sites is associated with PM0.39 - 2.5 and PM2.5-10 sizes. Considering an average breathing rate of 6 L min-1, an adult breathes about 8.64 m3 daily. The 210Po concentration in forest fires in Portugal was reported as 70 mBq m-3, resulting in an inhalation rate of 0.605 Bq d-1. Using the dose conversion factor of 3.3 × 10-6 Sv Bq-1, a 2 µSv d-1 dose is imparted due to inhalation; although it is not a permanent event, it can extend for weeks and months. It is interesting to put it in perspective: an individual who smokes a packet of cigarettes daily gets about 0.099 µSv d-1, which is significantly lower than the population exposure during forest fires.
CONCLUSION
Forest fires play a significant role in the redistribution of 210Po and 210Pb. These fires not only release these isotopes into the atmosphere but also facilitate their transport over considerable distances. The combustion of organic matter during forest fires liberates substantial quantities of 210Po and 210Pb into the air, where they can attach to aerosol particles. Studies have shown that these isotopes often exhibit elevated concentration ratios in the aftermath of forest fires, surpassing unity in some instances.
The resultant aerosols, laden with 210Po and 210Pb, disperse throughout the atmosphere. Fine and ultrafine aerosol particles become carriers for a significant portion of these isotopes. Research has indicated that a substantial percentage, sometimes over 70%, of 210Po activity in aerosols is associated with particles smaller than 2.5 µm. The ultrafine particles are reported to have the highest levels of these isotopes post-forest fires. This pattern aligns with observations not only in forests but also in areas affected by other combustion sources, such as industrial emissions across different geographical locations. The highest concentrations are observed in inhalable and respirable fractions of the aerosols that are more likely to enhance the radiation dose humans receive due to inhalation, i.e., the ~2 µSv d-1 dose from forest fires and ~ 0.002 - 0.042 µSv d-1 dose from industrial emissions. From the dose perspective, the climate change-mediated fires and increase in fossil fuel and coal-based power generation are likely to result in the redistribution of 210Po and 210Pb and impart a dose that is several orders of magnitude higher than the normal background doses and likely to significantly contribute to the 1 mSv permissible annual dose.
Understanding the dynamics of 210Po and 210Pb redistribution in the aftermath of forest fires is crucial for comprehending their environmental impact and potential implications for human health. Tracking the dispersion of these radionuclides and their attachment to aerosols can shed light on the broader implications of these natural events on atmospheric radioactivity and the subsequent exposure risks to ecosystems and populations downwind from these fire-affected regions.
DECLARATIONS
Acknowledgments
The authors appreciate the Kuwait Institute for Scientific Research’s support for project EM090C and the Kuwait Foundation for Advancements of Sciences for partially supporting the study PR18-14SE-01. The authors would also like to thank the Institute of Nuclear Sciences, EGE University, Turkiye for supporting the aerosol study.
Authors’ contributions
Conceptualized and designed the study: Uddin S
Performed data analysis: Gorgun AU, Behbehani M, Habibi N
Helped with the interpretation: Fowler SW , Filizok I
Done the data acquisition: Uddin S, Behbehani M
Provided the technical and material support: Fowler SW, Al-Murad M, Uddin S
Availability of data and materials
Not applicable.
Financial support and sponsorship
Kuwait Institute for Scientific Research funding for project EM090C and Kuwait Foundation for Advancement of Sciences grant PR18-14SE-01. The funding bodies had no role in the experiment design, collection, analysis and interpretation of data, and writing of the manuscript.
Conflicts of interest
All authors declared that there are no conflicts of interest.
Ethical approval and consent to participate
Not applicable.
Consent for publication
Not applicable.
Copyright
© The Author(s) 2023.
REFERENCES
1. Baskaran M. Radon: a tracer for geological, geophysical and geochemical studies. Springer Cham; 2016. p. 260.
2. Długosz-Lisiecka M. Excess of 210polonium activity in the surface urban atmosphere. Part (1) fluctuation of the 210Po excess in the air. Environ Sci Process Impacts 2015;17:458-64.
3. Długosz-Lisiecka M. The sources and fate of 210Po in the urban air: A review. Environ Int 2016;94:325-30.
4. UNSCEAR report. Sources, effects and risks of ionizing radiation. Available from: https://www.unscear.org/unscear/en/publications/1988.html [Last accessed on 27 Dec 2023].
5. Poet SE, Moore HE, Martell EA. Lead 210, bismuth 210, and polonium 210 in the atmosphere: Accurate ratio measurement and application to aerosol residence time determination. J Geophys Res 1972;77:6515-27.
6. Papastefanou C, Bondietti E. Mean residence times of atmospheric aerosols in the boundary layer as determined from 210Bi/210Pb activity ratios. J Aerosol Sci 1991;22:927-31.
7. Peirson DH, Cambray RS, Spicer GS. Lead-210 and polonium-210 in the atmosphere. Available from: http://www.gammaexplorer.com/wp-content/uploads/2014/03/9381-31656-1-PB.pdf [Last accessed on 27 Dec 2023].
8. Nevissi A, Beck JN, Kuroda PK. Long-lived radon daughters as atmospheric radioactive tracers. Health Phys 1974;27:181-8.
9. Semertzidou P, Piliposian GT, Appleby PG. Atmospheric residence time of 210Pb determined from the activity ratios with its daughter radionuclides 210Bi and 210Po. J Environ Radioactiv 2016;160:42-53.
10. Baskaran M. Po-210 and Pb-210 as atmospheric tracers and global atmospheric Pb-210 fallout: a review. J Environ Radioact 2011;102:500-13.
11. Carvalho FP. Origins and concentrations of 222Rn, 210Pb, 210Bi and 210Po in the surface air at Lisbon, Portugal, at the Atlantic edge of the European continental landmass. Atmospheric Environment 1995;29:1809-19.
12. Ram K, Sarin M. Atmospheric 210Pb, 210Po and 210Po/210Pb activity ratio in urban aerosols: temporal variability and impact of biomass burning emission. Tellus B: Chemical and Physical Meteorology 2012;64:17513.
13. Yi Y, Zhou P, Liu G. Atmospheric deposition fluxes of 7Be, 210Pb and 210Po at Xiamen, China. J Radioanal Nucl Chem 2007;273:157-62.
14. Carvalho FP, Oliveira JM, Malta M. Vegetation fires and release of radioactivity into the air. WIT Trans Biomed Health 2011;15:3-9.
15. Carvalho FP, Oliveira JM, Malta M. Exposure to forest fires, radioactivity and health risks. 8th International Symposium on Occupational Safety and Hygiene (SHO); 2012 126-130. Available from: https://hero.epa.gov/hero/index.cfm/reference/details/reference_id/2648989 [Last accessed on 22 Dec 2023].
16. Uddin S, Fowler SW, Behbehani M. 210Po in the environment: reassessment of dose to humans. Sustainability 2023;15:1674.
17. Carvalho FP, Oliveira JM, Malta M. Exposure to radionuclides in smoke from vegetation fires. Sci Total Environ 2014;472:421-4.
18. Behbehani M, Carvalho FP, Uddin S, Habibi N. Enhanced polonium concentrations in aerosols from the gulf oil producing region and the role of microorganisms. Int J Environ Res Public Health 2021;18:13309.
19. Behbehani M, Uddin S, Baskaran M. 210Po concentration in different size fractions of aerosol likely contribution from industrial sources. J Environ Radioact 2020;222:106323.
20. UNSCEAR. Sources and effects of ionizing radiation. Available from: https://www.unscear.org/unscear/en/publications/2008_1.html [Last accessed on 22 Dec 2023].
21. Eckerman K, Harrison J, Menzel H, Clement C. ICRP publication 119: compendium of dose coefficients based on ICRP publication 60. Ann ICRP 2013;42:1-130.
22. Evangeliou N, Balkanski Y, Cozic A, Hao WM, Møller AP. Wildfires in chernobyl-contaminated forests and risks to the population and the environment: a new nuclear disaster about to happen? Environ Int 2014;73:346-58.
23. Bae MS, Shin JS, Lee KY, Lee KH, Kim YJ. Long-range transport of biomass burning emissions based on organic molecular markers and carbonaceous thermal distribution. Sci Total Environ 2014;466-467:56-66.
24. MacCarthy J, Richter J, Tyukavina S, Weisse M, Harris N. The latest data confirms: forest fires are getting worse. Available from: https://www.wri.org/insights/global-trends-forest-fires [Last accessed on 22 Dec 2023].
25. Kim G, Hong YL, Jang J, Lee I, Hwang DW, Yang HS. Evidence for anthropogenic 210Po in the urban atmosphere of Seoul, Korea. Environ Sci Technol 2005;39:1519-22.
26. Tadmor J. Radioactivity from coal-fired power plants: a review. J Environ Radioactiv 1986;4:177-204.
27. Długosz-Lisiecka M. Excess of polonium-210 activity in the surface urban atmosphere. Part 2: origin of 210Po excess. Environ Sci Process Impacts 2015;17:465-70.
28. Suzuki T, Maruyama Y, Nakayama N, Yamada K, Ohta K. Measurement of the 210Po/210Pb activity ratio in size fractionated aerosols from the coast of the Japan sea. Atmospheric Environment 1999;33:2285-8.
29. Behbehani M, Uddin S. Atmospheric concentrations of 210Pb, 210Bi and 210Po in Kuwait. Available from: http://kdrviewer.kisr.edu.kw/BookViewer/?book_id=7205&keyword=Atmospheric%20Concentrations%20of%20210Pb,%20210Bi%20and%20210Po%20in%20Kuwait [Last accessed on 22 Dec 2023].
30. Carvalho FP. Tobacco smoking, indoor exposure and the lung radioactivity. Proceedings of the SHO2009: International Symposium on Occupational Safety and Hygiene; Guimaraes, Portugal. 5–6 February 2009; pp. 131–135.
31. Uddin S, Behbehani M, Fowler SW, Al-Ghadban A, Dupont S. Assessment of loss of 210Po from fish and shrimp by cooking and its effect on dose estimates to humans ingesting seafood. J Environ Radioact 2019;205-6:1-6.
32. Carvalho FP, Oliveira JM. Polonium in cigarette smoke and radiation exposure of lungs. Czech J Phys 2006;56:D697-703.
33. Uddin S, Habibi N, Fowler SW, et al. Aerosols as vectors for contaminants: a perspective based on outdoor aerosol data from Kuwait. Atmosphere 2023;14:470.
34. Carvalho FP, Oliveira JM, Malta M, Lemos ME. Radioanalytical assessment of environmental contamination around non-remediated uranium mining legacy site and radium mobility. J Radioanal Nucl Chem 2014;299:119-25.
35. Church T, Rigaud S, Baskaran M, et al. Intercalibration studies of 210Po and 210Pb in dissolved and particulate seawater samples. Limnology & Ocean Methods 2012;10:776-89.
36. Du J, Du J, Baskaran M, Bi Q, Huang D, Jiang Y. Temporal variations of atmospheric depositional fluxes of 7Be and 210Pb over 8 years (2006-2013) at Shanghai, China, and synthesis of global fallout data. JGR Atmospheres 2015;120:4323-39.
37. Simon SL, Ibrahim SA. The plant/soil concentration ratio for calcium, radium, lead, and polonium: Evidence for non-linearity with reference to substrate concentration. J Environ Radioactiv 1987;5:123-42.
38. Ibrahim S, Whicker F. Plant accumulation and plant/soil concentration ratios of 210Pb and 210Po at various sites within a uranium mining and milling operation. Environ Exp Bot 1987;27:203-13.
39. Whicker FW, Schultz V. Radioecology: nuclear energy and environment. Available from: https://inis.iaea.org/Search/searchsinglerecord.aspx?recordsFor=SingleRecord&RN=15009266 [Last accessed on 22 Dec 2023].
40. Behbehani M, Uddin S, Al-Rashed Z, et al. Atmospheric concentration of 210-Pb, 210-Bi, and 210-Po in Kuwait. Available from: https://www.researchgate.net/publication/343480947_Atmospheric_Concentration_of_210Pb_210Bi_and_210Po_in_Kuwait [Last accessed on 22 Dec 2023].
41. Behbehani M, Uddin S, Habibi N, et al. 210Po in ultrafine aerosol particles and its likelihood to mutate the microbial community. http://kdrviewer.kisr.edu.kw/BookViewer/?book_id=8484&keyword=? [Last accessed on 22 Dec 2023].
Cite This Article
Export citation file: BibTeX | RIS
OAE Style
Uddin S, Gorgun AU, Fowler SW, Behbehani M, Habibi N, Filizok I, Al-Murad M. Climate change - mediated atmospheric 210Po and
AMA Style
Uddin S, Gorgun AU, Fowler SW, Behbehani M, Habibi N, Filizok I, Al-Murad M. Climate change - mediated atmospheric 210Po and
Chicago/Turabian Style
Uddin, Saif, Aysun U. Gorgun, Scott W. Fowler, Montaha Behbehani, Nazima Habibi, Isik Filizok, Mohammad Al-Murad. 2023. "Climate change - mediated atmospheric 210Po and
ACS Style
Uddin, S.; Gorgun AU.; Fowler SW.; Behbehani M.; Habibi N.; Filizok I.; Al-Murad M. Climate change - mediated atmospheric 210Po and
About This Article
Copyright
Data & Comments
Data
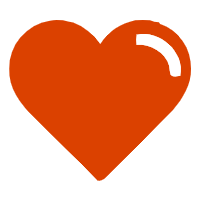

Comments
Comments must be written in English. Spam, offensive content, impersonation, and private information will not be permitted. If any comment is reported and identified as inappropriate content by OAE staff, the comment will be removed without notice. If you have any queries or need any help, please contact us at support@oaepublish.com.