Isolated shallow rocking foundation on different soils with varying embedment depth
Abstract
Although seismic design concepts integrated the idea of shallow rocking foundations as an effective way to dissipate the induced seismic energy, a practical design solution is yet to be established. To attain this objective, it is necessary to identify the key parameters that govern the rocking efficacy of foundation and eventually overall seismic performance of the entire structure. The primary focus of this study is to assess the key parameters such as soil type and embedment depth of footing in conjunction with varying rocking foundation efficacy on the seismic force and displacement demands of the Reinforced Concrete (RC) buildings. A simplified generic high rise RC building along with its foundation is considered for the analytical study which is designed and detailed as per relevant Indian Standards. To highlight the beneficial effects of rocking foundation, seismic responses of the RC buildings with varying foundation rocking efficacy are compared with the conventional foundation design philosophies by conducting nonlinear dynamic time history analyses. From the seismic responses, it is determined that the moment from column to foundation and base shear, owing to seismic action, decreases with an increasing settlement at the base of the foundation for rocking footing. It is also observed from the seismic responses that rocking foundations effectively de-amplifies the peak roof acceleration by utilizing the nonlinear soil responses during earthquakes. Hence, it can be depicted that the foundation rocking improves the overall stability of the buildings by decreasing the seismic force demands with a slight increase in seismic displacement demands. The investigation also indicates that the efficacy of rocking foundation is not sensitive to embedment depth of footing.
Keywords
INTRODUCTION
Researchers observed that buildings invariably go into an inelastic state under seismic excitation. Additionally, seismic design should control the damage due to seismic forces rather than avoiding it[1,2]. According to the present design philosophy, damage occurring in the critical members of the building is prevented by allowing the damage to less critical members first through strong column-weak beam concept, structural fuses, etc.[3,4]. Recent research indicates that such a design philosophy may also result in uneconomical and occasionally vulnerable buildings due to seismic events[5-7]. In general design practice, the nonlinear responses due to soil-foundation interaction are generally ignored for all practical purposes and considered that the additional forces during the seismic excitation will be resisted by the components of the superstructure itself[8]. However, nonlinear behaviour of the soil-foundation system can be considered appropriate to disperse the seismic energy transmitted to the buildings. This can be achieved by allowing the foundation to uplift, i.e., rocking of footing by providing an unconventional under proportioned footing termed as rocking footing or rocking foundations[9-14].
The mechanism of rocking foundation, as represented in Figure 1, illustrates that there is a possibility of uplift on one side of the footing during seismic shaking[15]. This uplift on one side of the footing transmits larger loads on the soil medium on the opposite side of the foundation. As a direct result of this, soil yielding may take place, which would result in the footing becoming plastically settled. On the other hand, foundation uplift causes a larger dissipation of energy and prevents the elements of the superstructure from yielding when there is a seismic force applied to the structure. Based on the experimental and numerical studies, several researchers[16-24] have suggested that rocking foundation has self-centring ability, elongates the structure period during excitation, and reduces the demands on the superstructure. Subsequently, simplified methods to predict the nonlinear rocking stiffnesses were provided[25-29]. However, rocking of the footing would not always be advantageous to the building, and in certain instances, it might even create adverse effects[30].
Figure 1. Comparison of failure mechanism of conventional foundation with rocking foundation[15].
Conventional foundation design approaches result in a highly flexible building made up of rigid foundation components, resulting in greater vulnerability to extensive damage during severe seismic events. As a result of these factors, repairing the damages is not cost-effective, so it is necessary to evaluate the feasibility of using a rocking foundation instead of the conventional rigid foundation. Response of rocking foundation depends on various structural parameters (aspect ratio of the building, stiffness, period of the building), soil properties (type of soil, relative density, cohesion), and ground motion characteristics (predominant period of excitation, amplitude, and direction of seismic motion). However, most of the studies, as mentioned earlier, focus on shallow footings on the ground level which is contrary to the actual foundation depth below the ground level kept for buildings. Limited studies[31] were available on the effect of embedment depth on the rocking efficacy of shallow footings. In addition to those studies related to the estimation of seismic force and displacement demands on the shear walls[32,33], field studies[34,35] on columns supported by the rocking shallow foundation are explored. However, the effect of foundation rocking on the multistory Reinforced Concrete (RC) frame buildings on varying rocking foundation efficacy has not been explored. Accordingly, this study aims to assess the effect of embedment depth with varying soil types on the seismic response of shallow rocking foundation of a RC frame. A comparative analytical study is undertaken on a high-rise RC building in conjunction with different soil conditions and with varying degrees of rocking. The fundamental time period of the building, roof drift, induced seismic moment and shear at column base and foundation settlement of conventionally designed footings are compared with rocking footings.
PARAMETRIC STUDY
A generic symmetrical plan, as presented in Figure 2, of a ten storey RC building with a storey height of
Modelling of superstructure and substructure
The considered building is modelled as a two-dimensional RC framed building, and only the external frame AB, as shown in Figure 2, has been considered for this study. The frame elements are designed as Special Moment-Resistant Frames (SMRF) as per relevant Indian standards[36-39]. Characteristic strength concrete (fck) and yield strength of reinforcement steel (fy) are considered as 40 and 550 Mpa, respectively, for the beam and column members. Fiber based distributed plasticity modelling approach is considered to simulate the nonlinear behaviour of frame members[41]. Validating with experimental data is essential to confirm the accuracy of the analytical modelling methodologies employed in this work.
The laboratory experiments on one bay two storey RC frame by Vecchio and Emara[42] are taken as a reference model for validation purposes. This model is chosen because Vecchio and Emara[42] carried out the pushover analysis on a two dimensional RC frame, and also thorough information about the experimental details and material properties used for their model are given by the author. The response of the simulated model in terms of capacity curve from the nonlinear static pushover analysis for both the OpenSees simulated model and experimental model is shown in Figure 3. A close agreement between the experimental and OpenSees models has been observed, which establishes the reliability of the simulated model, and hence, the proposed analytical model is used for further nonlinear analyses employed in this study.
Figure 3. Verification of the simulated analytical model with experimental results obtained from Vecchio and Emara[42] .
Similarly, the foundation of the RC frame is proportioned conventionally as per relevant Indian standards[43,44] to distribute the loads safely to the underlying soil. Initially, the footing was designed to experience 100% of the earthquake loads along with all the gravity loads coming from the superstructure. Later, the conventional footing is replaced with an increasing degree of rocking obtained by reducing its bearing area. Only the seismic loads coming from buildings are reduced to increase the rocking effect of foundation while no adjustments are made for loads due to self-weight of the members and expected live loads in the buildings to ensure serviceability condition of IS456[36]. Three types of soil conditions are chosen for this study, namely medium dense, dense, and very dense sand having angles of internal friction of 35°, 40°, and 45°, respectively, selected as per IS6403[44]. The unit weight and elastic modulus of the soil are considered as per the recommendation of Bowles[45] and Peck, Hanson and Thornburn[46]. Accordingly, the values for unit weight of the soil vary from 17 kN/m3 for medium dense sand to 22 kN/m3for very dense sand. Similarly, the elastic modulus of the medium dense sand is taken as 47.88 to 95.76 MPa for very dense sand. The details of the footing dimensions for conventional and rocking footings proportioned by assuming the soil conditions mentioned above are shown in Table 1.
Degree of foundation rocking with varying soil types considered in the study
Soil type | Footing dimensions | Footing nomenclature | Moment to shear ratio | FSv | Embedment depth of footing |
φ = 35° (MDS) | 4.0 × 4.0 m | CFD | 4.19 | 4.7 | 0.5, 1.0, 1.5, 2.0 and 2.5 m |
3.5 × 3.5 m | SR | 3.89 | 6.8 | ||
3.0 × 3.0 m | HR | 3.16 | 9.3 | ||
φ = 40° (DS) and φ = 45° (VDS) | 2.5 × 2.5 m | CFD | 3.5 | DS = 7.6 | |
VDS = 16.7 | |||||
2.0 × 2.0 m | SR | 3.3 | DS = 4.6 | ||
VDS = 10.6 | |||||
1.5 × 1.5 m | HR | 3.1 | DS = 2.5 | ||
VDS = 4.5 |
The supporting soil and foundation are modelled together as Beam on Nonlinear Winkler Foundation (BNWF)[47]. It is provided from the studies related to rocking foundation mentioned above that BNWF is one the most effective techniques for modelling the rocking foundation. The BNWF approach is conceptualized as a rigid footing supported by springs with zero length elements having parallel Q-Z springs used to simulate the vertical load settlement responses and two lateral springs at the edge of footing to simulate the passive load displacement behaviour (P-X springs) and shear sliding behaviour (T-X springs). The parameters pertaining to the BNWF are assumed as per the governing parameters and properties by Raychowdhury[47].
Seismic responses of buildings are highly sensitive to the applied ground motions in the event of earthquakes. The selected ground motions should be capable of capturing all the intensity levels, frequency content, duration, and all other parameters affecting seismic response of the building. Hence, 16 ground motions shown in Table 2 are selected for this study, with varying amplitude, frequency content and duration parameters. The seismic responses for all the buildings have been determined by performing nonlinear time history analysis as per FEMA356[48].
List of ground motions considered
Ground motion ID | Ground motion name | Station | Year | PGA (g) |
G1 | Hector | Amboy | 1999 | 0.023 |
G2 | Hector | Amboy | 1999 | 0.037 |
G3 | Kobe | Morigawachi | 1995 | 0.13 |
G4 | Kobe | Morigawachi | 1995 | 0.21 |
G5 | Kocaeli | Arcelik | 1999 | 0.21 |
G6 | Kocaeli | Arcelik | 1999 | 0.21 |
G7 | Imperial Valley | Delta | 1979 | 0.24 |
G8 | Landers | Coolwater | 1992 | 0.28 |
G9 | Imperial Valley | Delta | 1979 | 0.35 |
G10 | Loma Prieta | Gilroy Array #3 | 1989 | 0.37 |
G11 | Landers | Coolwater | 1992 | 0.42 |
G12 | Northridge | Beverly Hills | 1994 | 0.45 |
G13 | Loma Prieta | Gilroy Array #3 | 1989 | 0.56 |
G14 | Northridge | Beverly Hills | 1994 | 0.62 |
G15 | Duzce Turkey | Bolu | 1999 | 0.74 |
G16 | Duzce Turkey | Bolu | 1999 | 0.81 |
Effect of foundation rocking on fundamental natural period of the building
The distribution of base shear in the structural components is dependent on the fundamental natural period of the building, an important dynamic property. Figure 4 shows that the fundamental natural period of a building with a fixed base is significantly lower than the time period of the buildings calculated by considering footing and underlying soil.
The fundamental natural period of Conventional Footing Design (CFD) in medium dense and very dense sand is similar with an increase of 5% from the fixed base but lower than that of CFD in dense sand which shows an increase of 7%. The degree of increase is significantly lower for medium dense sand, whereas for dense and very dense sand, the improvement is rapid for the Heavy Rocking (HR) footing case. However, it is observed that time period of the building is not affected by varying embedment depths of footing. The lengthening period due to foundation rocking decreases the design base shear of the building.
Effect of foundation rocking with varying embedment depths on peak roof responses
For 16 ground motions, the seismic responses in terms of roof drift and roof accelerations for conventionally designed footings and rocking footings with various embedment depths of footings with different soil types are evaluated. Figure 5 depicts the observed peak roof drift for the considered ground motions with different foundation rocking conditions. From Figure 5, it is evident that the structural members on the conventionally designed footing experience higher peak roof drifts than the structural members with increasing foundation rocking, and it varies between 0.4% to 0.6% when the foundation size changes from Slight Rocking (SR) to HR.
Figure 5. Effect of foundation rocking on peak roof drift (median) for buildings on (A) medium dense sand; (B) dense sand; (C) very dense sand; at different footing embedment depths.
To comprehend the efficacy of foundation rocking on the inertial response in terms of peak roof acceleration for RC buildings, Figure 6 shows the effect on different foundation types considered. From Figure 6, it is evident that the peak roof acceleration decreases with increasing efficacy of foundation rocking and the maximum degree of reduction is about 20% to 25% for buildings on dense and very dense sand. However, responses were identical regardless of footing embedment depth.
Figure 6. Effect of foundation rocking on peak roof acceleration (median) for buildings on (A) medium dense sand; (B) dense sand; (C) very dense sand; at different footing embedment depths.
As a consequence of foundation uplift, roof displacement is found to increase with increasing rocking effect of foundation. Simultaneously, the roof acceleration transmitted into the superstructure is found to de-amplify, indicating the beneficial responses in the overall behaviour of the superstructure.
Effect of foundation rocking with varying embedment depths on peak foundation responses
Figures 7 and 8 depict the observed median responses for the considered ground motions in terms of peak moment transferred to the supporting footings and peak settlement at the foundation level. It is noted that the greatest reduction in peak moment is observed for HR footings on dense sand (35%), followed by HR footings on very dense sand (15%). In the remaining cases, i.e., for buildings on medium dense sand and SR footings, the degree of reduction was less than 10%, and it has been observed that the embedment depth of the foundation has no effect on the seismic responses for medium and very-dense sand.
Figure 7. Effect of foundation rocking on peak moment (median) transferred to the foundation for buildings on (A) medium dense sand; (B) dense sand; (C) very dense sand; at different footing embedment depths.
From Figure 8, the settlement at foundation increases substantially with the efficacy of foundation rocking, irrespective of the soil type. However, the observed settlements are within the permissible level of 50 mm as prescribed in IS8009[49]. On the other hand, varying embedment depth of the foundation did not affect the settlement responses. The settlement at the foundation increases because the narrower footings will result in lower bearing capacity and are more prone to have excessive settlement than the conventionally designed ones. The increase in settlement also indicates that the seismic force imparted on the RC buildings is significantly redistributed to the soil foundation interface.
Figure 8. Effect of foundation rocking on permanent foundation settlement (median) for buildings on (A) medium dense sand; (B) dense sand; (C) very dense sand; at different footing embedment depths.
The relative stiffness between the foundation and soil governs the roof drift
In addition to that, centrifugal studies on the embedded rocking footings by Hakhamaneshi and Kutter[31] indicate that the uplift mechanism of the foundation depends on the dilation features of the soil medium and soil ravelling mechanisms. In this study, soil is modelled using BNWF, i.e., parallel springs, to model footings’ settlement and rocking behaviour. Also, it must be noted that the foundation rocking mechanism is more predominant than the sliding mechanism due to the higher moment to shear ratio, as mentioned in Table 1. Hence, this study does not observe complex foundation rocking mechanisms due to embedment depth and its impact on foundation rocking. Therefore, rigorous nonlinear finite element analysis is required to understand the failure mechanism caused by foundation embedment depth, such as dilation and soil ravelling features.
The total base shear is calculated as a cumulative sum of base shear observed at all the bases of all columns, and Figure 9 shows the base shear for considered RC buildings with CFD, SR, and HR. For medium dense sand, the base shear is not significantly affected by increasing rocking efficacy and embedment depth since higher foundation sizes are generally provided for the medium dense sand soil types because they exhibit lower shear strength than dense and very dense sand. In contrast, for the very dense sand, the base shear tends to get reduced with increasing rocking efficacy and indicates that the rocking foundations impart lower seismic demand to RC buildings when compared with CFD cases.
Figure 9. Effect of foundation rocking on base shear (median) of buildings supported by (A) medium dense sand; (B) dense sand; (C) very dense sand; at different footing embedment depths.
Figure 10 illustrates the maximum force acting at the foundation soil in the form of spring force at the footing edge for the middle column. It is noted that the magnitude of spring force increases with decrease in footing size for medium dense sand. The degree of increase in end spring force is about 7.5% for SR footing and 3.5% for HR footing type. On the other hand, the magnitude of the end spring force is found to decrease for dense and very dense sand. However, no significant impact on the responses is observed for varying embedment depth of footing. As a consequence of the foundation uplift, the foundation settlement increases with the increasing rocking effect. On the other hand, maximum moment transferred to the foundation element and base shear at the foundation level decreases significantly, showing that increasing rocking effect reduces the overall seismic force demands of the building.
CONCLUSIONS
Soil foundation interface has the potential to disperse the seismic energy transmitted to the building through foundation uplifting. Present study investigated the effect of embedment depths of footings in conjunction with varying soil types on the rocking behaviour of shallow foundation. Also, the present study compares the effect of rocking foundation with conventionally designed footings and fixed base counterparts. The observed results from the eigen analysis indicate that the natural fundamental period lengthened with increasing rocking effect. Further, from the seismic responses, it is demonstrated that the rocking of footing decreases the roof acceleration with increasing roof drift. Also, the seismic moment induced from column base to foundation is reduced by about 35% for the very dense sand followed by dense sand, i.e., 15%, and finally, for medium dense sand, it is found to be approximately 10%. Further, the base shear exerted due to seismic action gets reduced with increasing rocking efficacy for buildings on dense and very dense sand. On the other hand, seismic settlement demand increases with increasing degree of rocking although the magnitude of the observed settlements was found to be within the maximum permissible limits. For medium dense and very dense sand, it has been observed that the effect of rocking is not considerably affected by an increase in embedment depth. This study shows that increasing rocking efficacy by under proportioning the footings shows overall beneficial effects to the RC buildings on dense and very dense sand by reducing the seismic force demands and damages due to the lateral force. However, rigorous nonlinear finite element analysis is required to understand the failure mechanism caused by foundation embedment depth, such as dilation and soil ravelling features. The results observed from this study indicate that rocking of footing is beneficial and reduces the seismic force demands on the superstructure than the conventionally designed footings.
DECLARATIONS
Acknowledgements
The authors highly acknowledge the reviewers for their valuable comments that have enhanced the quality of the manuscript.
Authors’ contributions
Conceptualization, methodology, software coding, analysis and writing: Kannan RM
Methodology, supervision, review, and editing: Haldar P, James N
Availability of data and materials
Not applicable.
Financial support and sponsorship
The authors cordially acknowledge resources provided by the Indian Institute of Technology Ropar and the Ministry of Human Resources and Development (MHRD), Govt. of India.
Conflicts of interest
All authors declared that there are no conflicts of interest.
Ethical approval and consent to participate
Not applicable.
Consent for publication
Not applicable.
Copyright
© The Author(s) 2024
REFERENCES
1. Bertero V. Strength and deformation capacities of buildings under extreme environments. Struct Eng Struct Mech 1977;53:29-79.
2. Paulay T. A simple seismic design strategy based on displacement and ductility compatibility. Earthq Eng Eng Seismol 1999;1:51-67. Available from: https://www.semanticscholar.org/paper/A-Simple-Seismic-Design-Strategy-Based-on-and-Paulay/cf65cb5e871dd2a18b5e645b9a73152cb8761a9f [Last accessed on 29 Jan 2024].
5. Indirli M. The 6th April 2009 L’aquila earthquake: from ruins to reconstruction, in seismicity and earthquake engineering, l’aquila earthquake of April 2009. 2010. Available from: https://www.researchgate.net/publication/259911243_Indirli_M_The_6th_April_2009_L%27Aquila_Earthquake_from_ruins_to_reconstruction_in_Seismicity_and_Earthquake_Engineering_L%27Aquila_Earthquake_of_April_2009 [Last accessed on 25 Jan 2024].
6. Kam WY, Pampanin S. The seismic performance of RC buildings in the 22 February 2011 christchurch earthquake. Struct Concr 2011;12:223-33.
7. Wallace JW, Massone LM, Bonelli P, et al. Damage and implications for seismic design of RC structural wall buildings. Earthq Spectra 2012;28:281-99.
8. Khamesi SO, Mir Mohammad Hosseini SM. A numerical study of influential parameters on rocking response of shallow foundations of single degree of freedom (SDOF) structures. J Eng Res 2023;11:100055.
9. Anastasopoulos I, Gazetas G, Loli M, Apostolou M, Gerolymos N. Soil failure can be used for seismic protection of structures. Bull Earthq Eng 2010;8:309-26.
10. Gajan S, Kutter BL, Phalen JD, Hutchinson TC, Martin GR. Centrifuge modeling of load-deformation behavior of rocking shallow foundations. Soil Dyn Earthq Eng 2005;25:773-83.
11. Hakhamaneshi M, Kutter BL, Gavras AG, et al. Database of rocking shallow foundation performance: slow-cyclic and monotonic loading. Earthq Spectra 2020;36:1585-606.
12. Gelagoti F, Kourkoulis R, Anastasopoulos I, Gazetas G. Rocking isolation of low-rise frame structures founded on isolated footings. Earthq Engng Struct Dyn 2012;41:1177-97.
13. Kannan R, James N, Haldar P. Influence of soil types on seismic behaviour of RC framed building with shear wall on rocking foundation. Proceedings of the 17th World Conference on Earthquake Engineering. 2020. Available from: https://www.researchgate.net/publication/361136355_Influence_of_Soil_Types_on_Seismic_Behaviour_of_RC_Framed_Building_with_Shear_Wall_on_Rocking_Foundation [Last accessed on 25 Jan 2024].
14. Kannan R, James N, Haldar P. Behaviour of mid rise buildings with shear wall rocking foundation system on medium dense site. Proceedings of the second ASCE India conference on challenges of resilient and sustainable infrastructure development in emerging economies (CRSIDE2020); 2-4 March 2020; Kolkata.
15. Gajan S, Soundararajan S, Yang M, Akchurin D. Effects of rocking coefficient and critical contact area ratio on the performance of rocking foundations from centrifuge and shake table experimental results. Soil Dyn Earthq Eng 2021;141:106502.
16. Agalianos A, Psychari A, Vassiliou MF, Stojadinovic B, Anastasopoulos I. Comparative assessment of two rocking isolation techniques for a motorway overpass bridge. Front Built Environ 2017;3:47.
17. Chen X, Li J. Seismic fragility analysis for tall pier bridges with rocking foundations. Adv Bridge Eng 2021;2:7.
18. Chiou J, Chen C, Hwang Y. Pushover and shaking table tests on a rocking-governed column-footing model on dry dense sand. J Chin Inst Eng 2018;41:247-58.
19. Hung H, Liu K, Chang K. Rocking behavior of bridge piers with spread footings under cyclic loading and earthquake excitation. Earthq Struct 2014;7:1001-24.
20. Taeseri D, Laue J, Anastasopoulos I. Non-linear rocking stiffness of embedded foundations in sand. Géotechnique 2019;69:767-82.
21. Taeseri D, Laue J, Martakis P, Chatzi E, Anastasopoulos I. Static and dynamic rocking stiffness of shallow footings on sand: centrifuge modelling. Int J Phys Model Geotech 2018;18:315-39.
22. Tsatsis A, Anastasopoulos I. Performance of rocking systems on shallow improved sand: shaking table testing. Front Built Environ 2015;1:9.
23. Wium J, van der Merwe J. Rocking shear wall foundations in regions of moderate seismicity. 2009; pp. 66-75. Available from: https://structurae.net/en/literature/conference-paper/rocking-shear-wall-foundations-in-regions-of-moderate-seismicity [Last accessed on 25 Jan 2024].
24. Algie TB, Pender MJ, Orense RP, Wotherspoon LM. Dynamic field testing of shallow foundations subject to rocking. 2010. Available from: http://db.nzsee.org.nz/2010/Paper15.pdf [Last accessed on 25 Jan 2024].
25. Acikgoz S, DeJong MJ. A simple model to quantify rocking isolation. Bull N Z Soc Earthq Eng 2018;51:12-22.
26. Acikgoz S, Ma Q, Palermo A, DeJong MJ. Experimental identification of the dynamic characteristics of a flexible rocking structure. J Earthq Eng 2016;20:1199-221.
27. Pisanò F, Di Prisco C, Lancellotta R. Soi-foundation modelling in laterally loaded historical towers. Géotechnique 2014;64:1-15.
28. Sieber M, Klar S, Vassiliou MF, Anastasopoulos I. Robustness of simplified analysis methods for rocking structures on compliant soil. Earthq Engng Struct Dyn 2020;49:1388-405.
29. Lu Y, Marshall AM, Hajirasouliha I. A simplified nonlinear sway-rocking model for evaluation of seismic response of structures on shallow foundations. Soil Dyn Earthq Eng 2016;81:14-26.
30. Psycharis IN. Effect of base uplift on dynamic response of SDOF structures. J Struct Eng 1991;117:733-54.
31. Hakhamaneshi M, Kutter BL. Effect of footing shape and embedment on the settlement, recentering, and energy dissipation of shallow footings subjected to rocking. J Geotech Geoenviron Eng 2016;142:04016070.
32. Gajan S, Kutter BL. Capacity, settlement, and energy dissipation of shallow footings subjected to rocking. J Geotech Geoenviron Eng 2008;134:1129-41.
33. Gavras AG, Kutter BL, Hakhamaneshi M, et al. Database of rocking shallow foundation performance: dynamic shaking. Earthq Spectra 2020;36:960-82.
34. Sharma K, Deng L. Field testing of rocking foundations in cohesive soil: cyclic performance and footing mechanical response. Can Geotech J 2020;57:828-39.
35. Sharma K. Field investigation and performance-based seismic design of rocking shallow foundations in cohesive soil. AB, Canada: University of Alberta Edmonton; 2019. Available from: https://era.library.ualberta.ca/items/663c0caf-4975-40b7-89a2-33202249c9c1/view/0d0f7d86-eaba-4a4c-8bd7-2c324a208c13/Keshab_Sharma_201905_PhD.pdf [Last accessed on 29 Jan 2024].
36. IS 456. Plain and reinforced concrete - code of practice [CED 2: cement and concrete]. New Delhi: Bureau of Indian Standards; 2000. Available from: https://law.resource.org/pub/in/bis/S03/is.456.2000.pdf [Last accessed on 29 Jan 2024].
37. IS 875-1. Code of practice for design loads (other than earthquake) for buildings and structures. Part 1 dead loads - unit weights of building materials and stored materials. New Delhi: Bureau of Indian Standards; 1987. Available from: https://law.resource.org/pub/in/bis/S03/is.875.1.1987.html [Last accessed on 29 Jan 2024].
38. IS 875-4. Code of practice for design loads (other than earthquake) for buildings and structures, Part 4: snow loads [CED 37: structural safety]. New Delhi: Bureau of Indian Standards; 1987. Available from: https://law.resource.org/pub/in/bis/S03/is.875.4.1987.pdf [Last accessed on 29 Jan 2024].
39. IS 13920. Ductile detailing of reinforced concrete structures subjected to seismic forces - code of practice [CED 39: earthquake engineering]. New Delhi: Bureau of Indian Standards; 1993. Available from: https://law.resource.org/pub/in/bis/S03/is.13920.1993.pdf [Last accessed on 29 Jan 2024].
40. IS 1893-1. Criteria for earthquake resistant design of structures, Part 1: general provisions and buildings [CED 39: earthquake engineering]. New Delhi: Bureau of Indian Standards; 2002. Available from: https://law.resource.org/pub/in/bis/S03/is.1893.1.2002.pdf [Last accessed on 29 Jan 2024].
41. McKenna F, Fenves G, Filippou F, et al. OpenSees. Berkeley: University of California; 2010. Available from: https://opensees.berkeley.edu/wiki/index.php/OpenSees_User [Last accessed on 29 Jan 2024].
43. IS 1904. Code of practice for design and construction of foundations in soils: general requirements [CED 43: soil and foundation engineering]. New Delhi: Bureau of Indian Standards; 1986. Available from: https://law.resource.org/pub/in/bis/S03/is.1904.1986.pdf [Last accessed on 29 Jan 2024].
44. IS 6403. Code of practice for determination of bearing capacity of shallow foundations. [CED 43: soil and foundation engineering]. New Delhi: Bureau of Indian Standards; 1981. Available from: https://law.resource.org/pub/in/bis/S03/is.6403.1981.pdf [Last accessed on 29 Jan 2024].
45. Bowles JE. Foundation analysis and design, fourth edition. 1988. Available from: https://trid.trb.org/view/311245 [Last accessed on 29 Jan 2024].
47. Prishati R. Nonlinear winkler-based shallow foundation model for performance assessment of seismically loaded structures. San Diego: University of California; 2008. Available from: https://escholarship.org/uc/item/6b7322bj [Last accessed on 29 Jan 2024].
48. FEMA 356. Prestandard and commentary for the seismic rehabilitation of buildings. Washington, DC, USA: Federal Emergency Management Agency; 2000; Available from: https://www.nehrp.gov/pdf/fema356.pdf [Last accessed on 29 Jan 2024].
49. IS 8009-1. Code of practice for calculation of settlements of foundations, Part 1: shallow foundations subjected to symmetrical static vertical loads [CED 43: soil and foundation engineering]. New Delhi: Bureau of Indian Standards; 1976. Available from: https://law.resource.org/pub/in/bis/S03/is.8009.1.1976.pdf [Last accessed on 29 Jan 2024].
Cite This Article
Export citation file: BibTeX | EndNote | RIS
OAE Style
Kannan RM, Haldar P, James N. Isolated shallow rocking foundation on different soils with varying embedment depth. Dis Prev Res 2024;3:1. http://dx.doi.org/10.20517/dpr.2023.29
AMA Style
Kannan RM, Haldar P, James N. Isolated shallow rocking foundation on different soils with varying embedment depth. Disaster Prevention and Resilience. 2024; 3(1): 1. http://dx.doi.org/10.20517/dpr.2023.29
Chicago/Turabian Style
R. Manoj Kannan, Putul Haldar, Naveen James. 2024. "Isolated shallow rocking foundation on different soils with varying embedment depth" Disaster Prevention and Resilience. 3, no.1: 1. http://dx.doi.org/10.20517/dpr.2023.29
ACS Style
Kannan, RM.; Haldar P.; James N. Isolated shallow rocking foundation on different soils with varying embedment depth. Dis. Prev. Res. 2024, 3, 1. http://dx.doi.org/10.20517/dpr.2023.29
About This Article
Copyright
Data & Comments
Data
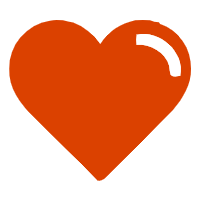
Comments
Comments must be written in English. Spam, offensive content, impersonation, and private information will not be permitted. If any comment is reported and identified as inappropriate content by OAE staff, the comment will be removed without notice. If you have any queries or need any help, please contact us at support@oaepublish.com.