Multidisciplinary research to advance the development of functional recovery for community resilience
Abstract
Functional recovery has the potential to serve as the link between asset-level design of the built infrastructure and community-level resilience to disasters. This article reviews current research and development efforts to advance the science-supporting post-earthquake recovery-based performance objectives for buildings and critical lifelines in the United States (US). We propose a holistic approach to the development of recovery-based design criteria that considers the various stakeholder perspectives within three distinct but interrelated stages of development: formulation of design guidance, codes, and standards; implementation of guidance, codes, and standards into practice; and evaluation of outcomes and impact. We propose a market-based stakeholder analysis that frames the diverse stakeholder perspectives within their role in supporting each stage: Policy Makers (the market makers), Decision Makers (the supply side), and End Users (the demand side). Within this context, we make two recommendations to support the development of recovery-based design standards: (1) economic evaluation should be conducted in conjunction with engineering design; (2) efforts at the formulation stage should be forward-looking to the implementation stage. Finally, we discuss challenges for implementation (defining critical functions, equity and community resilience, and monitoring, enforcement, and evaluation) and open questions for the future of functional recovery in supporting community resilience goals.
Keywords
INTRODUCTION
Natural hazards pose a significant risk to communities everywhere. Globally, deaths due to natural hazard events have fallen from a decade-high average of 500,000 deaths in the 1920s to 45,000 in the 2010s, a ten-fold decline[1]. On the other hand, global economic damages have been steadily increasing[2]. From the 1960s to the 1990s, the average of economic damages as a share of gross domestic product (GDP) more than doubled from 0.1% to 0.24% and has remained near that level since (0.22% in the 2010s). Since 1980, the United States (US) has experienced 341 weather and climate events with losses of at least $1 billion each (adjusted to 2022 USD) for a total cost in excess of $2.58 trillion[3]. In 2022 alone, the US had 18 such weather and climate events with a combined cost of $169.8 billion.
The impacts of natural hazard events on communities range from infrastructure damage, which may result in injuries and fatalities, to the interruption of critical lifeline services, the displacement of residents and local businesses, and disruptions to economic and socio-cultural systems[4]. Major earthquakes, such as the 1989 Loma Prieta (M 6.9) and 1994 Northridge (M 6.7) earthquakes, have been especially catastrophic, causing severe damage to single-and multi-family housing and hospitals and motivating significant policy changes[5]. Future earthquakes are expected to be even more devastating if no action is taken to improve building capacities. It is estimated that nearly half of the US population (approximately 150 million people) reside in regions at risk of experiencing a damaging earthquake within the next 50 years[6]. In regions of high seismic risk where an earthquake has not occurred for some time, simulated scenario studies predict deaths in the thousands, injuries in the tens of thousands, and hundreds of billions of dollars in direct economic losses, along with long-term, destabilizing impacts to community function[4].
Current building codes provide minimum safety requirements for most buildings to prevent collapse and ensure occupants can evacuate safely during an earthquake[7]. However, code-compliant buildings may nevertheless sustain significant damage and even be unusable or unrepairable after an earthquake. Moreover, as illustrated by major earthquakes such as the 1994 Northridge and 1995 Kobe (M 6.9) earthquakes, interruptions to the operation of critical facilities can cause widespread social and economic losses. The performance of lifeline infrastructure systems depends on numerous components designed and built over time, as well as standards, procedures, and material types[4].
Given that current design criteria do not explicitly ensure buildings and lifelines are functional or serviceable after an earthquake, there has been increasing interest from policymakers in the US to preserve functionality and protect against economic losses[8]. The most recent reauthorization of the National Earthquake Hazards Reduction Program (NEHRP), Public Law (PL) 115-307, included a heightened focus on achieving community resilience and a new requirement for the National Institute of Standards and Technology (NIST) and FEMA to provide recommendations to the US Congress for improving the built infrastructure to reflect performance goals stated in terms of post-earthquake reoccupancy and functional recovery time[4]. Beyond these national efforts, California Assembly Bill 393 tasked the California Building Standards Commission to explore the potential adoption and implementation of a “functional recovery” standard for building design[9].
In response to NEHRP PL 115-307, NIST and FEMA developed recommendations for research and development (R&D) in four key areas to advance hazard-resilient design for both the physical built environment and the social and economic systems that depend on the built environment[4,10]. The role of Congress in supporting hazard-resilient design includes supporting R&D in these four areas, encouraging local adoption of hazard-resilient building codes, adopting hazard-resilient code requirements for federally owned and leased buildings and lifeline infrastructure systems, and raising public and political awareness for the value of hazard-resilient buildings through education programs[4,11,12]. Recent legislative efforts to support hazard-resilient building design include the Disaster Savings and Resilient Construction Act of 2021 (HR 1984), which offered a tax credit for certain buildings designed and built according to resilient construction requirements, and the Consolidated Appropriations Act, 2022 (HRept 117-97), which tasked NIST and the National Oceanic and Atmospheric Administration (NOAA) to expand building design criteria to improve performance under weather and climate-related hazards[11].
In this article, we present ongoing efforts to improve the built environment through recovery-based performance goals within the context of two distinct but interdependent stages of seismic risk reduction policy development: formulation and implementation. In the next section, we present the concept of functional recovery and how it can support community resilience. We then review the current state of federal R&D efforts to advance formulation of recovery-based design standards and discuss challenges for implementation and the role of functional recovery in supporting community resilience goals. The following section presents the infrastructure policy development cycle to frame a stakeholder analysis that illustrates how community goals may not align with end user goals. Finally, we close with a discussion on the future of functional recovery, in particular for hazards beyond earthquakes.
FUNCTIONAL RECOVERY FOR COMMUNITY RESILIENCE
Community resilience is the ability to prepare for anticipated hazards, adapt to changing conditions, and withstand and recover rapidly from disruptions[13]. FEMA defines a community as “a group of people living in the same locality and under the same government, or a political subdivision of a state or other authority that has zoning and building code jurisdiction over a particular area”[14]. Thus, a community is a sociotechnical system in which buildings and lifeline infrastructure systems are interdependent components that support the needs of social and economic systems of the community[15,16]. However, there is often a disconnect between community resilience goals and the design, construction, and retrofit of individual infrastructure assets, such as buildings and critical lifelines[17]. The concepts of functionality and time to recovery of function are intended to provide that link through improved design and construction of individual buildings and lifeline infrastructure systems in a manner that aligns with community resilience goals. This shifts the focus from resilience planning and implementation activities taken at the community scale to building codes for individual buildings and industry standards for lifeline infrastructure[4].
The NIST-FEMA report to Congress defines functionality as a measure of how well a building or lifeline infrastructure system operates, delivers its required services, or meets its intended purpose[4]. Time to recovery of function is defined as a measure of how long it takes before a building or lifeline infrastructure system is functioning after an earthquake or other natural hazard event and is a means of defining performance that contributes to community resilience.
In terms of performance of buildings and critical lifelines, the NIST-FEMA report defines two key performance states for the recovery trajectory of an asset[4]: Reoccupancy is defined as a post-earthquake performance state in which a building is maintained or restored to allow safe re-entry for the purposes of providing shelter or protecting building contents.
Functional recovery is defined as a post-earthquake performance state in which a building or lifeline infrastructure system is maintained or restored to safely and adequately support the basic intended functions associated with the pre-earthquake use or occupancy of a building or the pre-earthquake service level of a lifeline infrastructure system. This performance level may be less than 100% restoration of pre-event service or functionality.
Within the framework of performance-based design, these performance states serve as targets for recovery-based objectives. A reoccupancy objective is reoccupancy achieved within an acceptable time following a specified earthquake, where the acceptable time might differ for various building uses. A functional recovery objective is functional recovery achieved within an acceptable time following a specified earthquake, where the acceptable time might differ for various building uses or lifeline services.
Designing for functional recovery represents a notable shift in design philosophy from current safety-based objectives, which emphasize the loss of life and prevention of serious injury, to recovery-based objectives. However, identifying and defining recovery-based objectives based on criteria of acceptable times requires the combined efforts of a broad spectrum of stakeholders, including policy makers, communities, and individuals[4]. The NIST-FEMA report suggests defining necessary and critical functions for a building or services for a lifeline infrastructure system, as well as acceptable targets for time to recovery of function within a performance objective, which can be tailored to a community context. As we discuss later, however, alignment from a community perspective may not capture the needs of the most impacted populations within a community, and improvements in recovery times should be designed to benefit those populations in particular.
Developing guidance, codes, and standards for functional recovery
In the US, there is no federal mandate for building codes and standards, in contrast to other earthquake-prone countries, such as Japan and Italy, that typically adopt national policies[5]. Instead, a seismic risk reduction policy is primarily the responsibility of state and local governments. The International Building Code (IBC) and the International Existing Building Code (IEBC) serve as the foundation (or “model code”) for building codes and standards that are eventually adopted locally by jurisdictions[18,19]. The versions that are ultimately adopted at the state or local level are often tailored to a locality’s needs (e.g., California’s codes focus more on earthquakes, while Florida’s focus more on hurricanes)[4].
Moreover, there does not exist a comparable “model code” for lifeline infrastructure systems (such as transportation, electric power, communication, gas and liquid fuel, and water and wastewater systems)[4]. There are no national-level requirements for retrofit and maintenance of these systems, and the development of industry-specific standards for hazard performance varies widely. Moreover, lifeline infrastructure systems are rarely developed with earthquake risk reduction in mind[4]. The construction, operation, and maintenance of a lifeline infrastructure system may be subject to numerous regulations and regulators, often with different sets of regulations for publicly and privately owned systems.
This decentralized system results in significant variation across communities with respect to the level of earthquake risk reduction encoded and enforced in design criteria for buildings and in the fractured approach to the design and retrofit of lifeline infrastructure systems[5]. An important implication is that there is a broad and diverse set of stakeholders potentially responsible for and affected by the development (as defined in the next section) of guidelines, codes, and standards for recovery-based design[7]. Federal agencies, such as NIST and FEMA, and programs, such as NEHRP, provide crucial leadership on risk mitigation and guidance informing policy development[11]. Importantly, local and regional initiatives can, and often do, also influence the guidelines, provisions, codes, and standards that are adopted at the national and international scale.
For instance, the devastating 1989 Loma Prieta Earthquake (M 6.9) and the 1994 Northridge Earthquake (M 6.7) left thousands of housing units uninhabitable and drew attention to the risk posed by soft-story buildings. These buildings are weak at the ground level due to large openings in perimeter walls for garage doors and store windows and few interior partition walls. In 1999, the City of Fremont, California, adopted an ordinance to establish retrofit standards and to have owners notified of potential earthquake hazards associated with their soft-story apartment buildings. The retrofit standards of Fremont formed the basis of the guidelines provided by the 2003 IEBC[5].
This example provides a model for the integration of local policies and practices, developed in the context of the needs of a particular community, into international codes and standards. Moreover, it suggests a potential path toward a more cohesive approach to the design and retrofit of lifeline infrastructure systems, as there is a need for industry-specific minimum guidelines for critical lifelines. In the next section, we summarize ongoing federal government research efforts to advance recovery-based design for buildings and lifeline infrastructure systems.
ONGOING RESEARCH TO ADVANCE RECOVERY-BASED DESIGN
In this section, we identify and provide additional context related to R&D efforts ongoing at the US. NIST to advance the formulation of recovery-based design standards for buildings and nonstructural systems. The efforts are categorized as (1) fundamental research on engineering design; (2) Developing guidelines for codes and standards; and (3) economic and social feasibility. These efforts are interdependent and together demonstrate a coordinated approach to the formulation of recovery-based design standards that can provide a foundation for successful implementation.
It should be noted that NIST also has several lifeline-related projects with forthcoming publications on roadways/bridges, public rail transportation, and water/wastewater and electric power functional recovery that will not be discussed in detail here as the research is preliminary. Nonetheless, the future development of functional recovery performance capacity for lifelines is essential not only for the health and financial well-being but also for the buildings that support the everyday lives of the public.
Fundamental research on engineering design
The joint NIST-FEMA report advocates for the development and availability of a framework for the prescriptive design of new buildings to achieve functional recovery performance[4]. This means that after an earthquake, a building may be restored to meet specific needs within an acceptable time frame to support the social and economic purposes of that building. The NIST-FEMA report stresses the utility of having nationally applicable minimums for functional recovery performance, with the ability for communities to exceed these requirements to enhance their performance and meet their specific needs. The report also recognizes that guidance, codes, and standards are needed for existing buildings and lifeline infrastructure systems.
Fundamental research on how to design for recovery-based performance objectives is the first step toward developing guidance, codes, and standards. The NIST-FEMA report suggests two design methods for buildings that may be used in the interim to achieve occupancy and functionality goals after a natural disaster: (1) Applying risk category (RC, as defined in the IBC) IV design to broader classes of buildings; and/or (2) utilizing performance-based design practice tailored to the needs of the asset and its users[4,11]. RC IV is associated with the most stringent design and quality assurance requirements, requiring buildings to have a reasonable probability of operating continually through and after a natural disaster at the design level hazard intensity[4,20]. The performance-based design methodology allows designers to analyze the consequences of building elements subjected to hazard events and adjust designs to meet desired performance goals[4]. The FEMA P-58 methodology provides guidelines and tools for performance-based design of structural and nonstructural building components[21].
State of the art structural design
To provide guidance on the most effective methods for enhancing structural design for functional recovery, the Earthquake Engineering Group at NIST is examining a range of lateral force resisting systems for commercial buildings in highly seismic regions. The systems include reinforced concrete moment frame, reinforced concrete shear wall, buckling-restrained braced frame, and steel moment frame, as illustrated in Table 1. All systems are designed in accordance with ASCE/SEI 7-16 requirements, except that a lower story drift limit and/or a higher importance factor is utilized to increase designed strength and stiffness and reduce expected post-earthquake recovery time[22,23]. A guideline document that provides minimum prescriptive design requirements to meet recovery-based design goals is in preparation[24].
Recovery-based design methods for structural systems[22]
Type | Height | Floor area (ft2) | Design methods |
Reinforced concrete moment frame | 4 and 12 stories | 14,400 | Drift limit (1%, 1.5%, or 2%) and importance factor (1, 1.5, or 2) |
Concrete shear wall | 4, 8, 12 stories | 14,400 | Drift limit and importance factor |
Buckling restraint braced frame | 4, 12 stories | 14,400 | Drift limit |
Steel moment frame | 4, 8, 12, 20 stories | 14,400 | Drift limit (1%, 1.5%, or 2%) and importance factor (1, 1.5, or 2) |
Other strategies to reduce repair time and costs include the use of seismic protection systems (e.g., base isolators, dampers, and rocking systems) to control structural and nonstructural damage and the use of redundant lateral systems (e.g., non-bearing shear wall) to provide alternate load paths and added strength and stiffness[25]. Moreover, a raft foundation can accommodate uneven settlements of the ground during earthquakes, preventing the collapse of upper structures[26]. Friction connections can dissipate energy through sliding rather than yielding elements, reducing repair costs for the steel moment frame[27].
State of the art nonstructural design
Nonstructural components play an important role in supporting the service and function of buildings. The NIST-sponsored project Seismic Analysis and Design of Nonstructural Components and Systems is intended to improve the seismic design of nonstructural systems and components. The project is a direct outcome of recommendations from a 2013 NIST report on “Development of NIST Measurement Science R&D Roadmap: Earthquake Risk Reduction in Buildings”, which identified nonstructural issues as a top priority[28]. The first phase of the project summarized industry standards applicable to nonstructural components, guidelines available for the design and installation of nonstructural components and systems, and methods that have been developed to measure response and validate the performance of nonstructural components and systems[29]. The second phase of this project reviewed ASCE 7-16 seismic requirements for nonstructural components and provided recommendations for changes to building codes and practice[30].
The Earthquake Engineering Group at NIST is analyzing a set of nonstructural designs for commercial buildings and evaluating their impacts on recovery time and repair costs. Three design strategies are considered: (1) Substituting nonstructural components with higher earthquake resistant capacities, such as equipment approved by the Special Seismic Certification Program (OSP)[31], along with increased anchorage; (2) Assigning a component importance factor (Ip) greater than ASCE/SEI 7-16 requirements to increase the strength and stiffness of bracing[20]; (3) Removing or replacing components with less vulnerable counterparts, such as removing drop panel ceilings in favor of an open concept or adding a seismic gap to stair joints. These strategies are integrated with enhanced structural designs [Table 2] to study the overall recovery performance of archetype buildings designed for functional recovery.
Guidance, codes, and standards for structural components
Category | Guidance, codes, and standards |
New and existing structures | ● ASCE/SEI 7-22[43], Minimum Design Loads for Buildings and Other Structures ● ASCE/SEI 41[44], Seismic Evaluation and Retrofit of Existing Buildings ● FEMA P-58-6[21], Guidelines for Performance-Based Seismic Design of Buildings |
Concrete structures | ● ACI 318[45], Building Code Requirements for Structural Concrete |
Steel structures | ● ANSI/AISC 341[46], Seismic Provisions for Structural Steel Buildings ● ANSI/AISC 360[47], Specification for Structural Steel Buildings |
Wood structures | ● ANSI/AWC[48] Special Design Provisions for Wind and Seismic ● ANSI/AWC[49] National Design Specification for Wood Construction |
Masonry structures | ● TMS 402/602[50] Building Code Requirements and Specification for Masonry Structures |
Other strategies to minimize earthquake impacts on nonstructural components include installing seismic snubbers for critical equipment, flexible connections for utility lines, and floor isolation systems to reduce vibration; relocating critical equipment to the base of buildings where accelerations are generally lowest; and increasing the separation of systems to avoid adverse interactions due to structural displacements or relative motions of structural and nonstructural components[25,30]. Moreover, new techniques are being developed by researchers to improve the performance of facade and cladding systems[32], partition walls[33,34], ceilings[35,36], and other vulnerable components[29,37].
Development of guidelines, codes, and standards for buildings
The fundamental research to advance the state of the art in structural and nonstructural design will ultimately be incorporated into updated guidelines, codes, and standards, in which NIST plays a critical role in supporting and advancing peer-based adoption processes. Moreover, while there is no definitive model code for critical lifelines, it should be noted that the efforts of NIST regarding critical lifelines also support the development of guidance, tools, and best practices, which will facilitate owner or community-led performance enhancements.
Guidance for recovery time
In the past few decades, it has become evident that societal expectations to be able to use housing and businesses and resume normal social functioning after an earthquake have outpaced the anticipated performance of currently existing buildings and infrastructure, which focus primarily on enabling human evacuation rather than building sustainability[37]. Significant efforts have been undertaken by federal personnel, engineers, academics, and codes and standards practitioners to advance recovery and resilience goals[38-40].
As noted earlier, this culminated in a 2018 Congressional request during the reauthorization of the NEHRP for FEMA and NIST to provide options for enabling functional recovery. Since delivering the recommendations in the NIST-FEMA report[4], there has been increased attention to the task of establishing acceptable recovery times for buildings and other infrastructure. This task is challenging for two reasons. Firstly, current codes and standards do not provide a direct mechanism to incorporate time targets. Secondly, the function of a building, especially its prioritization for recovery purposes with respect to the services it provides and its geographic location relative to its users within a community, does not consistently map to the current ways buildings are classified. Thus, new processes and mechanisms for incorporating functional recovery into guidelines, codes, and standards are being developed, in addition to new design specifications[7,37,41,42].
Guidance for structural components
Table 2 presents the guidelines, codes, and standards applicable to structural design, construction, and retrofit. The ASCE/SEI 7 code specifies minimum design load requirements for building components and other structures[43]. The ASCE/SEI 41 standard defines performance objectives of existing buildings and provides methods to evaluate and improve their performance[44]. The remaining provisions listed in Table 2 target specific structural types.
Guidance for nonstructural components
Table 3 presents the guidance, codes, and standards applicable to nonstructural design, installation, inspection, testing, evaluation, and retrofit. The FEMA E-74 guide for nonstructural risk reduction has been updated three times (1985, 1994, and 2012) to incorporate new lessons learned from earthquakes[51]. The guide explains the sources of nonstructural earthquake damage and the methods to reduce potential risks for schools, office buildings, retail stores, hotels, data centers, hospitals, museums, and light manufacturing facilities. The guide is intended for use by non-engineer audiences, such as building owners, facility and risk managers, and maintenance and safety personnel[51]. The rest of the provisions listed in Table 3 target specific building components and should be implemented by qualified designers and inspectors.
Guidance, codes, and standards for nonstructural components
Category | Guidance, codes, and standards |
Equipment and bracing in new and existing buildings | ● FEMA E-74[51], Reducing the Risks of Nonstructural Earthquake Damage – A Practical Guide ● FEMA P-58-6[21], Guidelines for Performance-Based Seismic Design of Buildings ● ASCE/SEI 7 Chapter 13[43], Minimum Design Loads for Buildings and Other Structures ● ASCE/SEI 41[44], Seismic Evaluation and Retrofit of Existing Buildings ● ASCE/SEI 19[52], Structural Applications of Steel Cables for Buildings |
Mechanical, electrical, and plumbing components | ● VISCMA 101[53], Seismic Restraint Specification for Mechanical, Electrical, and Plumbing Systems, formerly FEMA Seismic Restraint Installation Manuals 412, 413 and 414 ● VISCMA 102[54], Vibration Isolation Specification Guidelines for Mechanical, Electrical, and Plumbing Systems |
Suspended acoustic tile ceilings | ● ASTM C635[55], Standard Specification for the Manufacture, Performance, and Testing of Metal Suspension Systems for Acoustical Tile and Lay-in Panel Ceilings ● ASTM C636[56], Standard Practice for Installation of Metal Ceiling Suspension Systems for Acoustical tile and lay-In Panels, applied to seismic design categories A, B, D, E, and F ● ASTM E580[57], Standard Practice for Installation of Ceiling Suspension Systems for Acoustical Tile and Lay-in Panels in Areas Subject to Earthquake Ground Motions, applied to seismic design categories C, D, E, and F |
Elevators | ● ASME A17.1[58], Safety Code for Elevators and Escalators |
Fire protection systems | ● NFPA 13[59], Standard for Installation of Sprinkler Systems |
Economic and social feasibility
At the implementation stage, cost-effectiveness is the key driver for decision makers who supply functional recovery of buildings and critical lifelines[7]. While standards, such as ASTM’s Standard Guide for Developing a Cost-Effective Risk Mitigation Plan for New and Existing Constructed Facilities[60], and decision support tools, such as FEMA’s Benefit-Cost Analysis Guide[61], provide guidance for evaluating economic performance of risk reduction strategies, there is no standard approach for economic evaluation that incorporates the full breadth of stakeholder impacts from reducing post-earthquake recovery times. For instance, Zhang et al. (2023) observe that the literature on benefit-cost analysis (BCA) for earthquake risk reduction primarily focuses on building damage, repairs, and casualties, occasionally considering other impacts, such as business interruption and population displacement[62].
Although economic evaluation is typically conducted at the implementation stage, particularly the project planning stage, we recommend that economic evaluation be conducted at the formulation stage in tandem with engineering performance assessment of design options[7]. Stakeholder workshops hosted by NIST and FEMA asked participants to consider the factors most relevant to policy makers for assessing and comparing options to improve functional recovery[63]. The traditional economic evaluation criteria of cost and benefits were consistently voted as important, with cost identified as the most important. At a minimum, an iterative, coordinated engineering and economic assessment can be used to preclude economically infeasible design options. Ideally, the process would be applied beyond the R&D cycle to inform the formulation of guidelines for codes and standards.
To address these gaps, NIST (2022) presents a framework for both policy makers and decision makers to conduct economic evaluations of recovery-based design criteria[7]. The report provides a risk-based economic framework for selecting among candidate design options, as well as how building owners, occupants, and communities can evaluate the decision of whether to adopt a new functional recovery design standard. Importantly, the framework can be used in conjunction with the formulation of engineering design guidelines if there is a desire to ensure economic feasibility of candidates for design standards. The framework is not intended to replace existing economic analysis methods and tools but to complement existing methods and tools by providing a structured catalog of inputs (and methods for estimating them), as summarized in Table 4. In addition to this roadmap for economic analysis of functional recovery design, the report identifies measurement gaps and research needs for conducting a robust economic evaluation[7].
Summary of potential direct and indirect losses avoided associated with reoccupancy and functional recovery[7]
Category | Loss | Examples | Stakeholder |
Direct | Damage casualties | ● Building damage ● Contents damage | ● Owner ● Occupant |
Direct | Casualties | ● Injuries ● Fatalities | ● Occupant ● Occupant |
Indirect | Economic | ● Business interruption ● Supply chain disruption* | ● Occupant ● Upstream and downstream customers |
Social | ● Displacement ● Deterioration of mental health** ● Loss of social cohesion** ● Regressive hazard insurance** | ● Occupants ● Local community ● Local community ● Local community | |
Physical | ● Pollution due to repairs or demolition* ● Greenhouse gas emissions* | ● Global community ● Global community |
The recommendation that economic evaluation should occur iteratively and in parallel with engineering design efforts in order to assess feasibility is being put into practice. Fung et al. (2022) conducted benefit-cost analyses for various structural and nonstructural designs to provide evidence for the feasibility of potential recovery-based design options[64]. This research, led by an interdisciplinary team at NIST, is ongoing and will cover all four structural systems discussed in the previous section for a range of building heights and various combinations of structural and nonstructural improvements. The goal is not to provide design recommendations but to demonstrate how design and economic evaluation can be conducted together by providing feasible economic evaluations of plausible recovery-based design options.
The economic analyses conducted thus far consider a small fraction of potential benefits (in particular, business interruption and displacement) and costs that could be considered in an economic assessment. As shown in Table 4, the framework highlighted gaps and research needs with respect to data and quantification for a wide range of potential benefits and stakeholder groups that are typically not considered relevant for life safety[62]. Such impacts are important for evaluating social feasibility. In addition to cost and benefits, the NIST-FEMA stakeholder workshops identified feasibility, effectiveness, and equity as relevant evaluation criteria for assessing and comparing design options[63]. It is worth noting that while some aspects of social feasibility may be quantifiable in an economic evaluation or other quantification processes (e.g., metric or indicator development), others may be qualitative and must be evaluated in complement to an economic analysis as part of a holistic approach to recovery-based design. For instance, workshop attendees highlighted the need to consider complex issues such as governance, meeting the needs of underserved populations, and variation in the degree of buy-in across communities[63]. We discuss some of the challenges presented by a holistic approach that considers the complexity of social feasibility and end users below.
Finally, we note potential ambiguity with the blanket term “cost”, which can include upfront costs for new construction, retrofit costs, and maintenance costs, as well as costs associated with code implementation (including training and technical support), plan evaluation, and site inspection[7]. The NIST-FEMA workshops cautioned that care should be taken for evaluation criteria to be clearly defined[63]. For instance, “cost” was discussed in the context of a variety of metrics (e.g., cost to individual, life cycle cost, whomever is bearing the cost, upfront capital investments), and the relative ranking of evaluation criteria varied depending on the context. It is imperative that when discussing cost considerations and other metrics utilized for the evaluation of functional recovery design suitability, significant attention should be paid both to the assumptions people are making around the definition of these terms and also to important impacts or aspects that they may be leaving out.
POLICY DEVELOPMENT FOR RECOVERY-BASED DESIGN CRITERIA
A shift toward recovery-based design criteria necessitates a holistic, multidisciplinary approach that recognizes the social dimensions of disaster recovery and risk reduction. As Sobhaninia and Buckman (2022) show, the post-disaster recovery trajectory of a community depends as much on its pre-event socio-economic state as it does on the performance of infrastructure and emergency management throughout the event[65]. In other words, enhanced design criteria for improving time to recovery of function may be a necessary condition but is certainly not a sufficient condition to ensure resilience of a community. Understanding the breadth of social demands and expectations through the lens of the various stakeholder perspectives is essential to setting sufficient conditions for community resilience. In this section, we discuss the policy development cycle for infrastructure systems and frame competing interests through a stakeholder analysis that highlights the disconnect between community goals and end user needs.
The policy development cycle: formulation, implementation, and evaluation
The implication of the decentralized system in the US is that the development of recovery-based design criteria will require understanding and integrating multiple stakeholder perspectives. We propose that the development of infrastructure policy consists of three distinct but interdependent stages [Figure 1][66]. We define formulation of recovery-based design criteria as the integration of engineering design recommendations (including design criteria) and economic and social considerations (including acceptable times for recovery of function) into guidelines that inform codes and standards. In this sense, federal R&D efforts support the formulation of recovery-based design criteria at the global level. We define implementation of recovery-based design criteria as the (mandatory or voluntary) adoption, monitoring, and enforcement of design criteria at the local level. Thinking through the social impacts at each stage of the process will help us to identify the set of stakeholders. Moreover, as the preceding discussion makes clear, these two stages can and do inform one another, and feedback between the two is bidirectional.
Figure 1. Flowchart for the development of infrastructure policy and relevant stakeholder groups: formulation (global), implementation (local and global), and evaluation (local). Source: the authors.
As the figure shows, the third stage makes the policy development cycle iterative. Evaluation is defined as the systematic assessment of outcomes and impacts of a policy that has been implemented and provides a key feedback mechanism from implementation to formulation. This stage is critical for policy maintenance to identify what is working and what is not and to provide opportunities for policy readjustment. Ideally, evaluation should occur at regular intervals and is not a one-time activity. As this stage is highly localized and dependent on implementation, it is beyond the scope of the current article.
Defining realistic and meaningful target recovery times and design criteria will require that engineering professionals collaborate closely with social scientists and community-based officials to understand social needs and economic feasibility[7,67]. For instance, building occupancy type (e.g., residential or commercial) and RC are sensible defaults for defining critical functions of a building. However, it is unclear if such classifications are reasonable from the perspective of those who use and depend on the services of a building. The classic example is a school categorized as “Educational” occupancy and RC III. However, schools often provide additional services in a community, including shelter during a natural hazard event, vaccination sites, polling places for elections, and gathering places for community members[68,69].
Additionally, the benefits and costs of reducing time to recovery of function will be distributed across various stakeholders that may have conflicting goals. For instance, those paying for construction projects with recovery-based performance objectives may not be the same as those who benefit from reducing post-earthquake recovery times[7]. Likewise, those who bear the costs when disaster strikes are not usually the same as those who would have to pay for pre-disaster mitigation efforts. Thus, in formulating design guidelines for functional recovery, it will be important to consider economic feasibility in parallel in order to ensure that proposed designs are feasible given tradeoffs between desired risk mitigation and economic criteria such as benefits or costs[62].
Economic and social feasibility will also inform public perceptions, attitudes, and decisions regarding implementation of recovery-based design criteria. For instance, Sattar et al. (2019) identified a research need to understand behavior and decision-making for infrastructure owners and managers considering investments to maintain and repair their assets, including the decision and motivation to upgrade a building or lifeline infrastructure system[70].
Moreover, policy makers recognize that the perception of infrastructure risk to natural hazards of the public is often far removed from the level of expected performance encoded in current design criteria. For instance, a tall building designed for a life safety performance objective may require up to 7.5 months of repair to return to functionality after a design-level earthquake (roughly equivalent to ground motion shaking with a 10% probability of exceedance in 50 years) and over one year for a return to functionality after a risk-targeted maximum considered earthquake (roughly equivalent to ground motion shaking with a 2%-4% chance of exceedance in 50 years)[71].
The US Advisory Committee on Earthquake Hazards Reduction (ACEHR) observes that “Designing new buildings and retrofitting existing buildings to a functional recovery design objective will better align with public expectations regarding seismic performance of the infrastructure, enable our communities to recover more quickly following an earthquake, and ultimately achieve the resilience desired[72]”. Implementation of recovery-based design criteria is, therefore, likely to encounter unique communication challenges to support adoption, monitoring, and enforcement. The committee of experts authoring the report to Congress on options for achieving functional recovery highlighted the need to gather information from stakeholders to ensure that the development of functional recovery performance objectives meets the needs of the American public. In 2020, NIST held a series of workshops to gather input from subject matter experts regarding the definition of functional recovery and related concepts, as well as the timeframe that should be targeted for the return of functions (buildings) and services (lifelines systems)[63].
Who are the stakeholders? A market design perspective
We propose the following stakeholder analysis within the context of the formulation and implementation of recovery-based design standards. The international standard providing guidance on social responsibility called ISO 26000 defines a stakeholder as an “individual or group that has an interest in any decision or activity[73]”. Typical stakeholder analysis categorizes stakeholders by a range of impacts; e.g., primary stakeholders are most affected, and tertiary stakeholders are least affected by a decision or activity[73,74]. However, this framing is insufficient to capture the full lifecycle of new codes and standards development[7].
To support both formulation and implementation, we frame the stakeholder perspectives into three tiers based on their role in advancing recovery-based design criteria [Table 5]. As is evident from Figure 1 and Table 5, communities play an important role across all three stages of the policy development cycle.
Stakeholder analysis matrix for the formulation, implementation, and evaluation of recovery-based design standards in the United States, based on stakeholder role, influence, and interest in functional recovery policy development
Tier | Stakeholder | Role | Influence | Interest |
Policy Maker | ● Researchers ● Practitioners | Formulation | Guidelines to inform codes and standards | Evidence-based criteria |
SDOs and other professional organizations | Formulation | Formulate standards that become model codes | Uniformity and validity (through vetting) | |
Community | Formulation Implementation Evaluation | Adopts (by law) local versions of model codes | Legal standing for local construction, incentives | |
Decision Maker | Developers | Implementation | Voluntary compliance for new infrastructure | Profit from operation or sale of investment |
● Owners ● Operators ● Managers | Implementation | ● Voluntary compliance for existing infrastructure ● Tax base | ● Property values ● Managing operating expenditures ● Maintenance | |
Community | Formulation Implementation | Monitoring and enforcement | Plan review and quality control | |
End User | Building occupants | Implementation | Demand for residential and nonresidential facilities | ● Rental rates ● Services ● Sense of place ● Security |
Lifeline customers | Implementation | Demand for critical lifeline services | Basic needs are met | |
Upstream suppliers | Implementation | Demand for downstream customers | Minimizing disruptions | |
Downstream customers | Implementation | Demand for upstream products | Minimizing disruptions | |
Community | Formulation Implementation | ● Demand for cultural value, social cohesion, and public welfare ● Response and recovery | ● Social welfare ● Community resilience |
The Policy Makers: this group plays a key role in guiding and informing codes and standards and includes (engineering and social science) researchers and practitioners, standards development organizations (SDOs), and other professional organizations and communities (both local and global).
The Decision Makers: this group is responsible for implementing codes and standards and includes infrastructure owners and managers, developers, construction firms, and community-level individuals and organizations that provide plan initiation, review/inspection, quality control, and compliance functions.
The End Users: this is the group of beneficiaries of functional recovery performance and are most impacted by improved recovery times. This group includes building occupants (e.g., residents/visitors, businesses, and consumers) and lifeline customers (e.g., residential customers of electric power distribution), upstream suppliers and downstream customers in receipt of an asset’s services or functions, and the local community.
This framing of stakeholder perspectives can be interpreted within a fictitious process of designing a “market” for post-earthquake functionality. A market is simply an institution for the exchange of goods or services, where an institution is a particular set of rules that defines exchange within the market and the infrastructure that supports exchange (including physical, technological, and legal)[75]. As Kominers et al. (2017) note, market design focuses on reverse-engineering the rules and infrastructure for a market in order to align “market outcomes with society’s objectives beyond pure economic efficiency[75]”. Importantly, market exchange is not always facilitated by a price mechanism but is rather driven by incentives. For instance, market design has informed the rules and infrastructure supporting primary and secondary school admissions as well as kidney donations and exchanges. In these markets, there is no price mechanism at all, and market outcomes are driven by equity and ethical concerns.
From this perspective, the Policy Makers are considered the intermediaries (or “market makers”) who facilitate market activity by setting and enforcing the rules and providing the supporting infrastructure; the Decision Makers are the supply side who “produce” functional recovery; and the End Users are the demand side, who “consume” functional recovery. The immediate takeaway is that communities have a role in each of the market functions and thus can serve as the key link between the formulation and implementation stages.
This framing illustrates how community interests may fail to align with those of individual end users, depending on their role in the market. Communities may not be aware that they play multiple roles in the development of recovery-based design standards. Moreover, as end users themselves, communities are primarily interested in community resilience investments that maximize social welfare, as shown in Table 5. This is often operationalized in terms of Pareto efficiency: outcomes that maximize a social welfare criterion and cannot be improved without making some members of the community worse off[76-78]. In economics, however, it is well known that there are tradeoffs between equity and efficiency: two outcomes may be Pareto efficient but result in different distributions of individual welfare, and any specification of a social welfare function implicitly embodies ethical and moral judgments about individual welfare[79-81]. Thus, when formulating and implementing policy for functional recovery, communities should be mindful of their multiple roles in the market and consider how community resilience is achieved relative to all end user interests.
Finally, we note that we are not proposing that functional recovery should be provided through a marketplace. Rather, the process of designing a market provides a useful analogy for thinking through incentives across different market participants. In particular, it is analogous to the decentralized and often voluntary system of adopting codes and standards for buildings or guidance for critical infrastructure. As Kominers et al. (2017) note, markets may be “run freely by firms, regulated, or organized by governments; they may or may not involve monetary transfers; and they may or may not require/enforce participation[75]”.
This market design framing of stakeholder perspectives highlights the relationship between the two stages of policy development and how R&D at the formulation stage can incorporate end user perspectives to support implementation. In the next section, we discuss challenges for implementation of functional recovery within the context of multiple competing interests for recovery-based design criteria.
CHALLENGES FOR IMPLEMENTATION AND COMMUNITY RESILIENCE
Within the context of ongoing federal R&D to advance recovery-based design, we leverage the market design framing of stakeholder interests to examine the future of functional recovery at the next stage: implementation.
As we argue in the preceding, ongoing R&D efforts at the formulation stage should be conscious of providing a solid foundation for the implementation stage. The stakeholder analysis in Table 5 provides a template for feedback mechanisms across formulation and implementation based on competing interests by different groups of stakeholders. Based on expert feedback, a holistic evaluation of design options at the formulation stage should incorporate a consistent stakeholder perspective through which the evaluation is conducted: “are the options being assessed through the lens of the individual? The community? The Federal Government? Even with a consistent basis of evaluation criteria, the assessment of the option may depend on the perspective being applied[63]”.
Functional recovery, therefore, is about more than providing improved design standards. The functionality of a building is related to the resilience of the people and organizations who rely on it[82]. This is borne out in practice. The recovery and rebuilding efforts in New Zealand following the Christchurch earthquake sequence revealed that functional recovery depends critically on factors such as social and organizational preparedness and governance, in addition to the physical recovery of the asset and resources for repairs[83]. Zhan et al. (2023) conclude that functional recovery should be suitably contextualized within each stage of implementation, “including design and construction, maintaining and monitoring, and the post-earthquake inspection and assessment[83]”.
Within the context of ongoing federal R&D efforts to advance the formulation of recovery-based design criteria and the importance of a holistic perspective across both formulation and implementation, we discuss challenges for implementation and the potential link between functional recovery and community resilience.
Defining critical functions
As discussed earlier, defining critical functions of a building or critical services of a lifeline system will require understanding social needs. Indeed, expert feedback from the NIST-FEMA workshops suggests that the built environment should serve the social environment and that this should be an explicit consideration in the development of recovery-based design standards[63]. This sentiment has echoes of the philosophy underlying community resilience, which is that the built infrastructure exists to support social and economic systems[84-86].
Defining critical functions and services should, therefore, align with social functions, including supporting social institutions, such as non-profit and religious organizations, a community’s social capital, and cultural welfare[63]. As the preceding stakeholder analysis reveals, community context is important since social functions may be idiosyncratic across communities as well as within a community’s stakeholders-from developers and owners to occupants and customers. Each community will need to assess the role of the built infrastructure in supporting its specific social functions and balancing the needs of its decision makers and end users. Workshop participants cite the example of homeless shelters: across the country, they serve important vulnerable populations, but varied climate and seasonal weather mean that in some places, and at certain times, the need for post-disaster shelter is critical to ensure the preservation of life[63].
Equity and community resilience goals
As we observed earlier, recovery-based design criteria may be a necessary but not a sufficient condition for community resilience. Inequitable distributions of pre-and post-disaster resources result in events with disproportionate impacts on historically underserved communities, intensifying disaster risk and thus reducing overall resilience capacity and the ability of a community to bounce back[65]. This highlights a potential gap between post-event functionality and community resilience that depends in large part on equitable access to resources. Moreover, as the stakeholder analysis suggests, community resilience goals may be Pareto efficient without ensuring equity.
According to participants in the NIST-FEMA workshops, it is crucial to consider who will be most impacted by an event and not just where, as historically marginalized and underserved communities tend to experience the longest displacement times[63]. As Lindell (2007) observes, even if the community recovers, some neighborhoods, households, economic sectors, or individual businesses may never recover[87]. Therefore, anticipating the most vulnerable population segments and economic sectors is essential for implementation[65].
An individual’s loss of place can be the most catastrophic impact of a disaster[65,88]. Moreover, as Spoon et al. (2020) find in the aftermath of the 2015 Nepal (M 7.8) earthquake, place attachment, uncertainty, and mental well-being are interrelated for the most marginalized communities[89]. As noted in the NIST-FEMA workshops, enhanced recovery times should aim to reduce disparities across demographic characteristics for recovery[63].
Functional recovery can lessen physical, psychological, and emotional stress and trauma from displacement, but these issues must be considered explicitly in both formulation and implementation. The stakeholder analysis in Table 5 can support both the framing and integration of equity within community resilience goals. Formulation should account for decision makers and end users at the implementation stage and prioritize cost-effective solutions that are accessible to all members of a community. Implementation should prioritize end users in underserved communities that stand to lose the most from a disaster. At both stages, this raises important questions comparing recovery needs for new and existing buildings[90], how to prioritize service areas of critical lifelines, the distribution of benefits and impacts, and the communication and voices of underserved communities. Importantly, historically marginalized communities may not perceive the value of risk reduction or may feel a lack of trust about the process from the outset. It is important to incorporate these perspectives throughout the planning process and to make every effort to represent the shared values of all community members[63].
Monitoring, enforcement, and beyond
As defined above, implementation includes the (mandatory or voluntary) adoption, monitoring, and enforcement of design criteria at the local level. Monitoring and enforcement include administrative challenges, such as plan review and quality control, for proposed construction projects, which may be associated with non-negligible costs[91]. As illustrated in Table 5, this is a critical role in the supply side of the market for functional recovery. The implication is that communities need to resource functional recovery for success beyond simply providing enhanced design criteria, given that the recommendations are to provide minimum design criteria for improving recovery times.
The cost of not enforcing codes can be tragic, as evidenced by the 2023 Turkey-Syria Earthquakes (M 7.8)[92]. Over half of all buildings in Turkey (roughly 13 million apartments) were in violation of current building codes, including homes built without permits, buildings with unapproved extra stories or expanded balconies, and informal “squatter homes” inhabited by low-income residents. Such violations were allowed under an amnesty program unveiled in 2018, in which building code violations could be resolved by simply paying a fine to the agency in charge of enforcing building codes.
Implementation in the economic field of market design specifically means that the actual outcome of an institution coincides with the desired outcome. As Hurwicz (1993) shows, this will require diverting resources from the production of goods and services to the support of an enforcement and information processing system[93]. Formulating and codifying policies do not guarantee success on the ground if policies are not implemented well, including appropriately resourcing monitoring and enforcement processes[94]. As illustrated by the case of Turkey, failure to do so can have catastrophic consequences for the most vulnerable populations in a community.
Finally, even with fully resourced monitoring and enforcement, a policy may not achieve the desired outcome. Alternatively, it may be the case that the desired outcome is no longer desired. The evaluation phase is critical for checking the outcomes of the implementation phase and allowing for potential course correction. Evaluation is often conducted as an afterthought but should be carefully planned for at the implementation stage. However, as with monitoring and enforcement, evaluations will require sufficient resources.
DISCUSSION AND CONCLUSION
In this article, we discuss ongoing R&D efforts to advance functional recovery and the potential for functional recovery to support community resilience goals. We propose a holistic approach to the development of recovery-based design criteria that considers the various stakeholder perspectives within two distinct but interrelated stages of development: formulation of codes and standards at the global level and implementation of codes and standards at the local level. To frame the holistic approach, we propose a market-based stakeholder analysis that frames the diverse stakeholder perspectives within their role in supporting each stage: Policy Makers (the market makers), Decision Makers (the supply side), and End Users (the demand side).
After reviewing federal R&D efforts by NIST to advance formulation (including engineering design, development of guidelines for codes and standards, and economic and social feasibility), we discuss three key challenges for implementation: defining critical functions, equity, and monitoring, enforcement, and evaluation. The key takeaway from the challenges is that community goals may not fully account for or align with end user goals. Indeed, Amirzadeh et al. (2022) demonstrate ambiguities and diverse interpretations of the concept of (community) resilience[95].
Within the context of ongoing research and our stakeholder analysis, we propose two recommendations for the development of recovery-based design criteria. The first is that economic evaluation and engineering design should be conducted together at the formulation stage. This recommendation is being put into practice by multidisciplinary teams at NIST. We should note that a guideline document that provides minimum prescriptive design requirements to meet recovery-based design goals[24], as well as economic evaluation for a range of structural systems, are in preparation. The second recommendation is that the formulation stage should provide the appropriate foundation for implementation within a holistic approach to the development of recovery-based criteria. Ultimately, functional recovery is intended to support the End Users, and this should be considered throughout the policy development lifecycle. Moreover, the development cycle should be iterative through carefully planned and deliberate evaluation.
We close with a look to the future of functional recovery and several open questions that require further research.
● The first is whether and how functional recovery can support local (community) and global (national) climate adaptation goals. Within the broad context of earthquake engineering, the role and relationship with climate change is only recently beginning to be addressed and adds another layer of complexity due to the simultaneously local and global impacts of climate change and climate adaptation, as well as the relatively longer planning horizons for climate considerations[7].
● A second related but broader question is the nature of functional recovery for other hazards and whether it is feasible or context-specific to establish multi-hazard recovery-based design criteria. We anticipate that the process of development, including formulation, implementation, and evaluation, will be very similar and necessitate careful consideration of the various stakeholder perspectives. The social and economic considerations at each stage have already been identified as critical for resilient multi-hazard building design[96].
● Finally, within a market design perspective, one must think carefully about incentives to the supply side in order to encourage adoption in a voluntary system as in the US Incentives are a powerful tool to encourage first movers, as well as to support socially beneficial outcomes (such as prioritizing underserved communities) while targeting acceptable recovery times. Incentives could be especially powerful for critical lifelines, where best practices are being developed to inform sector-specific actions by private and public owners and operators[97]. However, the design of incentives may vary across communities and will require further research.
DECLARATIONS
Acknowledgments
These opinions, recommendations, findings, and conclusions do not necessarily reflect the views or policies of NIST or the United States Government.
Authors’ contributions
Made substantial contributions to the conception, literature review, and writing of the study and are actively involved in federal R & D efforts to advance functional recovery: Fung JF, Zhang Y, Johnson KJ, Cook DT, Sattar S
Availability of data and materials
Not applicable.
Financial support and sponsorship
None.
Conflicts of interest
All authors declared that there are no conflicts of interest.
Ethical approval and consent to participate.
Not applicable.
Consent for publication
Not applicable.
Copyright
© The Author(s) 2023.
REFERENCES
1. Ritchie H, Rosado P, Roser M. Our world in data: natural disasters. Available from: https://ourworldindata.org/natural-disasters. [Last accessed on 26 Jul 2023].
2. Munich Re. Natural disaster risks: rising trends in losses. Available from: https://www.munichre.com/en/risks/natural-disasters.html. [Last accessed on 26Jul 2023].
3. NOAA National Centers for Environmental Information (NCEI) US. Billion-dollar weather and climate disasters. Available from: https://www.ncei.noaa.gov/access/billions/. [Last accessed on 26 Jul 2023].
4. Sattar S, Kersting R, Arendt L, et al. Recommended options for improving the built environment for post-earthquake reoccupancy and functional recovery time. National Institute of Standards and Technology (NIST); 2021.
5. Zhang Y, Fung JF, Johnson KJ, et al. Review of seismic risk mitigation policies in earthquake-prone countries: lessons for earthquake resilience in the united states. J Earthq Eng 2022;26:6208-35.
6. United States Geological Survey (USGS). Nearly half of americans exposed potentially damaging earthquakes. Available from: https://www.usgs.gov/news/featured-story/nearly-half-americans-exposed-potentially-damaging-earthquakes. [Last accessed on 26 Jul 2023].
7. Fung J, Zhang Y, Johnson K, et al. A framework to evaluate the cost-effectiveness of recovery-based design. National Institute of Standards and Technology (NIST); 2022.
8. International Code Council (ICC). A national approach to seismic functional recovery for new construction: a roundtable discussion convened by the international code council and california building officials. 2019. Available from: http://media.iccsafe.org/2019_MarComm/Misc/19-17983_COMM_Seismic_RT_Report_FINAL_MIDrez.pdf. [Last accessed on 26 Jul 2023].
9. Panagioutou M. Earthquake resilience and functional recovery of buildings in the united states: problem statement and technical solutions. Available from: https://www.researchgate.net/publication/337290394_Earthquake_Resilience_and_Functional_Recovery_of_Buildings_in_the_United_States_Problem_Statement_and_Technical_Solutions. [Last accessed on26 Jul 2023].
10. Sattar S, McAllister T, Johnson KJ, et al. Research needs to support immediate occupancy building performance objective following natural hazard events. National Institute of Standards and Technology (NIST); 2018.
11. Rowan L. Hazard-Resilient Buildings: Sustaining occupancy and function after a natural disaster. Congressional Research Service (CRS); 2022. Available from: https://crsreports.congress.gov/product/pdf/R/R47215. [Last accessed on 26 Jul 2023].
12. Harris J, Johnson KJ, McCabe SL. ICSSC Biennial progress report for 2018: report on progress towards implementation of executive order 13717: establishing a federal earthquake risk management standard - reporting period: february 2, 2016 to february 1, 2018. National Institute of Standards and Technology (NIST); 2021.
13. National Institute of Standards and Technology (NIST). Community resilience program. Available from: https://www.nist.gov/programs-projects/community-resilience-program. [Last accessed on 26 Jul 2023].
14. Federal Emergency Management Agency (FEMA). Community. FEMA Glossary. 2020. Available from: https://www.fema.gov/glossary/community. [Last accessed on 26 July 2023].
15. Baek JS, Meroni A, Manzini E. A socio-technical approach to design for community resilience: a framework for analysis and design goal forming. Design Studies 2015;40:60-84.
17. Bruneau M, Chang SE, Eguchi RT, et al. A framework to quantitatively assess and enhance the seismic resilience of communities. Earthquake Spectra 2003;19:733-52.
18. ICC (International Code Council). 2018 International existing building code. Available from: https://codes.iccsafe.org/content/IEBC2018P4. [Last accessed on 26 Jul 2023].
19. ICC (International Code Council). 2018 international building code. Available from: https://codes.iccsafe.org/content/IBC2018. [Last accessed on 26 Jul 2023].
20. American Society of Civil Engineers (ASCE). Minimum Design Loads and Associated Criteria for Buildings and Other Structures, ASCE/SEI 7-16. 2017.
21. Federal Emergency Management Agency (FEMA). Guidelines for performance-based seismic design of buildings. FEMA; 2018. Available from: https://peer.berkeley.edu/sites/default/files/tbi-working-group-led-by-co-chairs-ron-ha-burger-and-jack-moehle_10.9.17_withlinks.pdf. [Last accessed on 26 Jul 2023].
22. Cook D, Sattar S. The effect of increased strength and stiffness requirements on the functional recovery performance of reinforced concrete special moment frames. Proceedings of the 12th National Conference on Earthquake Engineering, Earthquake Engineering Research Institute, Salt Lake City, UT; 2022.
23. Kersting RA, Fahnestock LA, Lopez WA. Seismic design of steel buckling-restrained braced frames: a guide for practicing engineers. National Institute of Standards and Technology (NIST); 2016.
24. Cook D, Sattar S, and Johnson KJ (2023). A framework to develop minimum prescriptive design requirements to meet recovery-based design goals. National Institute of Standards and Technology, Gaithersburg, Maryland. In Preparation.
25. Gebelein J, Barnard M, Burton H, et al. Considerations for a framework of resilient structural design for earthquakes. SEAOC Convention Proceedings, 2017. Available from: http://static-assets.hbrisk.com.s3.amazonaws.com/presentations/SEAOC+2017+Considerations+for+a+Framework+of+Resilient+Structural+Design+for+Earthquakes.pdf. [Last accessed on 26 Jul 2023]
26. Sullivan TJ. Post-earthquake reparability of buildings: the role of non-structural elements. Struct Eng Int 2020;30:217-23.
27. Yeow TZ, Orumiyehei A, Sullivan TJ, et al. Seismic performance of steel friction connections considering direct-repair costs. Bull Earthquake Eng 2018;16:5963-93.
28. Hooper J, Holmes W, Moehle J, et al. Development of NIST measurement science r&d roadmap: earthquake risk reduction in buildings. National Institute of Standards and Technology (NIST); 2013. Available from: https://tsapps.nist.gov/publication/get_pdf.cfm?pub_id=915478. [Last accessed on 26 Jul 2023].
29. Applied Technology Council (ATC). Seismic analysis, design, and installation of nonstructural components and systems - background and recommendations for future work. National Institute of Standards and Technology (NIST); 2017.
30. Applied Technology Council (ATC). Recommendations for improved seismic performance of nonstructural components. National Institute of Standards and Technology (NIST); 2017.
31. California Department of Health Care Access and Information (HCAI). OSHPD special seismic certification preapproval (OSP). Available from: https://hcai.ca.gov/construction-finance/preapproval-programs/oshpd-special-seismic-certification-preapproval-osp/. [Last accessed on 26 Jul 2023].
32. Tasligedik AS, Pampanin S. Rocking cantilever clay brick infill wall panels: a novel low damage infill wall system. J Earthq Eng 2017;21:1023-49.
33. Mulligan J, Sullivan TJ, Dhakal RP. Experimental seismic performance of partly-sliding partition walls. J Earthq Eng 2022;26:1630-55.
34. Araya-letelier G, Miranda E, Deierlein G. Development and testing of a friction/sliding connection to improve the seismic performance of gypsum partition walls. Earthquake Spectra 2019;35:653-77.
35. Pourali A, Dhakal RP, Macrae G, Tasligedik AS. Fully floating suspended ceiling system: experimental evaluation of structural feasibility and challenges. Earthquake Spectra 2017;33:1627-54.
36. Bianchi S, Ciurlanti J, Pampanin S. Comparison of traditional vs low-damage structural and non-structural building systems through a cost/performance-based evaluation. Earthquake Spectra 2021;37:366-85.
37. Earthquake Engineering Research Institute (EERI). Functional recovery: a conceptual framework with policy options. 2019. Available from: https://www.eeri.org/images/policy/EERI-Functional-Recovery-Conceptual-Framework-White-Paper-201912.pdf. [Last accessed on 26 Jul 2023].
38. Seismic Performance Prediction Platform (SP3). Announcing the new ATC-138 functional recovery (beta) method! Available from: https://sp3risk.com/2022/01/21/announcing-the-new-atc-138-functional-recovery-beta-method/. [Last accessed on 26 Jul 2023].
39. American Lifelines Alliance (ALA). Protecting our critical infrastructure: findings and recommendations from the american lifelines alliance roundtable. National Institute of Building Sciences (NIBS); 2005. Available from: https://www.americanlifelinesalliance.com/pdf/RoundtableReportWebPosted081705.pdf. [Last accessed on 26 Jul 2023].
40. Bilal M. Infrastructure Resilience Division of ASCE. Hazard-resilient infrastructure: analysis and design. Reston, VA: American Society of Civil Engineers; 2021.
41. Applied Technology Council (ATC). Proceedings of FEMA-sponsored workshop on functional recovery. 2022. ATC 58-7. Available from: https://www.atcouncil.org/docman/atc-publications/327-atc-58-7-fr-workshop-report-2022-2/file. [Last accessed on 26 Jul 2023]
42. International Code Council (ICC). Seismic functional recovery: resources. Available from: https://www.iccsafe.org/advocacy/seismic-functional-recovery-resources/. [Last accessed on 26 Jul 2023].
43. American Society of Civil Engineers (ASCE). Minimum design loads and associated criteria for buildings and other structures, ASCE/SEI 7-22. Available from: https://ascelibrary.org/doi/book/10.1061/9780784415788. [Last accessed on 26 Jul 2023].
44. American Society of Civil Engineers (ASCE). Seismic evaluation and retrofit of existing buildings, ASCE/SEI 41-17. Available from: https://ascelibrary.org/doi/book/10.1061/9780784414859. [Last accessed on 26 Jul 2023].
45. ACI Committee 318. 318-19(22): building code requirements for structural concrete and commentary (reapproved 2022). Available from: https://www.concrete.org/publications/internationalconcreteabstractsportal.aspx?m=details&ID=51716937. [Last accessed on 26 Jul 2023].
46. American Institute of Steel Construction. Seismic provisions for structural steel buildings. Available from: https://www.aisc.org/globalassets/aisc/publications/standards/seismic-provisions-for-structural-steel-buildings-ansi-aisc-341-16.pdf. [Last accessed on 26 Jul 2023].
47. American Institute of Steel Construction. Specification for structural steel buildings. Available from: https://www.aisc.org/globalassets/aisc/publications/standards/a360-16w-rev-june-2019.pdf. [Last accessed on 26 Jul 2023].
48. American Wood Council. 2021 SDPWS. Available from: https://awc.org/publications/2021-sdpws/. [Last accessed on 26 Jul 2023].
49. American Wood Council. 2018 NDS. Available from: https://awc.org/publications/2018-nds/. [Last accessed on 26 Jul 2023].
50. The Masonry Society. TMS 402/602-22 building code requirements and specification for masonry structures. Available from: https://masonrysociety.org/product/tms-402-602-22/. [Last accessed on 26 Jul 2023].
51. FEMA. Reducing the risks of nonstructural earthquake damage-a practical guide. Available from: https://www.fema.gov/sites/default/files/2020-07/fema_earthquakes_reducing-the-risks-of-nonstructural-earthquake-damage-a-practical-guide-fema-e-74.pdf. [Last accessed on 26 Jul 2023].
52. American Society of Civil Engineers (ASCE). Structural applications of steel cables for buildings. Available from: https://ascelibrary.org/doi/book/10.1061/9780784414392. [Last accessed on 26 Jul 2023].
53. Vibration Isolation and Seismic Control Manufacturer’s Association (VICSMA). Seismic restraint specification guidelines for mechanical, electrical and plumbing systems. VISCMA 101-15. Available from: https://viscma.com/wp-content/uploads/VISCMA_101-15_Seismic_Restraint_Specification_Guidelines_for_Mechanical_Electrical_and_Plumbing_Systems.pdf. [Last accessed on 26 Jul 2023].
54. Vibration Isolation and Seismic Control Manufacturer’s Association (VICSMA). Vibration isolation specification guidelines for mechanical, electrical and plumbing systems. VISCMA 102-12. Available from: https://viscma.com/wp-content/uploads/VISCMA_102-12_Vibration_Isolation_Specification_Guidelines_for_Mechanical_Electrical_and_Plumbing_Systems.pdf. [Last accessed on 26 Jul 2023].
55. ASTM International. C635/C635M-22: Standard specification for manufacture, performance, and testing of metal suspension systems for acoustical tile and lay-in panel ceilings. Available from: https://www.astm.org/c0635_c0635m-22.html. [Last accessed on 26 Jul 2023].
56. ASTM International. C636/C636M-19: Standard practice for installation of metal ceiling suspension systems for acoustical tile and lay-in panels. Available from: https://www.astm.org/c0636_c0636m-19.html. [Last accessed on 26 Jul 2023].
57. ASTM International. E580/E580M-22: Standard practice for installation of ceiling suspension systems for acoustical tile and lay-in panels in areas subject to earthquake ground motions. Available from: https://www.astm.org/e0580_e0580m-22.html. [Last accessed on 26 Jul 2023].
58. American Society of Mechanical Engineers (ASME). A17.1/CSA B44-2016: Handbook on safety code for elevators and escalators. Available from: https://www.asme.org/codes-standards/find-codes-standards/a17-1-csa-b44-handbook-safety-code-elevators-escalators. [Last accessed on 26 Jul 2023].
59. National Fire Protection Association (NFPA). NFPA 13: Standard for the installation of sprinkler systems. Available from: https://www.nfpa.org/codes-and-standards/all-codes-and-standards/list-of-codes-and-standards/detail?code=13. [Last accessed on 26 Jul 2023].
60. ASTM International. E2506-15(2020)e1: Standard guide for developing a cost-effective risk mitigation plan for new and existing constructed facilities. Available from: https://www.astm.org/e2506-15r20e01.html. [Last accessed on 26 Jul 2023].
61. Federal Emergency Management Agency (FEMA). FEMA BCA toolkit 6.0 installation instructions. Available from: https://www.fema.gov/fact-sheet/fema-bca-toolkit-60-installation-instructions. [Last accessed on 27 Jul 2023].
62. Zhang Y, Fung JF, Cook D, et al. Benefit-cost analysis for earthquake resilient building design and retrofit: the state of the art and future research needs. Under review 2023.
63. Abrahams L, Van Pay L, Sattar S, et al. NIST-FEMA post-earthquake functional recovery workshop report. National Institute of Standards and Technology (NIST); 2021.
64. Fung JF, Cook DT, Zhang Y, et al. Economic considerations for recovery-based design. Proceedings of The 12th National Conference in Earthquake Engineering, Earthquake Engineering Research Institute, Salt Lake City, Utah; 2022.
65. Sobhaninia S, Buckman ST. Revisiting and adapting the Kates-Pijawka disaster recovery model: a reconfigured emphasis on anticipation, equity, and resilience. Int J Disast Risk Re 2022;69:102738.
66. Burke A. The stages of policy development. Available from: https://openoregon.pressbooks.pub/ccj230/chapter/3-3-the-stages-of-policy-development/. [Last accessed on 27 Jul 2023].
67. Kolozvari K, Terzic V. Methodology for developing practical recovery-based design requirements for buildings. Eng Struct 2023;274:115102.
68. Johnson KJ, Sattar S, Segura C, et al. Lessons on recovery of function from Anchorage, Alaska after the 2018 cook inlet earthquake. Available from: https://tsapps.nist.gov/publication/get_pdf.cfm?pub_id=929893. [Last accessed on 27 July 2023]
69. Tsu VD, LaMontagne DS, Atuhebwe P, et al. National implementation of HPV vaccination programs in low-resource countries: lessons, challenges, and future prospects. Prev Med 2021;144:106335.
70. Sattar S, Segura C, Johnson KJ, et al. Building design considerations to support immediate occupancy performance objectives. 12th Canadian Conference on Earthquake Engineering, Quebec, Canada; 2019. Available from: https://tsapps.nist.gov/publication/get_pdf.cfm?pub_id=927374. [Last accessed on 27 Jul 2023]
71. Molina Hutt C, Hulsey AM, Kakoty P, et al. Toward functional recovery performance in the seismic design of modern tall buildings. Earthquake Spectra 2022;38:283-309.
72. Advisory Committee on Earthquake Hazards Reduction (ACEHR). Effectiveness of the national earthquake hazards reduction program: a report from the advisory committee on earthquake hazards reduction. 2019. Available from: https://nehrp.gov/pdf/September_2019_ReporttotheNISTDirector.pdf. [Last accessed on 27 Jul 2023].
73. International Organization for Standardization (ISO). ISO 26000 and OECD guidelines-practical overview of the linkages. Available from: https://www.iso.org/publication/PUB100418.html. [Last accessed on 27 Jul 2023].
75. Kominers SD, Teytelboym A, Crawford VP. An invitation to market design. Oxford Rev Econ Pol 2017;33:541-71.
76. Bocchini P, Frangopol DM. Optimal resilience- and cost-based postdisaster intervention prioritization for bridges along a highway segment. J Bridge Eng 2012;17:117-29.
77. Zhang W, Nicholson C. A multi-objective optimization model for retrofit strategies to mitigate direct economic loss and population dislocation. Sustain Resil Infras 2016;1:123-36.
78. Zhang J, Li G, Zhang M. Multi‐objective optimization for community building group recovery scheduling and resilience evaluation under earthquake. Computer aided Civil Eng 2022;38:1657-76.
80. Chakravarty SR. Equity and efficiency as components of a social welfare function. Int J Econ Theory 2009;5:181-99.
81. Fleurbaey, Marc. Normative economics and economic justice. The Stanford Encyclopedia of Philosophy (Spring 2022 Edition). Available from: https://plato.stanford.edu/archives/spr2022/entries/economic-justice/. [Last accessed on 27 Jul 2023].
82. Singh RR, Bruneau M, Stavridis A, Sett K. Resilience deficit index for quantification of resilience. Resilient Cities and Structures 2022;1:1-9.
83. Zhan S, Chang-Richards A, Elwood K, et al. Post-earthquake functional recovery: a critical review. Proceedings of the 2022 New Zealand Society for Earthquake Engineering Annual Technical Conference. Available from: http://13.237.132.70/handle/nzsee/2507. [Last accessed on 27 Jul 2023]
84. McAllister T. Community resilience planning guide for buildings and infrastructure systems, Volume I. National Institute of Standards and Technology (NIST); 2015.
85. Gilbert SW, Butry DT, Helgeson JF, Chapman RE. Community resilience economic decision guide for buildings and infrastructure systems. National Institute of Standards and Technology (NIST); 2015.
86. Helgeson, J, P Lavappa, and D Webb. EDGe$ (Economic Decision Guide Software) online tool, Version 1.0, National Institute of Standards and Technology (NIST). Available from: https://data.commerce.gov/edge-economic-decision-guide-software-online-tool-version-10. [Last accessed on 27 Jul 2023.
88. Prewitt Diaz JO, Dayal A. Sense of place: a model for community based psychosocial support programs. Australasian Journal of Disaster And Trauma studies 2008;63(8);820-827.Available fron: https://www.massey.ac.nz/~trauma/issues/2008-1/prewitt_diaz.htm. [Last accessed on 27 Jul 2023].
89. Spoon J, Hunter CE, Gerkey D, et al. Anatomy of disaster recoveries: tangible and intangible short-term recovery dynamics following the 2015 Nepal earthquakes. Int J Disast Risk Re 2020;51:101879.
90. Ghosh SK. Is seismic design by US codes and standards deficient? Structure 2019. Available from: https://www.structuremag.org/?p=14725. [Last accessed on 27 Jul 2023].
91. Tokas CV. Nonstructural components and systems-designing hospitals for post earthquake functionality. Available from: https://ascelibrary.org/doi/10.1061/41168%28399%2945.[Last accessed on 7 Sep 2023]
92. Bilginsoy Z, Fraser S. Turkey’s lax policing of building codes known before quake. AP News 2023. Available from: https://apnews.com/article/politics-2023-turkey-syria-earthquake-government-istanbul-fbd6af578a6056569879b5ef6c55d322. [Last accessed ON 27 Jul 2023].
93. Hurwiz L. Implementation and enforcement in institutional modeling. In: Barnett WA, Hinich MJ, Schofield NJ, editors. International Symposium in Economic Theory and Econometrics. Cambridge University Press; 1993.
94. Cerna L. The nature of policy change and implementation: a review of different theoretical approaches. Organisation for Economic Development (OECD) 2013. Available from: https://www.oecd.org/education/ceri/The%20Nature%20of%20Policy%20Change%20and%20Implementation.pdf. [Last accessed on 27 Jul 2023].
95. Amirzadeh M, Sobhaninia S, Sharifi A. Urban resilience: a vague or an evolutionary concept? Sustain Cities Soc 2022;81:103853.
96. Johnson KJ, Fung JF, McAllister T, et al. Social and economic components of resilient multihazard building design. Nat Hazards Rev 2020:21(1).
97. Scott DR, Cerino AC, Pekelnicky RG, Yu K. Resilience for critical facitilies. Available from: https://nvlpubs.nist.gov/nistpubs/gcr/2023/NIST.GCR.23-037.pdf.[Last accessed on 7 Sep 2023]
Cite This Article
Export citation file: BibTeX | EndNote | RIS
OAE Style
Fung JF, Zhang Y, Johnson KJ, Cook DT, Sattar S. Multidisciplinary research to advance the development of functional recovery for community resilience. Dis Prev Res 2023;2:13. http://dx.doi.org/10.20517/dpr.2023.15
AMA Style
Fung JF, Zhang Y, Johnson KJ, Cook DT, Sattar S. Multidisciplinary research to advance the development of functional recovery for community resilience. Disaster Prevention and Resilience. 2023; 2(3): 13. http://dx.doi.org/10.20517/dpr.2023.15
Chicago/Turabian Style
Juan F. Fung, Yating Zhang, Katherine J. Johnson, Dustin T. Cook, Siamak Sattar. 2023. "Multidisciplinary research to advance the development of functional recovery for community resilience" Disaster Prevention and Resilience. 2, no.3: 13. http://dx.doi.org/10.20517/dpr.2023.15
ACS Style
Fung, JF.; Zhang Y.; Johnson KJ.; Cook DT.; Sattar S. Multidisciplinary research to advance the development of functional recovery for community resilience. Dis. Prev. Res. 2023, 2, 13. http://dx.doi.org/10.20517/dpr.2023.15
About This Article
Copyright
Data & Comments
Data
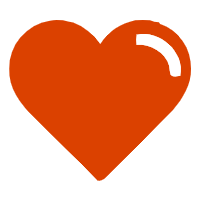
Comments
Comments must be written in English. Spam, offensive content, impersonation, and private information will not be permitted. If any comment is reported and identified as inappropriate content by OAE staff, the comment will be removed without notice. If you have any queries or need any help, please contact us at support@oaepublish.com.